ABSTRACT
Since its discovery the cellular prion protein (encoded by the Prnp gene) has been associated with a large number of functions. The proposed functions rank from basic cellular processes such as cell cycle and survival to neural functions such as behavior and neuroprotection, following a pattern similar to that of Moore's law for electronics. In addition, particular interest is increasing in the participation of Prnp in neurodegeneration. However, in recent years a redefinition of these functions has begun, since examples of previously attributed functions were increasingly re-associated with other proteins. Most of these functions are linked to so-called “Prnp-flanking genes” that are close to the genomic locus of Prnp and which are present in the genome of some Prnp mouse models. In addition, their role in neuroprotection against convulsive insults has been confirmed in recent studies. Lastly, in recent years a large number of models indicating the participation of different domains of the protein in apoptosis have been uncovered. However, after more than 10 years of molecular dissection our view is that the simplest mechanistic model in PrPC-mediated cell death should be considered, as Ockham's razor theory suggested.
KEYWORDS:
IS THERE PLENTY OF ROOM AT THE BOTTOM OF PrPC?
“There's Plenty of Room at the Bottom” was a lecture given by physicist Richard Feynman at the American Physical Society meeting at Caltech in December 1959. He was particularly interested in the possibility of increasing computer circuitry especially in ultramicroscopes to achieve higher resolution than the electron microscopes of the time. In fact, Feyman´s talk is considered for many researchers the starting point of modern nanotechnology. The talk was republished in the 1990s, 25 years after Gordon Moore, director of research and development at Fairchild Semiconductors and co-founder of IBM, hypothesized in Electronics Magazine a doubling every year in the number of components per integrated circuit (later called Moore's Law). In fact, Moore's view of electronics also expanded to molecular biology when Rob Carlson predicted in 2003 a hypothetical increase in DNA sequencing capabilities (measured by cost and performance) with similar doubling to that of Moore's law (the Carlson curve, published by The Economist, August 31, 2006). However, Moore foresaw in March, 2015 that the rate of circuit progress would reach saturation: ”I guess I see Moore's law dying here in the next decade or so, but that's not surprising!.“ We have the same perception, with the inclusion of new circuits in a finite space reaching saturation point. In fact, this saturation seemed to have started in 2011 ( obtained from https://commons.wikimedia.org/wiki/User:Wgsimon). In addition, the Carlson curve was rendered outdated in 2008 with the development of the new DNA sequencing technologies.
FIGURE 1. Evolution of Moore's law from 1971 to 2011. Source: https://commons.wikimedia.org/wiki/User:Wgsimon through Creative Commons Attribution-ShareAlike License (https://creativecommons.org/licenses/by-sa/3.0/).
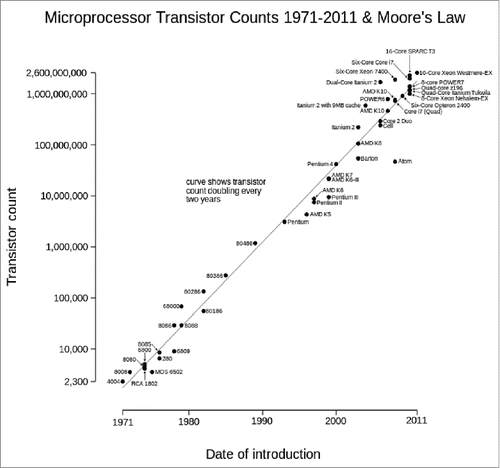
Now, we need to move in this commentary from theoretical electronics to neuroscience, and in particular to prion biology. Numerous manuscripts dealing with PrPC begin with sentences similar to these: “The physiological function of the prion protein is not yet known” or “PrPC plays a key role in the pathogenesis of prion diseases, but its physiologic function remains unclear.” This occurs simply as a result of the published descriptions, since we would be astonished with the “terrific” number of distinct cellular processes linked to the cellular prion protein (a GPI-anchored protein with a finite molecular space of 231 residues): cell survival and differentiation,Citation1,2 oxidative stress,Citation3,4 copper homeostasis,Citation5,6 cell proliferationCitation7,8 and cell-cell signalingCitation9,10 are all fully associated with or participated in by PrPC. In fact, most of these functions were deduced or supported by experiments using as experimental model one of first generated Prnpo/o mice: the Zurich I mouse.Citation11 Using this mouse, the first studies were directed to clearly determine that Prnp expression is mandatory to prion infection and propagation (e.g.Citation12). But the few functional alterations initially described in Zurich I were complemented by Edinburgh Prnpo/o mice, the second model generated around this time.Citation13 However, since 1992, sequentially published studies have identified a large number of phenotypic effects of the absence of Prnp including depressive-like behavior,Citation14,15 cognitive deficits,Citation16 peripheral myelin deficits,Citation17 age-dependent behavioral abnormalities,Citation18 altered olfaction,Citation19 altered circadian rhythms,Citation13 altered associational learning,Citation20 altered sleep recovery,Citation21 altered increased susceptibility to oxidative stress,Citation3 increased excitotoxicityCitation22-25 and altered neural stem cell proliferation.Citation1,26 The descriptions of new functions almost followed the slope of the Moore's law but in some cases with controversy (see refs. Citation27 and Citation28 for details) (see Appendix for some examples). For a GPI-anchored protein, the proposed list of functions seemed to be at least disproportionate. Relevantly, last year functional descriptions reached a plateau and some functions started to be re-assigned to other proteins after careful re-evaluation of the role of PrPC in these processes. In this respect, one putative explanation is to consider that some of these functions are not directly mediated by PrPC and might depend on extracellular or intracellular partners of the protein. This may be the case for some functions, but while other extracellular interactions and their physiological relevance are well established (for example with adhesion molecules,Citation29,30 lipoprotein receptors,Citation31 laminin receptor,Citation32,33 amyloid proteins,Citation34 and metallic ionsCitation5), the intracellular partners linked to the GPI-binding protein PrPC are also numerous: anti-apoptotic proteins,Citation35 cytoskeletal proteins,Citation36,37 enzymes,Citation34 and synaptic proteins.Citation38,39 For some of them clear biological relevance is still unknown, warranting further study.Citation27,40 Taking into account that “dubium sapientiae initium,” as Descartes had it, researchers cannot state the functions or the particular role of a single protein in a specific physiological event taking as information source analysis of a transgenic mouse. In this commentary, we will summarize the current knowledge of some predicted functions of PrPC, especially related to its putative participation in synaptic plasticity, neuroprotection, and neurodegeneration.
ZÜRICH I KNOCKOUT MICE AND THEIR PITFALLS
Revealing evidence indicates that PrPC is not the main actor for some of the above-mentioned functions in B6129 PrnpZrchl/Zrchl knockout mice. In fact, a number of Prnp-flanking genes associated with the 129/Sv genotype in B6129 mixed mice have been described in Zurich I mice.Citation41 A ratio of 60% to 2% of 129/Sv specific markers between Prnp0/0 and Prnp+/+ mice was determined.Citation42 These genes were introduced during the generation of the transgenic mice and are retained in Prnpo/o progeny of congenic B6.129 PrnpZrchl/Zrchl after numerous (>10 –15) crosses of B6129 Prnp0/0 with C57BL/6 mice.Citation26,41 In a recent studyCitation43 we determined, using a commercially available SNP analysis, that in backcrossed mice (5 to 6 rounds) enrichment of the C57BL/6-associated SNPs increased from ≈60% to ≈93% in the progeny. Thus, B6.129 PrnpZrchl/Zrchl wild type and mutant mice may still differ at these (≈6–7%) additional 129 polymorphic loci (i.e.: Mmu2 genomic region close to Prnp) accidentally present in Prnp0/0.Citation44,45 This presence and parallel effects were suggested by Steele and co-workers in a seminar review published in this journal in 2007.Citation28 But one of the first demonstrations of the putative unwanted effects due to the presence of polymorphic 129 regions was indicated by A. Aguzzi's lab in 2010, when analyzing the effects of Mfge8 ablation in prion infection and disease evolution in Prnpo/o mice.Citation46 In the study, the absence of Mfge8 in a B6.129 background increases the appearance of prion disease after inoculation, in contrast to C57BL/6 Mfge80/0 inoculated mice.Citation46 In addition, Calella and coworkers described quantitatively the changes in the relative percentage of these loci after crossing.Citation42 Three years later, one identified gene, the signal regulatory protein α (SIRPα), was described for the first time as being responsible for a previously PrPC-associated phagocytic function in macrophages.Citation45 These masking functions associated with this locus are especially relevant if we take into account that Prnp-overexpressing mice (Tg20) generated in mixed B6129 PrnpZrchl/Zrchl background carry several copies of the polymorphic loci, since in most cases they are also crossed with previously backcrossed B6.129 PrnpZrchl/Zrchl mice.Citation41 In this scenario, it is reasonable to assume that the data obtained using Prnpo/o or Tg20 in physiological studies (from genomic to electrophysiological) may render conflicting results, as observedCitation22,23,43,47-56 (see alsoCitation28). However, these side effects in an undetectable manner might also occur in other mouse models with overexpressed modified forms of PrPC using the Prnp null-background of the Zurich I mice.Citation17,57,58,59 In fact, at the transcriptional level, comparison of the mRNA expression profile reported in 2 studies revealed that B6.129 and FVB/N Prnp0/0 mice share only very few overexpressed genes in the adult hippocampus.Citation54,55 This result, as suggested,Citation55,60 might reflect, among other possibilities i) direct transcriptional effects of the absence of Prnp on different genetic backgrounds, or ii) a summation of changes linked to the deficiency of functional PrPC together with the side effects linked to the 129-associated loci in the strains used in these studies: B6.129 Prnp0/0 (B6129 backcrossed with C57BL/6 mice for 15 generationsCitation54 and FVB/N mice (B6129 backcrossed with FVB/N > 20 generations).Citation55 In fact, some of these 129-associated loci very close to Prnp could be detected after ≈20 backcrossings in B6.129 mice (A. Aguzzi personal communication).
The number of these “Prnp-flanking genes” in the B6129 knockout mouse compared to C57BL6/J is large as demonstrated by A. Aguzzi's lab.Citation41 Thus, a re-evaluation of the published functional data concerning PrPC is mandatory in order to clarify this and to delineate PrPC functions in neural and non-neural tissues. Alternatively, researchers may study these putative functions in different biological systems in more controlled models far from the mixed mice. Indeed, in a recently published study we dissected the participation of these 129/Sv-associated genes in neurotransmission and kainate-induced cell death in different mouse strains and in vitro.Citation43
INVOLVEMENT OF PrPC IN NEUROTRANSMISSION AND NEURAL PLASTICITY: A PUZZLE BEING CLARIFIED A STEP AT A TIME
Different laboratories have described, in several Prnp0/0 strains including congenic B6.129 PrnpZrchl/Zrchl, enhanced sensitivity to seizures after the administration of epileptogenic drugs such as kainic acid (kainate, KA), N-methyl-d-aspartic acid (NMDA), pilocarpine, and pentylenetetrazol (PTZ), suggesting a neuroprotective role of PrPC against excitotoxic convulsive insults (e.g.,Citation22-25,40,53). However, other studies have suggested that PrPC is not involved in KA-mediated excitotoxicity and that the observed differences between wild type and Prnp-deficient mice are associated with the genetic background of the mice used in the experiments.Citation51,52 In addition, an electrophysiological study indicated that PrPC-expressing neurons in vitro are more resistant against 3 different convulsive treatments (including PTZ).Citation50 However, this last observation, obtained using crossed B6129 + FVB mice, was not expected based on previously published data by the same group using B6129 Prnp0/0 mice.Citation61
Can we determine the role of PrPC in KA-mediated neurotoxicity in a region such us the hippocampus by using different mice strains? Our answer is affirmative, although the cytotoxic effects of KA in the mouse hippocampus are strongly strain-dependent.Citation62-67 Following intraperitoneal KA administration, the large majority of pyramidal neurons of the hippocampus die in the FVB/N (FVB) mouse, while the pyramidal neurons of the C57BL/6 strain remain largely healthy.Citation62-67 Thus it is reasonable to consider that the intrinsic background might play a role in the results reported by Ratte and coworkers.Citation50 Obviously these genetic differences may mask or dilute the participation of Prnp in KA-mediated neurotoxicity. In our study we were unable to identify specific participation of PrPC in KA-treated FVB/N Prnp0/0 and FVB/N Prnp+/+ mice.Citation43 In contrast, in B6129 and B6.129 Prnp0/0, KA-mediated effects (cell death, astrogliosis, and increased presence of pro-inflammatory molecules) were identifiable, as previously reportedCitation23,53 and corroborated using 129/Ola Prnp0/0 miceCitation43 (whithout Prnp-flanking genes). In addition, these genomic influences do not explain per se the neuroprotective properties of PrPC observed in KA-treated neuroblastoma cell lines carrying different dosages of the Prnp gene.Citation43,53 In addition, regulatory participation of PrPC in neurotransmission and neuroprotection, and in other cellular functions, has also been demonstrated with acute modulation of Prnp expression in neural cell lines (seeCitation68) and in other organisms (e.g., zebrafish).Citation69 We believe that functions of this protein in neurotransmission and neuroprotection are currently supported by i) their binding to glutamate receptor subunits (e.g.,, GluR6/7, NR2D, GluR1/2, mGluR1/5),Citation24,53,70,71 ii) their binding to ion channels,Citation49 iii) their regulation of GluR6/7- and NR2D-mediated signaling,Citation53 and iv) a recently published observation indicating that PrPC and copper cooperatively inhibit NMDA receptor through S-nitrosylation enhancing neuroprotection.Citation25 However, if we compare the number and level of the convulsive seizures between B6129 and B6.129 Prnp0/0 mice, a clear decrease in the seizure level can be seen in parallel to a decrease in the 129-associated loci between B6129 and B6.129 mice. Using this approximation we may assume that not only PrPC but also unidentified 129/Sv-associated gene/s contribute to the KA-mediated sensitivity observed in B6129 Prnp0/0 mice. Moreover, B6129 Prnp0/0 mice overexpressing truncated forms of PrPC showed additional increased degeneration that B6129 Prnp0/0 mice suggesting that susceptible neurons become highly reactive to cellular stress induced by KA, indicating that specific domains of the protein may play a role in triggering cell death in certain physiological and non-physiological conditions.
MOLECULAR DISSECTION OF THE PARTICULAR FUNCTIONS OF PrPC IN CELL DEATH: LESSONS FROM TRUNCATED FORMS, ANTIBODIES, AND PEPTIDES
The sequence of PrPC can be divided into 2 structurally well-defined regions: a long, flexible N-terminal flexible tail (approximately the first 100 residues) and a globular C-terminal domain containing 3 α-helices and 2 β-strands flanking the first α-helix (). The flexible tail also has distinctive features: a small charged region (CC1), an octarepeat (OR) region, and a central domain (CD), which in turn comprises a second charge cluster (CC2) and a hydrophobic region (HR). In order to demonstrate that the N-terminal domain is mainly responsible for cooper-binding in anti-oxidative protection, Zeng and co-workers demonstrated that when the N-terminal domain of PrPC is tethered to the plasma membrane, this modified PrPC largely compromised cell survival due to the resulting inability to control cellular stress.Citation72 In fact, by using other methods (antibody treatment) it has been determined that the proximity of the flexible tail of the protein to the cellular membrane leads to cell death by activating reactive oxygen species (ROS) generation.Citation73,74 This has also been indicated by using transgenic mice.Citation59 Surprisingly, the location of cell death and its timing in the recently described FTgpi155 mouse (lacking 141 to 225 residues)Citation59 are rather similar to what is reported in other mice lacking domains of PrPC: i.e., ΔF35.Citation75 This anatomical correlation of cell death relies on the stronger activity of the Prnp promoter in cerebellar granule cells in these mice. However, ΔF35 mediated cell death cannot be reverted by overexpressing anti-apoptotic molecules, indicating that both caspase 3 and non-caspase 3 directed mechanisms are mediating ΔF35-associated cell death. Thus, overexpressing truncated forms of the protein in cultured cells could be also an alternative approach to determining the functions of particular domains of PrPC in cytotoxicity. In our studies, we determined that the expression of ΔF35 and ΔCD in neuroblastoma (N2a) cells is cytotoxic and activates Caspase 3, thereby corroborating previous resultsCitation76 (see alsoCitation68). However, only ΔCD was able to increase ROS production in transfected cells. This correlates with results using FTgpi155 mice, since in both cases the flexible tail (containing the OR) is close to plasma membrane. As indicated above, the FTgpi155 mice showed similar degeneration to ΔF35 mice; however ΔC4 mice (lacking) do not display cerebellar degeneration but are more susceptible to ischemic insults with increased ROS generation.Citation77 Concerning the effects of antibodies we need to consider several scenarios. First, antibodies (e.g., SAF61, recognizing residues 142 to 160 of PrPC) are able to aggregate PrPC in the plasma membrane, activating fyn at lipid rafts and triggering cell death.Citation9 In this process the activity of ERK1/2 and NADPH oxidase plays a crucial role.Citation78,79 Indeed, the use of similar antibodies against PrPC in vivo also leads to cell death.Citation80 The antibodies used in the in vivo experiments were IgG P and IgG D13 (recognizing residues 95 to 105 region of the PrPC). Second, antibodies directed to the globular domain are able to induce cell death in vitro and in vivo independently of PrPC aggregation.Citation73,74 In contrast, the injection of the ICSM35 antibody (recognizing PrPC epitopes 93 to 105) and ICSM18 (recognizing residues 143 to 153 of PrPC) failed to induce cell death in the hippocampus of C57BL10 mice.Citation81 In fact, it has been observed that the use of antibodies (POM1) directed to the globular domains triggers similar neurotoxic responses to those of pathogenic prion by approaching the flexible N-terminal domain to the plasma membrane.Citation74
FIGURE 2. (A) Domain organization of PrPC (mouse sequence). (B) Examples of some derived truncated forms used in in vitro and in vivo studies. The effects of their transfection in cells are indicated from (++) strong effect to negligible (−) effects in apoptosis. The examples of truncated PrPC forms are summarized from.Citation68,94,106,107 Results obtained in several studies reinforced data obtained by D.R. Brown's Lab in 2003.Citation108(C) Epitope mapping of some antibodies used in cytotoxicity studies. The name and recognized PrPC region is indicated in each case. Green antibodies indicate that their use is non-cytotoxic in contrast to red antibodies.
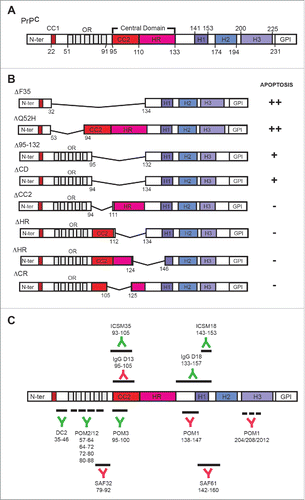
Some years ago, the use of synthetic peptides was revealed as a new alternative for analyzing prion neurotoxicity.Citation82 In fact one of the most widely used peptides of PrP(106–126) with aggregating properties needed the terminal half of the HR region but not the OR in order to be neurotoxic.Citation83 This result was in line with a previously published manuscript indicating that this peptide is able to induce ROS production in cultured neurons,Citation84 which could also be increased by overexpressing PrPC.Citation85 In fact, mice lacking the aa 105–125 of PrPC showed early death during the postnatal period.Citation86 Thus, although the existence of a survival signal interacting at this level or PrPC to trigger neuroprotective signals could not be ruled out, one should be tempted to strongly consider Ockham's razor theory in this scenario: “Among competing hypotheses, the one with the fewest assumptions should be selected.” It may be that these antibodies acting on the globular domain, the infective prion, or the mimicking peptides are blocking the intrinsic activity of the N-terminal domain, as happens when the domain is approached or tethered to the plasma membrane. Thus, the homeostatic cellular function of PrPC could be lost, triggering a cell-death mechanism (). However, we should forget that other perspective was offered by Walter Chatton: “If 3 things are not enough to verify an affirmative proposition about things, a fourth must be added, and so on.” The coming years and experiments will reveal whether we should apply Ockham's razor, or not, concerning PrPC-mediated cell death.
FIGURE 3. Scheme illustrating the effect of the expression of particular truncated forms of PrPC (B-E), treatment with GD-directed antibodies (F), peptides recognizing the CD region (G), aggregating antibodies recognizing GD and OR regions (H), and pathogenic prion protein (I). Absence of the OR in B and C leads to increased apoptosis. In contrast, PrPC lacking the CD but more relevantly lacking both the GD and the CD induced increased neurotoxicity. In contrast, aggregating antibodies (H), GD-directed antibodies (F), peptides (G), and the pathogenic prion (I) lead to profound changes in the 3D organization of PrPC in the membrane, which triggers the approach of the N-terminal region to the plasma membrane (red curved arrow) leading to increased ROS production and cell death as observed in PrPC constructs with artificial tethering of the N-terminal to the membrane. In these conditions, PrPC recycling is very low and their homeostatic function is lost.
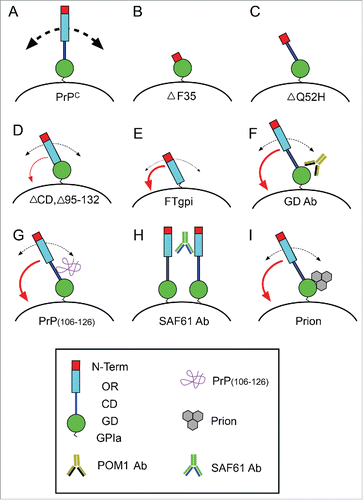
DISCLOSURE OF POTENTIAL CONFLICTS OF INTEREST
No potential conflicts of interest were disclosed.
ACKNOWLEDGMENTS
The authors want to thank Robert Sloviter (Morehouse School of Medicine, Atlanta, Georgia USA) for advice and helpful comments on KA-mediated excitotoxicity over the years, and Adriano Aguzzi (Institute of Neurobiology, Zürich, Switzerland) for comments concerning Prnp-flanking genes. We would like to thank former and current members of our groups for the encouraging discussions about Prnp functions over time. The authors thank Tom Yohannan for editorial advice.
Funding
This research was supported by the Spanish Ministry of Science and Innovation (BFU2012–32617), FP7-PRIORITY, the Generalitat de Catalunya (SGR2014–1218), CIBERNED (PI2014/02–4 and PRY-14–114), La Caixa Obra Social Foundation, La Marato de TV3, the Basque Foundation of Health and Innovation Research (BIO12/AL/004), and the Spanish Prion Network AGL2015–71764-REDT to JADR.
REFERENCES
- Steele AD, Emsley JG, Ozdinler PH, Lindquist S, Macklis JD. Prion protein (PrPc) positively regulates neural precursor proliferation during developmental and adult mammalian neurogenesis. Proc Natl Acad Sci U S A 2006; 103:3416-21; PMID:16492732; http://dx.doi.org/10.1073/pnas.0511290103
- Llorens F, Carulla P, Villa A, Torres JM, Fortes P, Ferrer I, del Río JA. PrP(C) regulates epidermal growth factor receptor function and cell shape dynamics in Neuro2a cells. J Neurochem 2013; 127:124-38; PMID:23638794
- Brown DR, Nicholas RS, Canevari L. Lack of prion protein expression results in a neuronal phenotype sensitive to stress. J Neurosci Res 2002; 67:211-24; PMID:11782965; http://dx.doi.org/10.1002/jnr.10118
- Wong BS, Liu T, Li R, Pan T, Petersen RB, Smith MA, Gambetti P, Perry G, Manson JC, Brown DR, et al. Increased levels of oxidative stress markers detected in the brains of mice devoid of prion protein. J Neurochem 2001; 76:565-72; PMID:11208919; http://dx.doi.org/10.1046/j.1471-4159.2001.00028.x
- Brown DR, Qin K, Herms JW, Madlung A, Manson J, Strome R, Fraser PE, Kruck T, von Bohlen A, Schulz-Schaeffer W, et al. The cellular prion protein binds copper in vivo. Nature 1997; 390:684-7; PMID:9414160; http://dx.doi.org/10.1038/37733
- Vassallo N, Herms J. Cellular prion protein function in copper homeostasis and redox signalling at the synapse. J Neurochem 2003; 86:538-44; PMID:12859667; http://dx.doi.org/10.1046/j.1471-4159.2003.01882.x
- Liang J, Pan Y, Zhang D, Guo C, Shi Y, Wang J, Chen Y, Wang X, Liu J, Guo X, et al. Cellular prion protein promotes proliferation and G1/S transition of human gastric cancer cells SGC7901 and AGS. FASEB J 2007; 21:2247-56; PMID:17409275; http://dx.doi.org/10.1096/fj.06-7799com
- Miranda A, Ramos-Ibeas P, Pericuesta E, Ramirez MA, Gutierrez-Adan A. The role of prion protein in stem cell regulation. Reproduction 2013; 146:R91-9; PMID:23740082; http://dx.doi.org/10.1530/REP-13-0100
- Mouillet-Richard S, Ermonval M, Chebassier C, Laplanche JL, Lehmann S, Launay JM, Kellermann O. Signal transduction through prion protein. Science 2000; 289:1925-8; PMID:10988071; http://dx.doi.org/10.1126/science.289.5486.1925
- Hugel B, Martinez MC, Kunzelmann C, Blattler T, Aguzzi A, Freyssinet JM. Modulation of signal transduction through the cellular prion protein is linked to its incorporation in lipid rafts. Cell Mol Life Sci 2004; 61:2998-3007; PMID:15583862; http://dx.doi.org/10.1007/s00018-004-4318-2
- Bueler H, Fischer M, Lang Y, Bluethmann H, Lipp HP, DeArmond SJ, Prusiner SB, Aguet M, Weissmann . Normal development and behaviour of mice lacking the neuronal cell-surface PrP protein. Nature 1992; 356:577-82; PMID:1373228; http://dx.doi.org/10.1038/356577a0
- Brandner S, Raeber A, Sailer A, Blattler T, Fischer M, Weissmann C, et al. Normal host prion protein (PrPC) is required for scrapie spread within the central nervous system. Proc Natl Acad Sci U S A 1996; 93:13148-51; PMID:8917559; http://dx.doi.org/10.1073/pnas.93.23.13148
- Tobler I, Gaus SE, Deboer T, Achermann P, Fischer M, Rulicke T, Moser M, Oesch B, McBride PA, Manson JC. Altered circadian activity rhythms and sleep in mice devoid of prion protein. Nature 1996; 380:639-42; PMID:8602267; http://dx.doi.org/10.1038/380639a0
- Gadotti VM, Bonfield SP, Zamponi GW. Depressive-like behaviour of mice lacking cellular prion protein. Behav Brain Res 2012; 227:319-23; PMID:21439331; http://dx.doi.org/10.1016/j.bbr.2011.03.012
- Beckman D, Santos LE, Americo TA, Ledo JH, de Mello FG, Linden R. Prion Protein Modulates Monoaminergic Systems and Depressive-like Behavior in Mice. J Biol Chem 2015; 290:20488-98; PMID:26152722; http://dx.doi.org/10.1074/jbc.M115.666156
- Criado JR, Sanchez-Alavez M, Conti B, Giacchino JL, Wills DN, Henriksen SJ, Race R, Manson JC, Chesebro B, Oldstone MB. Mice devoid of prion protein have cognitive deficits that are rescued by reconstitution of PrP in neurons. Neurobiol Dis 2005; 19:255-65; PMID:15837581; http://dx.doi.org/10.1016/j.nbd.2005.01.001
- Bremer J, Baumann F, Tiberi C, Wessig C, Fischer H, Schwarz P, Steele AD, Toyka KV, Nave KA, Weis J, et al. Axonal prion protein is required for peripheral myelin maintenance. Nat Neurosci 2010; 13:310-8; PMID:20098419; http://dx.doi.org/10.1038/nn.2483
- Schmitz M, Greis C, Ottis P, Silva CJ, Schulz-Schaeffer WJ, Wrede A, Koppe K, Onisko B, Requena JR, Govindarajan N, et al. Loss of prion protein leads to age-dependent behavioral abnormalities and changes in cytoskeletal protein expression. Mol Neurobiol 2014; 50(3):923-36.
- Le Pichon CE, Valley MT, Polymenidou M, Chesler AT, Sagdullaev BT, Aguzzi A, Firestein S. Olfactory behavior and physiology are disrupted in prion protein knockout mice. Nat Neurosci 2009; 12:60-9; PMID:19098904; http://dx.doi.org/10.1038/nn.2238
- Kishimoto Y, Hirono M, Atarashi R, Sakaguchi S, Yoshioka T, Katamine S, Kirino Y. Age-dependent impairment of eyeblink conditioning in prion protein-deficient mice. PLoS One 2013; 8:e60627.
- Sanchez-Alavez M, Conti B, Moroncini G, Criado JR. Contributions of neuronal prion protein on sleep recovery and stress response following sleep deprivation. Brain Res 2007; 1158:71-80; PMID:17570349; http://dx.doi.org/10.1016/j.brainres.2007.05.010
- Walz R, Amaral OB, Rockenbach IC, Roesler R, Izquierdo I, Cavalheiro EA, Martins VR, Brentani RR. Increased sensitivity to seizures in mice lacking cellular prion protein. Epilepsia 1999; 40:1679-82; PMID:10612329; http://dx.doi.org/10.1111/j.1528-1157.1999.tb01583.x
- Rangel A, Burgaya F, Gavin R, Soriano E, Aguzzi A, Del Rio JA. Enhanced susceptibility of Prnp-deficient mice to kainate-induced seizures, neuronal apoptosis, and death: Role of AMPA/kainate receptors. J Neurosci Res 2007; 85:2741-55; PMID:17304577; http://dx.doi.org/10.1002/jnr.21215
- Khosravani H, Zhang Y, Tsutsui S, Hameed S, Altier C, Hamid J, Chen L, Villemaire M, Ali Z, Jirik FR, et al. Prion protein attenuates excitotoxicity by inhibiting NMDA receptors. J Gen Physiol 2008; 131:i5; PMID:18504311; http://dx.doi.org/10.1085/JGP1316OIA5
- Gasperini L, Meneghetti E, Pastore B, Benetti F, Legname G. Prion protein and copper cooperatively protect neurons by modulating NMDA receptor through S-nitrosylation. Antioxid Redox Signal 2015; 22:772-84; PMID:25490055; http://dx.doi.org/10.1089/ars.2014.6032
- Bribian A, Fontana X, Llorens F, Gavin R, Reina M, Garcia-Verdugo JM, Torres JM, de Castro F, del Río JA. Role of the cellular prion protein in oligodendrocyte precursor cell proliferation and differentiation in the developing and adult mouse CNS. PLoS One 2012; 7:e33872; PMID:22529900; http://dx.doi.org/10.1371/journal.pone.0033872
- Nicolas O, Gavin R, del Rio JA. New insights into cellular prion protein (PrPc) functions: the “ying and yang” of a relevant protein. Brain Res Rev 2009; 61:170-84; PMID:19523487; http://dx.doi.org/10.1016/j.brainresrev.2009.06.002
- Steele AD, Lindquist S, Aguzzi A. The prion protein knockout mouse: a phenotype under challenge. Prion 2007; 1:83-93; PMID:19164918; http://dx.doi.org/10.4161/pri.1.2.4346
- Schmitt-Ulms G, Legname G, Baldwin MA, Ball HL, Bradon N, Bosque PJ, Crossin KL, Edelman GM, DeArmond SJ, Cohen FE, et al. Binding of neural cell adhesion molecules (N-CAMs) to the cellular prion protein. J Mol Biol 2001; 314:1209-25; PMID:11743735; http://dx.doi.org/10.1006/jmbi.2000.5183
- Santuccione A, Sytnyk V, Leshchyns'ka I, Schachner M. Prion protein recruits its neuronal receptor NCAM to lipid rafts to activate p59fyn and to enhance neurite outgrowth. J Cell Biol 2005; 169:341-54; PMID:15851519; http://dx.doi.org/10.1083/jcb.200409127
- Parkyn CJ, Vermeulen EG, Mootoosamy RC, Sunyach C, Jacobsen C, Oxvig C, Moestrup S, Liu Q, Bu G, Jen A, et al. LRP1 controls biosynthetic and endocytic trafficking of neuronal prion protein. J Cell Sci 2008; 121:773-83; PMID:18285446; http://dx.doi.org/10.1242/jcs.021816
- Gauczynski S, Peyrin JM, Haik S, Leucht C, Hundt C, Rieger R, Krasemann S, Deslys JP, Dormont D, Lasmézas CI, et al. The 37-kDa/67-kDa laminin receptor acts as the cell-surface receptor for the cellular prion protein. EMBO J 2001; 20:5863-75; PMID:11689427; http://dx.doi.org/10.1093/emboj/20.21.5863
- Rieger R, Edenhofer F, Lasmezas CI, Weiss S. The human 37-kDa laminin receptor precursor interacts with the prion protein in eukaryotic cells. Nat Med 1997; 3:1383-8; PMID:9396609; http://dx.doi.org/10.1038/nm1297-1383
- Parkin ET, Watt NT, Hussain I, Eckman EA, Eckman CB, Manson JC, Baybutt HN, Turner AJ, Hooper NM. Cellular prion protein regulates β-secretase cleavage of the Alzheimer amyloid precursor protein. Proc Natl Acad Sci U S A 2007; 104:11062-7; PMID:17573534; http://dx.doi.org/10.1073/pnas.0609621104
- Kurschner C, Morgan JI. The cellular prion protein (PrP) selectively binds to Bcl−2 in the yeast two-hybrid system. Brain Res Mol Brain Res 1995; 30:165-8; PMID:7609638; http://dx.doi.org/10.1016/0169-328X(95)00013-I
- Brown DR, Schmidt B, Kretzschmar HA. Prion protein fragment interacts with PrP-deficient cells. J Neurosci Res 1998; 52:260-7; PMID:9590434; http://dx.doi.org/10.1002/(SICI)1097-4547(19980501)52:3%3c260::AID-JNR2%3e3.0.CO;2-B
- Nieznanski K, Nieznanska H, Skowronek KJ, Osiecka KM, Stepkowski D. Direct interaction between prion protein and tubulin. Biochem Biophys Res Commun 2005; 334:403-11; PMID:16004966; http://dx.doi.org/10.1016/j.bbrc.2005.06.092
- Keshet GI, Bar-Peled O, Yaffe D, Nudel U, Gabizon R. The cellular prion protein colocalizes with the dystroglycan complex in the brain. J Neurochem 2000; 75:1889-97; PMID:11032878; http://dx.doi.org/10.1046/j.1471-4159.2000.0751889.x
- Spielhaupter C, Schatzl HM. PrPC directly interacts with proteins involved in signaling pathways. J Biol Chem 2001; 276:44604-12; PMID:11571277; http://dx.doi.org/10.1074/jbc.M103289200
- Llorens F, Del Rio JA. Unraveling the neuroprotective mechanisms of PrP (C) in excitotoxicity. Prion 2012; 6:245-51; PMID:22437735; http://dx.doi.org/10.4161/pri.19639
- Nuvolone M, Kana V, Hutter G, Sakata D, Mortin-Toth SM, Russo G, et al. SIRPalpha polymorphisms, but not the prion protein, control phagocytosis of apoptotic cells. J Exp Med 2013; 210:2539-52; PMID:24145514; http://dx.doi.org/10.1084/jem.20131274
- Calella AM, Farinelli M, Nuvolone M, Mirante O, Moos R, Falsig J, Mansuy IM, Aguzzi A. Prion protein and Abeta-related synaptic toxicity impairment. EMBO Mol Med 2010; 2:306-14; PMID:20665634; http://dx.doi.org/10.1002/emmm.201000082
- Carulla P, Llorens F, Matamoros-Angles A, Aguilar-Calvo P, Espinosa JC, Gavin R, Ferrer I, Legname G, Torres JM, del Río JA. Involvement of PrP(C) in kainate-induced excitotoxicity in several mouse strains. Sci Rep 2015; 5:11971; PMID:26155834; http://dx.doi.org/10.1038/srep11971
- Gerlai R. Gene-targeting studies of mammalian behavior: is it the mutation or the background genotype? Trends Neurosci 1996; 19:177-81; PMID:8723200; http://dx.doi.org/10.1016/S0166-2236(96)20020-7
- Smithies O, Maeda N. Gene targeting approaches to complex genetic diseases: atherosclerosis and essential hypertension. Proc Natl Acad Sci U S A 1995; 92:5266-72; PMID:7777495; http://dx.doi.org/10.1073/pnas.92.12.5266
- Kranich J, Krautler NJ, Falsig J, Ballmer B, Li S, Hutter G, et al. Engulfment of cerebral apoptotic bodies controls the course of prion disease in a mouse strain-dependent manner. J Exp Med 2010; 207:2271-81; PMID:20837697; http://dx.doi.org/10.1084/jem.20092401
- Herms JW, Kretzchmar HA, Titz S, Keller BU. Patch-clamp analysis of synaptic transmission to cerebellar purkinje cells of prion protein knockout mice. Eur J Neurosci 1995; 7:2508-12; PMID:8845956; http://dx.doi.org/10.1111/j.1460-9568.1995.tb01049.x
- Lledo PM, Tremblay P, DeArmond SJ, Prusiner SB, Nicoll RA. Mice deficient for prion protein exhibit normal neuronal excitability and synaptic transmission in the hippocampus. Proc Natl Acad Sci U S A 1996; 93:2403-7; PMID:8637886; http://dx.doi.org/10.1073/pnas.93.6.2403
- Mercer RC, Ma L, Watts JC, Strome R, Wohlgemuth S, Yang J, Cashman NR, Coulthart MB, Schmitt-Ulms G, Jhamandas JH, et al. The prion protein modulates A-type K+ currents mediated by Kv4.2 complexes through dipeptidyl aminopeptidase-like protein 6. J Biol Chem 2013; 288:37241-55; PMID:24225951; http://dx.doi.org/10.1074/jbc.M113.488650
- Ratte S, Vreugdenhil M, Boult JK, Patel A, Asante EA, Collinge J, Jefferys JG. Threshold for epileptiform activity is elevated in prion knockout mice. Neuroscience 2011; 179:56-61; PMID:21277354; http://dx.doi.org/10.1016/j.neuroscience.2011.01.053
- Striebel JF, Race B, Chesebro B. Prion protein and susceptibility to kainate-induced seizures: genetic pitfalls in the use of PrP knockout mice. Prion 2013; 7:280-5; PMID:23851597; http://dx.doi.org/10.4161/pri.25738
- Striebel JF, Race B, Pathmajeyan M, Rangel A, Chesebro B. Lack of influence of prion protein gene expression on kainate-induced seizures in mice: studies using congenic, coisogenic and transgenic strains. Neuroscience 2013; 238:11-8; PMID:23415788; http://dx.doi.org/10.1016/j.neuroscience.2013.02.004
- Carulla P, Bribian A, Rangel A, Gavin R, Ferrer I, Caelles C, Del Río JA, Llorens F. Neuroprotective role of PrPC against kainate-induced epileptic seizures and cell death depends on the modulation of JNK3 activation by GluR6/7-PSD-95 binding. Mol Biol Cell 2011; 22:3041-54; PMID:21757544; http://dx.doi.org/10.1091/mbc.E11-04-0321
- Rangel A, Madronal N, Gruart A, Gavin R, Llorens F, Sumoy L, Torres JM, Delgado-García JM, Del Río JA. Regulation of GABA(A) and glutamate receptor expression, synaptic facilitation and long-term potentiation in the hippocampus of prion mutant mice. PLoS One 2009; 4:e7592; PMID:19855845; http://dx.doi.org/10.1371/journal.pone.0007592
- Benvegnu S, Roncaglia P, Agostini F, Casalone C, Corona C, Gustincich S, Legname G. Developmental influence of the cellular prion protein on the gene expression profile in mouse hippocampus. Physiol Genomics 2011; 43:711-25; PMID:21406608; http://dx.doi.org/10.1152/physiolgenomics.00205.2010
- Brenner HR, Herczeg A, Oesch B. Normal development of nerve-muscle synapses in mice lacking the prion protein gene. Proc Biol Sci 1992; 250:151-5; PMID:1282260; http://dx.doi.org/10.1098/rspb.1992.0143
- Fischer M, Rulicke T, Raeber A, Sailer A, Moser M, Oesch B, Brandner S, Aguzzi A, Weissmann C. Prion protein (PrP) with amino-proximal deletions restoring susceptibility of PrP knockout mice to scrapie. EMBO J 1996; 15:1255-64; PMID:8635458
- Weissmann C, Fischer M, Raeber A, Bueler H, Sailer A, Shmerling D, Rülicke T, Brandner S, Aguzzi A. The use of transgenic mice in the investigation of transmissible spongiform encephalopathies. Rev Sci Tech 1998; 17:278-90; PMID:9638817
- Dametto P, Lakkaraju AK, Bridel C, Villiger L, O'Connor T, Herrmann US, Pelczar P, Rülicke T, McHugh D, Adili A, et al. Neurodegeneration and unfolded-protein response in mice expressing a membrane-tethered flexible tail of PrP. PLoS One 2015; 10:e0117412; PMID:25658480; http://dx.doi.org/10.1371/journal.pone.0117412
- Llorens F, Ferrer I, del Rio JA. Gene expression resulting from PrPC ablation and PrPC overexpression in murine and cellular models. Mol Neurobiol 2014; 49:413-23; PMID:23949728; http://dx.doi.org/10.1007/s12035-013-8529-0
- Collinge J, Whittington MA, Sidle KC, Smith CJ, Palmer MS, Clarke AR, Jefferys JG. Prion protein is necessary for normal synaptic function. Nature 1994; 370:295-7; PMID:8035877; http://dx.doi.org/10.1038/370295a0
- Schauwecker PE, Steward O. Genetic determinants of susceptibility to excitotoxic cell death: implications for gene targeting approaches. Proc Natl Acad Sci U S A 1997; 94:4103-8; PMID:9108112; http://dx.doi.org/10.1073/pnas.94.8.4103
- McKhann GM, 2nd, Wenzel HJ, Robbins CA, Sosunov AA, Schwartzkroin PA. Mouse strain differences in kainic acid sensitivity, seizure behavior, mortality, and hippocampal pathology. Neuroscience 2003; 122:551-61; PMID:14614919; http://dx.doi.org/10.1016/S0306-4522(03)00562-1
- Schauwecker PE. Modulation of cell death by mouse genotype: differential vulnerability to excitatory amino acid-induced lesions. Exp Neurol 2002; 178:219-35; PMID:12504881; http://dx.doi.org/10.1006/exnr.2002.8038
- Schauwecker PE. Complications associated with genetic background effects in models of experimental epilepsy. Prog Brain Res 2002; 135:139-48; PMID:12143336; http://dx.doi.org/10.1016/S0079-6123(02)35014-3
- McCord MC, Lorenzana A, Bloom CS, Chancer ZO, Schauwecker PE. Effect of age on kainate-induced seizure severity and cell death. Neuroscience 2008; 154:1143-53; PMID:18479826; http://dx.doi.org/10.1016/j.neuroscience.2008.03.082
- Liu L, Hamre KM, Goldowitz D. Kainic acid-induced neuronal degeneration in hippocampal pyramidal neurons is driven by both intrinsic and extrinsic factors: analysis of FVB/N<–>C57BL/6 chimeras. J Neurosci 2012; 32:12093-101; PMID:22933793; http://dx.doi.org/10.1523/JNEUROSCI.6478-11.2012
- Sakudo A, Onodera T. Prion protein (PrP) gene-knockout cell lines: insight into functions of the PrP. Front Cell Dev Biol 2014; 2:75; PMID:25642423
- Fleisch VC, Leighton PL, Wang H, Pillay LM, Ritzel RG, Bhinder G, Roy B, Tierney KB, Ali DW, Waskiewicz AJ, et al. Targeted mutation of the gene encoding prion protein in zebrafish reveals a conserved role in neuron excitability. Neurobiol Dis 2013; 55:11-25; PMID:23523635; http://dx.doi.org/10.1016/j.nbd.2013.03.007
- Watt NT, Taylor DR, Kerrigan TL, Griffiths HH, Rushworth JV, Whitehouse IJ, Hooper NM. Prion protein facilitates uptake of zinc into neuronal cells. Nat Commun 2012; 3:1134; PMID:23072804; http://dx.doi.org/10.1038/ncomms2135
- Beraldo FH, Arantes CP, Santos TG, Machado CF, Roffe M, Hajj GN, Lee KS, Magalhães AC, Caetano FA, Mancini GL, et al. Metabotropic glutamate receptors transduce signals for neurite outgrowth after binding of the prion protein to laminin gamma1 chain. FASEB J 2011; 25:265-79; PMID:20876210; http://dx.doi.org/10.1096/fj.10-161653
- Zeng F, Watt NT, Walmsley AR, Hooper NM. Tethering the N-terminus of the prion protein compromises the cellular response to oxidative stress. J Neurochem 2003; 84:480-90; PMID:12558968; http://dx.doi.org/10.1046/j.1471-4159.2003.01529.x
- Sonati T, Reimann RR, Falsig J, Baral PK, O'Connor T, Hornemann S, Yaganoglu S, Li B, Herrmann US, Wieland B, et al. The toxicity of antiprion antibodies is mediated by the flexible tail of the prion protein. Nature 2013; 501:102-6; PMID:23903654; http://dx.doi.org/10.1038/nature12402
- Herrmann US, Sonati T, Falsig J, Reimann RR, Dametto P, O'Connor T, Li B, Lau A, Hornemann S, Sorce S, et al. Prion infections and anti-PrP antibodies trigger converging neurotoxic pathways. PLoS Pathog 2015; 11:e1004662; PMID:25710374; http://dx.doi.org/10.1371/journal.ppat.1004662
- Shmerling D, Hegyi I, Fischer M, Blattler T, Brandner S, Gotz J, Rülicke T, Flechsig E, Cozzio A, von Mering C, et al. Expression of amino-terminally truncated PrP in the mouse leading to ataxia and specific cerebellar lesions. Cell 1998; 93:203-14; PMID:9568713; http://dx.doi.org/10.1016/S0092-8674(00)81572-X
- Nicolas O, Gavin R, Braun N, Urena JM, Fontana X, Soriano E, Aguzzi A, del Río JA. Bcl−2 overexpression delays caspase-3 activation and rescues cerebellar degeneration in prion-deficient mice that overexpress amino-terminally truncated prion. FASEB J 2007; 21:3107-17; PMID:17494993; http://dx.doi.org/10.1096/fj.06-7827com
- Mitteregger G, Vosko M, Krebs B, Xiang W, Kohlmannsperger V, Nolting S, Hamann GF, Kretzschmar HA. The role of the octarepeat region in neuroprotective function of the cellular prion protein. Brain Pathol 2007; 17:174-83; PMID:17388948; http://dx.doi.org/10.1111/j.1750-3639.2007.00061.x
- Schneider B, Mutel V, Pietri M, Ermonval M, Mouillet-Richard S, Kellermann O. NADPH oxidase and extracellular regulated kinases 1/2 are targets of prion protein signaling in neuronal and nonneuronal cells. Proc Natl Acad Sci U S A 2003; 100:13326-31; PMID:14597699; http://dx.doi.org/10.1073/pnas.2235648100
- Gavin R, Braun N, Nicolas O, Parra B, Urena JM, Mingorance A, Soriano E, Torres JM, Aguzzi A, del Río JA. PrP(106-126) activates neuronal intracellular kinases and Egr1 synthesis through activation of NADPH-oxidase independently of PrPc. FEBS Lett 2005; 579:4099-106; PMID:16023105; http://dx.doi.org/10.1016/j.febslet.2005.06.037
- Solforosi L, Criado JR, McGavern DB, Wirz S, Sanchez-Alavez M, Sugama S, DeGiorgio LA, Volpe BT, Wiseman E, Abalos G, et al. Cross-linking cellular prion protein triggers neuronal apoptosis in vivo. Science 2004; 303:1514-6; PMID:14752167; http://dx.doi.org/10.1126/science.1094273
- Klohn PC, Farmer M, Linehan JM, O'Malley C, Fernandez de Marco M, Taylor W, et al. PrP antibodies do not trigger mouse hippocampal neuron apoptosis. Science 2012; 335:52; PMID:22223800; http://dx.doi.org/10.1126/science.1215579
- Forloni G, Angeretti N, Chiesa R, Monzani E, Salmona M, Bugiani O, Tagliavini F. Neurotoxicity of a prion protein fragment. Nature 1993; 362:543-6; PMID:8464494; http://dx.doi.org/10.1038/362543a0
- Sakudo A, Nakamura I, Lee DC, Saeki K, Ikuta K, Onodera T. Neurotoxic prion protein (PrP) fragment 106-126 requires the N-terminal half of the hydrophobic region of PrP in the PrP-deficient neuronal cell line. Protein Pept Lett 2007; 14:1-6; PMID:17266643
- Gavin R, Urena J, Rangel A, Pastrana MA, Requena JR, Soriano E, Aguzzi A, Del Río JA. Fibrillar prion peptide PrP(106-126) treatment induces Dab1 phosphorylation and impairs APP processing and Abeta production in cortical neurons. Neurobiol Dis 2008; 30:243-54; PMID:18374587; http://dx.doi.org/10.1016/j.nbd.2008.02.001
- Pietri M, Caprini A, Mouillet-Richard S, Pradines E, Ermonval M, Grassi J, Kellermann O, Schneider B. Overstimulation of PrPC signaling pathways by prion peptide 106-126 causes oxidative injury of bioaminergic neuronal cells. J Biol Chem 2006; 281:28470-9; PMID:16864581; http://dx.doi.org/10.1074/jbc.M602774200
- Li A, Christensen HM, Stewart LR, Roth KA, Chiesa R, Harris DA. Neonatal lethality in transgenic mice expressing prion protein with a deletion of residues 105-125. EMBO J 2007; 26:548-58; PMID:17245437; http://dx.doi.org/10.1038/sj.emboj.7601507
- Vassallo N, Herms J, Behrens C, Krebs B, Saeki K, Onodera T, Windl O, Kretzschmar HA. Activation of phosphatidylinositol 3-kinase by cellular prion protein and its role in cell survival. Biochem Biophys Res Commun 2005; 332:75-82; PMID: 15896301; http://dx.doi.org/10.1016/j.bbrc.2005.04.099
- Li QQ, Sun YP, Ruan CP, Xu XY, Ge JH, He J, Xu ZD, Wang Q, Gao WC. Cellular prion protein promotes glucose uptake through the Fyn-HIF-2alpha-Glut1 pathway to support colorectal cancer cell survival. Cancer Sci 2011; 102:400-6; PMID:21265952; http://dx.doi.org/10.1111/j.1349-7006.2010.01811.x
- Bravard A, Auvre F, Fantini D, Bernardino-Sgherri J, Sissoeff L, Daynac M, Xu Z, Etienne O, Dehen C, Comoy E, et al. The prion protein is critical for DNA repair and cell survival after genotoxic stress. Nucleic Acids Res 2015; 43:904-16; PMID:25539913; http://dx.doi.org/10.1093/nar/gku1342
- Suzuki G, Shimazu N, Tanaka M. A yeast prion, Mod5, promotes acquired drug resistance and cell survival under environmental stress. Science 2012; 336:355-9; PMID:22517861; http://dx.doi.org/10.1126/science.1219491
- Haik S, Peyrin JM, Lins L, Rosseneu MY, Brasseur R, Langeveld JP, Tagliavini F, Deslys JP, Lasmézas C, Dormont D. Neurotoxicity of the putative transmembrane domain of the prion protein. Neurobiol Dis 2000; 7:644-56; PMID:11114262; http://dx.doi.org/10.1006/nbdi.2000.0316
- Wang X, Bowers SL, Wang F, Pu XA, Nelson RJ, Ma J. Cytoplasmic prion protein induces forebrain neurotoxicity. Biochim Biophys Acta 2009; 1792:555-63; PMID:19281844; http://dx.doi.org/10.1016/j.bbadis.2009.02.014
- Vilches S, Vergara C, Nicolas O, Sanclimens G, Merino S, Varon S, Acosta GA, Albericio F, Royo M, Del Río JA, et al. Neurotoxicity of prion peptides mimicking the central domain of the cellular prion protein. PLoS One 2013; 8:e70881; PMID:23940658; http://dx.doi.org/10.1371/journal.pone.0070881
- Vilches S, Vergara C, Nicolas O, Mata A, Del Rio JA, Gavin R. Domain-specific activation of death-associated intracellular signalling cascades by the cellular prion protein in neuroblastoma cells. Mol Neurobiol 2015; PMID:26250617.
- White AR, Maher F, Brazier MW, Jobling MF, Thyer J, Stewart LR, Thompson A, Gibson R, Masters CL, Multhaup G, et al. Diverse fibrillar peptides directly bind the Alzheimer amyloid precursor protein and amyloid precursor-like protein 2 resulting in cellular accumulation. Brain Res 2003; 966:231-44; PMID:12618346; http://dx.doi.org/10.1016/S0006-8993(02)04173-2
- Lauren J, Gimbel DA, Nygaard HB, Gilbert JW, Strittmatter SM. Cellular prion protein mediates impairment of synaptic plasticity by amyloid-β oligomers. Nature 2009; 457:1128-32; PMID: 19242475; http://dx.doi.org/10.1038/nature07761
- Kostylev MA, Kaufman AC, Nygaard HB, Patel P, Haas LT, Gunther ECg, Vortmeyer A, Strittmatter SM. Prion-protein-interacting amyloid-β oligomers of high molecular weight are tightly correlated with memory impairment in multiple Alzheimer mouse models. J Biol Chem 2015; 290:17415-38; PMID:26018073; http://dx.doi.org/10.1074/jbc.M115.643577
- Nygaard HB, Strittmatter SM. Cellular prion protein mediates the toxicity of β-amyloid oligomers: implications for Alzheimer disease. Arch Neurol 2009; 66:1325-8; PMID:19901162
- Kudo W, Lee HP, Zou WQ, Wang X, Perry G, Zhu X, Smith MA, Petersen RB, Lee HG. Cellular prion protein is essential for oligomeric amyloid-β-induced neuronal cell death. Hum Mol Genet 2012; 21:1138-44; PMID:22100763; http://dx.doi.org/10.1093/hmg/ddr542
- Gimbel DA, Nygaard HB, Coffey EE, Gunther EC, Lauren J, Gimbel ZA, Strittmatter SM. Memory impairment in transgenic Alzheimer mice requires cellular prion protein. J Neurosci 2010; 30:6367-74; PMID:20445063; http://dx.doi.org/10.1523/JNEUROSCI.0395-10.2010
- Barry AE, Klyubin I, Mc Donald JM, Mably AJ, Farrell MA, Scott M, Walsh DM, Rowan MJ. Alzheimer disease brain-derived amyloid-β-mediated inhibition of LTP in vivo is prevented by immunotargeting cellular prion protein. J Neurosci 2011; 31:7259-63; PMID:21593310; http://dx.doi.org/10.1523/JNEUROSCI.6500-10.2011
- Fluharty BR, Biasini E, Stravalaci M, Sclip A, Diomede L, Balducci C, La Vitola P, Messa M, Colombo L, Forloni G, et al. An N-terminal fragment of the prion protein binds to amyloid-β oligomers and inhibits their neurotoxicity in vivo. J Biol Chem 2013; 288:7857-66; PMID:23362282; http://dx.doi.org/10.1074/jbc.M112.423954
- Ordonez-Gutierrez L, Torres JM, Gavin R, Anton M, Arroba-Espinosa AI, Espinosa JC, Vergara C, Del Río JA, Wandosell F. Cellular prion protein modulates β-amyloid deposition in aged APP/PS1 transgenic mice. Neurobiol Aging 2013; 34:2793-804; PMID:23831375; http://dx.doi.org/10.1016/j.neurobiolaging.2013.05.019
- Um JW, Kaufman AC, Kostylev M, Heiss JK, Stagi M, Takahashi H, Kerrisk ME, Vortmeyer A, Wisniewski T, Koleske AJ, et al. Metabotropic glutamate receptor 5 is a coreceptor for Alzheimer abeta oligomer bound to cellular prion protein. Neuron 2013; 79:887-902; PMID:24012003; http://dx.doi.org/10.1016/j.neuron.2013.06.036
- Risse E, Nicoll AJ, Taylor WA, Wright D, Badoni M, Yang X, Farrow MA, Collinge J. Identification of a compound that disrupts binding of amyloid-β to the prion protein using a novel fluorescence-based assay. J Biol Chem 2015; 290:17020-8; PMID:25995455; http://dx.doi.org/10.1074/jbc.M115.637124
- Sakudo A, Wu G, Onodera T, Ikuta K. Octapeptide repeat region of prion protein (PrP) is required at an early stage for production of abnormal prion protein in PrP-deficient neuronal cell line. Biochem Biophys Res Commun 2008; 365:164-9; PMID:17981146; http://dx.doi.org/10.1016/j.bbrc.2007.10.158
- Lee DC, Sakudo A, Kim CK, Nishimura T, Saeki K, Matsumoto Y, Yokoyama T, Chen SG, Itohara S, Onodera T. Fusion of Doppel to octapeptide repeat and N-terminal half of hydrophobic region of prion protein confers resistance to serum deprivation. Microbiol Immunol 2006; 50:203-9; PMID:16547418; http://dx.doi.org/10.1111/j.1348-0421.2006.tb03787.x
- Cui T, Daniels M, Wong BS, Li R, Sy MS, Sassoon J, Brown DR. Mapping the functional domain of the prion protein. Eur J Biochem 2003; 270:3368-76; PMID:12899694; http://dx.doi.org/10.1046/j.1432-1033.2003.03717.x
APPENDIX
Examples of Pubmed citations on PrPC functions
Paragraphs illustrating 3 examples of manuscripts describing some functions of the PrPC. These manuscripts were selected as examples with the Pubmed search of the subject “cellular prion protein” and the particular process as keyword. As examples, the keywords cell survival, neurotoxicity and Alzheimer's disease were used. Notice that in each description several studies reported roles for PrPC at different levels, and in some cases these are controversial.
Cell survival and cellular prion protein
PrPC increase cell proliferation and survivalCitation1
PrPC absence increases proliferation of hippocampal precursorsCitation26
PrPC mediate cell cycle on neuroblastoma cellsCitation2
Cell survival by acting on PIK3 kinaseCitation87
Cell survival promoting glucose uptake in cancer cellsCitation88
Cell survival after genotoxic stressCitation89
Yeast prion promote drug resistance and cell survivalCitation90
Neurotoxicity induced by the cellular prion protein or its domains in vitro and in vivo
A syntethic peptide of PrPC is neurotoxicCitation79,82
Description of the neurotoxicity of the putative transmembrane domain of PrPC.Citation91
Description of the neurotoxic potential of the cytoplasmic domain of PrPCCitation92
Peptides mimicking the central domain of PrPC triggers cell-death pathwaysCitation93
PrPC aggregating antibodies induced cell deathCitation9
Membrane tethered PrPC triggers cell death in miceCitation59
The flexible tail of PrPC mediates cell-death induced by PrP antibodiesCitation73
PrPC-directed antibodies do not trigger apoptosisCitation81
Distinct domains of PrPC triggers different intracellular cell-death signalsCitation94
Cellular prion protein and Alzheimer´s disease (Aβ).
PrPC–derived peptides bind to amyloid precursor protein (APP)Citation95
PrPC regulates BACE1 activityCitation34 and APP processingCitation84
PrPC mediates impairment of synaptic plasticity mediated by AβCitation96,97
PrPC mediates the toxicity of Aβ oligomersCitation98,99
PrPC is not involved in Aβ-mediated toxicity and synaptic plasticityCitation42
PrPC expression is needed for memory impairment in mouse models of Alzheimer diseaseCitation100
PrPC immunotargeting in vivo prevents Aβ-mediated LTP inhibitionCitation101
The N-terminal domain of PrPC binds to Aβ oligomersCitation102
PrPC modulate Aβ production and deposition in mouse modelsCitation103
Metabotropic glutamate receptor 5 is a coreceptor of Aβ oligomers and PrPCCitation104
Blocking Aβ binding to PrPC as terapeuthic strategy for Alzheimer´s disease.Citation105