ABSTRACT
In recent years, resveratrol has been shown to protect against metabolic damage, including obesity-associated subfertility/infertility. In the present study, proteomic alterations in testicular tissues were investigated by tandem mass tag (TMT) in mice fed with a high-fat diet (HFD) without or with resveratrol supplementation (HFD+RSV). Serum testosterone levels, spermatozoa parameters and testicular histological morphology were assessed. Resveratrol treatment was shown to significantly reduce serum cholesterol, prevent the HFD-induced reductions in serum testosterone and spermatozoa parameters, and decrease the ultrastructural degeneration of testicular tissues. The comparative proteomics analysis revealed 58 differentially expressed proteins between the HFD and control groups and 38 differentially expressed proteins between the HFD and HFD+RSV groups. Gene ontology (GO) term enrichment and Kyoto Encyclopedia of Genes and Genomes (KEGG) pathway analysis revealed that the most highly enriched differential proteins were correlated to spermatozoa function and cholesterol metabolism. The real-time RT-PCR and western blotting results confirmed the differential expression of the corresponding proteins related to spermatozoa function that were identified by proteomics. The present study provides new insight into the mechanisms of the beneficial effects of resveratrol, and may present it as a potential therapeutic strategy for obesity-associated male subfertility/infertility.
Abbreviations:TMT: Tandem mass tag; HFD: High-fat diet; RSV: Resveratrol; GO: Gene ontology; Protein–proteinKEGG: Kyoto Encyclopedia of Genes and Genomes; RT-PCR: Reverse transcription-polymerase chain reaction; SDS-PAGE: Sodium dodecyl sulfate-polyacrylamide gel electrophoresis; PVDF: Polyvinylidene fluoride; ECL: Enhanced chemiluminescence; RIPA: Radio-immunoprecipitation assay; CTRL: Control; PPI: interaction; RIA: Radioimmunoassay; T: Testosterone; TG: Triglycerides; TC: Total cholesterol; LDL-c: Low-density lipoprotein cholesterol; HDL-c: High-density lipoprotein cholesterol; Crisp1: Cysteine-rich secretory protein 1; SIRT1: Sirtuin 1; GPx5: Glutathione peroxidase 5; Svs4: Seminal vesicle secretory protein 4; Tssk3: Testis-specific serine kinase 3; Pate4: Prostate and testis expressed 4; Sva: Seminal vesicle antigen; Lcn5: Lipocalin 5; Spinkl: Serine protease inhibitor, Kazal type-like
KEYWORDS:
Introduction
Obesity is associated with various health problems, and significantly increases the risk for diabetes, dyslipidemia and hypertension. In recent years, the fact that diet-induced obesity has detrimental effects on male fertility has gained attention. Clinical data has shown that obese men or those with metabolic syndrome have significantly reduced spermatozoa parameters and sex hormone levels (Du Plessis et al. Citation2010; Macdonald et al. Citation2013). Furthermore, impaired steroidogenesis and spermatogenesis can be caused by diet-induced obesity in animals (Ghanayem et al. Citation2010; Borges et al. Citation2017). Emerging data has indicated that obesity not only reduces spermatozoa quality but also causes molecular structural changes in spermatogenic cells, ultimately affecting the function of spermatozoa and fertility (Shukla et al. Citation2014).
A red wine polyphenol naturally found in grapes, resveratrol is regarded to possess potential therapeutic values for obesity-associated metabolic diseases, and various pharmacologic properties with multiorgan effects. Treatment with resveratrol affects energy metabolism, and shows protection by reducing obesity-associated metabolic damage with promising therapeutic value (Timmers et al. Citation2011; Zheng et al. Citation2012; Springer and Moco Citation2019). Furthermore, resveratrol participates in the complex regulation of the mTORC1 pathway and autophagy (Alayev et al. Citation2015). In addition to the systemic health benefits regarding metabolic syndrome, resveratrol supplementation in diabetic mice improves fertility by attenuating testicular oxidative damage (Zhao et al. Citation2018), and inhibiting the testicular damage caused by chloride or iron/ascorbate (Mojica-Villegas et al. Citation2014; Archana et al. Citation2018). Furthermore, the antiperoxidative effects of resveratrol have been shown to account for its protection of steroidogenesis and spermatozoa quality. However, in a fertilization test in vitro, the coadministration of resveratrol promoted boar spermatozoa function without the involvement of antioxidant defensive mechanisms (Bucci et al. Citation2018). Notably, resveratrol can activate sirtuin 1 (SIRT1), which also plays important roles in gamete function and reproduction, with a therapeutic potential for infertility (Tatone et al. Citation2018). At present, the impact of resveratrol on proteins related to spermatozoa function in obesity-associated subfertility/infertility remains unclear.
In a previous study, resveratrol was investigated to inhibit the decline of Leydig cell steroidogenesis and attenuate the inflammation in epididymal adipose tissues of diet-induced obese mice (Wang et al. Citation2015a). The present study was designed to explore the testicular protein changes associated with steroidogenesis and spermatogenesis in mice fed a high-fat diet (HFD), with or without resveratrol treatment. Tandem mass tag (TMT) technology was used to assess the proteomic alterations in testicular tissues. Proteomics has the potential to provide novel information to be employed as a high-throughput analysis, and proteomics techniques have been utilized to explore the mechanisms by which lifestyle and environmental factors influence fertility with greater coverage of the proteome (Huang et al. Citation2016; Li et al. Citation2016; Xie et al. Citation2018). These present findings provide new data on the therapeutic value of resveratrol in obesity-associated subfertility/infertility, and promote the development of new potential biomarkers for obesity-associated metabolic damage.
Results and discussion
Body weight, serum lipids, and testosterone
Unfavorable diets with excess fat lead to an overweight state and obesity-associated diseases, which are associated with male infertility. Although the contributions of low spermatozoa quality and decreased sex hormone levels to obesity-associated infertility/subfertility have been widely acknowledged, the molecular mechanisms remain to be determined. In the present study, the body weight, serum lipids and testosterone in each group was first evaluated. It was found that there was a significant increase in body weight in the HFD group, when compared to the CTRL group. However, the body weight increased much less in the HFD+RSV group. Furthermore, a significant difference occurred in body weights between the HFD and HFD+RSV groups. Serum TC, LDL-c and HDL-c, but not TG, significantly increased in the HFD group, when compared to the CTRL group. Resveratrol treatment significantly reduced the serum cholesterol levels. Furthermore, serum testosterone also declined in the HFD group, and was improved in the HFD+ RSV group ().
Table 1. Effects of high-fat diet with (HFD+RSV) or without (HFD) resveratrol treatment on mice. Control mice without resveratrol (CTRL) or with resveratrol (CTRL+RSV) (means ± SEM).
Spermatozoa parameters
Resveratrol has been widely studied due to its potential health benefits. Therefore, the impact of resveratrol on spermatozoa parameters was also investigated. As shown in , the HFD caused a significant increase in abnormal spermatozoa and reductions in spermatozoa count and motility, when compared to the CTRL group. The resveratrol treatment significantly prevented the HFD-induced reductions in spermatozoa parameters and improved spermatozoa quality (). However, the CTRL+RSV group did not exhibit a significant difference in body weight, serum lipids, testosterone, or spermatozoa parameters, when compared with the CTRL group (). Therefore, the following parameters were not assessed for the CTRL+RSV group.
Testicular morphological structure
Obesity causes systematic metabolic changes, and clinical data have showed that males subfertiliy/infertiliy and obesity are closely correlated (Du Plessis et al. Citation2010; Macdonald et al. Citation2013). Several mechanisms are considered to be involved in the detrimental effects of obesity on male fertility, including hormone dysregulation and oxidative damage (Liu and Ding Citation2017). Resveratrol supplementation may promote testicular antioxidant status and inhibited testicular apoptosis in diabetic mouse model (Zhao et al. Citation2018). It is necessary to investigate the impact of HFD on the testicular tissues and protective effects conferred by resveratrol. Seminiferous tubules were intact in mice of each group (). Compared with control, the mean seminiferous tubule diameter in HFD group did not show significant differences. Disorganization of the series of spermatogenic cells could be found in the HFD group. The significant decrease of Johnsen’s score was identified in the HFD group compared with the CTRL group, while it was significantly improved in HFD+RSV group. There was no significant difference between CTRL and CTRL+RSV ().
Table 2. Morphometric evaluation and Johnsen’s score of seminiferous tubules in testis of mice fed high-fat diet (HFD), high-fat diet with additional resveratrol (HFD+RSV), and control mice without (CTRL) or with resveratrol (CTRL+RSV) (means±SEM).
Figure 1. The representive light microscopic images of the testicular tissues. Magnification: ×200. Bar = 50μm. The testicular tissues collected from the CTRL group, CTRL+RSV group, HFD group, and HFD+RSV group, were cut into 5 μm thick slice and stained by HE (hematoxylin-eosin) staining method. The disorganization in spermatogenic cells of seminiferous tubules in HFD group was shown. The testicular structure of CTRL+RSV group showed no alterations compared to CTRL group.
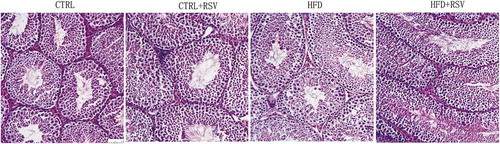
Ultrastructural degeneration occurred in the testicular tissues of HFD-fed mice, as evidenced by swelling, vacuolar mitochondria and dilated endoplasmic reticulum in spermatogenic cells, Sertoli cells and Leydig cells. The thickened basement membranes of seminiferous tubules were also identified in HFD-fed mice. Resveratrol treatment attenuated the HFD-induced ultrastructural damage to testicular tissues ().
Figure 2. The representive ultrastructures of the testicular tissues were presented. The testicular tissues collected from the CTRL group, HFD group, and HFD+RSV group, were cut into ultrathin slice and observed by transmission electron microscopy. The degeneration could be significantly observed in spermatogenic epithelia of seminiferous tubles in the HFD group, which was improved by the resveratrol treatment. The arrows indicate the mitochondria in spermatogenic cells.
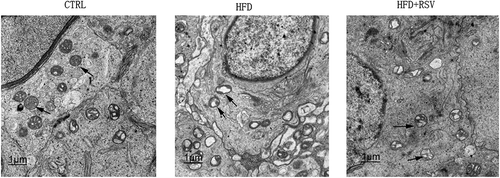
Testicular proteins differentially expressed in response to HFD and resveratrol
A total of 7,241 proteins were identified in mice testicular tissues in the present study. The differentially expressed proteins (P < 0.05) were designated at a fold change cutoff ratio of >1.2 (upregulated) or <0.83 (downregulated). The comparative TMT proteomics revealed 58 differentially expressed proteins between the HFD and CTRL groups. Among these proteins, 39 proteins were downregulated, while 19 proteins were upregulated (). By comparing the HFD and HFD+RSV groups, 38 differentially expressed proteins were identified, among which 15 differentially expressed proteins were downregulated and 23 differentially expressed proteins were upregulated (). A number of important proteins related to spermatozoa function, such as Crisp1, Svs3a, Pate4, Svs4, Svs5, Spinkl and Tssk3, were downregulated in the HFD group, and upregulated in the HFD+RSV group.
Table 3. Differential testicular proteins expressions in mice fed with a high-fat diet (H) compared with normal control (C).
Table 4. Differential testicular proteins expressions in mice fed with a high-fat diet with resveratrol treatment (R) compared with those without treatment (H).
GO analysis
GO analysis was performed for the designated differential proteins among groups above. The GO function classification of these proteins was based on the biological process, cellular component and molecular function. The top 20 generally changed GO terms were compared. In comparing the HFD and CTRL groups, the most significantly enriched biological process terms were associated with sperm capacitation, sperm motility, lipoprotein particle remodeling, cholesterol esterification and steroid esterification. With respect to molecular function, the most significantly enriched terms included structural molecule activity, cholesterol transporter activity and sterol transporter activity. For the cellular component, the most enriched terms were correlated with intermediate filament, cytoskeleton, keratin, extracellular region and extracellular space ().
Figure 3. The GO (gene ontology) function classification of the differential protein identified by the TMT-based proteomics. The GO enrichment analysis of proteins from the testis samples was performed according to the BP (biological process), MF (molecular function) and CC (cellular component). The comparison between the (A) HFD group and CTRL group, and between the (B) HFD group and HFD+RSV group was performed.
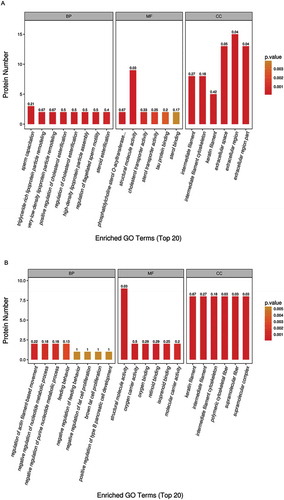
In comparing the HFD+RSV and HFD groups, the most enriched biological process terms were associated with the regulation of actin filament-based movement and the negative regulation of nucleotide metabolism. The representative terms related to molecular function were structural molecule activity, oxygen carrier and binding activity, and retinoid binding. For the cellular component, the most enriched terms were keratin filament, intermediate filament and cytoskeleton ().
KEGG analysis
In the KEGG analysis, a number of pathways were significantly enriched in the HFD group, when compared to the CTRL group, which included some classical pathways, such as the mTOR, cAMP and Hedgehog signaling pathways. The estrogen signaling pathway and cholesterol metabolism were the top two listed pathways ().
Figure 4. The pathway analysis of all differentially expressed proteins was based on the KEGG (Kyoto Encyclopedia of Genes and Genomes) database. The comparison between the (A) HFD group and CTRL group, and between the (B) HFD group and HFD+RSV group was performed.
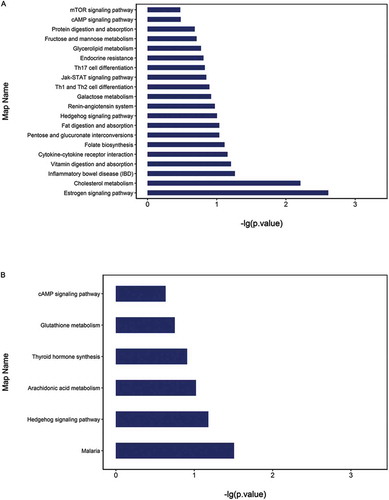
In comparing the HFD+RSV and HFD groups, the cAMP signaling pathway, the Hedgehog signaling pathway and glutathione metabolism were significantly enriched according to the differential proteins. The malaria pathway consisted of proteins associated with oxygen carriers ().
The GO and KEGG pathway enrichment analyses revealed that HFD also influenced cholesterol metabolism in addition to spermatozoa function. These present results were consistent with the findings of most studies that revealed the therapeutic values of resveratrol in hypercholesterolemia and atherosclerosis (Juhasz et al. Citation2011; Chen et al. Citation2012). Normal lipid metabolism is essential for both normal spermatogenesis and steroidogenesis (Sèdes et al. Citation2018). Disorders of cholesterol metabolism induced by HFD may negatively impact the structural integrity of the spermatozoa membrane and steroid metabolism, contributing to the decline in fertility in obese individuals. Therefore, the regulation of lipid metabolism by resveratrol may play an important role in protecting against HFD-induced defects in spermatogenesis and steroidogenesis. However, the number of proteins differentially regulated by resveratrol in the present study was small, and this was perhaps because other alterations, such as protein modifications, were involved. Furthermore, the effect of resveratrol on protein phosphorylation or acetylation was not evaluated, although these modifications have been reported as potential molecular mechanisms of resveratrol (Alayev et al. Citation2014). Another previous study reported that changes in epigenetic modifiers occurred, and the molecular profiles of germ cells were altered in the testes and spermatozoa obtained from obese males (Palmer et al. Citation2012). The exact mechanisms and roles of protein modifications in mediating the effect of resveratrol on fertility needs to be further explored.
Protein–protein interaction (PPI) analysis
In order to investigate the interactions between differentially expressed proteins in the HFD group, when compared with the CTRL group, a PPI network was established based on differentially expressed proteins. The proteins involved in spermatozoa function comprised an interaction network, and these were all downregulated, except for Prm2 (). A PPI network based on differentially expressed proteins in the HFD+RSV and HFD groups was established. These proteins, which were correlated to spermatozoa function and glutathione metabolism, and included in the interaction network, were all upregulated ().
Figure 5. A PPI (protein–protein interaction) network based on differentially expressed proteins was established using the Cytoscape software. The comparison between the (A) HFD group and CTRL group, and between the (B) HFD group and HFD+RSV group was performed. The proteins were presented as nodes: red nodes represent the upregulated proteins, and green nodes represent the downregulated proteins.
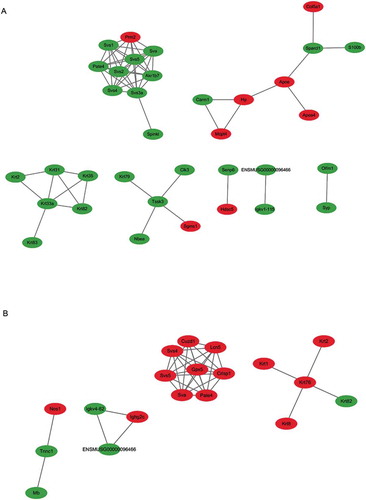
The protein network based on those differentially expressed spermatozoa proteins revealed a complex interaction with glutathione peroxidase 5 (GPx5). The expression of these spermatozoa proteins was significantly improved by resveratrol treatment, when compared with HFD alone. GPx5 has been shown to protect extremely fragile spermatozoa cells from peroxidative injury during post-testicular maturation in the epididymis and from the detrimental effects of diabetes (Taylor et al. Citation2013). GPxs are utilized to inactivate reactive oxygen species in spermatozoa, because rich polyunsaturated fatty acids usually make mammalian spermatozoa more susceptible to lipid peroxidation (Taylor et al. Citation2013). These present results indicate that the stimulation of glutathione antioxidants contributed to the ability of resveratrol to protect spermatocytes from HFD-induced lipid peroxidation.
mTOR is a key regulator of various fundamental cellular functions. It regulates metabolism, cell growth, proliferation, protein and lipid synthesis. mTOR signaling is responsive to nutrient and growth factors, and regulate cell survival and autophagy (Alayev et al. Citation2015). The mTOR pathway appeared to be positively activated in the testes of HFD mice, as shown by the upregulation of WDR59, which is a component of the GATOR2 complex (Peng et al. Citation2017). WDR59 was downregulated in the HFD+RSV group, when compared to the HFD group. Accumulating evidence has implicated resveratrol in complex mechanisms in the mTOR pathway and autophagy. Unlike other tissues, testicular tissues, especially Leydig cells, have fairly active autophagy (Yi and Tang Citation1995; Gao et al. Citation2018). The dysregulation of autophagy may also affect testicular function under conditions of HFD-induced obesity (Mu et al. Citation2017).
Expression of the identified proteins
In order to verify the differential protein expression levels, real-time RT-PCR was performed to assess the mRNA levels of five differentially expressed genes correlated to spermatozoa function (Crisp1, Pate4, Tssk3, Svs4 and Lcn5) (). Western blotting was further performed to verify the protein expression levels of CRISP1 and PATE4. The differential protein expressions of PATE4 in each group were in accordance with the differential mRNA levels. The protein level of CRISP1 was significantly decreased in HFD group, but the difference of mRNA levels was not statistically significant between HFD and CTRL group ().
Figure 6. Five differentially expressed genes related to spermatozoa function (Crisp1, Pate4, Tssk3, Svs4, and Lcn5) were verified by quantitative RT-PCR. The mRNA expression levels of these genes were normalized to GAPDH levels, as measured by real-time RT-PCR in the CTRL, HFD and HFD+RSV groups. Data were presented as mean ± standard error of the mean (SEM). *P < 0.05 vs. CTRL, #P < 0.05 vs. HFD.
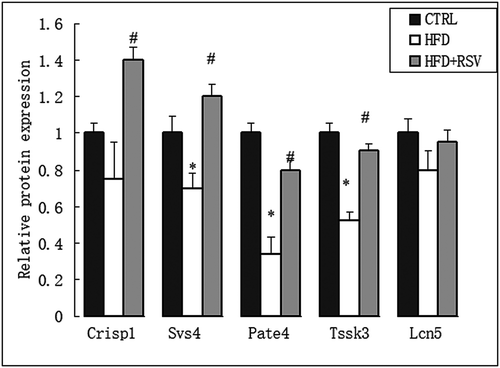
Figure 7. Spermatozoa function-related proteins were detected by Western blot. Representative images of the CTRSP 1, PATE 4 expression in the CTRL, HFD and HFD+RSV groups. The quantification of protein expression levels were presented with normalized to GAPDH levels in right panel. Data were presented as mean ± standard error of the mean (SEM). *P < 0.05 vs. CTRL, #P < 0.05 vs. HFD.
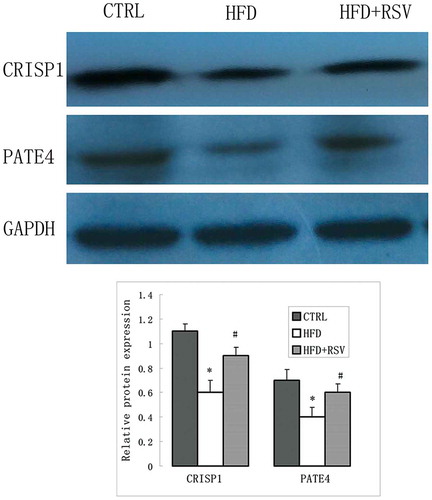
A number of proteins correlated to spermatozoa function were upregulated by resveratrol treatment. Interestingly, CRISP1 was the most significantly upregulated gene by resveratrol treatment. CRISP1 is a multifunctional protein that mediates spermatozoa binding to the zona pellucida, and regulates capacitation (Cohen et al. Citation2011; Hu et al. Citation2018). A recent report indicated that the disruption of epididymal CRISP4 function accounted for the decline in spermatozoa motility and fertilization capacity associated with obesity. Kockout mice revealed that epididymal CRISPs were involved in the spermatozoa function, and contributed to the immune-privileged environment for spermatozoa maturation (Borges et al. Citation2017). CRISP1 has been regarded as a major epididymal protein. These present results revealed that this protein plays vital roles in the testis, and can be upregulated by resveratrol. The Tssks family is an important spermatozoa function protein, and most of the Tssk members only expressed in the testis except Tssk6 (Wang et al. Citation2015b). Tssk4 knockout resulted in serious defects in spermatozoa motility, because it is required for the structural integrity of spermatozoa flagellum (Wang et al. Citation2015c). The Svs family plays important roles in regulating spermatozoa capacitation (Araki et al. Citation2016). Pate4, another name Svs7, is necessary for fertility mainly due to its involvement in copulatory plug formation (Noda et al. Citation2018). The upregulation of these spermatozoa proteins by resveratrol treatment further confirms its beneficial effect on sperm quality and fertility.
In conclusion, the differential expression profiles of testicular proteins in HFD mice with/without resveratrol treatment was investigated by proteomics analysis. The most highly enriched differential proteins were associated with reproductive processes and cholesterol metabolism. Resveratrol treatment attenuated the deleterious effects on testicular structure and function induced by HFD, and significantly improved spermatozoa quality. These present results shed new insights into the mechanisms underlying obesity-associated male subfertility/infertility, and the therapeutic potential of resveratrol.
Materials and methods
Animal experiments
The C57BL/6J male mice (eight weeks old) were purchased from the Model Animal Research Center of Nanjing University. A total of 24 male C57BL/6J mice were randomly divided into two groups: HFD group (n = 12), fed with HFD alone (0.5% cholesterol, 10% lard, 10% yolk powder, 59.5% maintenance materials and 20% cane sugar; BiotechHD Co. Ltd., Beijing, China); HFD+RSV group (n = 12), HFD combined with intragastric resveratrol treatment (400 mg/kg/day; Nanjing Zelang Medical Technology, Nanjing, PRC) (Lagouge et al. Citation2006). Carboxymethylcellulose (10 g/L) was used to dissolve the resveratrol. C57BL/6J mice on a standard diet served as controls: CTRL group (n = 12), without resveratrol; CTRL+RSV group (n = 8), with resveratrol. The standard diet was identical to the maintenance materials in the HFD. All animal procedures were approved by the local Institute Animal Ethics Committee, and were under the control of the Committee on the Control and Supervision of Experiments on Animals.
At the end of the experiment, which was after 18 weeks, each mouse was weighed and sacrificed, and the testis and epididymal tissues were dissected. Then, the blood samples were collected, and the serum levels of testosterone were detected using a radioimmunoassay (RIA) kit (Beijing North Institute of Biological Technology, Beijing, China). Triglycerides (TG), total cholesterol (TC), low-density lipoprotein cholesterol (LDL-c) and high-density lipoprotein cholesterol (HDL-c) were quantified using enzymatic colorimetric methods on an Olympus AU640 automated analyzer.
Assessment of spermatozoa motility and morphology
One cauda epididymis from each animal was used to collect the spermatozoa, and these were placed in M199 medium, cut into pieces, and incubated for 30 minutes in a 5% CO2/air incubator at 37°C to release spermatozoa. The spermatozoa suspension was used for the subsequent assessment of spermatozoa parameters. Spermatozoa motility was assessed using an ocular reticle on an Olympus light microscope (BX51, Japan) at 400× magnification. At least 200 spermatozoa were counted in each spermatozoa sample. The spermatozoa motility data were presented as the number of progressive motile spermatozoa per the total number of spermatozoa within the grid.
For the assessment of spermatozoa morphology, 10 μl of the spermatozoa suspension was pipetted onto a slide, air-dried, fixed in acetone, and stained by the modified Papanicolaou staining method using Orange G-6, Mayer’s hematoxylin solution and EA-50. At least 200 spermatozoa were observed under an Olympus light microscope (BX51, Japan) at 1,000× magnification (oil objective), and evaluated for morphology.
Hematoxylin-eosin (HE) staining and histological analysis
hemotoxylinTesticular samples were processed routinely by paraffin techniques, and 5μm thick sections were cut and stained with and eosin dye. Seminiferous tubules were observed with Nikon Eclipse 80i light microscope (Nikon, Tokyo, Japan). Their diameters and the height of the spernatogenic epithelia were measured under low magnification with software IPP6.0 (Media Cybernetics, Silver Spring, MD, USA). The 10 circular tubules were randomly identified for statistics for each section of the testis. Spermatogenesis was evaluated with Johnsen’s score (Guneli et al. Citation2008). Each tubule cross section was graded according to the following criteria: 10 = complete spermatogenesis and perfect tubules; 9 = many spermatozoa present and disorganized spermatogenesis; 8 = only a few spermatozoa present; 7 = no spermatozoa but many spermatids present; 6 = only a few spermatids present; 5 = no spermatozoa or spermatids but many spermatocytes present; 4 = only a few spermatocytes present; 3 = only spermatogonia present; 2 = no germ cells but only Sertoli cells present; 1 = no germ cells and no Sertoli cells present.
Transmission electron microscopy
The procedures for the electron microscopy were performed in a routine manner. First, small blocks of approximately 1 mm3 were cut from the testicular tissues, and fixed in 2.5% glutaral solution. Then, these were embedded in araldite. After ultrathin sections were obtained, these were stained with uranyl acetate and lead citrate. Then, the fine structures of testicular tissues were evaluated with a Zeiss EM 900 transmission electron microscope (Zeiss, Oberkochen, Germany).
TMT-based proteomics
The testicular tissues obtained from each group were frozen in liquid nitrogen and routinely processed for TMT proteomics analysis (Shanghai Applied Protein Technology, Shanghai, China). group (Each n = 12) has three duplicate samples. Each 150-mg tissue sample contains mixtures of part of single testis from 4 mice in a group. Briefly, the samples were homogenized, lysed and digested. Then, the TMT reagent (Thermo Fisher Scientific) was used to label the resulting peptides, according to manufacturer’s instructions. The TMT-labeled digested samples were fractionated into 10 fractions using a Pierce High pH Reversed-Phase Fractionation kit.
Each fraction that contains the peptide mixture was processed for HPLC using an Easy nLC (Proxeon Biosystems, presently Thermo Fisher Scientific). A Q Exactive mass spectrometer (Thermo Scientific) was used for the LC-MS/MS analysis. The protein identification and quantification were accomplished using the MASCOT engine (Matrix Science, London, UK; version 2.2) and Proteome Discoverer 1.4.
The protein sequences of differentially expressed proteins were retrieved based on the UniProtKB database (Release 2016_10). Then, a bioinformatic analysis was performed. Blast2GO10 (Version 3.3.5) was used for the gene ontology (GO) annotation, which included the main steps, such as sequence blast, GO mapping, GO annotation and annotation augmentation.
Based on the online Kyoto Encyclopedia of Genes and Genomes (KEGG) database (http://geneontology.org/), the sample differential proteins were blasted to retrieve their functional orthologs (KOs) and mapped to KEGG pathways.
Fisher’s exact test was used to analyze the GO enrichment on three ontologies (biological process, molecular function, and cellular component) and the KEGG pathway enrichment. The background dataset was referred to all quantified protein annotations. The derived P-values were further adjusted using the Benjamini-Hochberg correction for multiple testing. It was considered significant only when the functional categories and pathways had a P-value of <0.05. The protein interactions (PPIs) were analyzed using the Cytoscape software (3.2.1) through the STRING database (http://string-db.org).
RNA isolation and real-time RT-PCR analysis
Fifty mg of mouse testis tissue were homogenized, and the total RNA was extracted using TRIzol reagent. The routine procedures of the real-time reverse transcription-polymerase chain reaction (RT-PCR) were performed to determine the mRNA level. First, the cDNA was obtained after a 1-μg aliquot of total RNA was reverse transcribed using a PrimeScript II kit (Takara Biotechnology, Dalian, China). The real-time RT-PCR analysis was used to evaluate the mRNA level with a Real-Time PCR/SYBR Green kit (Takara Biotechnology, Dalian, China) on an ABI StepOne™ Real-Time PCR System. All samples were run in triplicate. The primer pairs for the PCR produced by Biotech (Shanghai, PRC) are presented in . Gapdh levels were used for normalization.
Table 5. Primer sequences for genes analyzed by real-time RT-PCR.
Western-blotting
Protein was extracted from mouse testis tissue using RIPA lysis buffer, and separated via sodium dodecyl sulfate-polyacrylamide gel electrophoresis (SDS-PAGE). Polyvinylidene fluoride (PVDF) membranes were used to blot the proteins, followed by incubation with the primary antibody mouse anti-CRISP1 monoclonal antibody (R&D systems) and rabbit anti-PATE4 polyclonal antibody (Invitrogen). Then, HRP-conjugated secondary antibody was incubated, and the protein bands were detected using enhanced chemiluminescence (ECL). Afterward, the protein levels were normalized by the GAPDH level.
Statistical analysis
The data were presented as mean ± standard error of the mean (SEM). The statistical method of one-way ANOVA was used to analyze the differences between groups. A significance was considered at P < 0.05.
Author contributions
Conceived, designed the experiments, and wrote the manuscript: Z-mL; . performed the experiments: M-yL; . Analyzed the data: CC.
Acknowledgments
The authors are grateful to Qi Wang, Department of Pathology, The First Affiliated Hospital of the University of Science and Technology of China, for the electron microscopy.
Disclosure statement
No potential conflict of interest was reported by the authors.
Additional information
Funding
References
- Alayev A, Berger SM, Holz MK. 2015. Resveratrol as a novel treatment for diseases with mTOR pathway hyperactivation. Ann N Y Acad Sci. 1348(1):116–123.
- Alayev A, Doubleday PF, Berger SM, Ballif BA, Holz MK. 2014. Phosphoproteomics reveals resveratrol-dependent inhibition of Akt/mTORC1/S6K1 signaling. J Proteome Res. 13((12):):5734–5742.
- Araki N, Kawano N, Kang W, Miyado K, Yoshida K, Yoshida M. 2016. Seminal vesicle proteins SVS3 and SVS4 facilitate SVS2 effect on sperm capacitation. Reprod. 152(4):313–321.
- Archana D, Supriya C, Girish BP, Kishori B, Reddy PS. 2018. Alleviative effect of resveratrol on polyvinyl chloride-induced reproductive toxicity in male Wistar rats. Food Chem Toxicol. 116:173–181.
- Borges BC, Garcia-Galiano D, da Silveira Cruz-machado S, Han X, Gavrilina GB, Saunders TL, Auchus RJ, Hammoud SS, Smith GD, Elias CF. 2017. Obesity-induced infertility in male mice is associated with disruption of Crisp4 expression and sperm fertilization capacity. Endocrinol. 158(9):2930–2943.
- Bucci D, Spinaci M, Yeste M, Mislei B, Gadani B, Prieto Martinez N, Love C, Mari G, Tamanini C, Galeati G, et al. 2018. Combined effects of resveratrol and epigallocatechin-3-gallate on post thaw boar sperm and IVF parameters. Theriogenol. 117:16–25.
- Chen Q, Wang E, Ma L, Zhai P. 2012. Dietary resveratrol increases the expression of hepatic 7α-hydroxylase and ameliorates hypercholesterolemia in high-fat fed C57BL/6J mice. Lipids Health Dis. 11(1):56–63.
- Cohen DJ, Maldera JA, Vasen G, Ernesto JI, Muñoz MW, Battistone MA, Cuasnicú PS. 2011. Epididymal protein CRISP1 plays different roles during the fertilization process. J Androl. 32(6):672–678.
- Du Plessis SS, Cabler S, McAlister DA, Sabanegh E, Agarwal A. 2010. The effect of obesity on sperm disorders and male infertility. Nat Rev Urol. 7(3):153–161.
- Gao F, Li G, Liu C, Gao H, Wang H, Liu W, Chen M, Shang Y, Wang L, Shi J, et al. 2018. Autophagy regulates testosterone synthesis by facilitating cholesterol uptake in Leydig cells. J Cell Biol. 217(6):2103–2119.
- Ghanayem BI, Bai R, Kissling GE, Travlos G, Hoffler U. 2010. Diet-induced obesity in male mice is associated with reduced fertility and potentiation of acrylamide-induced reproductive toxicity. Biol Reprod. 82(1):96–104.
- Guneli E, Tugyan K, Ozturk H, Gumustekin M, Cilaker S, Uysal N. 2008. Effect of melatonin on testicular damage in streptozotocin-induced diabetes rats. Eur Surg Res. 40(1):354–360.
- Hu J, Merriner DJ, O’Connor AE, Houston BJ, Furic L, Hedger MP, O’Bryan MK. 2018. Epididymal cysteine-rich secretory proteins are required for epididymal sperm maturation and optimal sperm function. Mol Hum Reprod. 24(3):111–122.
- Huang Q, Luo L, Alamdar A, Zhang J, Liu L, Tian M, Eqani SA, Shen H. 2016. Integrated proteomics and metabolomics analysis of rat testis: mechanism of arsenic-induced male reproductive toxicity. Sci Rep. 6:32518.
- Juhasz B, Das DK, Kertesz A, Juhasz A, Gesztelyi R, Varga B. 2011. Reduction of blood cholesterol and ischemic injury in the hypercholesteromic rabbits with modified resveratrol, longevinex. Mol Cell Biochem. 348(1–2):199–203.
- Lagouge M, Argmann C, Gerhart-Hines Z, Meziane H, Lerin C, Daussin F, Messadeq N, Milne J, Lambert P, Elliott P, et al. 2006. Resveratrol improves mitochondrial function and protects against metabolic disease by activating SIRT1 and PGC-1alpha. Cell. 127(6):1109–1122.
- Li H, He Y, Yan J, Zhao Q, Di C, Zhang H. 2016. Comparative proteomics reveals the underlying toxicological mechanism of low sperm motility induced by iron ion radiation in mice. Reprod Toxicol. 65:148–158.
- Liu Y, Ding Z. 2017. Obesity, a serious etiologic factor for male subfertility in modern society. Reprod. 154(4):R123–R131.
- Macdonald AA, Stewart AW, Farquhar CM. 2013. Body mass index in relation to semen quality and reproductive hormones in New Zealand men: a cross-sectional study in fertility clinics. Hum Reprod. 28(12):3178–3187.
- Mojica-Villegas MA, Izquierdo-Vega JA, Chamorro-Cevallos G, Sánchez-Gutiérrez M. 2014. Protective effect of resveratrol on biomarkers of oxidative stress induced by iron/ascorbate in mouse spermatozoa. Nutrients. 6(2):489–503.
- Mu Y, Yan WJ, Yin TL, Zhang Y, Li J, Yang J. 2017. Diet-induced obesity impairs spermatogenesis: a potential role for autophagy. Sci Rep. 7:43475.
- Noda T, Fujihara Y, Matsumura T, Oura S, Kobayashi S, Ikawa M. 2018. Seminal vesicle secretory protein 7, PATE4, is not required for sperm function but for copulatory plug formation to ensure fecundity. Biol Reprod. 100(4):1035–1045.
- Palmer NO, Bakos HW, Fullston T, Lane M. 2012. Impact of obesity on male fertility, sperm function and molecular composition. Spermatogenesis. 2(4):253–263.
- Peng M, Yin N, Li MO. 2017. SZT2 dictates GATOR control of mTORC1 signalling. Nature. 543(7645):433–437.
- Sèdes L, Thirouard L, Maqdasy S, Garcia M, Caira F, Lobaccaro JA, Beaudoin C, Volle DH. 2018. Cholesterol: a gatekeeper of male fertility? Front Endocrinol (Lausanne). 9:369.
- Shukla KK, Chambial S, Dwivedi S, Misra S, Sharma P. 2014. Recent scenario of obesity and male fertility. Andrology. 2(6):809–818.
- Springer M, Moco S. 2019. Resveratrol and its human metabolites-effects on metabolic health and obesity. Nutrients. 11(1):1–17.
- Tatone C, Di Emidio G, Barbonetti A, Carta G, Luciano AM, Falone S, Amicarelli F. 2018. Sirtuins in gamete biology and reproductive physiology: emerging roles and therapeutic potential in female and male infertility. Hum Reprod Update. 24(3):267–289.
- Taylor A, Robson A, Houghton BC, Jepson CA, Ford WC, Frayne J. 2013. Epididymal specific, selenium-independent GPX5 protects cells from oxidative stress-induced lipid peroxidation and DNA mutation. Hum Reprod. 28(9):2332–2342.
- Timmers S, Konings E, Bilet L, Houtkooper RH, van de Weijer T, Goossens GH, Hoeks J, van der Krieken S, Ryu D, Kersten S, et al. 2011. Calorie restriction-like effects of 30 days of resveratrol supplementation on energy metabolism and metabolic profile in obese humans. Cell Metab. 14(5):612–622.
- Wang HJ, Wang Q, Lv ZM, Wang CL, Li CP, Rong YL. 2015a. Resveratrol appears to protect against oxidative stress and steroidogenesis collapse in mice fed high-calorie and high-cholesterol diet. Andrologia. 47(1):59–65.
- Wang P, Huo HL, Wang SY, Miao YW, Zhang YY, Zhang QL, Li FQ, Liu LX, Li WZ, Zeng YZ, et al. 2015b. Cloning, sequence characterization, and expression patterns of members of the porcine TSSK family. Genet Mol Res. 14(4):14908–14919.
- Wang X, Wei Y, Fu G, Li H, Saiyin H, Lin G, Wang Z, Chen S, Yu L. 2015c. Tssk4 is essential for maintaining the structural integrity of sperm flagellum. Mol Hum Reprod. 21(2):136–145.
- Xie C, Shen H, Zhang H, Yan J, Liu Y, Yao F, Wang X, Cheng Z, Tang TS, Guo C. 2018. Quantitative proteomics analysis reveals alterations of lysine acetylation in mouse testis in response to heat shock and X-ray exposure. Biochim Biophys Acta Proteins Proteom. 1866(3):464–472.
- Yi J, Tang XM. 1995. Functional implication of autophagy in steroid-secreting cells of the rat. Anat Rec. 242(2):137–146.
- Zhao Y, Song W, Wang Z, Wang Z, Jin X, Xu J, Bai L, Li Y, Cui J, Cai L. 2018. Resveratrol attenuates testicular apoptosis in type 1 diabetic mice: role of Akt-mediated Nrf2 activation and p62-dependent Keap1 degradation. Redox Biol. 14:609–617.
- Zheng J, Chen LL, Zhang HH, Hu X, Kong W, Hu D. 2012. Resveratrol improves insulin resistance of catch-up growth by increasing mitochondrial complexes and antioxidant function in skeletal muscle. Metab. 61(7):954–965.