Abstract
Both vitamin A and E support female reproduction and embryonic development. These vitamins have been associated with decreased fertility or failure to end the pregnancy in animals. An observational study was conducted on follicular fluid (FF) samples to determine the concentrations of fat-soluble vitamins of women undergoing in vitro fertilization and its correlation with assisted reproductive technology characteristics and pregnancy outcomes. Moreover, the effects of all-trans-retinoic acid (atRA) and alpha-tocopherol on granulosa cell viability, apoptosis, autophagy and hormonal production were evaluated. No association was identified between fat-soluble vitamin concentrations in FF and infertility aetiology, body mass index or woman’s age. There were differences in follicular antioxidant profiles and ovarian response stimulation. In vitro evaluation of atRA and alpha-tocopherol reveals that, at physiological concentrations, both compounds may affect the viability of granulosa cells. In addition, these compounds are able to protect granulosa cells from oxidative stress, as well as to affect estradiol and progesterone production. Our data suggest that atRA and alpha-tocopherol levels should be well controlled as they may have implications in the function and viability of granulosa cells and highlights retinol as a marker of the oxidative defenses within ovary environment.
Introduction
Besides ovulation dysfunction and uterine causes, lifestyle factors represent major risk factors for reproductive and pregnancy-related disorders. Unhealthy lifestyle behaviors, which include inadequate diet, tobacco smoking, alcohol consumption and/or drug abuse, have a negative impact on female fertility (de Angelis et al. Citation2020). The follicular fluid (FF) is the natural environment for the final phase of oocyte maturation. Several studies have addressed its composition and impact on oocyte growth and quality. Thus, variations in FF composition are associated with the development of embryos arising from in vitro fertilization (IVF) procedures. However, the importance of oxidative stress in FF of women undergoing ovarian stimulation remains largely unknown. Ovarian stimulation may directly impact stress markers and affect assisted reproduction outcomes. The classical IVF cycles are associated with the production of reactive oxygen/nitrogen species (ROS/RNS) and oxidant-antioxidant balance disturbance (Agarwal et al. Citation2022). Furthermore, the gonadotrophin-releasing hormone (GnRH) antagonist protocol has been related to increased oxidative stress (Ma et al. Citation2021). The mechanisms are multiple, but include oxidative damage and granulosa cells (GC) apoptosis through changes in the expression of key transcription factors (Nishihara et al. Citation2018). It also involves a decrease on oxidative defenses namely a reduced expression of catalase and glutathione peroxidase (Ma et al. Citation2021).
It is now clear that an adequate ROS balance is crucial for normal ovarian functions. ROS may exert either negative or positive effects on mammalian ovaries. Although epidemiologic studies have shown that high levels of ROS are associated with ovarian toxicity and result in the gradual loss of fertility (Agarwal et al. Citation2012), oxidative stress is required for ovulation and the inhibition of ROS generation disturbs ovulation (Shkolnik et al. Citation2011). In mice, nitric oxide (NO) production is essential for in vitro fertilization (IVF) and early embryo development (Kim et al. Citation2004). Melatonin antioxidant activity has also been described as a potential protection against ovarian aging and maintenance of mitochondrial redox balance (Song et al. Citation2016). On the contrary, higher follicular NO levels were associated with advanced fragmentation of embryos (Lee et al. Citation2004). Therefore, although limited levels of ROS/RNS are necessary, high concentrations can have deleterious effects (Shkolnik et al. Citation2011). However, such effects have never been demonstrated in human ovarian response. In turn, antioxidants regulate the overproduction of ROS and may present benefits in GC protection. Through diet we can obtain various fat-soluble antioxidants, particularly vitamin A and E. Vitamin A refers either to retinol and its esters (preformed vitamin A) or to certain carotenoids, especially β-carotene (provitamin A). Besides alpha-tocopherol, the largest biologically active form, vitamin E also includes beta-and gamma-tocopherols. These vitamins exhibit multiple biological actions that can protect against disease. For example, reduced plasmatic vitamin levels are associated with an increased risk of several chronic diseases and cancer. In addition, vitamin E is a chain-breaking antioxidant that protects cell membranes from lipid peroxidation (Szuwart et al. Citation2000).
Both vitamin A and E support female reproduction and embryonic development. Studies in cattle, sheep and pigs with retinoids show an increase in oocyte fertilization competence (Whaley et al. Citation2000; Ikeda et al. Citation2005). In bovine, vitamin A supplementation showed a positive effect on fertility in artificial insemination, whereas in vitro oocyte studies provide evidence of a role for retinoids in oocyte cytoplasmic maturation and fertilization competence (Ikeda et al. Citation2005). Moreover, at the time of oocyte retrieval, all-trans retinoic acid (atRA) levels, the predominant physiological form of Vitamin A, were associated with quality embryos (Pauli et al. Citation2013). Although several lines of evidence suggest that retinoids have teratogenic actions during embryogenesis, a prospective study, which examined 423 pregnancies exposed to a ‘high’ dose of vitamin A, did not provide any definitive conclusion (Mastroiacovo et al. Citation1999). Vitamin E deficiency also causes decreased fertility or failure to end the pregnancy in animals (Agarwal et al. Citation2006). The mechanisms are unclear, but may involve regulation of epithelial cell growth and differentiation. In porcine, retinoid acid inhibits GC differentiation (Hattori et al. Citation2000), through inhibition of LH-R and FSH-induced transcription (Minegishi et al. Citation2000). In addition, embryo germ cells lacking vitamin A cannot enter meiosis (Kumar et al. Citation2011). These findings assist in understanding the molecular mechanism underlying the effect of fat-soluble vitamins on GC and reproductive function.
Little research is available on the effect of antioxidants on human fertility. We designed an observational study to determine the concentrations of fat-soluble vitamins in the FF of women undergoing IVF/ICSI. This study assesses the correlation between follicular fat-soluble vitamin levels and age, BMI, ART characteristics and fertility outcomes as well as with the infertility etiologies. Despite numerous animal studies, there is very little knowledge about the effects of these antioxidants on the viability and function of human GC. Neverthless, few further clues exist regarding the levels of fat-vitamins in FF and their role in GC protection during oxidative stress. Thus, our objectives for this study were to (i) evaluate the vitamin A and E levels on the FF collected on the day of oocyte retrieval; (ii) investigate the potential relationship between FF antioxidant profile and IVF/ICSI outcome; (iii) determine the effects of atRA and alpha-tocopherol on human granulosa cells (hGC) and in the COV434 cell line viability and function; (iv) evaluate its potential protective function upon oxidative stress-induced GC.
Results
A total of 172 patients participated in the current study. The average age of women was 34.9 ± 3.3 years, and the average BMI was 24.1 ± 3.9 kg/m2. The characteristics of these participants as well as other assisted reproductive technologies (ART) characteristics (duration of infertility, follicle number, number of oocytes retrieved, and number of embryos transferred) and the implantation and clinical pregnancy rates are summarized in . From our participants, 23 were diagnosed with endometriosis, 35 as hormonal factors, 56 diagnoses were unexplained, whereas 58 were controls (i.e., male factor infertility or tubal factor).
Table 1. Demographic and clinical characteristics and follicular vitamin levels of the participating women in the total cohort.
Fat-soluble vitamin levels in FF
The analysis revealed the presence of vitamin A, E and carotenoids in all FF studied. Vitamin E had an average concentration of 4022.3 ± 1424.9 nmol/L. As expected, alpha-tocopherol was more abundant than beta- and gamma-tocopherol (3884.4 ± 1388.4, 59.4 ± 31.9 and 74.49 ± 39.9, respectively). Vitamin A levels ranged from 3707 to 28,398 nmol/L, whereas beta-carotene levels ranged from 33.73 to 636.13 nmol/L and lutein from 0.29 to 230.76 nmol/L. Vitamin A and beta-carotene concentrations from the same women correlated weakly with vitamin E levels (r2 = 0.578) but did not correlate with beta-carotene levels (r2 = 0.238) (data not shown).
Correlation of follicular vitamin levels and ART characteristics or fertility outcomes
The concentration of vitamins in FF was poorly correlated with patients age, BMI and serum hormone levels, with correlation coefficients in the range of −0.136 – 0.164 for women age, −0.348 – 0.030 for BMI and −0.217 – 0.156 for serum hormonal levels (). Similarly, there were no associations in follicular vitamin concentrations with either fertility treatment outcomes and ART characteristics () or clinical infertility diagnoses (tubal or male factor infertility, unexplained, endometriosis, hormonal factors) (). However, the mean gamma-tocopherol concentrations were significantly lower in the pool from endometriosis patients compared with hormonal factors or, for beta-carotene, between hormonal factor and unexplained infertility patients ().
Figure 1. A comparison of follicular gamma-tocopherol and beta-carotene levels (nM/L) among specific groups of patients accordingly with the etiology of infertility. (A) The levels of gamma-tocopherol in FF from women with hormonal factors are higher when compared with FF levels from endometriosis patients. (B) Higher follicular beta-carotene levels in women with unexplained infertility when compared with endometriosis patients. Data are presented as median. Means were compared using Kruskal–Wallis and multiple comparisons were assessed by Mann–Whitney test. Significant differences are denoted by *p < 0.05 vs. endometriosis group and #p < 0.05 vs. hormonal factors.
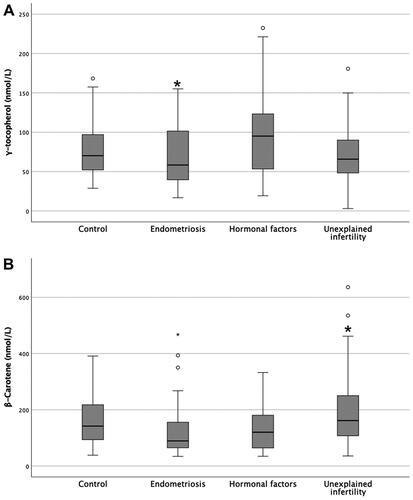
Table 2. Association of patient characteristics and the follicular vitamin levels (correlation coefficients).
Table 3. Vitamin levels in follicular fluid accordingly with infertility aetiology.
Effect of all-trans-retinoic acid and alpha-tocopherol on GCs viability and morphology
To determine the effects of both vitamin A and E treatment on GC viability and function, the hGC and COV434 were exposed to physiological concentrations of either atRA, the major active form of retinoids naturally present in mammals, or alpha-tocopherol (1–50 µM). As demonstrated in only 50 µM atRA induced a reduction in cell viability at all assayed times in hGCs. In contrast, alpha-tocopherol does not reduce granulosa cell viability in hGCs (). The hGCs proliferation even increased after 72 h of exposure to 50 µM alpha-tocopherol (). In turn, in COV434, after long exposure periods to concentrations higher than 10 µM for atRA or 5 µM, there is a reduction on cell viability (). After 72 h, both atRA and alpha-tocopherol at 10 µM induced changes on cell density and cellular morphology as observed in Giemsa staining. These effects were observed in both hGCs and COV434 cells ().
Figure 2. Effects of atRA and alpha-tocopherol on granulosa cell viability and morphology. (A) In both hGCs and COV434 cells, atRA induced a significant decrease in granulosa cell viability after 24 h, 48 h or 72 h post-treatment assessed by MTT assay. The effects of alpha-tocopherol on the reduction of granulosa cell viability were more pronounced in COV434 cells. (B) The atRA at 10 µM or alpha-tocopherol at 10 µM did not affect per se cell density nor morphology assessed by Giemsa staining after 72 h. Graphs are representative of three independent assays with three replicates for each concentration of the compounds. Data are presented as mean ± SEM (*p < 0.05 compared to respective untreated control; one-way ANOVA with Tukey’s ad hoc post-test).
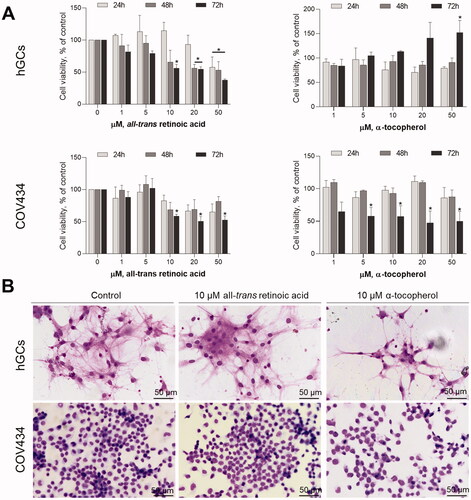
Effect of all-trans-retinoic acid and alpha-tocopherol on GCs death
According to the results of the MTT assay, cell viability was affected by the treatment of both COV434 and hGCs cells with 50 µM of atRA or alpha-tocopherol. To further determine if the reduction in cell viability induced by atRA at 50 µM might be attributable to apoptosis, Höechst staining and caspase -3/-7 activities assay were conducted. In hGC, there were no alterations in nuclear morphology induced by atRA or alpha-tocopherol. As evidenced by Höechst staining, there was an increase in chromatin condensation and some apoptotic bodies in COV434 cells exposed for 48 h with either atRA or alpha-tocopherol (). However, our results did not show an increase in caspase -3/-7 activities at hGC nor COV434 cells when exposed to atRA (). Because autophagy is linked with multiple forms of cellular stress, we wondered whether an autophagy-associated response could be triggered after atRA or alpha-tocopherol exposure. First, we addressed the formation of acidic vesicular organelles (AVOs) with acridine orange staining by fluorescence microscopy and then the processing of an autophagic marker protein, LC3-II, was detected by Western blotting. In hGC cells exposed to alpha-tocopherol and atRA at 10 µM stained AVOs were observed, though this effect was not observed in COV434 cells (). Nevertheless, it should be noted that neither atRA or alpha-tocopherol increased total LC3 levels compared with control cells ().
Figure 3. Effects of atRA and alpha-tocopherol on nuclear morphology and caspase -3/-7 activities. (A) In hGCs cells, neither atRA nor alpha-tocopherol at 50 µM induced increase in chromatin condensation when compared with control after 48 h of exposure. In COV434 cells, both atRA and alpha-tocopherol at 50 µM induced nuclear condensation after 48 h of exposure. (B) At 50 µM, atRA and alpha-tocopherol did not induce caspases-3/-7 activities neither in hGCs nor in COV434 cells. Data are presented as mean ± SEM (*p < 0.05 compared with respective untreated control; one-way ANOVA with Tukey’s ad hoc post-test). Staurosporine (STS; 100 nM) was used as positive control for caspases -3/-7 activities. Significant differences are denoted as *(p < 0.05) compared with respective untreated control; one-way ANOVA (with Tukey’s ad hoc post-test).
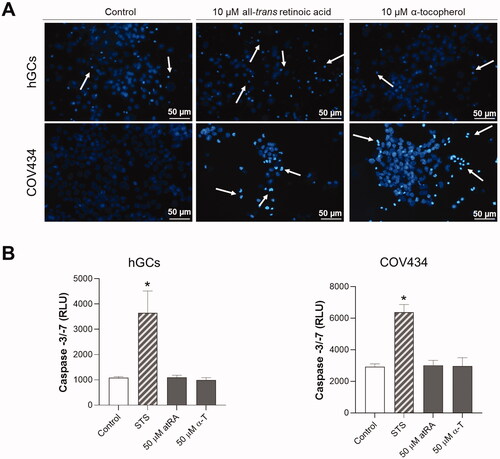
Figure 4. Evaluation of atRA and alpha-tocopherol effects on autophagy of granulosa cells after 72 h. (A) Fluorescent photographs of hGCs and COV434 cells after atRA treatment at 10 μM and alpha-tocopherol at 10 μM in comparison with control stained with fluorescent dye acridine orange (AO). AO staining assay demonstrated that alpha-tocopherol, but not atRA, increased the number of autophagosomes. (B) Representative Western-blot analysis for LC3 and beta-actin as well as the quantification of the total LC3/actin ratio. For total LC3/actin ratio determination, the Western blots were normalised to beta-actin and densitometric analysis was performed using image processing software. Etoposide (1 μM) was used as positive control (PC) of autophagy induction. Values are mean ± SEM of at least three independent experiments. Significant differences are denoted as *(p < 0.05) compared with respective untreated control (Control/C); one-way ANOVA (with Tukey’s ad hoc post-test).
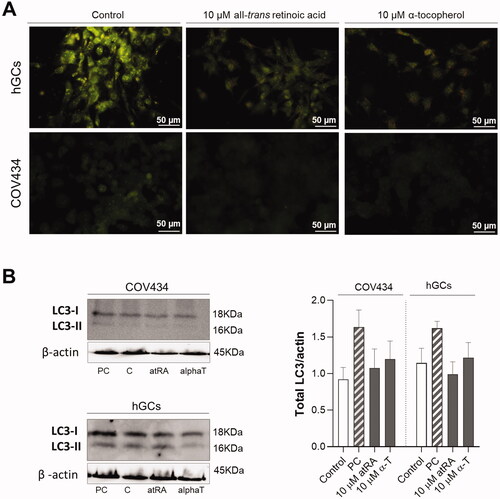
As ROS/RNS play important roles in follicular development and survival, we explored the effects of either atRA or alpha-tocopherol in ROS/RNS generation by granulosa cells. The t-Butyl hydroperoxide (tBHP) treatment significantly increased ROS/RNS species at the concentration of 5 μM. After tBHP insult, the treatment of both hGC or COV434 cells with either atRA or alpha-tocopherol for 72 h reduced ROS/RNS production when compared with the tBHP treatment alone ().
Figure 5. Evaluation of atRA and alpha-tocopherol in ROS/RNS formation before and after cell stress induction. GC treated with atRA and alpha-tocopherol at different concentrations (1–50 μM) for 72 h and then co-treated with tBHP 5 μM in comparison with tBHP alone, assessed by fluorescence assay with DCFH-DA probe. Significant differences are denoted as *(p < 0.05) compared with tBHP alone; one-way ANOVA (with Tukey’s ad hoc post-test).
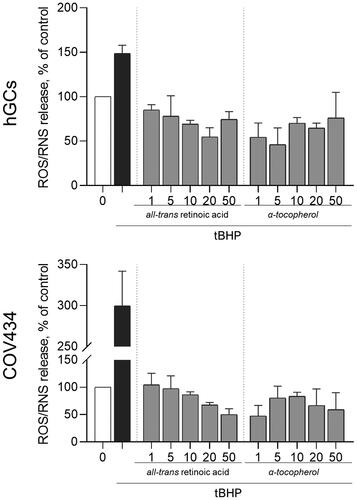
Effect of alpha-tocopherol and retinol on progesterone production by GCs
To explore the influence of atRA or alpha-tocopherol on endocrine function, GCs were cultured for five days after atRA or alpha-tocopherol treatments and analyzed for estrogen and progesterone production levels. In hGC, FSH stimulated both estradiol and progesterone secretion. The combination of FSH with either atRA or alpha-tocopherol (10 µM) significantly inhibited the FSH-stimulated release of progesterone. This effect was also observed in COV434 cells, though the amounts of progesterone were lower than the observed in hGC (). Contrary to progesterone, these compounds have no effect in estradiol levels indicating that excessive atRA or alpha-tocopherol might inhibit progesterone secretion by GCs.
Figure 6. Analysis of atRA and alpha-tocopherol treatments on the release of progesterone and estradiol and on the mRNA levels of steroidogenic enzymes in human GCs. After the five-day incubation period, FSH combined with either 10 µM of atRA (A) or alpha-tocopherol (C) caused an inhibition of estradiol and progesterone release by granulosa cells. FSH stimulation induced changes in the transcriptions (mRNA level) of the steroidogenic enzymes in both hGCs and COV434 cells by quantitative real-time RT-PCR method. (B) atRA induced an reduction in the expression of StAR and aromatase, whereas Cyp11a1, 3β-HSD and 17β-HSD expression did not change significantly after atRA stimulation. The alpha-tocopherol at 10 µM for five days caused a dose-dependent decrease in the secretion of progesterone and in the expressions of StAR and Cyp11a1, but did not cause a notable change in the 3β-HSD mRNA expression (*p < 0.05 compared with respective untreated control; #p < 0.05 compared with FSH treatment; one-way ANOVA with Tukey’s ad hoc post-test).
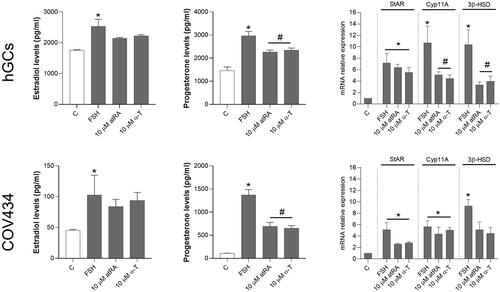
Next, we examined the effects of atRA or alpha-tocopherol at 10 µM for five days on the expression of steroidogenic-related genes. Real-time PCR results for StAR, Cyp11a1, and 3β-hydroxysteroid dehydrogenase (3β-HSD) mRNA are shown in . The combination of FSH with either atRA or alpha-tocopherol (10 µM) resulted in a significant reduction of StAR mRNA levels. In addition, Cyp11a1 mRNA expression also decreased in response to atRA in both cell models, though alpha-tocopherol only decreased Cyp11a1 in COV434 cells. In contrast, 3β-HSD mRNA levels remained unaltered ().
Discussion
There are a myriad of factors, including female age, ovarian reserve, and the effect of ovarian stimulation, that determine the success of ART. Several studies have investigated the association between ART outcome and FF composition, namely, anti-mullerian hormone, pregnancy-associated plasma protein-A and cholesterol ester transfer protein. Few reports have addressed the follicular levels of fat-soluble vitamins. Furthermore, the relationship between IVF outcome(s) and vitamin A and E levels remains controversial.
Vitamins seem to play a key role in oocyte maturation, since their deficiencies have been linked to a decreased fertility in animals (Lerchbaum and Obermayer-Pietsch Citation2012). Although vitamin A and E concentrations in FF of women have been previously quantified (Skowrońska et al. Citation2020), direct determination of the detailled composition of each vitamin including alpha-, beta-, gamma-tocoferol, beta-carotene and retinol as far as we know, has not been reported. We observed that retinol and alpha-tocopherol are the dominant forms of vitamin A and E in FF, respectively. During the human menstrual cycle, the expression of retinoid receptors and the synthesis of atRA in the reproductive tissue are influenced by the change in the pattern of steroid exposure (Jiang et al. Citation2018); however, no correlation was observed between FF vitamins and hormone levels, as well as with age or BMI. In addition, we did not find any association between ART characteristics or embryo quality and follicular vitamin levels. Hence, it appears that the FF level of fat-soluble vitamins has a complex relationship with clinical outcomes. Present findings differ from the previous conclusions on this subject showing that FF concentration of both vitamins A and E can accurately predict the success of subsequent fertilization (Skowrońska et al. Citation2020; Gode et al. Citation2019). We then examined the association between FF vitamin levels and the etiology of infertility. Although present at very low levels, gamma-tochopherol levels were lower in endometriosis patients suggesting that the antioxidant system is less active in women with endometriosis.
The retinoids naturally present in mammals exist in different configurations with the atRA the major active form. High FF levels of atRA were positively correlated with patient serum concentrations and with the highest quality embryos (Pauli et al. Citation2013). It has been shown that human cumulus granulosa cells (CGC) are a primary site of retinoid uptake and atRA biosynthesis supporting the essential role of retinoids in ovarian steroid production and oocyte maturation in mammals (Best et al. Citation2015). In bovine, atRA may promote cytoplasmic maturation oocytes via its modulatory effects on the gene expression of gonadotrophin receptors, cyclooxygenase-2 and/or nitric oxide synthase in CGC (Ikeda et al. Citation2005). In addition, follicular atresia is closely related to both apoptosis and autophagy of GC in ovarian follicles (Zhou et al. Citation2019). The role of atRA and alpha-tocopherol in the human granulosa cells is unclear. Therefore, in a second set of experiments, we adressed the role of atRA and alpha-tocopherol in GC viability and function. Contrary to alpha-tocopherol, GCs exposed to atRA for long periods reduced their viability. It suggests that vitamin A levels disregulation within the human ovary may be detrimental due to its involvement in GC death. Neverthless, we did not observe signals of apoptosis or autophagy of GCs exposed to both atRA and alpha-tocopherol.
It is pivotal to better understand the underlying cause for poor response to stimulation to maximize the chance of success of IVF treatment cycles. This result can be independently influenced by oxidative stress or decreased production of hormones by GC, thereby resulting in poor ART outcomes. There has been some recent attention to oxidative balance modulation at cellular and systemic levels by fat-soluble vitamins. Based on epidemiologic studies, these compounds were suggested to be an important dietary antioxidant, preventing pathologies associated to oxidative stress such as cancer, atherosclerosis, neurodegenerative diseases and other age-related conditions (Evans and Lawrenson Citation2012; Stepaniak et al. Citation2016). Some studies have addressed the effects of ROS imbalance in oocyte maturation, ovulation, luteolysis and follicle atresia and suggested that oxidative stress might be influenced by micronutrient intake (Silvestris et al. Citation2019; Soto-Heras and Paramio Citation2020).
It is apparent that GC dysfunction contributes to abnormal folliculogenesis and, thus, disruption of the oxidant/antioxidant balance negatively affects assisted reproduction outcome. Here, we observed that ROS/RNS produced by GC were inhibited by both atRA and alpha-tocopherol. This suggests that vitamins may play a role in the alteration of the oxidative products secreted from the GC. Our data confirmed numerous publications reporting the antioxidant capability of vitamin A and E that, accordingly, are regarded as potent antioxidants. It has been reported that these vitamins effectively scavenge oxidizing free radicals by inhibiting lipid peroxidation, thus lowering free radicals and ROS spreading (Le Prell et al. Citation2007). In this regard, within FF, these vitamins may play a key role in oocyte quality and maturation. Recently, decreased atRA levels were associated with disrupted meiosis in developing oocytes (Kam Draskau et al. Citation2022) as well as atRA reduced the proportion of degenerated oocytes and improved first polar body extrusion on camel cumulus-oocyte complex experiments (Saadeldin et al. Citation2019). Moreover, it has been demonstrated that retinoic acid supplementation enhances nuclear oocyte maturation, cleavage and maturation rates, blastocyst formation and embryo development in in vitro embryo production of livestock (Abdelnour et al. Citation2019). Therefore, appropriate consumption of these vitamins may have significant effects on redox status of follicular microenvironment and, ultimately, on IVF outcome.
At the same time, we observed that both atRA and alpha-tocopherol, at physiological concentrations, may modulate hormonal production by GC, a pivotal process in oocyte development. At hGC, both compounds induced a decrease in progesterone production but not in estradiol. As expected, large luteal cells express CYP11A1, an enzyme known to be crucial for progesterone production. In addition, 3β-hydroxysteroid dehydrogenase (3β-HSD) and steroidogenic acute regulatory enzyme (StAR) were found in cultured GC. Altoghether, these enzymes are indispensable for progesterone synthesis. In hGC, CYP11A1 and 3β-HSD enzymes massively declined over atRA treatment, which indicates a loss of steroidogenic function, particularly on progesterone production that must be tightly regulated to ensure a successful pregnancy. Elevated progesterone production that occur after LH surge is required to regulate follicular rupture and luteinization, which are crucial for infertility treatments including treatments based on natural menstrual cycles (Dozortsev and Diamond Citation2020).
It is now generally believed that atRA is the form of vitamin A that supports both male and female reproduction as well as embryonic development. Here, we evidenced that within human ovary, these vitamins are critical for hormonal regulation. Particularly vitamin A should be highly regulated as increased concentrations may negatively affect GCs viability, and thus oocyte development. Several published reports have identified dietary, demographic and lifestyle variables that affect serum concentrations of fat-soluble vitamins in adults. However, there are few published data of this nature on women of reproductive age. The association of FF vitamin A, vitamin E and carotenoids with health outcomes among women undergoing ART as influencing factors should be carefully considered when analyzing and interpreting data and subsequent conclusions about diet/health relationships. We demonstrate that vitamin A is involved in FF homeostasis, suggesting the existence of a close functional correlation between follicular protein and micronutrients. Therefore, dietary intake can severely affect follicular physiology.
Overall, our data indicate that vitamin A plays an important role in determining the number of oocytes retrieved for stimulation, but does not inform the effectiveness of interventions that alter vitamin A concentrations and, thus, the ovarian response. In addition, our study identifies that both vitamin A and E have an impact on the production of steroid hormones from the GC of the ovary, which are an important predictor of ovarian response. This could be caused by antioxidant properties of vitamin A, which may protect the oocyte from oxidative stress damage. The concentration of vitamin A and E in FF must be tightly regulated, but the exact mechanisms responsible for this regulation are not fully understood. At the same time, further studies are needed to clarify the contribution of vitamins A and E to the modulation of GC viability and function.
Materials and methods
Study design and participants
We conducted an observational study, enrolling women attending the Fertility Unit of Centro Hospitalar Vila Nova de Gaia/Espinho E.P.E. (CHVNG/E) between January 2017 and June 2018. A total of 172 patients were enrolled in this study. All patients had been unable to conceive naturally for at least one year before entering the study. Inclusion criteria were an age ≤ 39 years, body mass index (BMI) from 18 to 40 kg/m2, and diagnosis of endometriosis (n = 23), hormonal factor (n = 35) and idiopathic infertility (n = 56). The control group was composed of women with tubal factor or no evidence of female infertility and were treated mainly due to a male infertility factor (n = 58). Women who had multiple etiologies for infertility were excluded from the analysis. The baseline characteristics of the participating women were compiled from medical records and are presented in .
Study procedures
The FF was collected at the time of oocyte retrieval from women undergoing ovarian stimulation for classic IVF, intracytoplasmic sperm injection (ICSI) or both. All the patients were treated with GnRH analogues and recombinant human follicle stimulating hormone (r-hFSH) plus recombinant human luteinizing hormone (r-hLH) or r-hFSH alone for multifollicular development. FF was pooled, centrifuged to separate cellular debris, divided into aliquots and immediately stored at −80°C. The volume and the appearance of each FF sample were recorded. Only aspirates of follicles which were not contaminated with blood and containing an oocyte were included in the analysis.
Measurement of fat-soluble vitamins
Fat extraction was performed on the basis of the Smedes method, with minor adjustments (Cruz and Casal Citation2018), using 2.5 mL portions of FF. The lipidic extract was analyzed by normal-phase HPLC (Jasco, Japan) equipped with DAD (Jasco MD – 4010, Japan), and a dual-channel fluorescence detector (FLD, Jasco FP – 4025, Japan) as described previously (Cruz and Casal Citation2018). Separation was achieved on a Luna silica column (100 mm × 3 mm; 3 µm, Phenomenex, USA), with a gradient of hexane and 1,4-dioxane. The standards α-tocopherol, β-tocopherol, γ-tocopherol, beta-carotene, lutein, retinyl palmitate and tocol (internal standard) were purchased from diverse suppliers. Quantification was based on fluorescence (tocopherols and retinols) and DAD (carotenes and lutein) signals.
Primary granulosa cells, cell lines and cell culture conditions
For primary human granulosa cells (hGC) cultures, FF from women diagnosed with tubal factor infertility and/or male factor infertility was used (other causes of infertility like endometriosis, ovulatory factor, uterine factor or unexplained infertility were excluded). The hGCs were then isolated by using Percoll density gradient centrifugation as previously described (Costa et al. Citation2021). The human granulosa cell line COV434 (#88081201) was purchased from The European Collection of Authenticated Cell Cultures (ECACC). Both cell models were cultured in DMEM/F12 culture medium supplemented with 10% fetal bovine serum (FBS) and 1% of AB-AM, and incubated at 37°C in a humidified 5% CO2 atmosphere. Unless otherwise stated, all reagents were purchased from Invitrogen.
Cell staining and viability analysis
For cell viability determination, COV434 and hGC were seeded in 96-well plates at a density of 3.5 × 105 and 7.0 × 105, respectively. After 24 h, cells were exposed to either all-trans-retinoic acid (atRA) or α-tocopherol (0.01–50 µM) for 24, 48 and 72 h and the tetrazolium-based MTT assay was used to determine cell viability. For morphology evaluation, cells were exposed to either atRA or α-tocopherol for 48 h in 24-well plates. For Giemsa and Höechst staining, cells were fixed with a 4% paraformaldehyde solution. Giemsa stain was added to the cells for 30 min, then cells were observed by light microscopy. For Höechst and Acridine Orange staining, cells were incubated with 0.5 μg/ml Höechst 33342 or 5 μg/mL Acridine Orange (AO) at 37°C for 20 min and were observed by fluorescence microscopy (Eclipse Ci, Nikon, Japan).
Evaluation of caspase-3/-7 activities
COV434 and hGC were seeded in 96-well white plate and incubated for 48 h in the absence or presence of atRA (50 μM) or α-tocopherol (50 μM). At the end of the incubation time, Caspase-Glo -3/-7 reagent (Promega, #G811C) was added, according to the manufacturer’s instructions. The plates were incubated for 1 h and the resultant luminescence was measured in the Microplate Luminometer (BioTek Instruments, Winooski, VT, USA). A positive control assay was conducted using staurosporine (STS; 1 μM), which was added 12 h before the end of the experiment.
Tissue processing for biochemical analysis
For Western blots, proteins (25 μg) were separated by sodiumdodecylsulphate polyacrylamide gel electrophoresis under denaturing and reducing conditions using SDS and 2-mercaptoethanol (SDS-PAGE) and then transferred onto polyvinylidene difluoride membranes as previously described (Fonseca et al. Citation2020). After blocking with 5% (w/v) nonfat dry milk for 1 h at room temperature, membranes were incubated overnight at 4°C with primary antibody for LC3 I/II (1:200; Cell Signaling Technology, Boston, USA; #12741). Following washing, membranes were then incubated with secondary antibody for 1 h and immunoreactive bands were visualized by enhanced chemiluminescence (Advansta, CA, USA) in a ChemiDocTM Touch Imaging System (BioRad, Laboratories Melville, NY, USA). Membranes were then stripped and re-incubated with anti-β-actin (1:500; sc-47778) to control loading variation. For total LC3/actin ratio determination, the Western blots were normalized to beta-actin and densitometric analysis was performed using Image Lab 6.0.1 software (BioRad, Laboratories Melville, NY, USA). Etoposide (1 μM) was used as positive control of autophagy induction.
To analyze the mRNA levels, cells were cultured for five days and total RNA was extracted using chloroform/isopropanol precipitation and then reverse transcribed into first-strand complementary DNA (cDNA) according to the Transcriptor first strand cDNA synthesis kit (Grisp, Portugal; #GK34.0100). Quantitative real-time PCR analyses were performed using the SYBRGreen PCR Master Mix (Applied Biosystem, #4309155) and indicated primers (Supplementary Table 1) on the StepOnePlus Real-Time PCR System. Relative gene expression was normalized to GAPDH and mRNA abundance was quantified using the 2−ΔΔCt method.
Evaluation of intracellular reactive oxygen and nitrogen species (ROS/RNS)
COV434 and hGC cells were seeded in 96-well black plates and treated with either atRA (0.1 − 50 μM) or α-tocopherol (0.1 − 50 μM) for 72 h. To determine ROS/RNS, cells were incubated with the probe 2′-7′-dichlorodihydrofluorescein (DCDHF-DA) for 1 h at room temperature and an oxidative stress exogenous inducer tert-Butyl hydroperoxide (tBHP) at 5 μM. Fluorescence, proportional to the cellular levels of ROS/RNS, was measured using the Microplate Fluorimeter (excitation: 485 ± 10 nm; emission: 530 ± 12.5 nm) over the course of 30 min.
Hormonal quantification
After isolation, hGC were plated in 24-well plates for 24 h and then treated with atRA (10 μM) or α-tocopherol (10 μM) during five days. In every treatment, one unit of FSH (Sigma-Aldrich,USA) and the substractum androstenedione (10 nM) dissolved in DMSO were added. Cell culture media was collected and centrifuged at 300 g. Estradiol (R&D systems #KGE014) and progesterone (Invitrogen #EIAP4C21) secretion were evaluated by Competitive ELISA kit according to the manufacturer’s instructions and standardized to cell protein.
Statistical analyses
Demographic and cycle characteristic data are expressed as mean ± SD (range) or median (interquartile range) where appropriate. Variables not normally distributed were log transformed to normalize their distribution and reported as medians with interquartile ranges. Means were compared using analysis of variance (ANOVA) or Kruskal–Wallis test. Correlations between variables of interest were performed using Pearson’s or Spearman coefficients (except when stated otherwise). Statistical analyses were performed using SPSS version 25. Statistical significance was set to p < 0.05.
Ethics approval and consent to participate
The present study was approved by the Centro Hospitalar Vila Nova de Gaia/Espinho E.P.E. (CHVNG/E) Ethics Committee and by the National Data Protection Commission (authorization number 526/2017). All patients were informed about the study and signed an informed consent form, according to the Declaration of Helsinki.
Authors' contributions
Contributed to the conception of the study, data analysis and manuscript preparation: BF; contributed to the conception of the study: BP; helped with the collection of follicular fluid: EF, LC; performed the analysis of the vitamins: RC, SC; contributed to the performance of the statistical analysis with constructive discussions: PO; contributed to the data analysis and study preparation: IR. All authors read and approved the final study.
Abbreviations | ||
3β-HSD | = | 3β-hydroxysteroid dehydrogenase |
AO | = | acridine orange |
ART | = | assisted reproductive technology |
atRA | = | all-trans-retinoic acid |
BMI | = | body mass index |
cDNA | = | complementary DNA |
CGC | = | cumulus granulosa cells |
DCDHF-DA | = | 2′-7′-dichlorodihydrofluorescein |
FF | = | follicular fluid |
FSH | = | follicle-stimulating hormone |
GC | = | granulosa cells |
hGC | = | human granulosa cells |
ICSI | = | intracytoplasmic sperm injection |
IVF | = | in vitro fertilization |
LH | = | luteinizing hormone |
NO | = | nitric oxide |
PC | = | positive control |
qRT-PCR | = | reverse transcription and real-time PCR |
RNS | = | nitrogen species |
ROS | = | reactive oxygen |
StAR | = | steroidogenic acute regulatory enzyme |
tBHP | = | tert-Butyl hydroperoxide |
Supplemental Material
Download MS Word (17.2 KB)Acknowledgments
The authors thank the whole staff from the CHVNG/E for their assistance.
Disclosure statement
No potential conflict of interest was reported by the authors
Additional information
Funding
References
- Abdelnour SA, Abd El-Hack ME, Swelum AA, Saadeldin IM, Noreldin AE, Khafaga AF, Al-Mutary MG, Arif M, Hussein EOS. 2019. The usefulness of retinoic acid supplementation during in vitro oocyte maturation for the in vitro embryo production of livestock: a review. Animals. 9(8):561.
- Agarwal A, Aponte-Mellado A, Premkumar BJ, Shaman A, Gupta S. 2012. The effects of oxidative stress on female reproduction: a review. Reprod Biol Endocrinol. 10:49.
- Agarwal A, Gupta S, Sikka S. 2006. The role of free radicals and antioxidants in reproduction. Curr Opin Obstet Gynecol. 18(3):325–332.
- Agarwal A, Maldonado Rosas I, Anagnostopoulou C, Cannarella R, Boitrelle F, Munoz LV, Finelli R, Durairajanayagam D, Henkel R, Saleh R. 2022. Oxidative stress and assisted reproduction: a comprehensive review of its pathophysiological role and strategies for optimizing embryo culture environment. Antioxidants. 11(3):477.
- Best MW, Wu J, Pauli SA, Kane MA, Pierzchalski K, Session DR, Woods DC, Shang W, Taylor RN, Sidell N. 2015. A role for retinoids in human oocyte fertilization: regulation of connexin 43 by retinoic acid in cumulus granulosa cells. Mol Hum Reprod. 21(6):527–534.
- Costa L, Moreia-Pinto B, Felgueira E, Ribeiro A, Rebelo I, Fonseca BM. 2021. The major endocannabinoid anandamide (AEA) induces apoptosis of human granulosa cells. Prostaglandins Leukot Essent Fatty Acids. 171:102311.
- Cruz R, Casal S. 2018. Direct analysis of vitamin A, vitamin E, carotenoids, chlorophylls and free sterols in animal and vegetable fats in a single normal-phase liquid chromatographic run. J Chromatogr A. 1565:81–88.
- de Angelis C, Nardone A, Garifalos F, Pivonello C, Sansone A, Conforti A, Di Dato C, Sirico F, Alviggi C, Isidori A, et al. 2020. Smoke, alcohol and drug addiction and female fertility. Reprod Biol Endocrinol. 18(1):21.
- Dozortsev DI, Diamond MP. 2020. Luteinizing hormone-independent rise of progesterone as the physiological trigger of the ovulatory gonadotropins surge in the human. Fertil Steril. 114(2):191–199.
- Evans JR, Lawrenson JG. 2012. Antioxidant vitamin and mineral supplements for preventing age-related macular degeneration. Cochrane Database Syst Rev. 7(7):CD000253.
- Fonseca BM, Cunha SC, Goncalves D, Mendes A, Braga J, Correia-da-Silva G, Teixeira NA. 2020. Decidual NK cell-derived conditioned medium from miscarriages affects endometrial stromal cell decidualisation: endocannabinoid anandamide and tumour necrosis factor-alpha crosstalk. Hum Reprod. 35(2):265–274.
- Gode F, Akarsu S, Dikmen ZG, Tamer B, Isik AZ. 2019. The effect follicular fluid vitamin A, E, D and B6 on embryo morphokinetics and pregnancy rates in patients receiving assisted reproduction. Gynecol Obstet Reprod Med. 25(2):89–95.
- Hattori M, Takesue K, Nishida N, Kato Y, Fujihara N. 2000. Inhibitory effect of retinoic acid on the development of immature porcine granulosa cells to mature cells. J Mol Endocrinol. 25(1):53–61.
- Ikeda S, Kitagawa M, Imai H, Yamada M. 2005. The roles of vitamin A for cytoplasmic maturation of bovine oocytes. J Reprod Dev. 51(1):23–35.
- Jiang YW, Chen L, Taylor RN, Li CJ, Zhou X. 2018. Physiological and pathological implications of retinoid action in the endometrium. J Endocrinol. 236(3):R169–R188.
- Kam Draskau M, Ravn Ballegaard AS, Ramhoj L, Bowles J, Svingen T, Spiller CM. 2022. AOP key event relationship report: linking decreased retinoic acid levels with disrupted meiosis in developing oocytes. Curr Res Toxicol. 3:100069.
- Kim BH, Kim CH, Jung KY, Jeon BH, Ju EJ, Choo YK. 2004. Involvement of nitric oxide during in vitro fertilization and early embryonic development in mice. Arch Pharm Res. 27(1):86–93.
- Kumar S, Chatzi C, Brade T, Cunningham TJ, Zhao XL, Duester G. 2011. Sex-specific timing of meiotic initiation is regulated by Cyp26b1 independent of retinoic acid signalling. Nat Commun. 2:151.
- Le Prell CG, Hughes LF, Miller JM. 2007. Free radical scavengers vitamins A, C, and E plus magnesium reduce noise trauma. Free Radic Biol Med. 42(9):1454–1463.
- Lee TH, Wu MY, Chen MJ, Chao KH, Ho HN, Yang YS. 2004. Nitric oxide is associated with poor embryo quality and pregnancy outcome in in vitro fertilization cycles. Fertil Steril. 82(1):126–131.
- Lerchbaum E, Obermayer-Pietsch B. 2012. Vitamin D and fertility: a systematic review. Eur J Endocrinol. 166(5):765–778.
- Ma Y, Zhao Z, Hao G, Cui N, Fan Y, Cao Y, Tan Z, Geng J, Fan L, Du H, et al. 2021. Effects of multicycle gonadotropin-releasing hormone antagonist protocols on oxidative stress of follicular fluid and ovarian granulosa cells. Hum Cell. 34(5):1324–1334.
- Mastroiacovo P, Mazzone T, Addis A, Elephant E, Carlier P, Vial T, Garbis H, Robert E, Bonati M, Ornoy A, et al. 1999. High vitamin A intake in early pregnancy and major malformations: a multicenter prospective controlled study. Teratology. 59(1):7–11.
- Minegishi T, Hirakawa T, Kishi H, Abe K, Ibuki Y, Miyamoto K. 2000. Retinoic acid (RA) represses follicle stimulating hormone (FSH)-induced luteinizing hormone (LH) receptor in rat granulosa cells. Arch Biochem Biophys. 373(1):203–210.
- Nishihara T, Matsumoto K, Hosoi Y, Morimoto Y. 2018. Evaluation of antioxidant status and oxidative stress markers in follicular fluid for human in vitro fertilization outcome. Reprod Med Biol. 17(4):481–486.
- Pauli SA, Session DR, Shang W, Easley K, Wieser F, Taylor RN, Pierzchalski K, Napoli JL, Kane MA, Sidell N. 2013. Analysis of follicular fluid retinoids in women undergoing in vitro fertilization: retinoic acid influences embryo quality and is reduced in women with endometriosis. Reprod Sci. 20(9):1116–1124.
- Saadeldin IM, Swelum AA, Elsafadi M, Mahmood A, Yaqoob SH, Alfayez M, Alowaimer AN. 2019. Effects of all-trans retinoic acid on the in vitro maturation of camel (Camelus dromedarius) cumulus-oocyte complexes. J Reprod Dev. 65(3):215–221.
- Shkolnik K, Tadmor A, Ben-Dor S, Nevo N, Galiani D, Dekel N. 2011. Reactive oxygen species are indispensable in ovulation. Proc Natl Acad Sci U S A. 108(4):1462–1467.
- Silvestris E, Lovero D, Palmirotta R. 2019. Nutrition and female fertility: an interdependent correlation. Front Endocrinol (Lausanne)). 10:346.
- Skowrońska P, Kunicki M, Pastuszek E, Konieczna L, Bączek T, Łukaszuk K. 2020. Follicular fat-soluble vitamins as markers of oocyte competency. Syst Biol Reprod Med. 66(2):112–121.
- Song C, Peng W, Yin SN, Zhao JM, Fu BB, Zhang JC, Mao TC, Wu HB, Zhang Y. 2016. Melatonin improves age-induced fertility decline and attenuates ovarian mitochondrial oxidative stress in mice. Sci Rep. 6:35165.
- Soto-Heras S, Paramio MT. 2020. Impact of oxidative stress on oocyte competence for in vitro embryo production programs. Res Vet Sci. 132:342–350.
- Stepaniak U, Micek A, Grosso G, Stefler D, Topor-Madry R, Kubinova R, Malyutina S, Peasey A, Pikhart H, Nikitin Y, et al. 2016. Antioxidant vitamin intake and mortality in three Central and Eastern European urban populations: the HAPIEE study. Eur J Nutr. 55(2):547–560.
- Szuwart T, Brzoska T, Luger TA, Filler T, Peuker E, Dierichs R. 2000. Vitamin E reduces platelet adhesion to human endothelial cells in vitro. Am J Hematol. 65(1):1–4.
- Whaley SL, Hedgpeth VS, Farin CE, Martus NS, Jayes FCL, Britt JH. 2000. Influence of vitamin A injection before mating on oocyte development, follicular hormones, and ovulation in gilts fed high-energy diets. J Anim Sci. 78(6):1598–1607.
- Zhou J, Peng X, Mei S. 2019. Autophagy in ovarian follicular development and atresia. Int J Biol Sci. 15(4):726–737.