ABSTRACT
The ability to induce ovulation with an injection of GnRH facilitated the development of fixed-time artificial insemination (AI) protocols. However, animals that exhibit estrus prior to fixed-time AI have greater pregnancy success. Thus, the objectives of the present experiments were to determine the impact of estrus expression prior to fixed-time AI on timing of conception and to characterize the role of preovulatory estradiol in regulating changes in expression of uterine genes. In experiment 1, data were collected from 4,499 beef cows inseminated by fixed-time AI in 31 different herds. Animals that did not conceive to AI but exhibited estrus before timed-AI were more likely to conceive during cycle 1 after AI, and overall conceived earlier in the breeding season compared to animals that did not exhibit estrus. In experiment 2, beef cows were synchronized using a fixed-time AI protocol. Uterine horn biopsies and blood samples were collected on Day 0, 5, 10, or 16. Concentrations of estradiol on Day 0 did not influence expression of progesterone receptor, ER beta, or oxytocin receptor. Increased concentrations of estradiol on Day 0 increased expression of ER alpha from Days 0 to 5 of the estrous cycle. Furthermore, cows with increased concentrations of estradiol on Day 0 had increased expression of inhibin beta A, and uterine milk protein precursor. Thus, animals that do not exhibit estrus prior to fixed-time AI had decreased breeding season pregnancy success and conceived later in the breeding season, and preovulatory concentrations of estradiol likely play a major role in this establishment of pregnancy, not only directly by regulating uterine gene expression, but also indirectly throughout the subsequent estrous cycle.
KEYWORDS:
Introduction
Fixed-time AI (FTAI) protocols have been developed to eliminate the need for estrous detection, greatly decreasing labor requirements and increasing the number of cows inseminated by AI. These protocols are possible since gonadotropin-releasing hormone (GnRH) is capable of inducing ovulation regardless of estrus expression or the corresponding rise in estradiol (Ryan et al. Citation1998). However, induction of ovulation without expression of estrus has been reported to have decreased conception rates (Bridges et al. Citation2012a; Whittier et al. Citation2013; Hill et al. Citation2014) likely from inadequate estrogen concentrations to properly regulate the uterine environment for subsequent sperm transport and gamete-conceptus survival (Perry and Perry Citation2008a, Citation2008b). Thus, cows that did not exhibit standing estrus around the time of GnRH-induced ovulation (± 24 h) had decreased concentrations of estradiol at AI and decreased pregnancy success compared to cows that did exhibit estrus (Perry et al. Citation2005, Citation2007; Richardson et al. Citation2016). Research by Atkins et al. (Citation2013) and Jinks et al. (Citation2013) reported that estradiol concentrations at GnRH-induced ovulation (d 0; time insemination would occur) affected pregnancy success of embryo recipient cows independent of progesterone concentrations on d 7. Among donor cows, increased concentrations of estradiol on d 0 tended to improve embryo quality and viability without affecting embryo stage (Jinks et al. Citation2013).
During the estrous cycle, the endometrium undergoes changes in composition and differentiation status. These changes are mainly regulated by estradiol, progesterone, and oxytocin (Spencer et al. Citation2004), and expression of uterine oxytocin and steroid receptors change throughout the estrous cycle (Robinson et al. Citation2001). Thus, the uterine environment changes dynamically during the estrous cycle, and this environment plays a vital role in maternal recognition of pregnancy, elongation, and attachment through secretion of nutrients, growth factors, immunosuppressive agents, enzymes, and ions that are necessary for proper embryonic growth and development (Geisert et al. Citation1992; Gray et al. Citation2001). More specifically, whether or not an animal was exposed to elevated concentrations of estradiol has been reported to impact gene expression within the endometrium (Bridges et al. Citation2012b). Estradiol-induced expression of endometrial receptors, production of uterine proteins (Bartol et al. Citation1981), and also influenced the expression of many genes involved in uterine extracellular matrix remodeling (Bauersachs et al. Citation2005). Bridges et al. (Citation2012b) determined nuclear progesterone receptors in the deep glandular epithelium and mRNA abundance of ERα in the uterine epithelium was decreased among animals that had decreased preovulatory estradiol concentrations compared to animals that were exposed to elevated estradiol concentrations during the preovulatory period on d 15 after insemination. On d 19 after insemination, Davoodi et al. (Citation2016) reported that genes associated with maternal immune system suppression (IGLL1, CXCL10, MX2, SLPI), attachment between the endometrium and conceptus (MMP19, MYL12A), and CL maintenance (OXT, COX2, FPr, CYP11A, BAX) were favorably expressed in cows that exhibited estrus near the time of AI.
In addition, the timing of changes in the uterine environment is of critical importance to embryo survival. Estradiol has been reported to play a direct role in the regulation of the biological clock in the uterus (Nakamura et al. Citation2005). A number of transgenic mouse models have implicated members of the circadian clock genes in proper mammalian reproductive function (Kennaway Citation2005; Boden and Kennaway Citation2006). In cattle, synchrony between a transferred embryo and the uterus must be ± 24 h for proper embryo attachment to occur (Hasler Citation2001). Thus from these studies, we hypothesized that endogenous preovulatory estradiol concentrations regulate the uterine clock during the subsequent estrous cycle, and is necessary for proper fertilization and early embryonic survival. Therefore, the objectives of the present experiments were to determine the impact of estrus expression prior to fixed-time AI on timing of when cows conceive during the breeding season, and to characterize the role of estradiol at time of GnRH-induced ovulation in regulating changes in uterine expression of hormone receptors (estradiol receptor alpha, estradiol receptor beta, progesterone receptor, and oxytocin receptor) and uterine proteins that might initiate down-stream effects on uterine function and pregnancy success (period 1, proenkephalin-A, inhibin beta A, and uterine milk protein precursor).
Results
Experiment 1 – Timing of conception
Animals that exhibited estrus before fixed-time AI had increased (P < 0.01) pregnancies to AI (64 ± 1.3% vs 45 ± 1.6%, respectively; n = 4,499) and increased pregnancies during the entire breeding season (93 ± 0.7% vs 89 ± 1.1%, respectively; n = 4,499) compared to animals that did not exhibit estrus. There was a tendency for age/parity (P < 0.09) on pregnancies to AI or on pregnancies during the entire breeding season. With heifers tending (P = 0.08) to have greater pregnancies to AI cows (57 ± 5.3% and 54 ± 5.1%, respectively), but cows tended (P = 0.07) to have greater pregnancies during the breeding season compared to heifers (92 ± 1.0% and 90 ± 1.2%, respectively). However, days postpartum did impact initial pregnancies to AI (P = 0.01; cows that were 60 days postpartum or less had decreased AI pregnancies compared to cows that were 80 or 100 days postpartum), but days postpartum had no impact on animals conceiving during the breeding season (P = 0.71). Body condition score at AI had no impact on pregnancies to AI (P = 0.11), but did impact pregnancies during the entire breeding season (P = 0.02); cows that were a condition score 4 had decreased success (86.3 ± 2.5%) compared to cows in a condition of 5 or 6 (92.1 ± 2.4% and 93.0 ± 2.5%, respectively). Of the animals that did not conceive to fixed-time AI but did conceive during the breeding season (n = 1,116), expression of estrus before fixed-time AI resulted in animals conceiving earlier in the breeding season (P < 0.01; d 36 ± 1) compared to animals that did not exhibit estrus before fixed-time AI (day 41 ± 1). Age/parity also influenced when animals conceived during the breeding season with heifers conceiving earlier than cows (P = 0.01; day 35 ± 1.3 and 39 ± 0.6; respectively). There was no estrus expression by age interaction (P = 0.83). Furthermore, timing of conception during the breeding season was not influenced by days postpartum (P = 0.25) or body condition score (P = 0.28; n = 704). There was no difference (P = 0.74) in embryonic loss between groups (2.3 ± 0.3% and 2.1 ± 0.4% for estrus and no estrus, respectively).
When only animals that did not conceive to fixed-time AI were evaluated there was an impact of exhibiting estrus before fixed-time AI on the percentage of animals that conceived during cycle 1 (P < 0.01; days 10 to 31 of the breeding season; n = 1577). More animals that exhibited estrus before fixed-time AI conceived during the first return to estrus (days 10 to 31 of the breeding season) compared to animals that did not exhibit estrus before fixed-time AI (P < 0.01; 58 ± 9% vs 38 ± 7%). Furthermore, age had a significant impact on conceiving during this first return to estrus (P < 0.01), with a greater percentage of heifers conceiving in cycle 1 compared to cows (39 ± 2% vs 30 ± 2%). However, there was no estrus by age interaction (P = 0.18). During the second (days 32 to 53; n = 1063) and third (days 54 to 75; n = 612) cycles of the breeding season there was no effect of estrus (P > 0.57; 72.6 ± 10.1% vs 78.0 ± 8.5% and 64.5 ± 17.6% vs 75.0 ± 16.5% for no estrus and estrus within cycle 2 and 3, respectively), age (P > 0.82), or an estrus by age interaction (P > 0.24). During the fourth (after day 75; n = 510) cycle of the breeding season a greater percentage of animals that did not exhibit estrus before the start of the breeding season conceived (P = 0.02; 15 ± 2% vs 9 ± 2% for no estrus and estrus, respectively). But, there was no effect of age (P = 0.65) or an estrus by age interaction (P= 0.55).
Experiment 2
Circulating concentrations of estradiol on Day 0 (P < 0.001) were greater in estrus cows compared to non-estrus cows (). There was also a significant impact of preovulatory estradiol (P = 0.03), time (P < 0.01), and a preovulatory estradiol by time interaction (P < 0.01) on circulating concentrations of progesterone. Concentrations of progesterone did not differ (P > 0.13) between treatments on Day 0, 5, or 10 but were greater (P < 0.001) on Day 16 in estrus cows compared to non-estrus cows ().
Hormone receptors
There were no differences (P = 0.74) in expression of progesterone receptor between estrus (8.7 ± 0.7) and non-estrus (8.3 ± 0.7) cows. There was an effect of day (P < 0.01; ). Overall, expression was similar (P = 0.25) on Day 0 (13.9 ± 1.1) and Day 5 (12.2 ± 1.1), then decreased (P < 0.01) to Day 10 (5.8 ± 1.0) and tended to further decrease (P = 0.07) to Day 16 (2.2 ± 1.4). When separating treatment-by-day, expression in estrus cows was similar between days 0 and 5 (P = 0.89) and then decreased (P < 0.01) to days 10 and 16 which were similar (P = 0.20). Among non-estrus cows, expression was similar between days 0 and 5 (P = 0.16) and then decreased (P = 0.01) to days 10 and tended (P = 0.08) to further decreases to day 16.
Figure 3. Relative expression of progesterone receptor on Days 0, 5, 10, and 16 of the estrous cycle for cows in the estrus and non-estrus groups. Days having different superscripts are different (ab and acP < 0.01; bcP = 0.07).
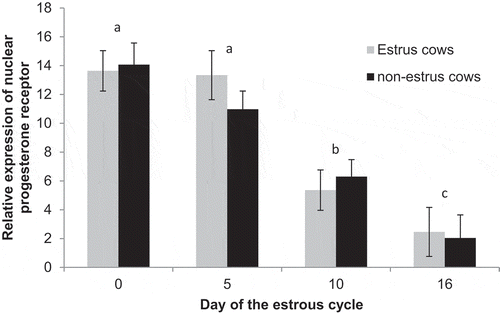
Expression of ER alpha did not differ (P = 0.72) between estrus (13.7 ± 1.6) and non-estrus (12.9 ± 1.7) cows. There was an effect of day (P < 0.01; ), with expression being greater on Day 5 (21.9 ± 2.4) compared to Day 0 (P = 0.02; 13.1 ± 2.5), Day 10 (P = 0.04; 15.0 ± 2.2), and Day 16 (P < 0.01; 3.3 ± 3.1). Furthermore, expression was similar (P = 0.56) between Days 0 and 10, and both were greater (P < 0.02) than Day 16. Expression of ER alpha in estrus cows increased from Day 0 to 5 (P < 0.01), then decreased from day 5 to 10 (P = 0.01) and tended (P = 0.06) to further decrease to day 16. Among non-estrus cows, expression remained similar from Day 0 to day 5 and 10 (P > 0.58) but then decreased to day 16 (P < 0.03; ). Furthermore, expression tended to differ between estrus cows and non-estrus cows on day 5 (P = 0.10).
Figure 4. Relative expression of estrogen receptor alpha on Days 0, 5, 10, and 16 of the estrous cycle for cows in the estrus and non-estrus groups. Bars having different superscripts are different (abP < 0.05; acP < 0.02; bcP < 0.01; bdP < 0.09; ceP < 0.07).
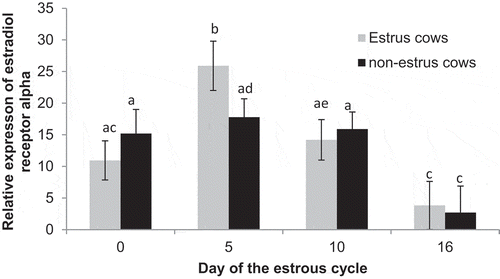
Expression of ER beta did not differ (P = 0.28) between estrus cows (7.1 ± 1.0) and non-estrus cows (5.5 ± 1.1). There tended (P = 0.06) to be a day effect with increased expression on Days 0 (6.2 ± 1.6; P = 0.09), 5 (7.5 ± 1.5; P = 0.04), and 10 (9.6 ± 1.4; P < 0.01) compared to Day 16 (1.9 ± 2.0; ), and expression tended (P = 0.08) to differ between estrus cows and non-estrus cows on day 5.
Figure 5. Relative expression of estrogen receptor beta on Days 0, 5, 10, and 16 of the estrous cycle for cows in the estrus and non-estrus groups. Days having different superscripts are different (acP < 0.03; bcP = 0.09).
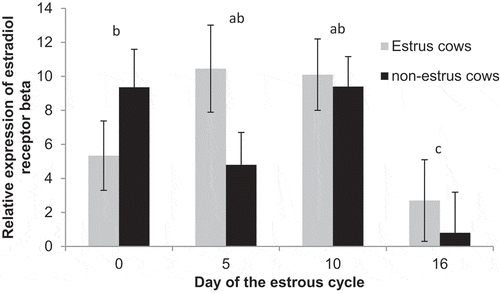
Expression of oxytocin receptor did not differ (P = 0.12) between estrus cows (4.8 ± 0.5) and non-estrus cows (3.7 ± 0.4). There was an effect of day (P < 0.01) with expression decreasing (P < 0.01) from Day 0 (9.6 ± 0.7) to Day 5 (4.2 ± 0.7) and further decreasing to Day 10 (1.5 ± 0.6) and Day 16 (1.7 ± 0.9). There were no days that expression differed between treatments (P > 0.0.25; ).
3.2 Uterine proteins
Expression of Period 1 was increased (P = 0.03) in estrus cows (11.9 ± 1.4) compared to non-estrus cows (7.6 ± 1.3). Furthermore, there was an effect of day, expression was increased on Days 5 (13.1 ± 2.0) and 10 (13.9 ± 1.9) compared to Day 0 (P < 0.01; 4.8 ± 2.1). Expression on Day 16 (7.2 ± 2.6) tended to be decreased compared to Day 10 (P = 0.08) and Day 5 (P= 0.10) but was similar to Day 0 (P = 0.47; ). Among estrus cows, expression increased (P = 0.01) from day 0 to 5, and remained elevated from day 5 to 10 to 16 (P > 0.38). Among non-estrus cows expression was similar between day 0 and 5 (P = 0.17), and 5 and 10 (P = 0.31), but was increased on day 10 compared to day 0 (P = 0.03) and 16 (P = 0.04). Furthermore, expression tended (P = 0.09) to be greater in estrus cows on day 5 compared to non-estrus cows.
Figure 7. Relative expression of Period 1 on Days 0, 5, 10, and 16 of the estrous cycle for cows in the estrus and non-estrus groups. Days having different superscripts are different (P < 0.05).
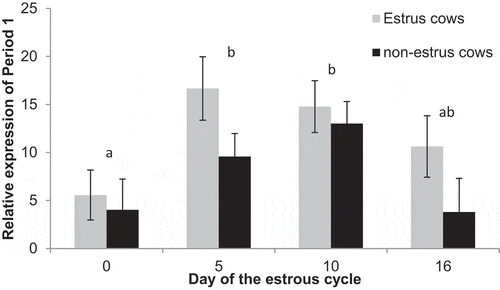
Expression of proenkephalin-A did not differ (P = 0.27) between estrus cows (10.7 ± 3.6) and non-estrus (4.9 ± 3.6). However, there was an effect of day (P = 0.02), expression was not detected on Day 0, but increased (P < 0.01) from Day 5 (4.6 ± 5.3) to Day 10 (21.6 ± 4.9), then tended to decrease (P = 0.09) from Day 10 to Day 16 (5.1 ± 6.8). Among estrus cows, expression increased from day 5 to 10 (P = 0.02) and tended to decrease to day 16 (P = 0.09). However, among non-estrus cows expression did not change between any days (P > 0.18), but expression tended (P = 0.07) to be decreased on day 10 among non-estrus compared to estrus cows ().
Figure 8. Relative expression of proenkephalin-A on Days 0, 5, 10, and 16 of the estrous cycle for cows in the estrus and non-estrus groups. Days having different superscripts are different (abP < 0.03).
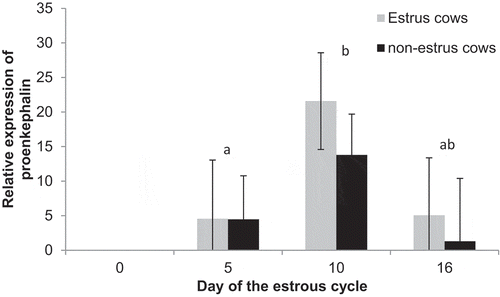
Expression of inhibin beta A was greater (P = 0.01) in estrus cows (2.7 ± 0.4) compared to non-estrus (1.0 ± 0.4) cows. Furthermore, there was an effect of day (P < 0.01), with expression being increased on Day 0 (6.1 ± 0.6) compared to Days 5 (0.5 ± 0.6), 10 (0.4 ± 0.6), and 16 (0.5 ± 0.8). There was also an estrus by day interaction (P < 0.01). Expression was greater (P < 0.01) in estrus cows compared to non-estrus cows on Day 0, but did not differ between treatments on Day 5, 10, or 16 ().
Figure 9. Relative expression of inhibin beta A on Days 0, 5, 10, and 16 of the estrous cycle for cows in the estrus and non-estrus groups. Bars having different superscripts are different (ab, ac, and adP < 0.01; bcP < 0.05; bdP < 0.10).
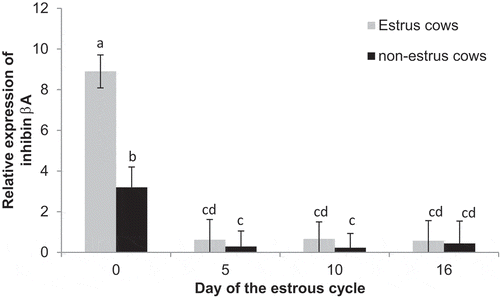
Expression of uterine milk protein precursor was greater (P = 0.03) in estrus cows (3.4 ± 0.8) compared to non-estrus (0.9 ± 0.8) cows. There was also a day effect (P = 0.03), with expression decreased (P < 0.01) from Day 0 (7.9 ± 1.1) to Days 5 (0.03 ± 1.2), 10 (0.4 ± 1.1), and 16 (0.7 ± 1.5). Furthermore, there was an estrus by day interaction (P = 0.01), with expression increased on Day 0 among estrus cows compared to the rest of the estrous cycle, but expression was similar among all days of the cycle in non-estrus cows ().
Discussion
Estradiol plays a pivotal role in initiation of standing estrus and the ovulatory cascade (see review Allrich Citation1994). It has also been reported to play a direct role in the regulation of the biological clock in the uterus (Nakamura et al. Citation2005). In the present study, more animals that exhibited standing estrus prior to fixed-time AI conceived to AI. This is similar to previous studies that have reported expression of estrus prior to fixed-time AI directly affected pregnancies per AI (Richardson et al. Citation2016). Interestingly, not only did more animals that exhibited estrus prior to fixed-time AI conceive to AI, but more of these animals conceived during the breeding season and they conceived earlier in the breeding season. This indicates that the preovulatory rise in estradiol may be critical to the timing of changes that occur in the uterus, and induction of ovulation without expression of estrus may require additional time to correct the timing in the uterus.
In ovariectomized cows, treatment with estradiol was required before progesterone treatment to inhibit an oxytocin-induced release of PGFM (Kieborz-Loos et al. Citation2003), and estradiol production by preovulatory follicles has also been correlated with the number of progesterone receptors present in the uterine endometrium (Zollers et al. Citation1993). In the present study, circulating concentrations of estradiol on d 0 were greater in animals that exhibited estrus compared to animals that did not. Furthermore, it appears that animals that did not exhibit standing estrus experienced earlier regression of the corpus luteum than animals that exhibited estrus as circulating concentrations of progesterone were decreased on day 16 among animals that did not exhibit estrus. Up-regulation of the oxytocin receptors plays a vital role in initiating luteal regression (McCracken et al. Citation1999). Transcript abundance of oxytocin receptor did not differ between estrus and non-estrus cows, and, therefore, likely is not the main factor resulting in the decrease in circulating progesterone on day 16 in non-estrus cows or in previously reported differences in fertility between estrus and non-estrus cows. Transcript abundance did follow a normal pattern in both groups with decreased expression from Day 0 to Day 5 and then continued to decrease to Day 10 and Day 16. Abundance of oxytocin receptor mRNA in luminal epithelium and superficial glands was elevated prior to Day 6 but during Days 6 to 16, oxytocin receptor transcript was not detectable or very lowly abundant (Robinson et al. Citation2001). Similarly staining for the oxytocin receptor was greatest during metestrus (Days 1 to 6), then decreased during diestrus (Days 7 to 16), but in contrast to the present study and other studies looking a transcript abundance protein increased on Day 16 of the estrous cycle (Kimmins and MacLaren Citation2001).
The timing of changes in the uterine environment are of critical importance to embryo survival. In cattle, synchrony between a transferred embryo and the uterus must be ± 24 hr (Hasler Citation2001), and ovarian estradiol plays an important role in establishing the timing of uterine receptivity (Ozturk and Demir Citation2010). During the estrous cycle, changes occur in the endometrium regarding its composition and differentiation status. These changes are mainly regulated by estradiol, progesterone, and oxytocin (Spencer et al. Citation2004), and transcript abundance of uterine oxytocin and steroid receptors (estradiol receptor alpha and nuclear progesterone receptor) change throughout the estrous cycle (Robinson et al. Citation2001).
In the present study, transcript abundance of ER alpha was greater on Day 5 compared to Days 0, 10, and 16 among estrus cows, but transcript abundance was similar on Days 0, 5, and 10 among non-estrus cows, whereas transcript abundance of ER beta was similar between estrus and non-estrus cows throughout the estrous cycle. From these results, there is very little evidence to suggest changes in transcription of ER alpha or ER beta are responsible for the observed improved fertility associated with estrus animals. This seems logical because there was no difference at Day 0, and it can be assumed that estradiol acting through its receptors would have set in motion down-stream pathways by the later stages of the estrous cycle. Robinson and co-workers (Citation2001) reported fluctuations in abundance of ER alpha mRNA throughout the estrous cycle with transcript abundance elevated on the day of estrus, decreasing on Day 2, increasing again during the early luteal phase, then decreasing during the mid- to late luteal phase before increasing again on Day 20 of the estrous cycle. Immunostaining for ER alpha in uterine epithelium and stroma throughout the estrous cycle revealed that the protein was in greatest quantities early in the estrous cycle and decreased during the early luteal phase (Robinson et al. Citation2001; Martin et al. Citation2008). An increase in protein was observed on Days 10 to 14 in luminal epithelium but was not seen in superficial glands or deep glands (Robinson et al. Citation2001) and was lowest during the late luteal phase in all cell types (Robinson et al. Citation2001; Martin et al. Citation2008). Both ER alpha and ER beta bind estrogen and the same estrogen response elements and can form either homodimers of heterodimers (Saunders Citation1998). However, ER beta binds estradiol with a lower affinity than ER alpha (Kuiper et al. Citation1996, Citation1997), and Brandenberger and co-workers (Citation1997) have reported ER beta transcripts in human uterine tissue but at a decreased abundance compared to ER alpha.
Cows that had a normal length luteal phase had greater preovulatory concentrations of estradiol compared to cows that experienced a short luteal phase (Sheffel et al. Citation1982; Garcia-Winder et al. Citation1986; Garverick et al. Citation1988; Braden et al. Citation1989), but exogenous estradiol increased uterine progesterone receptors (Zelinski et al. Citation1980) and permitted progesterone to coordinate the timing of prostaglandin F2α secretion (Zollers et al. Citation1993). Therefore, estradiol may not only have a direct effect on regulating the uterine environment but may also have an indirect role by regulating response to progesterone through regulation of the progesterone receptor. In the present study, all cows had a normal length estrous cycle (based on expression of estrus) and there were no differences in transcript abundance of progesterone receptor between estrus and non-estrus cows. Therefore, previously reported differences in fertility between estrus and non-estrus cows is likely not regulated through changes in transcript abundance of progesterone receptor. This seems logical since changes in progesterone receptors have been reported to be associated with short estrous cycles, and the decrease in transcript abundance from Days 0 and 5 to Days 10 and 16 are similar to previous reported changes. Kimmins and MacLaren (Citation2001) reported that transcript abundance of progesterone receptor mRNA reached maximum concentrations by metestrus (Days 1 to 6) and were down-regulated during diestrus (Days 7 to 16). Similarly, abundance of progesterone receptor mRNA in the subepithelial stroma was elevated on Days 0 to 6 of the estrous cycle before decreasing through Day 15, and abundance in the superficial glands was increased from Day 0 to Day 8 before decreasing through Day 15 (Robinson et al. Citation2001). Similarly, immunostaining for progesterone receptor in the uterine stroma showed similar intensity on Days 0 and 5 but then intensity decreased on Days 9 and 13 of the estrous cycle before increasing again on day 19 (Martin et al. Citation2008), indicating transcript abundance and protein abundance are associated.
Estradiol has been reported to have a direct impact on oviductal secretion of glycoproteins (Buhi Citation2002) and in the regulation of the uterine biological clock (Nakamura et al. Citation2005). This has been demonstrated through a number of transgenic mouse models. These models have implicated members of the circadian clock gene family in the proper regulation of mammalian reproductive function (Kennaway Citation2005; Boden and Kennaway Citation2006). Chappell et al. (Citation2003) reported that under-expression of the CLOCK gene resulted in mice that had prolonged estrous cycles and decreased litter size. Under-expression of Bmal1 resulted in prolonged estrous cycles and decreased progesterone secretion (Ratajczak et al. Citation2009). Mice that had either the Period 1 or Period 2 gene knocked out had very few reproductive abnormalities at a young age, but when they became older they had increased incidence of anestrus and some reduction in litter size compared to wild type controls (Pilorz and Steinlechner Citation2008). In the present study, expression of Period 1 was increased among estrus cows compared to non-estrus cows. From these changes in uterine transcript abundance along with data from other studies, it could be concluded that the circadian clock genes may influence reproductive function in ruminants through effects on the uterus and the estrous cycle. He et al. (Citation2007) reported effects of steroid treatments on uterine Period 1 mRNA levels in ovariectomized mice. Estradiol stimulated Period 1 mRNA transcription in the uterine stroma, luminal epithelium, glandular epithelium, and myometrium, while progesterone only stimulated Period 1 mRNA transcription in the uterine stroma, luminal epithelium, and glandular epithelium. Thus, it is clear that ovarian steroids influence Period 1 mRNA transcript abundance in the uterus; however, the implications for mammalian fertility are unclear.
Uterine milk protein (SERPINA14) is known to be produced in the endometrium of ruminants during pregnancy and is likely involved in nutrition of the embryo/fetus, growth of the embryo/fetus, and suppression of the maternal immune system (Ing and Roberts Citation1989). Transcript abundance in the bovine uterus as determined by Real-time RT-PCR was up-regulated after stimulation with estradiol (Ulbrich et al. Citation2009). In the present study, transcript abundance was increased on the day of estrus among estrus cows but did not change across days of the cycle among non-estrus cows. Similarly, detection of the protein revealed increased protein concentrations on the day of estrus and was localized to the glandular epithelium (Ulbrich et al. Citation2009). Furthermore, transcript abundance was reported to be the greatest on the day of estrus, then decreased during the mid-luteal phase, but was again increased on Day 18 in pregnant cows compared to controls (Ulbrich et al. Citation2009). Thus, the different profiles of uterine milk protein across the resultant estrous cycle estrus and non-estrus cows suggests that the timing of uterine events in the non-estrus animals may not be functioning properly to support proper embryo development and survival.
At estrus there is also an up-regulation of several genes involved in remodeling of the extracellular matrix (Bauersachs et al. Citation2005), and changes in transcript abundance of inhibin beta A subunit (a member of the TGF-beta signaling pathway) in the bovine intercaruncle area suggests it is involved in coordinating endometrial remodeling (Ishiwata et al. Citation2003). The TGF-beta superfamily is known to be involved in changes in the endometrium, development of the placenta, and maintenance of the pregnancy (Jones et al. Citation2006). In the present study, inhibin beta A subunit was up-regulated in estrus cows compared to non-estrus cows and expression was greater in estrus cows on Day 0 compared to non-estrus cows. Similarly, Bauersachs and co-workers (Citation2005) reported the inhibin beta A subunit was produced during estrus.
Proenkephalin-A is the precursor of several individual enkephalins (Marx Citation1983). Transcript abundance of proenkephalin-A has been reported to increase fourfold during pregnancy in the rat uterus (Jin et al. Citation1988), and was increased by exposure to progesterone and decreased by exposure to RU486 (Cheon et al. Citation2002). Similarly, Jin and co-workers (Citation1988) reported that expression in the rat uterus was greatest at metestrus and diestrus. In the current study, proenkephalin-A mRNA was not detected on Day 0 and was not different between treatments. However, expression increased from Day 5 to Day 10, then decreased to Day 16 of the cycle. This again supports the hypothesis that the uterus in the non-estrus animals may not be functioning properly to support proper embryo development and survival, even among proteins that are regulated by progesterone.
In summary, in the present study and in previous studies cows that exhibit standing estrus around the time of fixed-time insemination had increased preovulatory concentrations of estradiol (Perry et al. Citation2005, Citation2007; Perry and Perry Citation2008a, Citation2008b) and increased pregnancy success (Perry et al. Citation2005, Citation2007) compared to cows that did not exhibit standing estrus. Thus, preovulatory concentrations of estradiol (expression of estrus) played a major role in the establishment and maintenance of pregnancy. This is supported by the results of experiment 2, even though there were only minor change in transcript abundance of estradiol receptors and no significant changes in transcript abundance of progesterone receptor, preovulatory concentrations of estradiol impacted uterine proteins that are known to be regulated by estradiol and progesterone (). Work by Bridges (Citation2007) reported no change in steady-state mRNA for the nuclear progesterone receptor but decreased staining intensity for the progesterone receptor among cows with decreased preovulatory concentrations of estradiol compared to cows with greater concentrations of preovulatory estradiol. Therefore, preovulatory concentrations of estradiol may not only have a direct effect on the uterine environment through estradiol receptors but also through regulation of the progesterone receptor. Furthermore, the timing of changes in expression of uterine milk protein precursor, inhibin beta A, proenkephalin-A, and Period 1 indicate that preovulatory concentrations of estradiol can have a prolonged effect on the uterine function and environment. This can be seen in the present study with cows that exhibited estrus prior to fixed-time AI not only having greater pregnancies per AI, but the ones that did not to conceive to AI conceived earlier during the subsequent breeding season compared to cows that were induced to ovulate without exhibiting estrus. Thus, preovulatory estradiol concentrations may impact fertility not only directly by regulating the uterine environment but also indirectly by regulating response to progesterone through regulation of the progesterone receptor.
Figure 11. Changes in uterine transcription abundance between cows that had elevated concentrations of estradiol prior to GnRH-induced ovulation (estrus) and animal that did not (non-estrus) in relationship to the steroid profile of a normal estrous cycle. Solid arrows indicate P < 0.05 dashed arrows indicate P < 0.10. (nd = not detected; nsd = no significant difference).
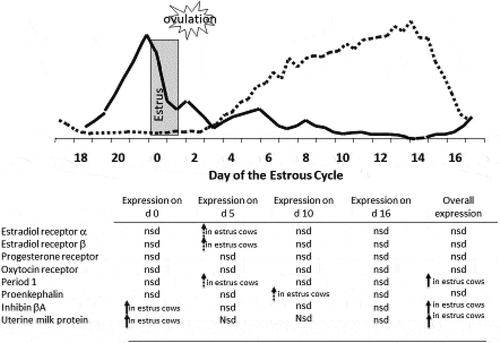
Materials and methods
All procedures were approved by the South Dakota State University Institutional Animal Care and Use Committee.
Experimental design – Experiment 1
Data were retrospectively collected from 4,499 animals (Bos taurus beef animals ages 13 months to 13 years; n = 3041 cows and 1458 heifers) in 31 different herds. All cows were postpartum lactating cows, and all heifers were virgin heifers (13 to 16 months of age at the start of the breeding season). Animals used in the study were mainly cross-bred animals consisting of the breeds of Angus, Hereford, Simmental, Charolais, Maine-Anjou, Red Angus, Limousin, and Gelbvieh. All animals in this analysis were synchronized using an injection of GnRH (100 µg as 2 mL of Factrel i.m.; Pfizer Animal Health, Madison, NJ) at insertion of an intravaginal progesterone device (Pfizer Animal Health, Madison, NJ) on day −9, an injection of prostaglandin F2α (PG; 25 mg as 5 mL of Lutalyse i.m.; Pfizer Animal Health, Madison, NJ) at device removal on day −2, and an injection of GnRH (100 µg of Factrel i.m.; Pfizer Animal Health, Madison, NJ) at time of AI on day 0. All studies had been conducted at South Dakota State University between 2004 and 2019. At time of CIDR removal, an estrus detection aid (EstroTect, Rockway Inc, Spring Valley, WI) was applied to each animal. Estrus response was recorded at time of AI based on the amount of the scratch-off coating that was removed. Animals with 50% or more of the coating removed were considered to be in estrus, and animals that were missing the estrus detection aid at time of insemination were removed from the analysis.
At each location one technician thawed all straws of semen and to minimize the effect of AI technician, each technician inseminated a similar number of animals in each group and used a similar number of straws from each sire used at each location. Pregnancies to AI were determined by a single transrectal ultrasonography using fetal size to determine fetal age that occurred between 28 and 72 days after AI. At each location, there was a minimum of 7 days between artificial insemination and introducing clean-up bulls to the herds to allow for accurate aging of the fetus by transrectal ultrasonography to determine AI sired pregnancies from bull-sired pregnancies. Final pregnancy status and day of conception was determined 30 to 45 days after the end of the breeding season by transrectal ultrasonography using fetal size (crown-rump length or head diameter) to determine fetal age. All animals were grouped as having conceived to AI, or into each of the possible return estrous cycles (cycle 1 – days 10 to 31, cycle 2 – days 32 to 53, cycle 3 – days 54 to 75, and cycle 4 – after day 75). Any animal in which a fetus was found during the first pregnancy determination but a fetus of the correct age was not present during the second pregnancy diagnosis was determined to have had embryonic loss. Similar to days to conception days postpartum was also grouped into 20-day intervals from calving to the day of AI, and animals were group according to their age/parity into virgin replacement heifers or cows (all animals that had calved and were lactating).
Experimental design – Experiment 2
Cycling Angus and Angus Cross beef cows (n = 60) at the South Dakota State University Beef Breeding Unit and Cow-Calf Unit were treated with 100 µg GnRH on day −9, 25 mg prostaglandin F2α on day −2, and 100 µg GnRH on day 0. Blood samples were collected on days 0, 5, 10, and 16 by venipuncture of the Jugular vein into 10 mL EDTA (K2) Vacutainer tubes (Becton, Dickinson, and Company) for determination of concentrations of estradiol and progesterone. Blood samples were immediately placed on ice. Samples were centrifuged at 1,200 x g for 30 min, and the plasma was harvested and frozen at −20° C until analyzed by Radioimmunoassay. Ovulatory follicle size was determined by transrectal ultrasonography at time of the second GnRH injection (day 0). Ovulation was confirmed by the disappearance of the ovulatory follicle 48 h after the GnRH injection. Animals that did not ovulate in response to the second GnRH injection, did not have a normal length luteal phase (>10 days), or exhibited estrus before d 0 of the estrous cycle were removed from the study (n = 28). Standing estrus was detected by visual observation with the aid of EstroTect estrus detection patches (Rockway, Inc., Spring Valley, WI). Animals that were detected in standing estrus were classified as estrus animals and animal that did not exhibit standing estrus were classified as non-estrus animals.
Radioimmunoassays
Radioimmunoassay (RIA) were performed on plasma samples to determine concentrations of progesterone using an in house RIA methods described by Engel et al. (Citation2008). All samples were run in two assays with internal controls of 0.8, 1.7, 3.2, 5.3, and 7.4 ng/mL. Inter- and intra-assay CV were 6.02% and 4.52%, respectively, and assay sensitivity was 0.4 ng/mL. In order to characterize circulating estradiol, a single in house RIA was performed using the methods described by Perry and Perry (Citation2008a). Internal controls of 4 and 8 pg/mL were included in the assay, and the intra-assay CV was 5.42% with an assay sensitivity was 0.5 pg/mL.
Tissue collection
Two samples of uterine endometrium were collected using a Jackson Uterine Biopsy instrument (Universal Surgical Instruments and Better Surgical Instrumentation) on day 0 (n = 10), 5 (n = 10), 10 (n = 12), or 16 (n = 10) as described by Bolzenius et al. (Citation2016). Briefly, the biopsy tool was closed and passed through the vagina and cervix. When the site of biopsy was reached (just prior to the curvature of the uterine horn ipsilateral to the corpus luteum) the jaws of the tool were then opened to collect the biopsy. The biopsy tool then remained closed until the samples were completely clear of the animal. These time points correspond to induced ovulation (day 0), 16 cell/early morula-stage (day 5), hatching (day 10), and maternal recognition of pregnancy (day 16). Cows were only biopsied on a single day except that cows biopsied on day 0 were also biopsied on day 16.
RNA isolation and quantitative real-time PCR
Total cellular RNA was extracted from bovine uterine tissue using TRI Reagent (Molecular Research Center, Inc.) as described by Bolzenious et al. (Citation2016). After purification, RNA concentrations were measured at 260 nm and 280 nm by spectrophotometry, and all RNA samples were diluted to 10ng/μl with RNase/DNase Free Water (MP Biomedicals), and concentration was determined by spectrophotometry. A single-step SYBR Green reaction was performed using the iScript One-step RT-PCR Kit with SYBR Green (Bio-Rad Laboratories, Inc.) and a Stratagene MX 3000P QPCR System. The SYBR Green reaction was performed for genes with the reverse transcription at 42°C for 30 min and 95°C for 10 min to inactivate reverse transcription. For all of the genes of interest, transcription was followed by 40 cycles of 30 sec at 95°C for melting; 1 min at the annealing temperatures (); and 30 sec at 72°C for extension. Primers () for oxytocin receptor and estradiol receptor alpha and beta were designed using Primer3 software (Rozen and Skaletsky Citation2000) or were previously published for GAPDH (Han et al. Citation2006), Period 1 (Cushman et al. Citation2007), progesterone receptor (Connor et al. Citation2005), and uterine milk protein precursor (UTMP), proenkephalin-A (PENK), and inhibin beta a (Bauersachs et al. Citation2005). Briefly, primers had amplification efficiencies of 1.02 to 1.45 and single-product melting curves. All amplicons were confirmed for product size on a 2% agarose gel and were verified for identity by sequencing (Iowa State University Genomics Core).
Table 1. Primer sequence, annealing temperature and reference for genes of interest.
Statistical analysis
All statistical analyses were conducted using SAS 9.2 (SAS Institute Inc., Cary, NC). For experiment 1, differences in Pregnancies to AI, pregnancies during the breeding season, and embryonic loss between animals that did and did not exhibit estrus prior to fixed-time AI were determined using the GLIMMIX procedure in SAS. The model included the influence of estrus expression, age of the animal, and their interaction. Data were analyzed using a binomial distribution and a logit link. Herd was included as a random variable, and thus the treatment by herd interaction was used as the error term. Cycle of the breeding season in which animal conceived were analyzed using the mixed procedure of SAS. Using the complete data set, the model included the influence of estrus expression, age of the animal, and their interaction. In an analysis of a subset of the data set, the model included influence of estrus expression, age of the animal, body condition score at the start of the breeding season, and/or days post calving (mature cows only). Herd was included as a random variable, and thus the treatment by herd interaction was used as the error term. Data are reported as least square means ± S.E.M., with significance declared at P < 0.05 and a tendency declared at 0.05 < P < 0.15. When the F statistic was significant (P < 0.05), mean separation was performed using the Tukey-Kramer unprotected test (Snedecor and Cochran Citation1989).
To determine the impact of estrus expression before fixed-time AI on the percentage of animals that conceived in each subsequent cycle, the percentage of animals that conceived during that cycle was calculated by dividing the number of animals that conceived during that cycles by the number of animals that were not pregnant at the beginning of that cycle. Differences in the percentage of animals that conceived during that cycle between animals that did and did not exhibit estrus prior to fixed-time AI were determined using the GLIMMIX procedure in SAS. The model included the influence of estrus expression, age of the animal, and their interaction.
For experiment 2, plasma concentrations of estradiol on the day of AI were analyzed as described by Larimore et al. (Citation2016) using the mixed procedure of SAS. Relative expression of uterine milk protein precursor, inhibin beta A, proenkephalin-A, Period 1, oxytocin receptor, progesterone receptor, and estradiol receptor alpha and beta were analyzed using the MIXED procedure of SAS with expression of GAPDH as a covariate. The model included the independent variables of treatment, day, and treatment by day. All interactions were compared using the Tukey-Kramer unprotected test. Data are reported as least square means ± S.E.M., with significance declared at P < 0.05 and a tendency declared at 0.05 < P < 0.15.
Author contributions
Each author has contributed to the research process. Conceived and designed the experiments: GP, RC. Performed the experiments: GP, RC, BP, AS, EN, JR, SP. Analyzed and interpreted data: GP, RC. Drafted the Manuscript and Figures: GP. Critically revised, and completed/corrected the manuscript, and approved the final version: GP, RC, BP, AS, EN, JR, SP.
Acknowledgments
Mention of trade names or commercial products in this publication is solely for the purpose of providing specific information and does not imply recommendation or endorsement by the U.S. Department of Agriculture or South Dakota State University. The U.S. Department of Agriculture (USDA) prohibits discrimination in all its programs and activities on the basis of race, color, national origin, age, disability, and where applicable, sex, marital status, familial status, parental status, religion, sexual orientation, genetic information, political beliefs, reprisal, or because all or part of an individual’s income is derived from any public assistance program (Not all prohibited bases apply to all programs.). Persons with disabilities who require alternative means for communication of program information (Braille, large print, audiotape, etc.) should contact USDA’s TARGET Center at (202) 720-2600 (voice and TDD). To file a complaint of discrimination, write to USDA, Director, Office of Civil Rights, 1400 Independence Avenue, S.W., Washington, D.C. 20250-9410, or call (800) 795-3272 (voice) or (202) 720-6382 (TDD). USDA is an equal opportunity provider and employer.
Disclosure statement
No potential conflict of interest was reported by the authors.
Additional information
Funding
References
- Allrich RD. 1994. Endocrine and neural control of estrus in dairy cows. J Dairy Sci. 77(9):2738–2744.
- Atkins JA, Smith MF, MacNeil MD, Jinks EM, Abreu FM, Alexander LJ, Geary TW. 2013. Pregnancy establishment and maintenance in cattle. J Anim Sci. 91(2):722–733.
- Bartol FF, Thatcher WW, Lewis GS, Bliss EL, Drost M, Bazer FW. 1981. Effect of estradiol-17beta on PGF and total protein content in bovine uterine flushings and peripheral plasma concentration of 13, 14-dihydro-15-keto-PGF(2alpha). Theriogenology. 15(4):345–358.
- Bauersachs S, Ulbrich SE, Gross K, Schmidt SE, Meyer HH, Einspanier R, Wenigerkind H, Vermehren M, Blum H, Sinowatz F, et al. 2005. Gene expression profiling of bovine endometrium during the oestrous cycle: detection of molecular pathways involved in functional changes. J Mol Endocrinol. 34(3):889–908.
- Boden MJ, Kennaway DJ. 2006. Circadian rhythms and reproduction. Reproduction. 132(3):379–392. eng.
- Bolzenius JK, Cushman RA, Perry GA. 2016. Expression of Na(+)/H(+) exchanger isoforms 1, 2, 3, and 4 in bovine endometrium and the influence of uterine pH at time of fixed-time AI of pregnancy success. Anim Reprod Sci. 171:98–107.
- Braden TD, King ME, Odde KG, Niswender GD. 1989. Development of preovulatory follicles expected to form short-lived corpora lutea in beef cows. J Reprod Fertil. 85(1):97–104.
- Brandenberger AW, Tee MK, Lee JY, Chao V, Jaffe RB. 1997. Tissue distribution of estrogen receptors alpha (ER-alpha) and beta (ER-beta) mRNA in the midgestational human fetus. J Clin Endocrinol Metab. 82(10):3509–3512. eng.
- Bridges GA. 2007. Impact of preovulatory estradiol concentrations on mechanisms affecting fertility in cattle. Columbus: The Ohio State University.
- Bridges GA, Ahola JK, Brauner C, Cruppe LH, Currin JC, Day ML, Gunn PJ, Jaeger JR, Lake SL, Lamb GC, et al. 2012a. Determination of the appropriate delivery of prostaglandin F2alpha in the five-day CO-Synch + controlled intravaginal drug release protocol in suckled beef cows. J Anim Sci. 90(13):4814–4822.
- Bridges GA, Mussard ML, Pate JL, Ott TL, Hansen TR, Day ML. 2012b. Impact of preovulatory estradiol concentrations on conceptus development and uterine gene expression. Anim Reprod Sci. 133(1–2):16–26.
- Buhi WC. 2002. Characterization and biological roles of oviduct-specific, oestrogen-dependent glycoprotein. Reproduction. 123(3):355–362. eng.
- Chappell PE, White RS, Mellon PL. 2003. Circadian gene expression regulates pulsatile gonadotropin-releasing hormone (GnRH) secretory patterns in the hypothalamic GnRH-secreting GT1-7 cell line. J Neurosci. 23(35):11202–11213. eng.
- Cheon YP, Li Q, Xu X, DeMayo FJ, Bagchi IC, Bagchi MK. 2002. A genomic approach to identify novel progesterone receptor regulated pathways in the uterus during implantation. Mol Endocrinol. 16(12):2853–2871. eng.
- Connor EE, Wood DL, Sonstegard TS, da Mota AF, Bennett GL, Williams JL, Capuco AV. 2005. Chromosomal mapping and quantitative analysis of estrogen-related receptor alpha-1, estrogen receptors alpha and beta and progesterone receptor in the bovine mammary gland. J Endocrinol. 185(3):593–603. eng.
- Cushman RA, Allan MF, Jones SA, Rupp GP, Echternkamp SE. 2007. Localization of Period 1 mRNA in the ruminant oocyte and investigations of its role in ovarian function. Anim Reprod Sci. 99(1–2):93–105. eng.
- Davoodi S, Cooke RF, Fernandes AC, Cappellozza BI, Vasconcelos JL, Cerri RL. 2016. Expression of estrus modifies the gene expression profile in reproductive tissues on Day 19 of gestation in beef cows. Theriogenology. 85(4):645–655.
- Engel CL, Patterson HH, Perry GA. 2008. Effect of dried corn distillers grains plus solubles compared with soybean hulls, in late gestation heifer diets, on animal and reproductive performance. J Anim Sci. 86(7):1697–1708.
- Garcia-Winder M, Lewis PE, Deaver DR, Smith VG, Lewis GS, Inskeep EK. 1986. Endocrine profiles associated with life span of induced corpora lutea in postpartum beef cows. J Anim Sci. 62(5):1353–1362.
- Garverick HA, Parfet JR, Lee CN, Copelin JP, Youngquist RS, Smith MF. 1988. Relationship of pre- and post-ovulatory gonadotropin concentrations to subnormal luteal function in postpartum beef cattle. J Anim Sci. 66(1):104–111.
- Geisert RD, Morgan GL, Short EC Jr., Zavy MT. 1992. Endocrine events associated with endometrial function and conceptus development in cattle. Reprod Fertil Dev. 4(3):301–305.
- Gray CA, Taylor KM, Ramsey WS, Hill JR, Bazer FW, Bartol FF, Spencer TE. 2001. Endometrial glands are required for preimplantation conceptus elongation and survival. Biol Reprod. 64(6):1608–1613.
- Han H, Austin KJ, Rempel LA, Hansen TR. 2006. Low blood ISG15 mRNA and progesterone levels are predictive of non-pregnant dairy cows. J Endocrinol. 191(2):505–512. eng.
- Hasler JF. 2001. Factors affecting frozen and fresh embryo transfer pregnancy rates in cattle. Theriogenology. 56(9):1401–1415. eng.
- He PJ, Hirata M, Yamauchi N, Hattori MA. 2007. Up-regulation of Per1 expression by estradiol and progesterone in the rat uterus. J Endocrinol. 194(3):511–519. eng.
- Hill SL, Perry GA, Mercadante VR, Lamb GC, Jaeger JR, Olson KC, Stevenson JS. 2014. Altered progesterone concentrations by hormonal manipulations before a fixed-time artificial insemination CO-Synch + CIDR program in suckled beef cows. Theriogenology. 82(1):104–113.
- Ing NH, Roberts RM. 1989. The major progesterone-modulated proteins secreted into the sheep uterus are members of the serpin superfamily of serine protease inhibitors. J Biol Chem. 264(6):3372–3379. eng.
- Ishiwata H, Katsuma S, Kizaki K, Patel OV, Nakano H, Takahashi T, Imai K, Hirasawa A, Shiojima S, Ikawa H, et al. 2003. Characterization of gene expression profiles in early bovine pregnancy using a custom cDNA microarray. Mol Reprod Dev. 65(1):9–18. eng.
- Jin DF, Muffly KE, Okulicz WC, Kilpatrick DL. 1988. Estrous cycle- and pregnancy-related differences in expression of the proenkephalin and proopiomelanocortin genes in the ovary and uterus. Endocrinology. 122(4):1466–1471. eng.
- Jinks EM, Smith MF, Atkins JA, Pohler KG, Perry GA, Macneil MD, Roberts AJ, Waterman RC, Alexander LJ, Geary TW. 2013. Preovulatory estradiol and the establishment and maintenance of pregnancy in suckled beef cows. J Anim Sci. 91(3):1176–1185.
- Jones RL, Stoikos C, Findlay JK, Salamonsen LA. 2006. TGF-beta superfamily expression and actions in the endometrium and placenta. Reproduction. 132(2):217–232. eng.
- Kennaway DJ. 2005. The role of circadian rhythmicity in reproduction. Hum Reprod Update. 11(1):91–101.
- Kieborz-Loos KR, Garverick HA, Keisler DH, Hamilton SA, Salfen BE, Youngquist RS, Smith MF. 2003. Oxytocin-induced secretion of prostaglandin F2alpha in postpartum beef cows: effects of progesterone and estradiol-17beta treatment. J Anim Sci. 81(7):1830–1836.
- Kimmins S, MacLaren LA. 2001. Oestrous cycle and pregnancy effects on the distribution of oestrogen and progesterone receptors in bovine endometrium. Placenta. 22(8–9):742–748. eng.
- Kuiper GG, Carlsson B, Grandien K, Enmark E, Haggblad J, Nilsson S, Gustafsson JA. 1997. Comparison of the ligand binding specificity and transcript tissue distribution of estrogen receptors alpha and beta. Endocrinology. 138(3):863–870. eng.
- Kuiper GG, Enmark E, Pelto-Huikko M, Nilsson S, Gustafsson JA. 1996. Cloning of a novel receptor expressed in rat prostate and ovary. Proc Natl Acad Sci US A. 93(12):5925–5930. eng.
- Larimore EL, Amundson OL, Bridges GA, McNeel AK, Cushman RA, Perry GA. 2016. Changes in ovarian function associated with circulating concentrations of estradiol before a GnRH-induced ovulation in beef cows. Domest Anim Endocrinol. 57:71–79.
- Martin I, Torres Neto R, Oba E, Buratini J Jr, Binelli M, Laufer-Amorim R, Ferreira JC. 2008. Immunohistochemical detection of receptors for oestradiol and progesterone in endometrial glands and stroma during the oestrous cycle in nelor (Bos Taurus indicus) cows. Reprod Dom Anim. 43:415–421.
- Marx JL. 1983. Synthesizing the opioid peptides. Science. 220(4595):395–397. eng.
- McCracken JA, Custer EE, Lamsa JC. 1999. Luteolysis: a neuroendocrine-mediated event. Physiol Rev. 79(2):263–323. eng.
- Nakamura TJ, Moriya T, Inoue S, Shimazoe T, Watanabe S, Ebihara S, Shinohara K. 2005. Estrogen differentially regulates expression of Per1 and Per2 genes between central and peripheral clocks and between reproductive and nonreproductive tissues in female rats. J Neurosci Res. 82(5):622–630. eng.
- Ozturk S, Demir R. 2010. Particular functions of estrogen and progesterone in establishment of uterine receptivity and embryo implantation. Histol Histopathol. 25(9):1215–1228. eng.
- Perry GA, Perry BL. 2008a. Effect of preovulatory concentrations of estradiol and initiation of standing estrus on uterine pH in beef cows. Domest Anim Endocrinol. 34(3):333–338.
- Perry GA, Perry BL. 2008b. Effects of standing estrus and supplemental estradiol on changes in uterine pH during a fixed-time AI protocol. J Anim Sci. 86:2928–2935.
- Perry GA, Smith MF, Lucy MC, Green JA, Parks TE, Macneil MD, Roberts AJ, Geary TW. 2005. Relationship between follicle size at insemination and pregnancy success. Proc Natl Acad Sci US A. 102(14):5268–5273.
- Perry GA, Smith MF, Roberts AJ, MacNeil MD, Geary TW. 2007. Relationship between size of ovulatory follicle and pregnancy success in beef heifers. J Anim Sci. 85:684–689.
- Pilorz V, Steinlechner S. 2008. Low reproductive success in Per1 and Per2 mutant mouse females due to accelerated ageing? Reproduction. 135(4):559–568. eng.
- Ratajczak CK, Boehle KL, Muglia LJ. 2009. Impaired steroidogenesis and implantation failure in Bmal1-/- mice. Endocrinology. 150(4):1879–1885. eng.
- Richardson BN, Hill SL, Stevenson JS, Djira GD, Perry GA. 2016. Expression of estrus before fixed-time AI affects conception rates and factors that impact expression of estrus and the repeatability of expression of estrus in sequential breeding seasons. Anim Reprod Sci. 166:133–140.
- Robinson RS, Mann GE, Lamming GE, Wathes DC. 2001. Expression of oxytocin, oestrogen and progesterone receptors in uterine biopsy samples throughout the oestrous cycle and early pregnancy in cows. Reproduction. 122(6):965–979.
- Rozen S, Skaletsky H. 2000. Primer3 on the WWW for general users and for biologist programmers. Methods Mol Biol. 132:365–386. eng.
- Ryan M, Mihm M, Roche JF. 1998. Effect of GnRH given before or after dominance on gonadotrophin response and fate of that follicle wave in postpartum dairy cows. J Reprod Fertil. 21:61.
- Saunders PTK. 1998. Oestradiol receptor beta. Rev Reprod. 3:164–171.
- Sheffel CE, Pratt BR, Ferrell WL, Inskeep EK. 1982. Induced corpora lutea in the postpartum beef cow. II. Effects of treatment with progestogen and gonadotropins. J Anim Sci. 54(4):830–836.
- Snedecor GW, Cochran WG. 1989. Statistical methods. 8th ed. Ames (Iowa): Iowa State University Press.
- Spencer TE, Johnson GA, Burghardt RC, Bazer FW. 2004. Progesterone and placental hormone actions on the uterus: insights from domestic animals. Biol Reprod. 71(1):2–10.
- Ulbrich SE, Frohlich T, Schulke K, Englberger E, Waldschmitt N, Arnold GJ, Reichenbach HD, Reichenbach M, Wolf E, Meyer HH, et al. 2009. Evidence for estrogen-dependent uterine serpin (SERPINA14) expression during estrus in the bovine endometrial glandular epithelium and lumen. Biol Reprod. 81(4):795–805. eng.
- Whittier WD, Currin JF, Schramm H, Holland S, Kasimanickam RK. 2013. Fertility in Angus cross beef cows following 5-day CO-Synch + CIDR or 7-day CO-Synch + CIDR estrus synchronization and timed artificial insemination. Theriogenology. 80:963–969.
- Zelinski MB, Hirota NA, Keenan EJ, Stormshak F. 1980. Influence of exogenous estradiol-17 beta on endometrial progesterone and estrogen receptors during the luteal phase of the ovine estrous cycle. Biol Reprod. 23(4):743–751.
- Zollers WG Jr., Garverick HA, Smith MF, Moffatt RJ, Salfen BE, Youngquist RS. 1993. Concentrations of progesterone and oxytocin receptors in endometrium of postpartum cows expected to have a short or normal oestrous cycle. J Reprod Fertil. 97(2):329–337.