ABSTRACT
Organisms rely on correctly folded proteins to carry out essential functions. Protein quality control factors guard proteostasis and prevent protein misfolding. When quality control fails and in response to diverse stresses, many proteins start to accumulate at specific deposit sites that maintain cellular organization and protect the functionality of coalescing proteins. These transitions involve dedicated proteins that promote coalescence and are facilitated by endo-membranes and cytoskeletal platforms. Moreover, several proteins make use of weak multivalent interactions or conformational templating to drive the formation of large-scale assemblies. Formation of such assemblies is often associated with a change in biochemical activity that can be used by cells to execute biochemical decisions in a localized manner during development and adaption. Since all assembly types impact cell physiology, their localization and dynamics need to be tightly regulated. Interestingly, at least some of the regulatory mechanisms are shared by functional membrane-less organelles and assemblies of terminally aggregated proteins. Furthermore, constituents of functional assemblies can aggregate and become non-functional during aging. Here we present the current knowledge as to how coalescing protein assemblies are spatially organized in cells and we postulate that failures in their spatial confinement might underscore certain aspects of aging and neurodegenerative diseases.
INTRODUCTION
Many proteins undergo regulated large-scale conformational transitions driving their assembly into multimeric bodies that promote cellular adaptation.Citation1 In contrast, unwanted structural changes in proteins, caused for example by mutations or quality control defects, can lead to terminal protein aggregation – a hallmark of aging and several degenerative diseases.Citation2 Historically the process of protein misfolding has been intensively studied, as it is considered a major threat to cellular physiology. From studies conducted mainly with reporter systems that generate a burden of misfolded proteins we have learned that cells have evolved elaborate quality control mechanisms which can refold or degrade misfolded proteins. Moreover both the artificially induced conditions and different physiological stresses lead to the assembly of protein inclusion bodies. Under physiological heat stress, the coalescence of proteins protects the functionality of protein complexes and does not lead to protein degradation.Citation3 In the case of some proteopathic diseases, the large assembled states have been shown to be less harmful to the organism than oligomeric states.Citation2 Perhaps the most extreme example of protein alternative folding are prion diseases such as Creutzfeldt-Jakob's, which are based on the infectious propagation of self-templating alternative protein conformations. However, even for these, which appear to be malignant, there are functional equivalents in biology. For instance prion-like activity appears to have a conserved functional significance in memory encoding.Citation4,5 Thus the formation of dedicated deposits represents both physiological and aberrant states respectively. The fate of these coalescing proteins depends on the case involved: some proteins form singular inclusions that are not, for example, shared during cell division, whereas others remain dispersed and can spread during division and between neighboring cells. Thus, elucidating the mechanisms responsible for recognition and routing of proteins into dedicated deposits enables us to understand basic mechanisms of cellular organization and has major implications for biomedical research. To understand how these different states are discriminated and coordinated during dynamic processes such as cell division, we need a comprehensive investigation of the responsible molecular mechanisms that control protein coalescence in space and time. Here we discuss regulation of the spatial organization of coalescing proteins and provide examples that highlight the consequences of these mechanisms to processes such as aging, memory and degenerative diseases.
Formation of a Single Coalescent Body as a Means of Protecting the Cell and its Siblings
Protein aggregation in cells often results in the formation of a single inclusion body. This phenomenon is conserved from prokaryotic E. coli to human cells, indicating that the spatial sequestration of aggregating species into single inclusions is a highly selected trait. For instance aggregate markersCitation6,7 such as the small heat-shock protein IbpACitation8 form a single inclusion body in bacterial cells.Citation9 Also both fission and budding yeast cells eventually tend to form a single inclusion marked by the disaggregase Hsp104 when experiencing stress-induced protein aggregation.Citation10,11 Over-expression of unstable proteins can also lead to the formation of single-compartment specific- deposit sites termed IPOD, JUNQCitation12 and INQ.Citation13 During replicative aging the non-stressed cells, but not their rejuvenated daughter cells, form a single protein deposit.Citation14,15 Remarkably the single inclusions from 2 old cells rapidly merge to create a unique deposit after cell-cell fusion through mating.Citation16 Protein coalescence into a single deposit is not unique to single-celled organisms. After proteasome inhibition, misfolded proteins accumulate at a unique site termed the aggresome.Citation17 Many proteins accumulate to form the aggresome including Cystic Fibrosis Transmembrane Conductance Regulator (CFTR), huntingtin,Citation18 α-synuclein,Citation19,20 and ataxin-3.Citation21
Thus most organisms have cellular mechanisms that spatially constrain protein aggregates to a single deposit (), which for example enables the asymmetric inheritance of this inclusion during cell division. Indeed, inclusions are associated with the old pole of dividing bacterial cells.Citation8 As a consequence the cell inheriting the old poleCitation22 ages faster than its sibling which does not inherit protein aggregates.Citation6 In fission yeast the exposure to proteotoxic stress results in the emergence of aging by the cell that inherits the protein inclusion,Citation10 and this is critically regulated through the fusion of aggregates by the small heat-shock protein Hsp16.Citation23,24 Similarly, in budding yeast the singularity of protein inclusions is promoted by the small heat-shock protein Hsp42.Citation15,25 The coalescence to a single deposit is conserved from fungi to mammals, as it also promotes the asymmetric inheritance of damaged proteins during mammalian cell division and thereby provides a fitness advantage to cells that are free of damage.Citation26 In spinocerebellar ataxia type-3 aged patients, it was the differentiated but not the stem intestinal cells which accumulated ataxin and polyglutamine aggregates.Citation27 Taken together, a wide variety of examples demonstrates that cells form a single inclusion body which allows for a spatial confinement of protein damage. In the event of cell division, this confinement favors the asymmetric partitioning of the potentially problematic constituents of these structures. Adding to this, the coalescence of proteins to larger deposits is also involved in the asymmetric partitioning of physiologically relevant assemblies.
FIGURE 1. A. Proteins, under several physiological or pathological triggers can accumulate in deposit sites that have diverse biophysical properties. These transitions into large assemblies are controlled by chaperones, disaggregases and aggregases. Disaggregases such as Hsp104 in fungi or the Hsp40-Hsp70-Hsp110 complex in metazoans have the ability to fragment large assemblies into small oligomers that promote spreading of these aggregates. Hsp40s proteins, through their membrane attachment, enable spatial control of the coalescing proteins thereby facilitating the confinement of the assemblies and their precursors. B. In budding yeast, upon aging, aggregate precursors are collected by Ydj1 to a single deposit site localized at the ER membrane. Diffusion barriers in the ER membrane at the bud neck, involving ceramide enriched membrane domains, impair the free diffusion of certain membrane bound proteins from the aged mother cell to the rejuvenated bud. These include aggregate precursors that are attached to the ER membrane by farnesylated Ydj1. Upon prolonged pheromone treatment Whi3 forms superassemblies, yet their mode of formation and asymmetric inheritance are unknown. During aging, Whi3 also aggregates and becomes non functional.
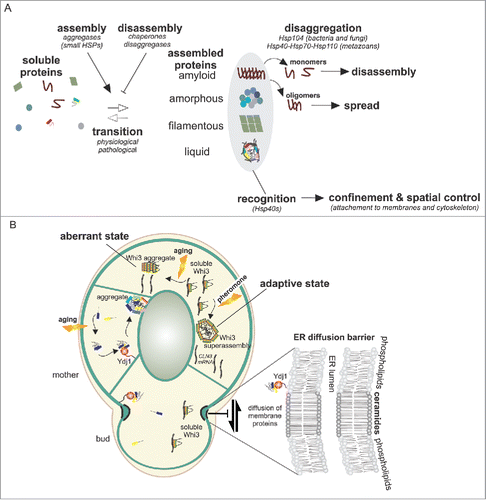
Many recent studies have highlighted the properties of various assemblies composed of proteins and in many cases RNA to partially isolate themselves from the surrounding cytoplasm through liquid-liquid phase separation.Citation28 Interestingly, these assemblies are reversible and often highly dynamic. The formation of such assemblies governs several cellular responses to environmental alterations.Citation1 For example, the soluble stress granule associated poly(A)-binding protein Pab1 assembles into hydrogels during thermal and pH stresses, and this phase transition promotes cellular fitness during stress.Citation29 Thus, coalescence of proteins to dedicated deposits is a conserved feature of cells in stressful situations and may also be used during physiological and unstressed conditions.
Protein Inclusions as Encoders of Asymmetrically Inherited Cellular Memories
A conserved process that relies on the mechanisms described above is the recently discovered encoding of memory through the assembly of activated mnemons or engrams.Citation4,5 When exposed to mating pheromone, budding yeast cells grow in a polarized manner (shmoo) toward the source of pheromone. If the mating partner is not encountered then the cell eventually stops shmooing and resumes vegetative growth to produce daughter cells even though pheromone is still present.Citation4,30 Remarkably, after resuming a cycling state, cells that have shmooed no longer respond to pheromone; however, daughter cells born from these mother cells still shmoo. A superassembly of the mRNA binding protein Whi3 was identified to encode this pheromone refractory state.Citation4 Importantly, this coalesced state of Whi3 remains in the mother cell during cell division, thus allowing the daughter cells to respond to pheromoneCitation4 (). Whi3 is the constituting member of mnemons – a class of proteins that are responsible for stable phenotypic states which are only present in those cells that experience the inducing signal. Whi3 aggregation is driven by a Q/N-rich prion-like domainCitation4,31 which is commonly found in eukaryotic proteomes,Citation31-33 thus giving rise to the question of whether mnemons exist outside of fungi.
In neurons of sea slugs, fruit flies and mice, the mRNA-binding and prion-like domain containing CPEB/Orb2 proteins are required for long-term memory due to their ability to undergo persistent aggregation upon synaptic stimulation.Citation34-37 The aggregation of CPEB proteins leads to translation of the mRNA targets, which in turn promote synaptic strength. This implies that mnemon-like activities in proteins are conserved and thus a prevalent form of molecular assemblies in the adaptive arsenal of eukaryotic cells. The overarching hypothesis of CPEB proteins would be that their aggregation at the activated synapse does not spread to neighboring synapses even though CPEB has prion properties in yeast.Citation34 How this coalesced memory-maintenance state is confined to the site of activation from yeast to mammals thus poses a perplexing question still to be answered. Similarly, whether or not the Whi3 mnemon adopts a self-templating prion-like state and how Whi3 super-assemblies are retained in the mother cell are unanswered questions.
How Are Coalescing Assemblies Spatially Confined in Cells?
As already detailed, the coalescence of diffusing particles is a powerful way to generate asymmetry.Citation15,23,24 However, this does not explain how coalescent bodies 1) form only in one of the 2 lineages and 2) are inherited by a specific lineage. Work in budding yeast has recently provided interesting insights into the mechanisms responsible for the asymmetric inheritance of different coalescent bodies that seem to use partially overlapping mechanisms. Heat-stress induced protein- coalescent bodies, for instance, localize to the ERCitation38 and preferentially to the vicinity of ER-mitochondria contact sites.Citation39 It has been suggested that these ER-mitochondria interfaces play an important role in asymmetric inheritance because the mitochondrial fission protein Fis1 was required for the maintenance of this asymmetry.Citation39 Surprisingly, it was recently found that at least some of these mitochondrially-targeted aggregated proteins are imported into the mitochondria where they are degraded by proteases such as Pim1.Citation40 Interestingly, the actin cytoskeleton also plays a role in asymmetric segregation of protein aggregates. Heat-induced aggregates associate with actin cables, and it has been suggested that this cable attachment results in passive transport of aggregates from the bud to the mother cell due to retrograde flow of polar actin cables.Citation41,42 More recently a link between actin, Hsp104 and the vacuolar protein Vac17 has revealed a potential role for endosomes in the collection of aggregates to a vacuole-associated inclusion which promotes their asymmetric inheritance.Citation43 Besides these active mechanisms, it has been suggested that the polarized growth and limited exchange of aggregates between mothers and buds is what largely accounts for the asymmetry.Citation10 Thus it appears that several overlapping mechanisms ensure the retention of stress-induced inclusions.
In contrast to stressed cells where the aggregates nucleate both in mothers and buds, during replicative aging the coalesced protein deposits appear only in the mother cell. Since the mechanisms ensuring proteostasis are not compromised during early aging,Citation15 we provided evidence that inclusions form through the collection of aggregate precursors which accumulate specifically in the mother cell over time. Somewhat similarly to other deposits, age-associated protein aggregates localize to the ER surfaces.Citation16 Taken together, the consensus from several aggregation models indicates that even tough they are membrane-less they localize and make use of endo-membranes to control their assembly and localization ().
This raises the question: How do the deposits and their precursors interact with cellular membranes? We identified the Hsp40 protein Ydj1 as a membrane anchor for aggregation precursors that were destined for the age-associated deposit.Citation16 Ydj1 is anchored to ER membranes by being post-translationally farnesylated in its C-terminal CAAX box. Citation44 We found that this lipidation of Ydj1 was required for the retention of aggregate seeds in the mother cellCitation16 (). This retention required the compartmentalization of the ER membrane by a diffusion barrier at the bud neck region.Citation45-47 The diffusion barriers are comprised of a sphingolipid-enriched lipid domain.Citation46 Thus it appears that these diffusion barriers can in part insulate cellular processes by repelling post-translationally lipidated proteins such as those harboring the branched and bulky farnesyl moiety. Since diffusion barriers are also found at the base of many cellular appendages and at the division plane of asymmetrically dividing metazoan cells,Citation48-50 this observation raises many interesting alternatives as to how cells might polarize their components so as to execute compartment-specific tasks. It is noteworthy that diffusion barriers and farnesylated Ydj1 are only confining aggregation precursors but not the large inclusions.Citation16 It is also interesting to note that, in addition to cytosolic aggregation precursors, misfolded proteins in the ER-lumen are also retained in the mother cell by diffusion barriers.Citation46 Taken together, we propose that the ER membrane functions as a platform to disaffiliate proteins which have changed their conformation from their native partners. This involves their recognition and anchorage to the ER by membrane-bound receptors such as DnaJ protein Ydj1, which facilitate the collection of deposit seeds into a single inclusion body only in the lineage that these precursors have formed.
Interestingly, in asymmetrically dividing neural stem cells of mice the asymmetric partition of ubiquitinated proteins also correlates with the strength of an ER diffusion barrier,Citation50 thus suggesting that some aspects are also likely to be conserved between budding yeast and mammalian cells. A recent study identified the deubiquitinase USP19 as an ER-bound receptor for misfolded proteins such as α-synuclein.Citation51 Intriguingly, this pathway promoted the secretion of misfolded proteins out of the cell, which could be impactful in cell-cell spread of certain aggregates. Thus, there are both similarities, but also clear mechanistic differences to what has been characterized in yeast. In cultured mammalian cells, the aggresome generally co-localizes with the centrosome. Disassembly of the microtubule network leads to dispersed fociCitation17 without disassembling the aggresome, suggesting that the formation but not the maintenance of aggresome is microtubule-dependent. Of note, a similar mechanism is used by the filamentous fungus Aspergillus nidulans, where the coalescence of heat-induced Hsp104-labeled aggregates was promoted by cytoplasmic microtubules and the motor protein dynein.Citation52 It might be that an increased concentration of smaller entities around the centrosome is sufficient to nucleate their fusion to a single and larger inclusion. Furthermore the intermediate filament vimentin cages the aggresomeCitation17 and may thus be involved in its maintenance.
In conclusion, many coalescing assemblies are captured by membranous and cytoskeletal structures in cells, which facilitates their spatial control. The most intensively studied budding yeast uses the following mechanisms to segregate protein inclusions to the mother cells: 1) coalesced proteins are collected at single deposit sites; 2) both large inclusions and their precursors are connected to internal membranes; 3) the retention of seeds is achieved through the compartmentalization of endo-membranes by diffusion barriers. However, there are also examples that appear to have escaped the singularity of most inclusions, evidenced most devastatingly by the spread of prion-like diseases. This gives rise to the question: How do certain coalescing conformers evade the existing confinement mechanisms?
Coalescing Assemblies that Spread Escape the Confinement
Not all aggregating proteins are confined during cell division. A prime example are prions that are formed by self-replicating altered protein conformations.Citation53 Prions were originally identified as infectious proteaceous elements that were later on shown to be composed of amyloid like fold of PrP protein, and to cause neurodegenerative disease.Citation54 It has now become evident that a large number of proteins can behave in a prion-like manner, and that this behavior underlies both diverse physiological processes and the pathogenicity of several human neurodegenerative diseases.Citation2,55,56 The alternative folds of prions can induce novel phenotypes deriving from a gain in the protein's function or a loss thereof which can in turn be beneficial or detrimental. In addition to canonical prions, proteins that harbor intrinsically disordered domains (IDRs) can adopt alternate non-amyloid-like states of activity which drive heritable traits.Citation57 Thus, canonical prions and some IDR-harboring proteins are able to evade the existing confinement mechanisms that were detailed in the previous chapters.
In budding yeast, prions represent heritable phenotypic switches that can drive cellular adaptation to changing environments; and hence prion states, for example the [PSI+] and the [MOT3+], are induced by environmental cues.Citation58,59,60 The [PSI+] prion formed by the altered conformation of the translation-termination factor Sup35 is the best characterized yeast prion. In its prion form, Sup35 exists in the equilibrium of soluble monomers and amyloid oligomers of various sizes. Importantly, the mitotic stability of the prion state critically depends on the existence of small oligomers that can diffuse from the mother to the bud. Oligomers tend to nucleate large inclusions which can be fragmented by the disaggregase Hsp104. If Hsp104 is disabled, propagons are unable to transmit to daughter cells.Citation61 Similarly, overexpression of Ydj1, which is involved in the compartmentalization of age-associated aggregates, promotes curing of the [PSI+] prion. Thus the transmission of propagons may be partially limited by the same pathway that prevents the diffusion of age-associate aggregate precursors from the aging mothers to the buds. Indeed, we found that detaching Ydj1 from the ER membrane intensified the Sup35 prion phenotype indicative of increased number of spreading propagons.Citation16 Interestingly, [PSI+] can also be propagated in S. pombeCitation62 and E. coli,Citation63,64 which in both cases requires the Hsp104/ClpB disaggregase activity for the mitotic spread. Thus, a balance between solubilization and aggregate fragmentation by disaggregases is critical for the mitotic stability of the prion state. However, not all prions depend on fragmentation by Hsp104, some require Hsp70 or Hsp90 chaperones for their propagation.Citation57 Overall, it is remarkable that the very same molecules which are induced during stress to prevent protein misfolding are also responsible for the spreading of prions. Furthermore, the fact that prions exist or can spread in organisms ranging from bacteria to fungi to plants to mammals suggests that the mechanisms which seed propagation of conformational states are conserved across most living organisms. Thus the next big challenge is to understand how the conformation-based recognition processes function to control the fate of aggregating proteins.
In mammals the conversion of the soluble prion protein PrPc(cellular) to the prion form PrPsc(scrapie) leads to self-sustaining formation of β-sheet-rich assemblies responsible for transmissible spongiform encephalopathies. Similar to yeast prions, PrPsc is found in various forms from small oligomers to large plaques. Mounting evidence suggests that the progression of many incurable neurodegenerative diseases such as frontotemporal dementia, Alzheimer, Huntington's and Parkinson disease might be underscored by prion-like spread (analogous to PrPsc) of aggregating proteins within the nervous system. However, in contrast to the prion PrPsc, the causative misfolded proteins driving these diseases are not considered infectious and are thus referred to as prionoidsCitation2 – although the potential human-to-human transmission of proteopathic Aβ seeds has recently been suggested.Citation65 As we have learned from yeast, the key determinant for spreading prions is their ability to self-propagate, which depends on the existence of small diffusible oligomers generated from the fragmentation of larger assemblies. While metazoans lack the Hsp104 disaggregases, the disaggregation is performed by a chaperone complex comprising Hsp110-Hsp40 and Hsp70.Citation66-68 In addition the PDZ serine protease HtrA1 has been found to dissolve and degrade Alzheimer disease-related tau and Aβ42 fibrils.Citation69,70 Thus it appears that, similar to yeast, the Hsp40 DnaJ proteins are in a key position to regulate the fate of aggregating proteins. In humans the DnaJ-proteins are comprised of approximately 50 proteins and such divergence could provide specificity for a large conformational landscape of different types of aggregates. Moreover, at least some of the human DnaJ-proteins such as DnaJ-A1 harbor a farnesylation motif, which implies that in addition to being adaptors for disaggregation and degradation they might also function as membrane-anchored receptors for aggregates perhaps subjecting them to diffusion barrier-mediated confinement.
Altogether it appears that the mechanisms of confinement and spreading may be dictated by the very same proteins but helped by recognition factors such as DnaJ proteins. Remarkably, the genome of Plasmodium falciparum encodes for more than 40 DnaJ proteins.Citation71 While P. falciparum has a small proteome (5268 proteins), nearly a quarter of its proteins contain a prion-like domain, suggesting that the repertoire of Hsp40 may have expanded to cope with such a proteome. This is particularly interesting because this parasite has to adapt to its different hosts' diverse environmental conditions such as temperature that might act as a cue to trigger adaptative prion-like domain containing protein assemblies. Thus, a fascinating avenue of future research is whether the DnaJ proteins and their potential to interact with membranes have co-evolved with the conformational landscape of coalescent proteins.
Are Dysfunctional Phase Transitions and Problems in their Spatial Confinement Drivers of Aging?
Aging is a major risk factor for most neurodegenerative diseases. However, we know little about how the sporadic forms of these protein-aggregation diseases are triggered and what leads to the progressive and eventually detrimental spread of the aggregation phenotype within the central nervous system. Research on model organisms for cellular and organismal aging has been and will continue to be very informative in understanding the cellular responses to aging and disease-associated aggregation. For instance work on budding yeast has shown that protein aggregates are a contributing factor to yeast-replicative aging – although at present it is not exactly known why age-associated aggregates form and how they limit the replicative life span. Our view is that by harboring a large repertoire of functionally coalescing proteins (e.g. those with prion-like domains), cells may become more susceptible to aberrant protein aggregations if such proteins escape their normal quality control. Such aberrant transitions can contribute to age-associated decline in organismal fitness. Indeed, the Whi3 protein that constitutes the functional memory (mnemon) of pheromone exposure readily aggregates erratically during aping, thereby impairing the mating ability of aged cells.Citation72
Similar to yeast cells, stem cells undergo replicative aging, i.e. their ability to undergo self-renewing divisions is limited.Citation73 Since stem cells are required for tissue regeneration, their declining ability to self-renew may contribute to tissue dysfunction and age-associated diseases. Because stem cells can divide asymmetrically into renewing and differentiating cells there is great interest in attaining an understanding of to what extent accumulation of cellular damages and/or impairment in asymmetric division could potentially contribute to the observed decline of stem cells to undergo self-renewing divisions during aging.Citation74 For instance, one possibility is that protein aggregates which may arise during aging could contribute to the observed decline in stem-cell renewal. Importantly, both diffusion-barrier strength and asymmetric inheritance of damaged proteins decreased with age in the neural stem cells of mice.Citation50
In summary, we are beginning to understand how cells organize themselves in response to internal or external changes, either by reducing non-functional and hazardous protein species through the formation of inclusion bodies, or by organizing their biochemical activities through the formation of large-scale assemblies. In both cases the outcome critically depends on how these assemblies are distributed in space. Thus, understanding how distinct assemblies are formed, recognized and controlled in space and time are major questions for future research.
DISCLOSURE OF POTENTIAL CONFLICTS OF INTEREST
No potential conflicts of interest were disclosed.
REFERENCES
- Saarikangas J, Barral Y. Protein aggregation as a mechanism of adaptive cellular responses. Curr Genet 2016; 62:711-24; PMID:27032776; https//doi.org/10.1007/s00294-016-0596-0
- Aguzzi A, Lakkaraju AKK. Cell biology of Prions and Prionoids: A status report. Trends Cell Biol 2016; 26:40-51; PMID:26455408; https//doi.org/10.1016/j.tcb.2015.08.007
- Wallace EWJ, Kear-Scott JL, Pilipenko EV, Schwartz MH, Laskowski PR, Rojek AE, Katanski CD, Riback JA, Dion MF, Franks AM, et al. Reversible, specific, active aggregates of endogenous proteins assemble upon heat stress. Cell 2015; 162:1286-98; PMID:26359986; https//doi.org/10.1016/j.cell.2015.08.041
- Caudron F, Barral Y. A super-assembly of Whi3 encodes memory of deceptive encounters by single cells during yeast courtship. Cell 2013; 155:1244-57; PMID:24315096; https//doi.org/10.1016/j.cell.2013.10.046
- Li L, Sanchez CP, Slaughter BD, Zhao Y, Khan MR, Unruh JR, Rubinstein B, Si K. A putative biochemical engram of long-term memory. Curr Biol 2016; 26(23):3143-56; PMID:27818176; https//doi.org/10.1016/j.cub.2016.09.054
- Winkler J, Seybert A, König L, Pruggnaller S, Haselmann U, Sourjik V, Weiss M, Frangakis AS, Mogk A, Bukau B. Quantitative and spatio-temporal features of protein aggregation in Escherichia coli and consequences on protein quality control and cellular ageing. EMBO J 2010; 29:910-23; PMID:20094032; https//doi.org/10.1038/emboj.2009.412
- Lloyd-Price J, Häkkinen A, Kandhavelu M, Marques IJ, Chowdhury S, Lihavainen E, Yli-Harja O, Ribeiro AS. Asymmetric disposal of individual protein aggregates in Escherichia coli, one aggregate at a time. J Bacteriol 2012; 194:1747-52; PMID:22287517; https//doi.org/10.1128/JB.06500-11
- Lindner AB, Madden R, Demarez A, Stewart EJ, Taddei F. Asymmetric segregation of protein aggregates is associated with cellular aging and rejuvenation. Proc Natl Acad Sci USA 2008; 105:3076-81; PMID:18287048; https//doi.org/10.1073/pnas.0708931105
- Carrió MM, Corchero JL, Villaverde A. Dynamics of in vivo protein aggregation: building inclusion bodies in recombinant bacteria. FEMS Microbiol Lett 1998; 169:9-15; PMID:9851031; https//doi.org/10.1016/S0378-1097(98)00444-3
- Coelho M, Dereli A, Haese A, Kühn S, Malinovska L, DeSantis ME, Shorter J, Alberti S, Gross T, Tolić-Nørrelykke IM. Fission yeast does not age under favorable conditions, but does so after stress. Curr Biol 2013; 23:1844-52; PMID:24035542; https//doi.org/10.1016/j.cub.2013.07.084
- Paoletti C, Quintin S, Matifas A, Charvin G. Kinetics of formation and asymmetrical distribution of Hsp104-bound protein aggregates in yeast. Biophys J 2016; 110:1605-14; PMID:27074685; https//doi.org/10.1016/j.bpj.2016.02.034
- Kaganovich D, Kopito R, Frydman J. Misfolded proteins partition between two distinct quality control compartments. Nature 2008; 454:1088-95; PMID:18756251; https//doi.org/10.1038/nature07195
- Miller SBM, Ho C-T, Winkler J, Khokhrina M, Neuner A, Mohamed MYH, Guilbride DL, Richter K, Lisby M, Schiebel E, et al. Compartment-specific aggregases direct distinct nuclear and cytoplasmic aggregate deposition. EMBO J 2015; 34:778-97; PMID:25672362; https//doi.org/10.15252/embj.201489524
- Erjavec N, Larsson L, Grantham J, Nyström T. Accelerated aging and failure to segregate damaged proteins in Sir2 mutants can be suppressed by overproducing the protein aggregation-remodeling factor Hsp104p. Genes Dev 2007; 21:2410-21; PMID:17908928; https//doi.org/10.1101/gad.439307
- Saarikangas J, Barral Y. Protein aggregates are associated with replicative aging without compromising protein quality control. Elife 2015; 4:1751; https//doi.org/10.7554/eLife.06197
- Saarikangas J, Caudron F, Prasad R, Moreno DF, Bolognesi A, Aldea M, Barral Y. Compartmentalization of ER-Bound Chaperone Confines Protein Deposit Formation to the Aging Yeast Cell. Curr Biol 2017; 27:773-83; PMID:28262489; https//doi.org/10.1016/j.cub.2017.01.069
- Johnston JA, Ward CL, Kopito RR. Aggresomes: a cellular response to misfolded proteins. J Cell Biol 1998; 143:1883-98; PMID:9864362; https//doi.org/10.1083/jcb.143.7.1883
- Waelter S, Boeddrich A, Lurz R, Scherzinger E, Lueder G, Lehrach H, Wanker EE. Accumulation of mutant huntingtin fragments in aggresome-like inclusion bodies as a result of insufficient protein degradation. Mol Biol Cell 2001; 12:1393-407; PMID:11359930; https//doi.org/10.1091/mbc.12.5.1393
- Lee HJ, Lee SJ. Characterization of cytoplasmic alpha-synuclein aggregates. Fibril formation is tightly linked to the inclusion-forming process in cells. J Biol Chem 2002; 277:48976-83; PMID:12351642; https//doi.org/10.1074/jbc.M208192200
- Tanaka M, Kim YM, Lee G, Junn E, Iwatsubo T, Mouradian MM. Aggresomes formed by alpha-synuclein and synphilin-1 are cytoprotective. J Biol Chem 2004; 279:4625-31; PMID:14627698; https//doi.org/10.1074/jbc.M310994200
- Ma H, Xiong H, Liu T, Zhang L, Godzik A, Zhang Z. Aggregate formation and synaptic abnormality induced by DSCR1. J Neurochem 2004; 88:1485-96; PMID:15009650; https//doi.org/10.1046/j.1471-4159.2003.02294.x
- Stewart EJ, Madden R, Paul G, Taddei F. Aging and death in an organism that reproduces by morphologically symmetric division. PLoS Biol 2005; 3:e45; PMID:15685293; https//doi.org/10.1371/journal.pbio.0030045
- Lade SJ, Coelho M, Tolić IM, Gross T. Fusion leads to effective segregation of damage during cell division: An analytical treatment. J Theor Biol 2015; 378:47-55; PMID:25934351; https//doi.org/10.1016/j.jtbi.2015.04.022
- Coelho M, Lade SJ, Alberti S, Gross T, Tolić IM. Fusion of protein aggregates facilitates asymmetric damage segregation. PLoS Biol 2014; 12:e1001886; PMID:24936793; https//doi.org/10.1371/journal.pbio.1001886
- Specht S, Miller SBM, Mogk A, Bukau B. Hsp42 is required for sequestration of protein aggregates into deposition sites in Saccharomyces cerevisiae. J Cell Biol 2011; 195:617-29; PMID:22065637; https//doi.org/10.1083/jcb.201106037
- Ogrodnik M, Salmonowicz H, Brown R, Turkowska J, Średniawa W, Pattabiraman S, Amen T, Abraham A-C, Eichler N, Lyakhovetsky R, et al. Dynamic JUNQ inclusion bodies are asymmetrically inherited in mammalian cell lines through the asymmetric partitioning of vimentin. Proc Natl Acad Sci USA 2014; 111:8049-54; PMID:24843142; https//doi.org/10.1073/pnas.1324035111
- Rujano MA, Bosveld F, Salomons FA, Dijk F, van Waarde MAWH, van der Want JJL, de Vos RAI, Brunt ER, Sibon OCM, Kampinga HH. Polarised asymmetric inheritance of accumulated protein damage in higher eukaryotes. PLoS Biol 2006; 4:e417; PMID:17147470; https//doi.org/10.1371/journal.pbio.0040417
- Hyman AA, Weber CA, Jülicher F. Liquid-liquid phase separation in biology. Annu Rev Cell Dev Biol 2014; 30:39-58; PMID:25288112; https//doi.org/10.1146/annurev-cellbio-100913-013325
- Riback JA, Katanski CD, Kear-Scott JL, Pilipenko EV, Rojek AE, Sosnick TR, Drummond DA. Stress-Triggered phase separation is an adaptive, evolutionarily tuned response. Cell 2017; 168:1028-1040.e19; PMID:28283059; https//doi.org/10.1016/j.cell.2017.02.027
- Moore SA. Yeast cells recover from mating pheromone alpha factor-induced division arrest by desensitization in the absence of alpha factor destruction. J Biol Chem 1984; 259:1004-10; PMID:6363399
- Alberti S, Halfmann R, King O, Kapila A, Lindquist S. A systematic survey identifies prions and illuminates sequence features of prionogenic proteins. Cell 2009; 137:146-58; PMID:19345193; https//doi.org/10.1016/j.cell.2009.02.044
- King OD, Gitler AD, Shorter J. The tip of the iceberg: RNA-binding proteins with prion-like domains in neurodegenerative disease. Brain Res 2012; 1462:61-80; PMID:22445064; https//doi.org/10.1016/j.brainres.2012.01.016
- Malinovska L, Kroschwald S, Alberti S. Protein disorder, prion propensities, and self-organizing macromolecular collectives. Biochim Biophys Acta 2013; 1834:918-31; PMID:23328411; https//doi.org/10.1016/j.bbapap.2013.01.003
- Si K, Lindquist S, Kandel ER. A neuronal isoform of the aplysia CPEB has prion-like properties. Cell 2003; 115:879-91; PMID:14697205; https//doi.org/10.1016/S0092-8674(03)01020-1
- Si K, Giustetto M, Etkin A, Hsu R, Janisiewicz AM, Miniaci MC, Kim J-H, Zhu H, Kandel ER. A neuronal isoform of CPEB regulates local protein synthesis and stabilizes synapse-specific long-term facilitation in aplysia. Cell 2003; 115:893-904; PMID:14697206; https//doi.org/10.1016/S0092-8674(03)01021-3
- Si K, Choi Y-B, White-Grindley E, Majumdar A, Kandel ER. Aplysia CPEB can form prion-like multimers in sensory neurons that contribute to long-term facilitation. Cell 2010; 140:421-35; PMID:20144764; https//doi.org/10.1016/j.cell.2010.01.008
- Keleman K, Krüttner S, Alenius M, Dickson BJ. Function of the Drosophila CPEB protein Orb2 in long-term courtship memory. Nat Neurosci 2007; 10:1587-93; PMID:17965711; https//doi.org/10.1038/nn1996
- Escusa-Toret S, Vonk WIM, Frydman J. Spatial sequestration of misfolded proteins by a dynamic chaperone pathway enhances cellular fitness during stress. Nat Cell Biol 2013; 15:1231-43; PMID:24036477; https//doi.org/10.1038/ncb2838
- Zhou C, Slaughter BD, Unruh JR, Guo F, Yu Z, Mickey K, Narkar A, Ross RT, McClain M, Li R. Organelle-based aggregation and retention of damaged proteins in asymmetrically dividing cells. Cell 2014; 159:530-42; PMID:25417105; https//doi.org/10.1016/j.cell.2014.09.026
- Ruan L, Zhou C, Jin E, Kucharavy A, Zhang Y, Wen Z, Florens L, Li R. Cytosolic proteostasis through importing of misfolded proteins into mitochondria. Nature 2017; 543(7645):443-446; PMID:28241148; https//doi.org/10.1038/nature21695
- Liu B, Larsson L, Caballero A, Hao X, Oling D, Grantham J, Nyström T. The polarisome is required for segregation and retrograde transport of protein aggregates. Cell 2010; 140:257-67; PMID:20141839; https//doi.org/10.1016/j.cell.2009.12.031
- Liu B, Larsson L, Franssens V, Hao X, Hill SM, Andersson V, Höglund D, Song J, Yang X, Oling D, et al. Segregation of protein aggregates involves actin and the polarity machinery. Cell 2011; 147:959-61; PMID:22118450; https//doi.org/10.1016/j.cell.2011.11.018
- Hill SM, Hao X, Grönvall J, Spikings-Nordby S, Widlund PO, Amen T, Jörhov A, Josefson R, Kaganovich D, Liu B, et al. Asymmetric inheritance of aggregated proteins and age reset in yeast are regulated by Vac17-dependent vacuolar functions. Cell Rep 2016; 16:826-38; PMID:27373154; https//doi.org/10.1016/j.celrep.2016.06.016
- Caplan AJ, Tsai J, Casey PJ, Douglas MG. Farnesylation of YDJ1p is required for function at elevated growth temperatures in Saccharomyces cerevisiae. 1992; 267:18890-5.
- Luedeke C, Frei SB, Sbalzarini I, Schwarz H, Spang A, Barral Y. Septin-dependent compartmentalization of the endoplasmic reticulum during yeast polarized growth. J Cell Biol 2005; 169:897-908; PMID:15967812; https//doi.org/10.1083/jcb.200412143
- Clay L, Caudron F, Denoth-Lippuner A, Boettcher B, Buvelot Frei S, Snapp EL, Barral Y. A sphingolipid-dependent diffusion barrier confines ER stress to the yeast mother cell. Elife 2014; 3:e01883; PMID:24843009; https//doi.org/10.7554/eLife.01883
- Shcheprova Z, Baldi S, Frei SB, Gonnet G, Barral Y. A mechanism for asymmetric segregation of age during yeast budding. Nature 2008; 454:728-34; PMID:18660802
- Hu Q, Milenkovic L, Jin H, Scott MP, Nachury MV, Spiliotis ET, Nelson WJ. A septin diffusion barrier at the base of the primary cilium maintains ciliary membrane protein distribution. Science 2010; 329:436-9; PMID:20558667; https//doi.org/10.1126/science.1191054
- Ewers H, Tada T, Petersen JD, Racz B, Sheng M, Choquet D. A Septin-dependent diffusion barrier at dendritic spine necks. PLoS One 2014; 9:e113916; PMID:25494357; https//doi.org/10.1371/journal.pone.0113916
- Moore DL, Pilz GA, Araúzo-Bravo MJ, Barral Y, Jessberger S. A mechanism for the segregation of age in mammalian neural stem cells. Science 2015; 349:1334-8; PMID:26383951; https//doi.org/10.1126/science.aac9868
- Lee J-G, Takahama S, Zhang G, Tomarev SI, Ye Y. Unconventional secretion of misfolded proteins promotes adaptation to proteasome dysfunction in mammalian cells. Nat Cell Biol 2016; 18:765-76; PMID:27295555; https//doi.org/10.1038/ncb3384
- Egan MJ, McClintock MA, Hollyer IHL, Elliott HL, Reck-Peterson SL. Cytoplasmic dynein is required for the spatial organization of protein aggregates in filamentous fungi. Cell Rep 2015; 11:201-9; PMID:25865884; https//doi.org/10.1016/j.celrep.2015.03.028
- Liebman SW, Chernoff YO. Prions in yeast. Genetics 2012; 191:1041-72; PMID:22879407; https//doi.org/10.1534/genetics.111.137760
- Colby DW, Prusiner SB. Prions. Cold Spring Harb Perspect Biol 2011; 3:a006833; PMID:21421910; https//doi.org/10.1101/cshperspect.a006833
- Newby GA, Lindquist S. Blessings in disguise: biological benefits of prion-like mechanisms. Trends Cell Biol 2013; 23:251-9; PMID:23485338; https//doi.org/10.1016/j.tcb.2013.01.007
- Si K. Prions: what are they good for? Annu Rev Cell Dev Biol 2015; 31:149-69; PMID:26407211; https//doi.org/10.1146/annurev-cellbio-100913-013409
- Chakrabortee S, Byers JS, Jones S, Garcia DM, Bhullar B, Chang A, She R, Lee L, Fremin B, Lindquist S, et al. Intrinsically disordered proteins drive emergence and inheritance of biological traits. Cell 2016; 167:369-381.e12; PMID:27693355; https//doi.org/10.1016/j.cell.2016.09.017
- Tyedmers J, Madariaga ML, Lindquist S. Prion switching in response to environmental stress. PLoS Biol 2008; 6:e294; PMID:19067491; https//doi.org/10.1371/journal.pbio.0060294
- Holmes DL, Lancaster AK, Lindquist S, Halfmann R. Heritable remodeling of yeast multicellularity by an environmentally responsive prion. Cell 2013; 153:153-65; PMID:23540696; https//doi.org/10.1016/j.cell.2013.02.026
- Garcia DM, Dietrich D, Clardy J, Jarosz DF. A common bacterial metabolite elicits prion-based bypass of glucose repression. Elife 2016; 5:e17978; PMID:27906649; https//doi.org/10.7554/eLife.17978
- Chernoff YO, Lindquist SL, Ono B, Inge-Vechtomov SG, Liebman SW. Role of the chaperone protein Hsp104 in propagation of the yeast prion-like factor [psi+]. Science 1995; 268:880-4; PMID:7754373; https//doi.org/10.1126/science.7754373
- Sideri T, Yashiroda Y, Ellis DA, Rodríguez-López M, Yoshida M, Tuite MF, Bähler J. The copper transport-associated protein Ctr4 can form prion-like epigenetic determinants in Schizosaccharomyces pombe. Microb Cell 2017; 4:16-28; PMID:28191457; https//doi.org/10.15698/mic2017.01.552
- Garrity SJ, Sivanathan V, Dong J, Lindquist S, Hochschild A. Conversion of a yeast prion protein to an infectious form in bacteria. Proc Natl Acad Sci USA 2010; 107:10596-601; PMID:20484678; https//doi.org/10.1073/pnas.0913280107
- Yuan AH, Garrity SJ, Nako E, Hochschild A. Prion propagation can occur in a prokaryote and requires the ClpB chaperone. Elife 2014; 3:e02949; PMID:25122461; https//doi.org/10.7554/eLife.02949
- Jaunmuktane Z, Mead S, Ellis M, Wadsworth JDF, Nicoll AJ, Kenny J, Launchbury F, Linehan J, Richard-Loendt A, Walker AS, et al. Evidence for human transmission of amyloid-β pathology and cerebral amyloid angiopathy. Nature 2015; 525:247-50; PMID:26354483; https//doi.org/10.1038/nature15369
- Shorter J. The mammalian disaggregase machinery: Hsp110 synergizes with Hsp70 and Hsp40 to catalyze protein disaggregation and reactivation in a cell-free system. PLoS One 2011; 6:e26319; PMID:22022600; https//doi.org/10.1371/journal.pone.0026319
- Rampelt H, Kirstein-Miles J, Nillegoda NB, Chi K, Scholz SR, Morimoto RI, Bukau B. Metazoan Hsp70 machines use Hsp110 to power protein disaggregation. EMBO J 2012; 31:4221-35; PMID:22990239; https//doi.org/10.1038/emboj.2012.264
- Nillegoda NB, Kirstein J, Szlachcic A, Berynskyy M, Stank A, Stengel F, Arnsburg K, Gao X, Scior A, Aebersold R, et al. Crucial HSP70 co-chaperone complex unlocks metazoan protein disaggregation. Nature 2015; 524:247-51; PMID:26245380; https//doi.org/10.1038/nature14884
- Tennstaedt A, Pöpsel S, Truebestein L, Hauske P, Brockmann A, Schmidt N, Irle I, Sacca B, Niemeyer CM, Brandt R, et al. Human high temperature requirement serine protease A1 (HTRA1) degrades tau protein aggregates. J Biol Chem 2012; 287:20931-41; PMID:22535953; https//doi.org/10.1074/jbc.M111.316232
- Poepsel S, Sprengel A, Sacca B, Kaschani F, Kaiser M, Gatsogiannis C, Raunser S, Clausen T, Ehrmann M. Determinants of amyloid fibril degradation by the PDZ protease HTRA1. Nat Chem Biol 2015; 11:862-9; PMID:26436840; https//doi.org/10.1038/nchembio.1931
- Pesce ER, Blatch GL. Plasmodial Hsp40 and Hsp70 chaperones: current and future perspectives. Parasitology 2014; 141:1167-76; PMID:24666996; https//doi.org/10.1017/S003118201300228X
- Schlissel G, Krzyzanowski MK, Caudron F, Barral Y, Rine J. Aggregation of the Whi3 protein, not loss of heterochromatin, causes sterility in old yeast cells. Science 2017; 355:1184-7; PMID:28302853; https//doi.org/10.1126/science.aaj2103
- Goodell MA, Rando TA. Stem cells and healthy aging. Science 2015; 350:1199-204; PMID:26785478; https//doi.org/10.1126/science.aab3388
- Moore DL, Jessberger S. Creating age asymmetry: Consequences of inheriting damaged goods in mammalian cells. Trends Cell Biol 2017; 27:82-92; PMID:27717533; https//doi.org/10.1016/j.tcb.2016.09.007