Abstract
Despite an increasing knowledge about the causes of cancer, this disease is difficult to cure and still causes far too high a death rate. Based on advances in our understanding of disease pathogenesis, novel treatment concepts, including targeting the tumor microenvironment, have been developed and are being combined with established treatment regimens such as surgical removal and radiotherapy. Yet it is obvious that we need additional strategies to prevent tumor relapse and metastasis. Given its exceptional high expression in most cancers with low abundance in normal tissues, tenascin-C appears an ideal candidate for tumor treatment. Here, we will summarize the current applications of targeting tenascin-C as a treatment for different tumors, and highlight the potential of this therapeutic approach.
Introduction
Over the past decades an increasing knowledge about the underlying causes of cancer has been acquired. Yet long term survival of cancer patients, in particular those with very malignant cancers such as glioblastoma and pancreatic cancer, is still very poor. Thus it is mandatory to improve the current treatment strategies and to develop new ones. Current strategies of cancer therapy are represented by surgical removal of cancerous tissue, often followed by irradiation and chemotherapy. In some cancer types these treatments cause shrinkage or apparent elimination of the primary tumor, yet in other cancer types the tumors become resistant and often metastasize. Moreover, irradiation and cytotoxic chemotherapy are frequently accompanied by severe side effects to intact organs impacting on the well-being of the patient. The latter aspect is highly relevant in terms of long-term survival given that a high percentage of the population is facing cancer during their ever increasing life span. Today it is known that a successful anti-cancer treatment does not only need to take into account the elimination of the cancer cells, but should also destroy the tumor bed ideally by reversion to conditions found in normal tissue. Since our understanding about the tumor specific microenvironment is still poor, specific and tailored targeting is rather difficult and as for example in case of the anti angiogenic approach, that nowadays is used as second or third line therapy in some cancers, is often less successful as anticipated or even metastasis promoting.Citation1 Due to its peculiar high expression in cancer tissue and functional link to tumor progression tenascin-C appears an excellent target for anti-cancer treatment (reviewed inCitation2). Several novel approaches have been developed over the past years such as the application of tenascin-C specific antibodies to deliver drugs and irradiation into cancer tissue, as well as peptides, nucleic acids or protein inhibitors to interfere with the expression or cancer specific effects of tenascin-C (reviewed inCitation2). Here we will review tenascin-C targeting concepts and approaches, among which some have already been used in human cancer patients. Since radiotherapy is a cornerstone of cancer management protocols, finally we will also address how currently applied radiotherapy in cancer impacts on tenascin-C expression and whether and how this potentially affects tumor relapse/secondary tumor formation and progression.
Exploiting High Tenascin-C Expression in Cancer for Diagnosis and Targeting
Here, we focused on how the expression of tenascin-C could be exploited for diagnosis and targeting because tenascin-C is highly expressed in cancer tissue where it can correlate with poor prognosis and tumor progression.Citation2,3 Formal evidence of its tumorigenesis promoting activity has recently been demonstrated in the first study using a stochastic murine multistage tumorigenesis model displaying either abundant or no tenascin-C.Citation4 Here tenascin-C promoted several events such as survival, proliferation, invasion, angiogenesis and lung metastasis formation by a mechanism that involved downregulation of Dickkopf-1 (DKK1) and activation of Wnt signaling.Citation4 Thus high expression of tenascin-C in malignant cancers might be useful for diagnosis. Indeed over the last years good antibodies have been generated that allow detection of tenascin-C expression by immunohistochemistry. Tissue staining is already applied in assessment of cancer and other diseases with high expression of tenascin-C such as fibrosisCitation5,6 and inflammation in myocarditis.Citation7
Tenascin-C is also found in body fluids such as blood and milk.Citation8,9 In patients with inflammatory bowel disease high tenascin-C serum levels correlated with disease severity and treatment response, thus could be suitable for disease assessment.Citation10 Whether tenascin-C levels in the blood of cancer patients have a prognostic value is currently not clear since the limited information derived from a study in colon cancer patients did not provide evidence for such a correlation.Citation8 Moreover, it was shown that serum level of tenascin-C has no predictive or prognostic roles on survival of epithelial ovarian cancer or breast cancer patients.Citation11,12 These data suggest that tenascin-C expression as cancer marker needs to be considered in a broader context. Tenascin-C was found to be upregulated together with several other ECM molecules in the so called AngioMatrix that is upregulated during the angiogenic switch of an experimental insulinoma model. Importantly, this gene signature had diagnostic value since its high expression correlated with shorter survival of colon cancer and glioma patients.Citation13 Thus we may need to revisit these results to define a limited number of genes that altogether could have the potential as diagnostic tool for the development of cancer blood screening tests in the future.
Since tenascin-C has pleiotropic effects on the behavior of tumor and stromal cells, in this way shaping the tumor microenvironment/tumor bed one could consider either interfering with the specific effects of tenascin-C and their underlying molecular mechanisms and/or directly blocking expression of tenascin-C as tools to combat cancer. Here, we will address both concepts and critically evaluate how our current knowledge about tenascin-C could be exploited for an anti-cancer treatment. There are several questions arising as e.g. how important is tenascin-C in the tumor stroma and how early is it expressed to potentially having an impact on shaping the tumor microenvironment? Would inhibition of tenascin-C expression be sufficient to prevent tumor onset and/or counteract tumor progression? Which cells express tenascin-C? Could it be useful to kill these cells comprising tumor and stromal cells (with insufficient information which ones are playing a role at which stage of tumorigenesis) to “clean” the tumor bed from tenascin-C? Would elimination of tenascin-C alone have an impact?
There is evidence for a potential early role of tenascin-C in the tumor stroma. Transgenic expression of stromelysin-1/MMP3 under control of the whey acidic protein promoter in the mammary gland led to massive remodeling of the tissue involving basement membrane disruption and tenascin-C induction.Citation14 These mice spontaneously developed premalignant and malignant lesions.Citation15 Moreover, tenascin-C was shown to promote luminal filling of MCF7 derived acini like structures and basement membrane disruption, 2 early events during mammary gland tumorigenesis. This could be blocked by c-Met inhibitors.Citation16
Tenascin-C may act in concert with other ECM molecules as suggested by its presence in the AngioMatrix that is induced during the angiogenic switch (representing an early step toward tumor progression). Tenascin-C was among the most highly induced genes of the AngioMatrix and, in the absence of tenascin-C the angiogenic switch was significantly reduced whereas it was increased in comparison to control conditions with already high levels upon expression of additional tenascin-C from a transgene.Citation4,13 These observations suggest 2 things, that tenascin-C is important and that it may work in concert with other ECM molecules. Tenascin-C seems to be part of a complex ECM network that has been described as matrix tracks/channels in melanomaCitation17 and other cancers associated with enhanced metastasis, thus presumably generating physical niches with particular biochemical properties not only during angiogenesis but also during metastasis which may have an impact on drug responsiveness (see Spenlé et al., this issue and reviewsCitation18 andCitation19).
Natural inhibition of tenascin-C expression
One question that arises from these data is whether we can use information about regulation of tenascin-C expression to prevent its induction during tumorigenesis? How the expression of tenascin-C is regulated has been intensively reviewed in this issue by Chiovaro and colleagues and elsewhere.Citation20 Several molecular pathways have been identified to induce tenascin-C expression including TGFβ and Wnt among others. A general inhibition of these pathways certainly will have too pleiotropic and presumably unwanted effects and thus would be unsuitable approaches. However could we exploit negative regulation of tenascin-C as potential means to block its expression in cancer? It was shown that miR-126 and miR-335 as well as GATA-6 binding factors and corticoids downregulate mRNA levels of tenascin-C (reviewed in Chiovaro et al., this issue). In light of these results blocking inflammation by corticoids could be useful as anti-cancer treatment to block tenascin-C expression.
Targeting tenascin-C expression with RNA interference technology
Recently novel RNA based technologies have been developed with the aim to specifically downregulate expression of tenascin-C (ribozymes, small interfering RNAs (siRNA)) or to deliver drugs into the tenascin-C rich microenvironment (aptamers, see below). Although the use of antagomirs is still technically demanding and poses many difficulties, tenascin-C expression was successfully targeted with ATN‑RNA (anti-tenascin-C-RNA), a double stranded RNA homologous to tenascin-C mRNA triggering its degradation. This approach was first tested in a few patientsCitation21,22 and then extended to 46 patients with grade II, III and IV glioma where ATN-RNA had been injected into the area of neoplastic infiltration upon surgical resection of the primary or recurrent tumors.Citation23 Patient outcome was assessed as overall survival (OS) and quality of life (Karnofsky Performance Scale) in comparison to 48 patients that had obtained only brachytherapy. First, it was noted that the well-being of the patient was better with ATN-RNA treatment. Moreover, OS in all 3 patient groups was increased by 4.8 weeks (grade II from 176.1 to 180.9 weeks), 13.2 weeks (grade III from 59.1 to 72.3 weeks) and 13.9 weeks with grade IV disease/glioblastoma (from 52.8 to 66.7 weeks) ().Citation23 Despite some benefit it is also clear that this treatment only provided a short survival prolongation. Based on in vitro stimulation of cultured cancer cells followed by analysis of the interferon response, the authors excluded that the effect is mediated by an innate immune response. This was not investigated in the treated patients; hence an interferon response toward the foreign RNA potentially may have had an impact on patient outcome. Inclusion of a patient group receiving non targeting RNA would clarify whether inhibition of tenascin-C together with a possible innate immune response potentially has synergistic effects.
Table 1. Strategies for tenascin-C targeted cancer therapeutics
Blocking tenascin-C inducing signaling with inhibitory drugs
Other potential strategies targeting phosphodiesterase III, angiotensin II type 1 receptor (AT-1) and angiotensin converting enzyme (ACE) had already been described elsewhereCitation2,18,24 and therefore only will be briefly revisited here. Cilostazol inhibits phosphodiesterase III preventing tenascin-C expression by vascular smooth muscle cells during neointimal hyperplasia.Citation25,26 AT-1 antagonists and ACE inhibitors could also be useful since they inhibit angiotensin II, which is a potent inducer of tenascin-C. These drugs were shown to block vascular tenascin-C expression in hypertensive patients.Citation27 Whether these drugs are also useful in cancer treatment had not yet been assessed.
Clearance of tenascin-C from the tumor matrix
Finally, as a component of the ECM network in the tumor stroma, tenascin-C is probably difficult to remove. During tumor onset and progression heavy tissue remodeling is observed which includes secretion of ECM molecules and ECM remodeling enzymes. We have poor knowledge about tissue and matrix normalization during healthy tissue homeostasis and even less in pathologies such as cancer. High ECM remodeling is also seen during wound healing where upon scar formation the provisional matrix that has some similarities with the tumor matrix including high tenascin-C expression is resorbed.Citation28 If we understood more about the molecular mechanism of ECM resorption during normal wound healing could that allow us to establish means to be applied in cancer to help to “normalize” the tumor matrix? Unfortunately, this information is not available.
Using Tenascin-C Expression as Address for Delivery of Drugs and Cancer Diagnostics
Development of antibodies targeting tenascin-C for clinical application
Antibodies can be linked to additional bioactive molecules such as cytokines or radionuclides for a targeted delivery of cytotoxic activities into the tumor. The localized administration to the tumor site may require lower dosage of the cytotoxic agents and thus may reduce side effects. Several antibodies have been developed to target the tumor specific expression of tenascin-C with the aim to deliver cytotoxic molecules into the tenascin-C rich tumor tissue. Those antibodies mainly recognize and bind the alternatively spliced domains A1 to D of the large isoform of tenascin-C that are found to be preferentially expressed in cancers.Citation2 Most of these antibodies have been examined in preclinical murine models of cancer but a few were evaluated in early phase clinical trials in humans (). A summary of the advances on antibodies BC-2, BC-4 and 81C6 and their use in cancer radiotherapy can be found elsewhere.Citation2,29 Here, we will focus on the antibody F16 since it had reached the most advanced application in clinical trials for cancer therapy and new results have been reported recently (see also Catania et al., this issue of Cell Adhesion & Migration).
The antibody F16 and its use in cytokine/chemokine combination therapy
A fully humanized antibody F16 that specifically recognizes the domain A1 of tenascin-C (generated by alternative splicing) has been developed by the company Philogen. When this antibody, in the small immunoprotein (SIP) format (only comprising the variable regions of the antibody to avoid complications with elimination), was injected into a mouse with a tumor derived from xenografted human glioblastoma cells, it was noted that the antibody accumulated selectively in the tenascin-C rich tumor but not in other parts of the body suggesting a good in vivo specificityCitation30 encouraging to exploit the possibility whether this antibody detects tenascin-C expression in human cancer tissue. Indeed, the F16-SIP recognized tenascin-C in several human cancer types such as in glioblastoma multiformeCitation31 (GBM), lymphoma,Citation32 renal cell carcinoma,Citation33 lung cancer,Citation34 head and neck cancerCitation35 and melanomaCitation36 which altogether suggested a broad application potential of this antibody. Subsequently the antibody was coupled to interleukin-2 (IL2; now available as ProleukinTM) with the aim to direct immune cells into the tumor for facilitating tumor destruction by the immune system. Upon combination with cytotoxic drugs such as paclitaxel or doxorubicin, F16-IL2 was proven to be superior in reducing the volume of tumors originating from xenografted human breast cancer cells over treatment with the chemotherapeutic agents alone.Citation37 Similar results were also obtained in tumors from subcutaneously grafted human GBM cells when applied in combination with the standard drug Temozolomide. This combination caused complete tumor remission. Also in an orthotopic setting GBM xenograft tumors were 70% smaller and the survival rate of the animals was prolonged from 3 to 6 months.Citation31 These results demonstrated in vivo recognition of tenascin-C by F16 in tumor tissue. Results from studies in cynomolgus monkeys suggested the absence of toxicity.Citation37 Together with a study in a patient with acute myeloid leukemia (AML) encouraged clinical trials and the results from the first completed phase I/II study are published in this issue of Cell Adhesion & Migration by Catania and collaborators.
In an AML patient with advanced disease having relapsed tumors at multiple sites a combination treatment of F16-IL2 together with cytarabine and local radiotherapy (to ameliorate cancer associated problems with swallowing and vessel compression) an immediate symptomatic improvement was seen shortly after therapy even before the first application of radiotherapy.Citation38 Despite the documented dramatic reduction of tumor volume after 14 days the tumors relapsed later (personal information, D. Neri) which may not be surprising taken into account the severity of the disease before treatment. Nevertheless, these results encouraged randomized phase I/II clinical trials.
As mentioned above, the first phase I/II human clinical study of F16-IL2 treatment in combination with doxorubicin on a larger patient cohort is reported in this special issue by Catania et al. The study was performed to determine safety, tolerability and dose escalation in 19 patients with different solid tumors. A recommended dose (25 MIU F16-IL2) in combination with doxorubicin (25 mg/mCitation2) was established that had been further applied in 10 patients with metastatic breast cancer. No serious adverse effects and no death incidence related to the combination therapy were observed. Toxicities induced by the drug were controllable and reversible. Anti-cancer activities were seen in 14/19 (Phase Ib) and in 9/10 breast cancer patients (Phase II).
A clinical study with a similar design is currently ongoing where F16-IL2 is applied together with paclitaxel in lung cancer. So far this study had demonstrated a save application with no adverse effects. In a few cases disease stabilization was even observed.Citation39 In 2013 another clinical trial was initiated, this time to evaluate a potential application of F16-IL2 together with paclitaxel in metastatic Merkel cell carcinoma ().
The antibody F16 is a fully humanized antibody suggesting full immuno compatibility when injected into human patients. However Teleukin (F16-IL2) represents a fusion protein of F16 with human IL2 connected via a short peptide linker. This artificial linker potentially could constitute a foreign recognition site for the immune system that was investigated. Whereas F16-IL2 was weakly immunogenic in monkeys,Citation37 no immunogenic response could be detected in any of the patients included in the clinical study presented by Catania et al. in this issue. Thus, F16 and its derivate F16-IL2 constitute highly specific monoclonal antibodies toward domain A1 of tenascin-C with a very good safety profile in human patients.
Potential use of F16 for radionuclide therapy
The antibody F16 has also been tested for a potential use in targeted radionuclide therapy (). F16 was labeled with iodine 124 (F16-124I) and was injected into 4 patients with head and neck cancer and antibody uptake was followed by immuno-PET imaging. Beside an antibody uptake in several other organs, a tumor-specific signal was visible in all patients 24 hours after injection.Citation40 Another phase I/II clinical trial is currently ongoing using F16 coupled to iodine 131 (F16-131I, Tenarad®) for radiotherapy in several cancer types.Citation41 A first result on dosage and efficacy was reported recently.Citation42 In this study, Tenarad was administered to 8 Hodgkin's lymphoma patients that were refractory to conventional treatment. The enrolled patients had excessively been treated with conventional therapies prior to Tenarad that they had received by intravenous injection (2.05 GBq/m2, 5–10 mg). It was observed that Tenarad did not cause any toxicity. Five patients received an additional treatment between 3–12 month after the initial dose in particular upon notice of disease stabilization (reduced number and/or size of lesions) upon the first administration.Citation42
Altogether, the current antibody based cancer therapeutics targeting tenascin-C have been tested in phase I/II clinical trials for safety, dose and toxicity issues using small patient cohorts. These studies show that these antibodies can be safely administered. Some reports even indicate that the specific strategy might be beneficial in severe cases of cancer that are refractory to conventional therapies to slow down disease progression in particular in combination with cytotoxic drugs. Studies on larger patient cohorts need to be performed to refine drug dosage, administration frequency and schedule.
Aptamer based targeting of tenascin-C
Aptamers are short oligonucleotides that, similar to monoclonal antibodies, specifically bind to a target molecule with very high affinity and selectivity.Citation43 Aptamers are stable, can be designed with desired length and specific binding affinities, and can be linked to other molecules such as cytokines. Using the SELEX technology on tenascin-C expressing U251 glioblastoma cells or on purified tenascin-C the aptamer “TTA1” was generated that showed high specificity and selectivity for human tenascin-C.Citation44 Further modifications improved biodistribution and led to the design of radionuclide labeled 99mTc-TTA1. Upon intravenous injection into tumor bearing nude mice 99mTc-TTA1 specifically had accumulated in the GBM xenograft tumor,Citation45 as well as in tumors induced by human colon, breast and rhabdomyosarcoma cells.Citation46 Thus, 99mTc-TTA1 appears to be useful for targeting several human cancer types. In the same group another aptamer was generated (GBI-10) and was used in vitro experiments to affinity purify target proteins followed by mass spectroscopy analysis. It was shown that GBI-10 can bind to several tenascin-C peptides and that these interactions are located along the whole tenascin-C protein, however its activity in in vivo models was not reported yet.Citation47
This approach was further extended to engineering a multimodal nanoparticle-based Simultaneously Multiple Aptamers and RGD Targeting (SMART) probe that targets nucleolin, integrin αvβ3 and tenascin-C at the same time. The SMART probe showed a better specificity and enhanced binding intensity to a number of different human cancer cells in vitro (C6 glioma, DU145 prostate, HeLa cervical, NPA thyroid papillary, A549 non-small lung cancer) as compared to the single target probes TTA1, RGD or AS1411 as control.Citation48 Again, a proof of concept using in vivo tumorigenesis models are not reported yet.
The advantages of aptamers over monoclonal antibodies are evident; they are small thus exhibit superior biodistribution, and they can be produced by chemical synthesis in high amounts at good reproducibility and at a lower budget. The characteristic of GBI-10 to target several binding sites of tenascin-C can be of interest to address multiple splice variants at the same time, whereas monoclonal antibodies only bind to one specific sequence. Finally, aptamers can be engineered to achieve specific pharmacological properties, to resist degradation or to avoid quick renal elimination.Citation49
Antibodies targeting tenascin-C for diagnostic imaging
The use of monoclonal antibodies will also be useful for noninvasive in situ tumor detection by SPECT imaging. Until today detection of a tumor by MRI, PET or ultrasound imaging is limited by its minimal size of 1 cm in diameter.Citation50 Molecular imaging is becoming increasingly important in cancer diagnosis by using cancer specific target based imaging probes. Using probes coupled to fluorescent dyes or radioisotopes in vivo had been applied in preclinical models.Citation51,52 With regard to the use of monoclonal antibodies in diagnostic imaging and radiotherapy, it is important to obtain a good delivery of radiation into the tumor but at the same time a proper manipulation of blood half-life is needed to reduce the risk of deiodination, hence increased accumulation in the thyroid.Citation29 Such a technical advance was recently reported using radioisotopes Cu or Tc upon coupling to monoclonal antibodies against tenascin-C.Citation53,54 For that purpose, based on the previously described ligand “L,”Citation55 an optimized phosphonated bifunctional chelate (BFC) “L*” was developed as a coordination site to ensure a covalent and stable complex formation between Cu(II) and the antibody.Citation53 The BFC was designed to give a better thermodynamic stability and to prevent hydrolysis in vivo. The synthesized L* was tested for its ability to be coupled to the monoclonal antibodies B28-13, an antibody recognizing human tenascin-C8 or to MTn12, an antibody recognizing murine tenascin-C.Citation56 This work has shown that the ligand L* can be coupled to primary amines but also to large biomolecules, the antibodies B28-13Citation53 and MTn12.Citation54 Immunostaining on respective human or murine cancer tissue further confirmed the ability of coupled B28-13 or MTn12 to retain its affinity toward tenascin-C.Citation53,54 Although a careful evaluation in vivo is still lacking, the developed activated phosphonated BFC, especially when coupled to antibodies targeting tenascin-C may provide a new powerful tool to improve metal based imaging diagnostics and radiotherapeutic applications.
Anti-cancer immunity and cancer immunotherapy
Using the approach of antibody based anti-cancer activities, the additional promotion of anti-cancer immunity can be beneficial leading to long lasting remissions. This strategy has been applied by coupling the antibody F16 to the proinflammatory cytokine IL2 as described above. An alternative to monoclonal antibodies is the use of therapeutic vaccines to induce antibody mediated immunity against cancer specific self molecules. Current anti-cancer vaccination approaches or the injection of monoclonal antibodies targeting cancer specific signaling pathways are still costly procedures and tumors develop resistance toward those drugs. A novel and custom made vaccination approach targeting tumor specific antigens, including the C-domain of tenascin-C that is found in many cancers,Citation2 is currently under development and had been protected by a patent (). This strategy is based on targeting antigens that are preferentially expressed in the tumor vasculature but not in healthy tissues. The vaccine is composed of a recombinant protein (e.g., C-domain of tenascin-C) fused to bacterial thioredoxin. Applied together with a potent adjuvant cocktail, the immune system recognizes the modified self protein as non self leading to antibody production against the selected target protein. Such a therapeutic vaccine has been developed to target extradomain B of fibronectin (FN-EDB) or extradomain A of fibronectin (FN-EDA) and has shown anti-tumor activities in an in vivo fibrosarcoma tumor modelCitation57 and in the MMTV-PyMT mouse model of breast cancer.Citation58 Vaccination of mice and rabbits against the C-domain of tenascin-C induced high serum titers of specific antibodies to the targetCitation59 but its effect in preclinical cancer models is not yet reported. Compared to the use of monoclonal antibodies, the vaccination approach would overcome costly repetitive injections as the organism produces an immune response and the tumor antigen specific antibodies by itself.
Immatics Biotechnologies is currently developing another peptide vaccine, the multi-peptide vaccine “IMA950,” which includes tenascin-C as a target. This approach is tailored for patients with glioblastoma (GBM). Using a proteomic approach, a human leukocyte antigen (HLA) class I allele A*02-associated GBM peptidome was identified based on 32 GBM stage IV tissue samples.Citation60 This analysis resulted to a set of 309 tumor-associated peptides (TUMAPs) that could be assigned to 148 different genes. Further in-depth analysis and validation studies let to a selection of 10 peptides that are highly expressed in GBM and at the same time showed the ability to elicit strong antigen-specific T cell responses in vitro. One of the 10 peptides corresponds to positions 3-11 of tenascin-C. Using patient-derived T cell clones that are specific for 2 of the 10 peptides showed cytotoxic activity against GBM cells in vitro.Citation60 The vaccine IMA950 comprises 9 TUMAPs eliciting cytotoxic T lymphocytes and 2 TUMAPs that target T-helper cells. Using IMA950, 3 phase 1 clinical studies have been initiated () and final results have been presented recently at the ESMO 2014 congress for the trial conducted by Cancer Research UK.Citation61 The purpose of that phase 1 study was to determine the safety and immunogenicity of IMA950 in glioblastoma patients. 45 patients received intradermal application of IMA950 together with GM-SCF (granulocyte-macrophage colony-stimulating factor) as an adjuvant. All patients received standard therapy (surgery and chemoradiotherapy) and one cohort additional treatment with temozolomide. 40 of the 45 patients were evaluable and have the treatment well tolerated. 90% of them showed an immune response to one TUMAP and 50% to several TUMAPs, exceeding the pre-defined goal of 60%.Citation62 In conclusion, this study shows that the development of a peptide vaccine targeting multiple TUMAPs, including tenascin-C, is safely applicable in GBM and elicits specific immune response in most of the patients. A beneficial effect on tumor development and patient survival needs to be investigated in additional phase 2 and 3 clinical studies.
In summary, the described approaches of antibody mediated and aptamer based strategies to target tenascin-C in cancer might open new powerful treatment opportunities in the future. These novel approaches in particular aptamers or therapeutic vaccination might be superior over conventional chemotherapy since their production is easier and cheaper, and targeting of multiple tumor antigens at once is possible. Unfortunately, advanced clinical trials are still missing. Currently, tumor specific drug delivery using monoclonal antibodies against tenascin-C represents the most advanced approach. Although some of the observations made in patients look promising more clinical trials need to be done, optimized and expanded to phase III for a proper evaluation as a standard cancer therapy. Moreover, an increased knowledge about the tumor-, patient- and stage specific expression of tenascin-C and its splice variants is required to develop more specific and tailored therapies for targeting tenascin-C. In conclusion, the here presented results underpin the importance and feasibility of a targeting strategy using tenascin-C expression as anti-cancer address.
Tenascin-C in Radiotherapy: Friend or Foe?
Radiotherapy or treatment by ionizing radiation comprises 50–70% of cancer treatments, making it the most frequently chosen therapy. It is conceivable that tumor ECM is affected by radiotherapy since radiotherapy can induce inflammation and fibrosis, 2 conditions where ECM is largely remodeled.Citation63 Except induction of collagens type I and III,Citation64 fibronectin, hyaluronic acid, matrix degrading enzymesCitation65 and Cyr61Citation66 by radiotherapy and, a possible radiotherapy protective role of cell adhesion to fibronectinCitation67 little is unknown how radiotherapy affects the ECM composition of a tumor and what impact that would have on tumor relapse. Given its high expression and reports demonstrating induction of tenascin-C by radiotherapy, here we will address whether radiotherapy has an impact on tenascin-C expression and what consequences this could have for patient outcome.
The major effect of radiation therapy is the induction of tumor cell death triggered by DNA damage (DNA double strand breaks) (). In addition effects on the tumor vasculature have also been reported to contribute to tumor shrinkage on one hand but may also contribute to tumor relapse/second tumor formation on the other hand.Citation68 Radiation is delivered by 3 approaches, metabolic radiation, external irradiation and brachytherapy (where the radiation source is placed inside or next to the area requiring treatment). External irradiation is the most frequently used technique to treat solid tumors (usually comprising a total dose of 20–70 Gy delivered by a daily fraction of 1.8–2 Gy).
Figure 1. Consequences of irradiation on the tumor microenvironment and tenascin-C radiotherapy is the most frequent regimen used to treat solid cancers by inducing the production of reactive oxygen (ROS) and nitrogen (NOS) species causing DNA damage and cell death. Depending on the location and the tumor type radiotherapy is efficient in diminishing tumor volume and reducing tumor growth thus prolonging patient survival. However, radiotherapy may also trigger tumor relapse and second cancers that often are more aggressive and highly metastatic. Understanding the impact of radiotherapy on the different cell types within the tumor ecosystem may help to improve radiotherapy efficiency by designing new concepts for combinations with tailored chemotherapy. Ionizing irradiation induces conversion of fibroblasts into carcinoma associated fibroblasts (CAF) that express TGFβ among other soluble factors and several ECM molecules among them tenascin-C (TNC) which may be involved in triggering irradiation associated fibrosis. Radiotherapy also causes endothelial cell death and thus hypoxia which in turn triggers new vessel formation through angiogenesis, vasculogenesis and potentially intussuception thus allowing tissue reoxygenation. Hypoxia triggers VEGFA and tenascin-C which together may be instrumental in new vessel formation. Radiotherapy has also abscopal effects on immune cells causing inflammation and cell immunity. Tenascin-C expression might be triggered by radiation induced inflammation as had been seen in other inflammatory contexts. Altogether side effects of radiotherapy on the tumor microenvironment might promote tumor relapse and 2nd tumor formation where tenascin-C could be a molecule with high targeting potential.
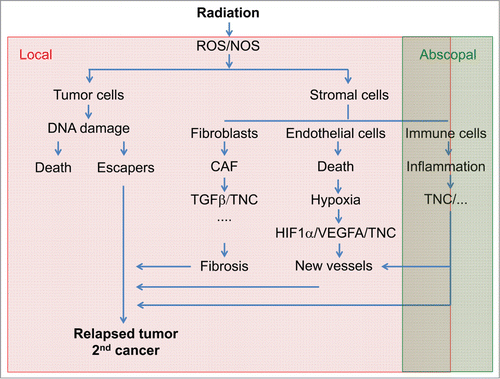
To reduce side effects, addressed by the normal tissue complication probability index (NTCP),Citation69 a lot of effort has been invested to improve local delivery of radiation to the tumor tissue with the intention to largely spare normal tissue. An important advance in radiotherapy has been the development of noninvasive live imaging which is based on combining PET (positron emission tomography) scan with a variety of radiopharmaceutical tracers and MRI (magnetic resonance imaging). This technology had improved the delineation of tumor circumferences for tumor removal by conventional resection or radiation surgery thus reducing side effects on the surrounding healthy tissue.Citation70
Stereotactic radiotherapy (SRT) is a rather new technology that combines stereotaxis, high precision of localization of the target in 3 dimensions, with delivery of high dose of ionizing radiation in 1-5 fractions during radiosurgery. SRT combined with MRI or PET scan delineation (depending on tumor location) improved the control of local tumor growth, increased overall survival of the patient and reduced unwanted irradiation side effects on the very radiosensitive hippocampus (e.g., inflicting memory loss). SRT is now widely used in other cancers such as liver and lung tumors and metastasis. In particular in the elderly with inoperable localized lung tumors SRT has proven to not only be successful in palliative but also in curative treatment.Citation71 The latest technological advance comprises hadrontherapy that is superior over IMRT. This technology is based on the use of hadron particles (protons, carbons) which allows to further enhance the local doseCitation72 and has been applied in more than 30 000 patients in more than 15 centers in the US. The most frequent application of this method are skull base tumors, pediatric cancer, ocular melanoma and prostate cancer. We speculate that combining these novel radiotherapy approaches together with tenascin-C targeting could be useful for treatment of inoperable tumors which had not yet been addressed in the clinics.
Despite these promising advances of IMRT and hadrontherapy that have largely improved and will continue to further improve treatment of cancer patients, substantial knowledge is missing about the underlying biology that impacts on normal tissue complication probabilities (NTCP) and long term effects as e.g., tumor relapse, secondary tumor development and metastasis.Citation73,74 To assess irradiation effects the “5-R” index (radiosensitivity, repopulation, redistribution, reoxygenation and repair) is applied. Although around 40% of cancer patients treated with radiotherapy seem to be curedCitation75 the majority is not. Late toxicity is an important effect that is seen after treatment and can pose a problem for many years post radiotherapy. In addition, the risk of a second cancer either representing tumor relapse or the appearance of a new tumor is another problem and the risks increase over the decades post radiotherapy. This problem could be more relevant in younger patients with a good prognosis.Citation76 Therefore it is obvious that additional criteria are needed.Citation77-79 There are several open questions that address the impact of irradiation on the tumor vasculature since radiotherapy causes inflammation and hypoxia which are implicated in tumor regrowth and metastasis. As mentioned above, also very little is known how radiotherapy affects the tumor bed and in particular the ECM, that can promote tumor angiogenesis and metastasis.Citation80-82 Given that ECM is rather stable and will persist after the end of radiotherapy it is important to understand what radiotherapy is doing to the ECM. Questions are arising whether there is a limited number of ECM molecules that are induced by radiotherapy and what their identity and role during and after radiotherapy is. We also would need to know how low/high radiation doses delivered once or by fractionation impact on the tumor microenvironment and on the adjacent normal tissue. These new schedules, mainly using ablative doses and/or proton beams combined with chemotherapy, in particular inflammatory drugs present future strategies that need to be explored more deeply.
In the following paragraphs we will address what knowledge had been gathered about the underlying biology of radiotherapy in experimental murine cancer models with a special focus on tenascin-C since tenascin-C is one (but not the only) ECM molecule that is induced by radiotherapy.Citation83 Given that tenascin-C can promote tumor angiogenesis and lung metastasis,Citation2,24 here we will critically review the literature to evaluate the question whether tenascin-C potentially plays a role in radiotherapy resistance and tumor relapse and progression associated with radiotherapy.
Impact of radiotherapy on the tumor microenvironment and on the expression of tenascin-C
Radiotherapy and the tumor bed effect (TBE)
For a long time it has been known that ionizing radiation induces changes in the tumor microenvironment thus potentially promoting tumor progression and relapse.Citation80 The influence of ionizing radiation on the tumor microenvironment had been experimentally addressed by grafting of tumor cells into pre-irradiated tissues. It was noticed that tumors grew slower when implanted into the pre-irradiated tissue but at the same time often had been more invasive and metastatic.Citation84 This effect was coined the “Tumor Bed Effect,” TBE. TBE is radiation dose dependent (5–20 Gy), occurs upon a single dose as well as upon fractionated doses and is a long-term effect since it was still seen 90 days after irradiation (reviewed inCitation81). Several reviews had summarized the molecular mechanisms underlying TBE upon irradiation and will not be further elaborated here.Citation80,81
The long lasting effect suggests that the ECM could be affected by radiotherapy and that alterations in the ECM may contribute to TBE. Indeed this has been demonstrated in squamous cell carcinoma and colon cancer cell xenografting models where the authors have described an important role of the matricellular protein Cyr61/CCN1 and its receptor αvβ5 on promoting lung metastasis upon pre-irradiation of the tumor bed. This effect was abrogated with Cilengitide, a peptide antagonist of the αv integrin subunit.Citation85 Yet it is not clear which cells had expressed Cyr61 and how the local Cyr61 rich microenvironment impacts on distant metastasis formation. Whether an increased risk of developing metastasis upon local recurrences after radiotherapy holds also true in human cancer patients is supported by some but not all studies (reviewed inCitation81), nor is it known whether a similar mechanism involving Cyr61 as seen in the murine model also applies to the human disease.
Induction of tenascin-C upon radiotherapy
A few studies have addressed the impact of radiotherapy on the expression of tenascin-C. Tenascin-C was seen to be induced by ionizing radiation (20 to 60 Gy) in skin cancer tissue of human patientsCitation83 and in pigsCitation86. Radiation with a similar dose also induced tenascin-C in the submandibular glands of experimental rats.Citation87 In particular in xerostomia (dry mouth) which is a complication of HNSCC (head and neck squamous cell carcinoma) radiotherapy (mean dose of 42 Gy in all patients) was noticed to induce tenascin-C.88 Irradiation of the orbital region of rabbits with a single 15 Gy dose also led to an increase of tenascin-C 72 hours post irradiation which was associated with dry eyes as side effect. However, a pre-treatment with lidocaine and amifostine to counteract the dry eye effect had also an impact on tenascin-C expression that was lowered.Citation89 Analysis of the impact of radiotherapy on tenascin-C expression is hampered by the fact that relapsed tumors arise after very heterogeneous treatment protocols and material for analysis is difficult to obtain since relapsed tumors are not always resected. Thus the impact of radiotherapy on tenascin-C induction has to be mainly addressed in murine models.
Radiotherapy induces inflammation, fibrosis and hypoxiaCitation90,91 which altogether represent conditions in multiple pathologies where tenascin-C is highly expressed and has proven to play a critical role in disease severity.Citation18,24 Irradiation also has an impact on the immune response and can trigger “cellular immunology.”Citation82 In particular, a high-dose and few-fraction schedule had been reported to trigger inflammation associated apoptosis that was characterized by the recruitment of dendritic cells to the irradiated site. Tumor specific immunity seems to be enhanced by radiotherapy but apparently only when large-fraction doses were applied.Citation92 Altogether these observations raise the question whether we can gain insight into potential consequences of abundantly expressed tenascin-C in irradiated tissue by understanding the role of tenascin-C in these other pathological contexts. Some of these aspects had been addressed in this issue by Giblin and Midwood and by Udalova et al.Citation93
Tenascin-C and radiation induced inflammation
Radiotherapy induces TGFβ and thus could play an important role in irradiation associated inflammation.Citation94 In early stages TGFβ may play a role in suppression of malignancy but at later stages may promote tumor progression.Citation95,96 Since TGFβ induces tenascin-C expression,Citation97 it is intriguing to speculate that this pathway may contribute to tenascin-C induction by radiotherapy. In addition, irradiation induced hypoxia or inflammation might also trigger tenascin-C expression by other pathways.Citation2 Moreover, it is possible that tenascin-C promotes TGFβ signaling which could have important consequences. In support of this possibility it was shown that tenascin-C enhances TGFβ induced EMT (epithelial to mesenchymal transition) like morphological changes in an integrin αvβ1 and α6β1 dependent manner in breast cancer cells.Citation98 Despite binding of TGFβ to tenascin-C99 it is largely obscure how this would be accomplished at the molecular level. Recently, it was shown that the fibrinogen domain of the family member tenascin-X binds to a LTBP-TGFβ complex thus enhancing TGFβ signaling (see also review by Valcourt et al. in this issue). Given substantial sequence homology between the fibrinogen domain of tenascin-C with that of tenascin-X, it is intriguing to speculate that a similar mechanism might also apply to tenascin-C. Enhanced TGFβ signaling could have multiple effects such as interference with irradiation induced immunity, triggering and enhancing new blood vessel formation and mobilizing resistant tumor cells. These possibilities are circumstantial and thus highly speculative but intriguing and need to be addressed by future research.
Tenascin-C and radiation induced endothelial apoptosis, hypoxia and revascularization
Endothelial cell apoptosis occurs within the first hour, peaks at 4 hours and ends at 6 hours after a single fraction of 5 Gy (Citation100, reviewed inCitation82). This process is started within seconds after irradiation not yet involving a DNA damage response but the production of ceramide through ASMase (sphingomyelinase), which is a proapoptotic messenger (reviewed inCitation82). Ionizing irradiation also appears to interfere with vascular endothelial growth factor (VEGFA) and fibroblast growth factor-2 (FGF-2) - induced angiogenesis in vivo, and suppresses endothelial cell proliferation, migration and sprouting in vitro. This would lead to hypoxia that triggers mechanisms to restore oxygenation via formation of new blood vessels which could include angiogenesis, vasculogenesis and intussusception (for review seeCitation101). It is possible that in post irradiated tissue TGFβ signaling and hypoxia driven induction of VEGFA and tenascin-C altogether play a role in new blood vessel formation. This is supported by a study showing that irradiation activated the TGFβ type I receptor/activin receptor-like kinase-5 (ALK5) angiogenesis promoting pathway. Pharmacological ALK5 inhibition restored radiation induced inhibition of endothelial cell migration and sprouting in vitro, and impairment of angiogenesis in vivo.Citation102 Since tenascin-C enhances TGFβ signalingCitation98, binds TGFβCitation99 and is upregulated during the angiogenic switchCitation13 it will be interesting to see whether tenascin-C potentiates the TGFβ effect on new blood vessel formation post irradiation.
Hypoxia, resulting from irradiation, is a major condition promoting angiogenesis to facilitate reoxygenation where VEGFA, induced by HIF1α, are important pro-angiogenic factors driving new vessel formation.Citation103 In addition, VEGFA was also shown to directly act on tumor cells by promoting survival, proliferation, migration and invasion.Citation104 In a potential scenario radiotherapy induces hypoxia which in turn triggers tenascin-C and VEGFA expression. Each molecule/pathway alone as well as in conjunction may promote new blood vessel formation and tumor cell survival, thus promoting tumor regrowth, progression and metastasis post irradiation (). This hypothesis is supported by data showing that VEGFA is instrumental in tenascin-C enhanced lung metastasis of 4T1 cells.Citation105 Yet a potential link to radiotherapy still has to be investigated.
Potential Role of Tenascin-C in Tumor Relapse and Resistance upon Drug Treatment
Tenascin-C is part of complex poorly understood ECM networks not only seen in cancer but also in other disease contexts such as inflammation and fibrosis. These matrices presumably provide cells with survival cues. It is possible that such niches protect tumor cells also from therapeutic drugs. This possibility is supported by a study where the authors have seen that a high tenascin-C expression in estrogen positive breast cancer correlated with Tamoxifen resistance in first line therapy.Citation106 Upon destruction of blood vessels with 2 anti-angiogenic drugs in a well-established insulinoma model and in a murine xenograft tumor model with Lewis lung carcinoma cells it was observed that revascularization occurred along so called matrix sleeves positive for collagen IV, interpreted as remnants of vascular basement membranes.Citation107 Whether these matrix sleeves contained tenascin-C had not been addressed but is likely since tenascin-C is found to be highly expressed around newly formed tumor blood vessels (reviewed inCitation19,Citation2). Given its instrumental role in promoting tumor angiogenesisCitation4,13 it is an intriguing possibility that tenascin-C may also promote revascularization upon anti angiogenic treatment.
Tenascin-C might have an impact on drug responsiveness and DNA repair due to its interaction with fibronectin. Both ECM molecules bind to each other and are frequently coexpressed in cancer tissue in particular around newly formed blood vessels.Citation19 Tenascin-C was shown to counteract cell adhesion to fibronectin through competition with syndecan-4 binding to fibronectin.Citation108 Thus both molecules might play a role as accomplices in tumor progression.Citation19 Recently it was shown that adhesion to fibronectin promotes DNA damage recognition and chemosensitization to cisplatin via the potentiation of the DNA damage signaling response (DDR) in human colon cancer cells and tumor derived myofibroblasts.Citation109 Cisplatin induced phosphorylation of γH2AX, a molecule that is implicated in safeguarding the mitotic process even in the absence of DNA damage.Citation110 This molecule serves as DNA damage sensor and is required for recruitment of DNA repair factors. Adhesion to fibronectin enhanced more phosphorylation of (γH2AX in response to cisplatin than in cells on BSA or another adhesive collagen IV containing substratum. This occurred in an integrin α5β1 dependent manner involving PI3K, ATM and JNK2. Altogether these results suggest that cells that do not die upon cisplatin treatment are empowered to repair their damaged DNA when grown attached on fibronectin.Citation109 Tenascin-C might particularly interfere with this activity by blocking cell adhesion to fibronectin. This possibility is indeed supported by the observation that adhesion of T98G GBM cells onto a fibronectin/tenascin-C substratum caused downregulation of γH2AX among several other molecules involved in DNA repair (e.g., several MCMs) in comparison to a fibronectin substratum.Citation111 In consequence cells with damaged DNA that escape cell death upon cisplatin treatment might be more prone to accumulate mutations when grown in a tenascin-C rich microenvironment.
Downregulation of DKK1 by tenascin-C might be relevant in cisplatin sensitivity. It had been demonstrated that DKK1 levels are increased by cisplatin in HNSCCCitation112 and GBMCitation113 presumably due to its p53 binding element in its promoter. DKK1 induction was not seen in cisplatin resistant CAL27 cells and resistance could be overcome by exogenously added DKK1.Citation112 Many cancers express high DKK1 levelsCitation114 which may render them susceptible to destruction by cisplatin. However tenascin-C downregulates DKK1 which may lead to niches with low DKK1 levels. Thus it is possible that cells in a tenascin-C rich microenvironment are less sensitive to cisplatin due to low DKK1 levels thus promoting selection of cisplatin resistant cells. This is an intriguing possibility that warrants further analysis.
Summary
Over the past 30 years we have gained significant knowledge about the structure and expression of tenascin-C as well as cell responses toward tenascin-C. This information has led to the development of novel concepts of cancer intervention therapies and cancer imaging and diagnosis opportunities. Although radiotherapy and cytotoxic drugs are the most important regimens in cancer treatment our knowledge about their impact on the tumor microenvironment and in particular on tenascin-C expression and function is rather limited. Upon filling these gaps tenascin-C and its associated signaling may turn out to be useful for the development of combination strategies to be applied together with radiotherapy as well as with cytotoxic drugs.
Disclosure of Potential Conflicts of Interest
No potential conflicts of interest were disclosed.
References
- Pàez-Ribes M, Allen E, Hudock J, Takeda T, Okuyama H, Viñals F, Inoue M, Bergers G, Hanahan D, Casanovas O. Antiangiogenic therapy elicits malignant progression of tumors to increased local invasion and distant metastasis. Cancer Cell 2009; 15:220-31; PMID:19249680; http://dx.doi.org/10.1016/j.ccr.2009.01.027
- Orend G, Saupe F, Schwenzer A, Midwood K. The Extracellular Matrix and Cancer: Regulation of Tumor Cell Biology by Tenascin-C. iConcept Press; 2014. ISBN: 978-1922227515
- Orend G, Chiquet-Ehrismann R. Tenascin-C induced signaling in cancer. Cancer Lett 2006; 244:143-63; PMID:16632194; http://dx.doi.org/10.1016/j.canlet.2006.02.017
- Saupe F, Schwenzer A, Jia Y, Gasser I, Spenlé C, Langlois B, Kammerer M, Lefebvre O, Hlushchuk R, Rupp T, et al. Tenascin-C downregulates wnt inhibitor dickkopf-1, promoting tumorigenesis in a neuroendocrine tumor model. Cell Rep 2013; 5:482-92; PMID:24139798; http://dx.doi.org/10.1016/j.celrep.2013.09.014
- Lieber CS, Weiss DG, Paronetto F, Veterans Affairs Cooperative Study 391 Group. Value of fibrosis markers for staging liver fibrosis in patients with precirrhotic alcoholic liver disease. Alcohol Clin Exp Res 2008; 32:1031-9; PMID:18422837; http://dx.doi.org/10.1111/j.1530-0277.2008.00664.x
- Päiväniemi OE, Maasilta PK, Alho HS, Vainikka TLS, Salminen U-S. Epithelial tenascin predicts obliterative airway disease. J Heart Lung Transplant Off Publ Int Soc Heart Transplant 2008; 27:400-7; PMID:18374876; http://dx.doi.org/10.1016/j.healun.2008.01.009
- Tsukada B, Terasaki F, Shimomura H, Otsuka K, Otsuka K, Katashima T, Fujita S, Imanaka-Yoshida K, Yoshida T, Hiroe M, et al. High prevalence of chronic myocarditis in dilated cardiomyopathy referred for left ventriculoplasty: expression of tenascin C as a possible marker for inflammation. Hum Pathol 2009; 40:1015-22; PMID:19297005; http://dx.doi.org/10.1016/j.humpath.2008.12.017
- Schenk S, Muser J, Vollmer G, Chiquet-Ehrismann R. Tenascin-C in serum: a questionable tumor marker. Int J Cancer 1995; 61:443-9; PMID:7538974; http://dx.doi.org/10.1002/ijc.2910610402
- Fouda GG, Jaeger FH, Amos JD, Ho C, Kunz EL, Anasti K, Stamper LW, Liebl BE, Barbas KH, Ohashi T, et al. Tenascin-C is an innate broad-spectrum, HIV-1-neutralizing protein in breast milk. Proc Natl Acad Sci U S A 2013; 110:18220-5; PMID:24145401; http://dx.doi.org/10.1073/pnas.1307336110
- Riedl S, Tandara A, Reinshagen M, Hinz U, Faissner A, Bodenmüller H, Buhr HJ, Herfarth C, Möller P. Serum tenascin-C is an indicator of inflammatory bowel disease activity. Int J Colorectal Dis 2001; 16:285-91; PMID:11686525; http://dx.doi.org/10.1007/s003840100312
- Didem T, Faruk T, Senem K, Derya D, Murat S, Murat G, Oznur K. Clinical significance of serum tenascin-c levels in epithelial ovarian cancer. Tumour Biol J Int Soc Oncodevelopmental Biol Med 2014; 35:6777-82; PMID:24722824; http://dx.doi.org/10.1007/s13277-014-1923-z
- Tastekin D, Tas F, Karabulut S, Duranyildiz D, Serilmez M, Guveli M, Vatansever S. Clinical significance of serum tenascin-C levels in breast cancer. Tumour Biol J Int Soc Oncodevelopmental Biol Med 2014; 35:6619-25; PMID:24696262; http://dx.doi.org/10.1007/s13277-014-1875-3
- Langlois B, Saupe F, Rupp T, Arnold C, van der Heyden M, Orend G, Hussenet T. AngioMatrix, a signature of the tumor angiogenic switch-specific matrisome, correlates with poor prognosis for glioma and colorectal cancer patients. Oncotarget 2014; 5: 10529-45; PMID:25301723
- Thomasset N, Lochter A, Sympson CJ, Lund LR, Williams DR, Behrendtsen O, Werb Z, Bissell MJ. Expression of autoactivated stromelysin-1 in mammary glands of transgenic mice leads to a reactive stroma during early development. Am J Pathol 1998; 153:457-67; PMID:9708806; http://dx.doi.org/10.1016/S0002-9440(10)65589-7
- Sternlicht MD, Bissell MJ, Werb Z. The matrix metalloproteinase stromelysin-1 acts as a natural mammary tumor promoter. Oncogene 2000; 19:1102-13; PMID:10713697; http://dx.doi.org/10.1038/sj.onc.1203347
- Taraseviciute A, Vincent BT, Schedin P, Jones PL. Quantitative analysis of three-dimensional human mammary epithelial tissue architecture reveals a role for tenascin-C in regulating c-met function. Am J Pathol 2010; 176:827-38; PMID:20042668; http://dx.doi.org/10.2353/ajpath.2010.090006
- Kääriäinen E, Nummela P, Soikkeli J, Yin M, Lukk M, Jahkola T, Virolainen S, Ora A, Ukkonen E, Saksela O, et al. Switch to an invasive growth phase in melanoma is associated with tenascin-C, fibronectin, and procollagen-I forming specific channel structures for invasion. J Pathol 2006; 210:181-91; PMID:16924594; http://dx.doi.org/10.1002/path.2045
- Midwood KS, Orend G. The role of tenascin-C in tissue injury and tumorigenesis. J Cell Commun Signal 2009; 3:287-310; PMID:19838819; http://dx.doi.org/10.1007/s12079-009-0075-1
- Van Obberghen-Schilling E, Tucker RP, Saupe F, Gasser I, Cseh B, Orend G. Fibronectin and tenascin-C: accomplices in vascular morphogenesis during development and tumor growth. Int J Dev Biol 2011; 55:511-25; PMID:21769776; http://dx.doi.org/10.1387/ijdb.103243eo
- Chiquet-Ehrismann R, Tucker RP. Tenascins and the importance of adhesion modulation. Cold Spring Harb Perspect Biol 2011; 3; a004960; PMID:21441591; http://dx.doi.org/10.1101/cshpers-pect.a004960
- Zukiel R, Nowak S, Wyszko E, Rolle K, Gawronska I, Barciszewska MZ, Barciszewski J. Suppression of human brain tumor with interference RNA specific for tenascin-C. Cancer Biol Ther 2006; 5:1002-7; PMID:16775434; http://dx.doi.org/10.4161/cbt.5.8.2886
- Wyszko E, Rolle K, Nowak S, Zukiel R, Nowak M, Piestrzeniewicz R, Gawrońska I, Barciszewska MZ, Barciszewski J. A multivariate analysis of patients with brain tumors treated with ATN-RNA. Acta Pol Pharm 2008; 65:677-84; PMID:19172848
- Rolle K, Nowak S, Wyszko E, Nowak M, Zukiel R, Piestrzeniewicz R, Gawronska I, Barciszewska MZ, Barciszewski J. Promising human brain tumors therapy with interference RNA intervention (iRNAi). Cancer Biol Ther 2010; 9:396-406; PMID:20118657; http://dx.doi.org/10.4161/cbt.9.5.10958
- Midwood KS, Hussenet T, Langlois B, Orend G. Advances in tenascin-C biology. Cell Mol Life Sci CMLS 2011; 68:3175-99; PMID:21818551; http://dx.doi.org/10.1007/s00018-011-0783-6
- Fujinaga K, Onoda K, Yamamoto K, Imanaka-Yoshida K, Takao M, Shimono T, Shimpo H, Yoshida T, Yada I. Locally applied cilostazol suppresses neointimal hyperplasia by inhibiting tenascin-C synthesis and smooth muscle cell proliferation in free artery grafts. J Thorac Cardiovasc Surg 2004; 128:357-63; PMID:15354092; http://dx.doi.org/10.1016/j.jtcvs.2003.11.015
- Yamamoto K, Onoda K, Sawada Y, Fujinaga K, Imanaka-Yoshida K, Yoshida T, Shimpo H. Locally applied cilostazol suppresses neointimal hyperplasia and medial thickening in a vein graft model. Ann Thorac Cardiovasc Surg Off J Assoc Thorac Cardiovasc Surg Asia 2007; 13:322-30; PMID:17954989
- Fischer JW. Tenascin-C: a key molecule in graft stenosis. Cardiovasc Res 2007; 74:335-6; PMID:17459357; http://dx.doi.org/10.1016/j.cardiores.2007.04.001
- Midwood KS, Williams LV, Schwarzbauer JE. Tissue repair and the dynamics of the extracellular matrix. Int J Biochem Cell Biol 2004; 36:1031-7; PMID:15094118; http://dx.doi.org/10.1016/j.biocel.2003.12.003
- Reilly RM. Monoclonal Antibody and Peptide-Targeted Radiotherapy of Cancer. Wiley VCH Book Chapter 5.4 2010; ISSN: 978-0-470-24372-5
- Brack SS, Silacci M, Birchler M, Neri D. Tumor-targeting properties of novel antibodies specific to the large isoform of tenascin-C. Clin Cancer Res 2006; 12:3200-8; PMID:16707621; http://dx.doi.org/10.1158/1078-0432.CCR-05-2804
- Pedretti M, Verpelli C, Marlind J, Bertani G, Sala C, Neri D, Bello L. Combination of temozolomide with immunocytokine F16-IL2 for the treatment of glioblastoma. Br J Cancer 2010; 103:827-36; PMID:20736949; http://dx.doi.org/10.1038/sj.bjc.6605832
- Schliemann C, Wiedmer A, Pedretti M, Szczepanowski M, Klapper W, Neri D. Three clinical-stage tumor targeting antibodies reveal differential expression of oncofetal fibronectin and tenascin-C isoforms in human lymphoma. Leuk Res 2009; 33:1718-22; PMID:19625084; http://dx.doi.org/10.1016/j.leukres.2009.06.025
- Berndt A, Kollner R, Richter P, Franz M, Voigt A, Berndt A, Borsi L, Giavazzi R, Neri D, Kosmehl H. A comparative analysis of oncofetal fibronectin and tenascin-C incorporation in tumour vessels using human recombinant SIP format antibodies. Histochem Cell Biol 2010; 133:467-75; PMID:20237793; http://dx.doi.org/10.1007/s00418-010-0685-y
- Pedretti M, Soltermann A, Arni S, Weder W, Neri D, Hillinger S. Comparative immunohistochemistry of L19 and F16 in non-small cell lung cancer and mesothelioma: two human antibodies investigated in clinical trials in patients with cancer. Lung Cancer 2009; 64:28-33; PMID:18799229; http://dx.doi.org/10.1016/j.lungcan.2008.07.013
- Schwager K, Villa A, Rosli C, Neri D, Rosli-Khabas M, Moser G. A comparative immunofluorescence analysis of three clinical-stage antibodies in head and neck cancer. Head Neck Oncol 2011; 3:25; PMID:21548989; http://dx.doi.org/10.1186/1758-3284-3-25
- Frey K, Fiechter M, Schwager K, Belloni B, Barysch MJ, Neri D, Dummer R. Different patterns of fibronectin and tenascin-C splice variants expression in primary and metastatic melanoma lesions. Exp Dermatol 2011; 20:685-8; PMID:21649738; http://dx.doi.org/10.1111/j.1600-0625.2011.01314.x
- Marlind J, Kaspar M, Trachsel E, Sommavilla R, Hindle S, Bacci C, Giovannoni L, Neri D. Antibody-mediated delivery of interleukin-2 to the stroma of breast cancer strongly enhances the potency of chemotherapy. Clin Cancer Res 2008; 14:6515-24; PMID:18927291; http://dx.doi.org/10.1158/1078-0432.CCR-07-5041
- Gutbrodt KL, Schliemann C, Giovannoni L, Frey K, Pabst T, Klapper W, Berdel WE, Neri D. Antibody-based delivery of interleukin-2 to neovasculature has potent activity against acute myeloid leukemia. Sci Transl Med 2013; 5:201ra118; PMID:24005158; http://dx.doi.org/10.1126/scitranslmed.3006221
- De Braud FG, Catania C, Onofri A, Pierantoni C, Cascinu S, Maur M, Masini C, Conte PF, Giovannoni L, Tasciotti A, et al. Combination of the immunocytokine F16-IL2 with doxorubicin or paclitaxel in patients with solid tumors: Results from two phase Ib trials. J Clin Oncol 29 ASCO Annu Meet 2011 2011; Abstract 2595.
- Heuveling DA, de Bree R, Vugts DJ, Huisman MC, Giovannoni L, Hoekstra OS, Leemans CR, Neri D, van Dongen GA. Phase 0 microdosing PET study using the human mini antibody F16SIP in head and neck cancer patients. J Nucl Med 2013; 54:397-401; PMID:23334725; http://dx.doi.org/10.2967/jnumed.112.111310
- Aloj L, D’Ambrosio L, Aurilio M, Marreno R, Neri D, Menssen HD, Giovannoni L, Di Gennaro F, Caraco C, Arcamone M, et al. Preliminary evaluation of radioimmunotherapy with Τenarad, a I-131 labeled antibody fragment targeting the extra-domain A1 of tenascin-C, in patients with refractory Ηodgkin lymphoma. J Clin Oncol 29 ASCO Annu Meet 2011 2011; Abstract 8063.
- Aloj L, D’Ambrosio L, Aurilio M, Morisco A, Frigeri F, Caraco C, Di Gennaro F, Capobianco G, Giovannoni L, Menssen HD, et al. Radioimmunotherapy with Tenarad, a 131I-labelled antibody fragment targeting the extra-domain A1 of tenascin-C, in patients with refractory Hodgkin's lymphoma. Eur J Nucl Med Mol Imaging 2014; 41:867-77; PMID:24435772; http://dx.doi.org/10.1007/s00259-013-2658-6
- Tuerk C, MacDougal S, Gold L. RNA pseudoknots that inhibit human immunodeficiency virus type 1 reverse transcriptase. Proc Natl Acad Sci U A 1992; 89:6988-92; PMID:1379730; http://dx.doi.org/10.1073/pnas.89.15.6988
- Hicke BJ, Marion C, Chang YF, Gould T, Lynott CK, Parma D, Schmidt PG, Warren S. Tenascin-C aptamers are generated using tumor cells and purified protein. J Biol Chem 2001; 276:48644-54; PMID:11590140; http://dx.doi.org/10.1074/jbc.M104651200
- Schmidt KS, Borkowski S, Kurreck J, Stephens AW, Bald R, Hecht M, Friebe M, Dinkelborg L, Erdmann VA. Application of locked nucleic acids to improve aptamer in vivo stability and targeting function. Nucleic Acids Res 2004; 32:5757-65; PMID:15509871; http://dx.doi.org/10.1093/nar/gkh862
- Hicke BJ, Stephens AW, Gould T, Chang YF, Lynott CK, Heil J, Borkowski S, Hilger CS, Cook G, Warren S, et al. Tumor targeting by an aptamer. J Nucl Med 2006; 47:668-78; PMID:16595502
- Daniels DA, Chen H, Hicke BJ, Swiderek KM, Gold L. A tenascin-C aptamer identified by tumor cell SELEX: systematic evolution of ligands by exponential enrichment. Proc Natl Acad Sci U A 2003; 100:15416-21; PMID:14676325; http://dx.doi.org/10.1073/pnas.2136683100
- Ko HY, Choi KJ, Lee CH, Kim S. A multimodal nanoparticle-based cancer imaging probe simultaneously targeting nucleolin, integrin alphavbeta3 and tenascin-C proteins. Biomaterials 2011; 32:1130-8; PMID:21071077; http://dx.doi.org/10.1016/j.biomaterials.2010.10.034
- Burnett JC, Rossi JJ. RNA-based therapeutics: current progress and future prospects. Chem Biol 2012; 19:60-71; PMID:22284355; http://dx.doi.org/10.1016/j.chembiol.2011.12.008
- Weissleder R. Molecular imaging in cancer. Science 2006; 312:1168-71; PMID:16728630; http://dx.doi.org/10.1126/science.1125949
- Banerjee SR, Pullambhatla M, Byun Y, Nimmagadda S, Green G, Fox JJ, Horti A, Mease RC, Pomper MG. 68Ga-labeled inhibitors of prostate-specific membrane antigen (PSMA) for imaging prostate cancer. J Med Chem 2010; 53:5333-41; PMID:20568777; http://dx.doi.org/10.1021/jm100623e
- Shah C, Miller TW, Wyatt SK, McKinley ET, Olivares MG, Sanchez V, Nolting DD, Buck JR, Zhao P, Ansari MS, et al. Imaging biomarkers predict response to anti-HER2 (ErbB2) therapy in preclinical models of breast cancer. Clin Cancer Res 2009; 15:4712-21; PMID:19584166; http://dx.doi.org/10.1158/1078-0432.CCR-08-2635
- Christine C, Koubemba M, Shakir S, Clavier S, Ehret-Sabatier L, Saupe F, Orend G, Charbonniere LJ. Synthesis of an activated phosphonated bifunctional chelate with potential for PET imaging and radiotherapy. Org. Biomol. Chem. 2012; 10:9183-90; PMID:23086384; http://dx.doi.org/10.1039/c2ob26452h
- Abada S, Lecointre A, Christine C, Ehret-Sabatier L, Saupe F, Orend G, Brasse D, Ouadi A, Hussenet T, Laquerrière P, et al. Phosphonated Chelates for Nuclear Imaging. Org. Biomol. Chem. 2014; 12: 9601-20; PMID:25338628; http://dx.doi.org/10.1039/C4OB01514B
- Abada S, Lecointre A, Elhabiri M, Charbonniere LJ. Formation of very stable and selective Cu(II) complexes with a non-macrocyclic ligand: can basicity rival pre-organization? Dalton Trans 2010; 39:9055-62; PMID:20725674; http://dx.doi.org/10.1039/c0dt00453g
- Aufderheide E, Ekblom P. Tenascin during gut development: appearance in the mesenchyme, shift in molecular forms, and dependence on epithelial-mesenchymal interactions. J Cell Biol 1988; 107:2341-9; PMID:2461951; http://dx.doi.org/10.1083/jcb.107.6.2341
- Huijbers EJ, Ringvall M, Femel J, Kalamajski S, Lukinius A, Abrink M, Hellman L, Olsson AK. Vaccination against the extra domain-B of fibronectin as a novel tumor therapy. Faseb J 2010; 24:4535-44; PMID:20634349; http://dx.doi.org/10.1096/fj.10-163022
- Femel J, Huijbers EJM, Saupe F, Cedervall J, Zhang L, Roswall P, Larsson E, Olofsson H, Pietras K, Dimberg A, et al. Therapeutic vaccination against fibronectin ED-A attenuates progression of metastatic breast cancer. Oncotarget 2014; 5:12418-27; PMID:25360764
- Huijbers EJ. Development of a Cancer Vaccine Targeting Tumor Blood Vessels. 2012; ISBN: 987-91-554-8317-3
- Dutoit V, Herold-Mende C, Hilf N, Schoor O, Beckhove P, Bucher J, Dorsch K, Flohr S, Fritsche J, Lewandrowski P, et al. Exploiting the glioblastoma peptidome to discover novel tumour-associated antigens for immunotherapy. Brain J Neurol 2012; 135:1042-54; PMID:22418738; http://dx.doi.org/10.1093/brain/aws042
- Halford S, Rampling R, James A, Peoples S, Mulholland P, Al-Salihi O, Twelves C, McBain C, Jefferies S, Kutscher S, et al. Results from a cancer research UK first in man phase I trial of Ima950 (a novel multi peptide vaccine) plus Gm-Csf in patients with newly diagnosed glioblastoma. Ann Oncol 2014; 25:iv364-5
- Immatics biotechnologies GmbH (2014). Promising results in phase 1 trial lead immatics to continue work on vaccine (IMA950) for glioblastoma. [Press release]. http://www.immatics.com/index.php?page=57&modaction=detail&modid=403&PHPSESSID=03b364a73eeccf59034b3463a64245f0
- Raghow R. The role of extracellular matrix in postinflammatory wound healing and fibrosis. FASEB J Off Publ Fed Am Soc Exp Biol 1994; 8:823-31; PMID:8070631
- Delanian S, Lefaix J-L. The radiation-induced fibroatrophic process: therapeutic perspective via the antioxidant pathway. Radiother Oncol J Eur Soc Ther Radiol Oncol 2004; 73:119-31; PMID:15542158; http://dx.doi.org/10.1016/j.radonc.2004.08.021
- Lafuma C, El Nabout RA, Crechet F, Hovnanian A, Martin M. Expression of 72-kDa gelatinase (MMP-2), collagenase (MMP-1), and tissue metalloproteinase inhibitor (TIMP) in primary pig skin fibroblast cultures derived from radiation-induced skin fibrosis. J Invest Dermatol 1994; 102:945-50; PMID:8006459; http://dx.doi.org/10.1111/1523-1747.ep12384118
- Kis E, Szatmári T, Keszei M, Farkas R, Ésik O, Lumniczky K, Falus A, Sáfrány G. Microarray analysis of radiation response genes in primary human fibroblasts. Int J Radiat Oncol • Biol • Phys 2006; 66:1506-14; PMID:17069989; http://dx.doi.org/10.1016/j.ijrobp.2006.08.004
- Cordes N, van Beuningen D. Cell adhesion to the extracellular matrix protein fibronectin modulates radiation-dependent G2 phase arrest involving integrin-linked kinase (ILK) and glycogen synthase kinase-3beta (GSK-3beta) in vitro. Br J Cancer 2003; 88:1470-9; PMID:12778079; http://dx.doi.org/10.1038/sj.bjc.6600912
- Ng Q-S, Goh V, Milner J, Padhani AR, Saunders MI, Hoskin PJ. Acute tumor vascular effects following fractionated radiotherapy in human lung cancer: In vivo whole tumor assessment using volumetric perfusion computed tomography. Int J Radiat Oncol Biol Phys 2007; 67:417-24; PMID:17236965; http://dx.doi.org/10.1016/j.ijrobp.2006.10.005
- Nagata Y. Recent advances in radiation oncology. Int J Clin Oncol 2014; 19:563; PMID:24962288; http://dx.doi.org/10.1007/s10147-014-0720-4
- Chino J, Das S, Wong T. Positron emission tomography in radiation treatment planning: the potential of metabolic imaging. Radiol Clin North Am 2013; 51:913-25; PMID:24010913; http://dx.doi.org/10.1016/j.rcl.2013.05.007
- De Neve W, De Gersem W, Madani I. Rational use of intensity-modulated radiation therapy: the importance of clinical outcome. Semin Radiat Oncol 2012; 22:40-9; PMID:22177877; http://dx.doi.org/10.1016/j.semradonc.2011.09.003
- Brada M, Bortfeld T. Proton therapy: the present and the future. Semin Radiat Oncol 2013; 23:75-6; PMID:23473683; http://dx.doi.org/10.1016/j.semradonc.2012.11.001
- Lawrence WF. Comparative effectiveness research in practice and policy for radiation oncology. Semin Radiat Oncol 2014; 24:54-60; PMID:24314343; http://dx.doi.org/10.1016/j.semradonc.2013.09.001
- Aneja S, Yu JB. Comparative effectiveness research in radiation oncology: stereotactic radiosurgery, hypofractionation, and brachytherapy. Semin Radiat Oncol 2014; 24:35-42; PMID:24314340; http://dx.doi.org/10.1016/j.semradonc.2013.08.004
- Ahmad SS, Duke S, Jena R, Williams MV, Burnet NG. Advances in radiotherapy. BMJ 2012; 345:e7765; PMID:23212681; http://dx.doi.org/10.1136/bmj.e7765
- Travis LB, Ng AK, Allan JM, Pui C-H, Kennedy AR, Xu XG, Purdy JA, Applegate K, Yahalom J, Constine LS, et al. Second malignant neoplasms and cardiovascular disease following radiotherapy. J Natl Cancer Inst 2012; 104:357-70; PMID:22312134; http://dx.doi.org/10.1093/jnci/djr533
- Bristow RG. Introduction: DNA repair and radiotherapy targeting: an overview. Semin Radiat Oncol 2010; 20:215-6; PMID:20832012; http://dx.doi.org/10.1016/j.semradonc.2010.06.002
- Garcia LM, Leblanc J, Wilkins D, Raaphorst GP. Fitting the linear-quadratic model to detailed data sets for different dose ranges. Phys Med Biol 2006; 51:2813-23; PMID:16723768; http://dx.doi.org/10.1088/0031-9155/51/11/009
- Kirkpatrick JP, Meyer JJ, Marks LB. The linear-quadratic model is inappropriate to model high dose per fraction effects in radiosurgery. Semin Radiat Oncol 2008; 18:240-3; PMID:18725110; http://dx.doi.org/10.1016/j.semradonc.2008.04.005
- Barcellos-Hoff MH, Park C, Wright EG. Radiation and the microenvironment - tumorigenesis and therapy. Nat Rev Cancer 2005; 5:867-75; PMID:16327765; http://dx.doi.org/10.1038/nrc1735
- Kuonen F, Secondini C, Rüegg C. Molecular pathways: emerging pathways mediating growth, invasion, and metastasis of tumors progressing in an irradiated microenvironment. Clin Cancer Res Off J Am Assoc Cancer Res 2012; 18:5196-202; PMID:22730447; http://dx.doi.org/10.1158/1078-0432.CCR-11-1758
- De Meerleer G, Khoo V, Escudier B, Joniau S, Bossi A, Ost P, Briganti A, Fonteyne V, Van Vulpen M, Lumen N, et al. Radiotherapy for renal-cell carcinoma. Lancet Oncol 2014; 15:e170-7; PMID:24694640; http://dx.doi.org/10.1016/S1470-2045(13)70569-2
- Riekki R, Jukkola A, Oikarinen A, Kallioinen M. Radiation therapy induces tenascin expression and angiogenesis in human skin. Acta Derm Venereol 2001; 81:329-33; PMID:11800138; http://dx.doi.org/10.1080/000155501317140025
- Bouchard G, Bouvette G, Therriault H, Bujold R, Saucier C, Paquette B. P re-irradiation of mouse mammary gland stimulates cancer cell migration and development of lung metastases. Br J Cancer 2013; 109:1829-38; PMID:24002607; http://dx.doi.org/10.1038/bjc.2013.502
- Monnier Y, Farmer P, Bieler G, Imaizumi N, Sengstag T, Alghisi GC, Stehle J-C, Ciarloni L, Andrejevic-Blant S, Moeckli R, et al. CYR61 and alphaVbeta5 integrin cooperate to promote invasion and metastasis of tumors growing in preirradiated stroma. Cancer Res 2008; 68:7323-31; PMID:18794119; http://dx.doi.org/10.1158/0008-5472.CAN-08-0841
- Geffrotin C, Tricaud Y, Crechet F, Castelli M, Lefaix JL, Vaiman M. Unlike tenascin-X, tenascin-C is highly up-regulated in pig cutaneous and underlying muscle tissue developing fibrosis after necrosis induced by very high-dose gamma radiation. Radiat Res 1998; 149:472-81; PMID:9588358; http://dx.doi.org/10.2307/3579787
- Bartel-Friedrich S, Lautenschläger C, Holzhausen H-J, Friedrich RE. Expression and distribution of tenascin in rat submandibular glands following irradiation. Anticancer Res 2010; 30:1593-8; PMID:20592347
- Hakim SG, Ribbat J, Berndt A, Richter P, Kosmehl H, Benedek GA, Jacobsen HC, Trenkle T, Sieg P, Rades D. Expression of Wnt-1, TGF-β and related cell-cell adhesion components following radiotherapy in salivary glands of patients with manifested radiogenic xerosto mia. Radiother Oncol J Eur Soc Ther Radiol Oncol 2011; 101:93-9; PMID:21885141; http://dx.doi.org/10.1016/j.radonc.2011.07.032
- Beutel J, Schroder C, von Hof K, Rades D, Kosmehl H, Wedel T, Sieg P, Geerling G, Hakim SG. Pharmacological prevention of radiation-induced dry eye-an experimental study in a rabbit model. Graefes Arch Clin Exp Ophthalmol Albrecht Von Graefes Arch Für Klin Exp Ophthalmol 2007; 245:1347-55; PMID:17318564; http://dx.doi.org/10.1007/s00417-007-0548-y
- Li XA. Adaptive Radiation Therapy. CRC Press; 2011; ISBN: 9781439816356
- Vujaskovic Z, Anscher MS, Feng QF, Rabbani ZN, Amin K, Samulski TS, Dewhirst MW, Haroon ZA. Radiation-induced hypoxia may perpetuate late normal tissue injury. Int J Radiat Oncol Biol Phys 2001; 50:851-5; PMID:11429211; http://dx.doi.org/10.1016/S0360-3016(01)01593-0
- Rubner Y, Wunderlich R, Rühle P-F, Kulzer L, Werthmöller N, Frey B, Weiss E-M, Keilholz L, Fietkau R, Gaipl US. How does ionizing irradiation contribute to the induction of anti-tumor immunity? Front Oncol 2012; 2:75; PMID:22848871; http://dx.doi.org/10.3389/fonc.2012.00075
- Udalova IA, Ruhmann M, Thomson SJP, Midwood KS. Expression and immune function of tenascin-C. Crit Rev Immunol 2011; 31:115-45; PMID:21542790; http://dx.doi.org/10.1615/CritRevImmunol.v31.i2.30
- Symon Z, Goldshmidt Y, Picard O, Yavzori M, Ben-Horin S, Alezra D, Barshack I, Chowers Y. A murine model for the study of molecular pathogenesis of radiation proctitis. Int J Radiat Oncol Biol Phys 2010; 76:242-50; PMID:20005457; http://dx.doi.org/10.1016/j.ijrobp.2009.07.1736
- Dancea HC, Shareef MM, Ahmed MM. Role of radiation-induced TGF-beta signaling in cancer therapy. Mol Cell Pharmacol 2009; 1:44-56; PMID:20336170; http://dx.doi.org/10.4255/mcpharmacol.09.06
- Bierie B, Moses HL. Transforming growth factor beta (TGF-beta) and inflammation in cancer. Cytokine Growth Factor Rev 2010; 21:49-59; PMID:20018551; http://dx.doi.org/10.1016/j.cytogfr.2009.11.008
- Jinnin M, Ihn H, Asano Y, Yamane K, Trojanowska M, Tamaki K. Tenascin-C upregulation by transforming growth factor-β in human dermal fibroblasts involves Smad3, Sp1, and Ets1. Oncogene 2004; 23:1656-67; PMID:15001984; http://dx.doi.org/10.1038/sj.onc.1207064
- Katoh D, Nagaharu K, Shimojo N, Hanamura N, Yamashita M, Kozuka Y, Imanaka-Yoshida K, Yoshida T. Binding of αvβ1 and αvβ6 integrins to tenascin-C induces epithelial-mesenchymal transition-like change of breast cancer cells. Oncogenesis 2013; 2:e65; PMID:23958855; http://dx.doi.org/10.1038/oncsis.2013.27
- De Laporte L, Rice JJ, Tortelli F, Hubbell JA. Tenascin C promiscuously binds growth factors via its fifth fibronectin type III-like domain. PloS One 2013; 8:e62076; PMID:23637968; http://dx.doi.org/10.1371/journal.pone.0062076
- Gulbins E. Regulation of death receptor signaling and apoptosis by ceramide. Pharmacol Res Off J Ital Pharmacol Soc 2003; 47:393-9; PMID:12676513
- Logsdon EA, Finley SD, Popel AS, Gabhann FM. A systems biology view of blood vessel growth and remodelling. J Cell Mol Med 2014; 18:1491-508; PMID:24237862; http://dx.doi.org/10.1111/jcmm.12164
- Imaizumi N, Monnier Y, Hegi M, Mirimanoff R-O, Rüegg C. Radiotherapy suppresses angiogenesis in mice through TGF-betaRI/ALK5-dependent inhibition of endothelial cell sprouting. PloS One 2010; 5:e11084; PMID:20552031; http://dx.doi.org/10.1371/journal.pone.0011084
- Ferrara N. Vascular endothelial growth factor: basic science and clinical progress. Endocr Rev 2004; 25:581-611; PMID:15294883; http://dx.doi.org/10.1210/er.2003-0027
- Goel HL, Mercurio AM. VEGF targets the tumour cell. Nat Rev Cancer 2013; 13:871-82; PMID:24263190; http://dx.doi.org/10.1038/nrc3627
- O’Connell JT, Sugimoto H, Cooke VG, MacDonald BA, Mehta AI, LeBleu VS, Dewar R, Rocha RM, Brentani RR, Resnick MB, et al. VEGF-A and tenascin-C produced by S100A4+ stromal cells are important for metastatic colonization. Proc Natl Acad Sci U S A 2011; 108:16002-7; PMID:21911392; http://dx.doi.org/10.1073/pnas.1109493108
- Helleman J, Jansen MPHM, Ruigrok-Ritstier K, van Staveren IL, Look MP, Meijer-van Gelder ME, Sieuwerts AM, Klijn JGM, Sleijfer S, Foekens JA, et al. Association of an extracellular matrix gene cluster with breast cancer prognosis and endocrine therapy response. Clin Cancer Res Off J Am Assoc Cancer Res 2008; 14:5555-64; PMID:18765548; http://dx.doi.org/10.1158/1078-0432.CCR-08-0555
- Mancuso MR, Davis R, Norberg SM, O’Brien S, Sennino B, Nakahara T, Yao VJ, Inai T, Brooks P, Freimark B, et al. Rapid vascular regrowth in tumors after reversal of VEGF inhibition. J Clin Invest 2006; 116:2610-21; PMID:17016557; http://dx.doi.org/10.1172/JCI24612
- Huang W, Chiquet-Ehrismann R, Moyano JV, Garcia-Pardo A, Orend G. Interference of tenascin-C with syndecan-4 binding to fibronectin blocks cell adhesion and stimulates tumor cell proliferation. Cancer Res 2001; 61:8586-94; PMID:11731446
- De Wever O, Sobczak-Thépot J, Vercoutter-Edouart A-S, Michalski J-C, Ouelaa-Benslama R, Stupack DG, Bracke M, Wang JYJ, Gespach C, Emami S. Priming and potentiation of DNA damage response by fibronectin in human colon cancer cells and tumor-derived myofibroblasts. Int J Oncol 2011; 39:393-400; PMID:21567080
- McManus KJ, Hendzel MJ. ATM-dependent DNA damage-independent mitotic phosphorylation of H2AX in normally growing mammalian cells. Mol Biol Cell 2005; 16:5013-25; PMID:16030261; http://dx.doi.org/10.1091/mbc.E05-01-0065
- Ruiz C, Huang W, Hegi ME, Lange K, Hamou M-F, Fluri E, Oakeley EJ, Chiquet-Ehrismann R, Orend G. Growth promoting signaling by tenascin-C [corrected]. Cancer Res 2004; 64:7377-85; PMID:15492259
- Gosepath EM, Eckstein N, Hamacher A, Servan K, von Jonquieres G, Lage H, Györffy B, Royer HD, Kassack MU. Acquired cisplatin resistance in the head-neck cancer cell line Cal27 is associated with decreased DKK1 expression and can partially be reversed by overexpression of DKK1. Int J Cancer J Int Cancer 2008; 123:2013-9; PMID:18688867; http://dx.doi.org/10.1002/ijc.23721
- Shou J, Ali-Osman F, Multani AS, Pathak S, Fedi P, Srivenugopal KS. Human Dkk-1, a gene encoding a Wnt antagonist, responds to DNA damage and its overexpression sensitizes brain tumor cells to apoptosis following alkylation damage of DNA. Oncogene 2002; 21:878-89; PMID:11840333; http://dx.doi.org/10.1038/sj.onc.1205138
- Niehrs C. Function and biological roles of the Dickkopf family of Wnt modulators. Oncogene 2006; 25:7469-81; PMID:17143291; http://dx.doi.org/10.1038/sj.onc.1210054
- Reardon DA, Akabani G, Coleman RE, Friedman AH, Friedman HS, Herndon JE, McLendon RE, Pegram CN, Provenzale JM, Quinn JA, et al. Salvage radioimmunotherapy with murine iodine-131-labeled antitenascin monoclonal antibody 81C6 for patients with recurrent primary and metastatic malignant brain tumors: phase II study results. J Clin Oncol 2006; 24:115-22; PMID:16382120; http://dx.doi.org/10.1200/JCO.2005.03.4082
- Reardon DA, Zalutsky MR, Akabani G, Coleman RE, Friedman AH, Herndon JE, McLendon RE, Pegram CN, Quinn JA, Rich JN, et al. A pilot study: 131I-antitenascin monoclonal antibody 81c6 to deliver a 44-Gy resection cavity boost. Neuro Oncol 2008; 10:182-9; PMID:18287339; http://dx.doi.org/10.1215/15228517-2007-053
- Zalutsky MR, Reardon DA, Akabani G, Coleman RE, Friedman AH, Friedman HS, McLendon RE, Wong TZ, Bigner DD. Clinical experience with alpha-particle emitting 211At: treatment of recurrent brain tumor patients with 211At-labeled chimeric antitenascin monoclonal antibody 81C6. J Nucl Med 2008; 49:30-8; PMID:18077533; http://dx.doi.org/10.2967/jnumed.107.046938
- Riva P, Arista A, Tison V, Sturiale C, Franceschi G, Spinelli A, Riva N, Casi M, Moscatelli G, Frattarelli M. Intralesional radioimmunotherapy of malignant gliomas. An effective treatment in recurrent tumors. Cancer 1994; 73:1076-82; PMID:8306250; http://dx.doi.org/10.1002/1097-0142(19940201)73:3+%3c1076::AID-CNCR2820731347%3e3.0.CO;2-Z
- Paganelli G, Bartolomei M, Ferrari M, Cremonesi M, Broggi G, Maira G, Sturiale C, Grana C, Prisco G, Gatti M, et al. Pre-targeted locoregional radioimmunotherapy with 90Y-biotin in glioma patients: phase I study and preliminary therapeutic results. Cancer Biother Radiopharm 2001; 16:227-35; PMID:11471487; http://dx.doi.org/10.1089/10849780152389410