Abstract
This paper reports a performance analysis for a new sustainable engineering application to beneficially reuse an abundant agricultural waste, coconut coir (Cocos nucifera), in evaporative cooling pads. Two small coconut coir pads of different configurations were fabricated and tested using a laboratory‐scale experimental arrangement. The air supply velocity was controlled and varied between 1.88 and 2.79 m s−1. Heat and mass transfer coefficients, evaporative cooling efficiency and pressure drop across the two types of coconut coir pad were analysed and compared with those of a commercial rigid media paper pad. Results show that the cooling efficiency of the manufactured coconut coir evaporative cooling pad was fairly good (about 50%) and close to that of the commercial paper pad (about 47%). The average pressure drop across the two coconut coir pads was 1.5 and 5.1 Pa respectively. Correlations for heat and mass transfer coefficients expressed using Nusselt and Sherwood numbers are also reported. In addition, the cooling potential of the coconut coir pads was analysed using the average climatic conditions of the central region in Thailand throughout the year. The analysis showed that the air temperature leaving the coconut coir pad varied from 23 to 28°C. Commercial development appears feasible given the coconut coir pad's good performance, lower cost and its availability throughout the country.
1. Introduction
It is well known that the evaporation of liquid water streaming along a wall reduces temperature inside a greenhouse, livestock farm or buildings (Jain and Tiwari Citation2002, Kittas et al. Citation2003, Willits Citation2003). This technique can improve working conditions for employees and the environment for plants and animals. In addition, evaporative cooling is inexpensive and can be used as an alternative process to mechanical refrigeration (ASHRAE Citation1983, Watt Citation1986). The wetted material behaviour can be classed as aspen pad and rigid media. Aspen pad behaviour is difficult to achieve in Thailand and rigid media is mostly imported. To reduce investment cost and promote sustainable engineering systems, research on alternative and cheap materials with reasonable thermal performance, comparable or better than rigid media, is necessary. Thailand agricultural data indicate that coconut production is about 1.4 million tonnes per year, of which more than 0.5 million tonnes per year is waste (Agricultural Statistics of Thailand Crop Year 2001/Citation02). In initial research, the authors found that coconut coir has the ability to adsorb air moisture (Khedari et al. Citation2003). In this paper, the authors report the use of this agricultural waste in a new engineering application as a direct evaporative cooling system. Many natural fibres can be used as evaporative cooling pad materials. For examples, jute tossa (Corchorus olitorius) has the highest average cooling efficiency of 62.1% compared with 55.1% for luffa fibers and 38.9% for date palm fibres (Phoenix dactylifera) for an air velocity of 2.4 m s−1 (Al‐Sulaiman 2006). The water hyacinth (Eichhornia crassipes) has also been studied and heat and mass transfer coefficients analysis showed that a 20 cm wide wetted surface of untransmuted water hyacinth was the best size to use for a evaporative cooling pad with cooling efficiency varying between 20 and 40% at air velocity varying between 1.5 and 3.6 m s−1 (Ubonwat Citation2001).
This paper presents an experimental investigation of the performance of coconut coir material used as a direct evaporative cooling pad. Heat and mass transfer coefficients were determined for the evaporative process for two different configurations of coconut coir pad media. To validate the results, they were compared with those deduced from Dowdy's correlation (Dowdy and Karabash Citation1988). Cooling efficiency, friction factor and pressure drop across the pads are also reported as these are useful for system engineering design. The performance of the coconut coir pads was also compared to a widely used commercial honeycomb rigid paper pad media in the evaporative cooling system in Thailand. Finally, in order to predict long‐term commercial potential, analysis of the cooling performance of the coconut coir pads at the average climatic conditions of the central region in Thailand throughout the year is reported and discussed.
2. Preparation and fabrication of coconut coir pads
The coconut coir pads were prepared using the following methodology. First, 600 grams of dried coir pieces (2×2×2 cm, shown in Figure ) were mixed thoroughly with 150 grams of latex glue. Next the coconut coir pieces were placed on a plate of 2 cm in depth, located in a forming box of 35×35×35 cm where they were pressed by hand until the mix had a square configuration. The forming box was removed and the coconut coir was pressed at 200 bar for 1 day to dry and consolidate it. After that the coconut coir sheet was cut into four rectangular pieces each of 13.5×7 cm.
In this study, two types of coir pad were considered: type 1, referred to as CC1, was made by cutting one piece of prepared coconut coir into six rectangular pieces 7 cm in length, 2.25 cm in width and 2 cm in thickness. Three coconut coir pieces were used in parallel and joined with six rectangular pieces of coir with latex glue, as shown in Figure . The arrangement of six rectangular coir pieces was divided into two big channels with opposing directions. For the alignment of the rectangular coir pieces, see Figure ; each rectangular coir piece was inclined at about 45 degrees.
The coconut coir pad type 2, referred to as CC2, was made by cutting the coconut coir into four rectangular pieces of 7 cm length, 6 cm width and 2 cm thickness. Next, two coconut coir pieces were attached to four rectangular coir pieces with latex glue, as shown in Figure . All four rectangular coir pieces were arranged with an inclination of about 6 degrees. Coconut coir pads were lined up alternatively to form the air channel to enhance the air motion. Figure shows the alignment of the rectangular coir pieces for the two pad types. The commercial rigid cellulose pad is the corrugated cellulose paper sheets with different flute angles, one steep (45 degrees) and one flat (45 degrees) that have been bonded together as shown in Figure . The dimensions of the three pads were 13.5×10×7 cm.
3. Experimental arrangement and test methodology
A small scale experimental arrangement was developed to determine the heat and mass transfer coefficients and cooling efficiency of the pads. The cross flow, direct evaporative cooler system consists of the rigid pad media, a tunnel, a water pump, a DC brushless fan, and a sprinkler, shown schematically in Figure . The tunnel is rectangular with 12 by 12 cm cross‐section and a length of 60 cm. It was using acrylic sheets of 5 mm thickness. Ambient air was forced to circulate through the tunnel by the fan. Cooling water was sprayed from above by a sprinkler onto the top surface of rigid media so that the processed air becomes humid and cold because of the heat and mass exchange between falling film water and air. Finally, the falling water was collected in a basin at the bottom of the evaporative cooler. This water was recycled to the sprinkler by means of a pump.
The rigid media was wetted before testing to ensure saturation prior to the test. At the beginning of each test, the initial velocity was maintained for 30 min. Then, for each test air velocity increment, at least 20 minutes was needed to ensure equilibrium between the rigid media and ambient air conditions.
The inlet and outlet air properties of the rigid media were measured and displayed at one minute intervals using humidity and temperature probes connected to a recorder (Testo 454). The dynamic pressure was measured by a differential pressure probe also connected to a Testo 454 recorder. The positions of the air probe and pressure probe measurements are shown in Figure . The accuracy of the measuring instruments used was: ±2% for relative humidity; ±0.05°C for temperature; ±0.03 m s−1 for velocity; and ±0.03 Pa for pressure.
It is obvious that operating conditions, such as incoming dry‐bulb air temperature and moisture content; water temperature; and flow rate affect the evaporative cooling pad performance significantly. However, in practice, neither ambient conditions nor water temperature is controlled in most commercial systems; only the supply air velocity (a term usually used for an evaporative process to denote that the velocity is that measured at the outlet of cooling pad) is adjusted according to the prescribed air flow rate required for the intended application. This is the methodology used in this research. The air velocity circulated through the wetted media was adjusted by controlling the DC brushless fan (model BFB1212HH, 12 V, 1.65 A). Four air supply velocities were considered, namely: 1.88, 2.21, 2.45 and 2.79 m s−1. The water temperature was assumed equal to the web bulb temperature of the incoming air. For each test condition, two tests were conducted for each specimen and the data presented and analysed in this paper are average results.
4. Mathematical models
The cooling efficiency of evaporative air cooling is measured by the saturation effectiveness or the evaporative saturation efficiency (η) (ASHRAE Standard, ANSI/ASHRAE Standard 133–2001). It is determined primarily by the measured temperatures of the air entering and exiting the rigid media using the following equation:
A mathematical expression of mass and heat balances with the evaporative saturation efficiency on the evaporative cooling rigid media may be written for the system as follows (Holman Citation1980, 1982):
The solid media can be considered to simulate a heat exchanger. Consequently the heat or mass transfer coefficient can be calculated with log mean temperature difference (ΔT) or density difference of water vapour (Δρ
v
) to proxy q and me
in Equations Equation(2) and Equation(3)
we obtain
A correlation form describing the convective heat transfer associated with cross flow direct evaporative cooling can be found in the form of a general empirical equation:
A correlation form of mass transfer occurring in a given flow regime, proceeding at similar rates, can also be found in empirical form:
The definitions of the above‐noted dimensionless numbers are expressed as follows:
For rigid cellulose evaporative media, Dowdy et al. (Citation1986) and Dowdy and Karabash (Citation1988) reported correlations to determine the convective heat and mass transfer coefficients as follows:
The friction factor calculation can be determined from the fundamental equation for computing the pressure drop of a fluid flowing through a rectangular straight duct which is:
The above correlations were used to validate our results and the test methodology.
5. Results and discussion
Although tests were conducted under different inlet conditions (see Table ), subjective and reasonable analysis could be conducted based on such different system parameters as saturation efficiency, pressure drop and the electricity consumption of fans.
Table 1. Typical test data for coconut coir types 1 and 2 and paper pad.
The average measured dry‐bulb temperature, air relative humidity and fan electrical power consumption for the different air supply velocities used in the tests for the three evaporative pad types tested are summarised in Table . The water temperature was assumed equal to the wet‐bulb temperature. The wet‐bulb temperature was calculated using the following equations from ASHRAE 41.6‐1994 (Citation1994). Using all the recorded raw data, an uncertainty analysis was conducted following ASHRAE Guideline 2‐Citation1986 (RA 90) (1986) with a confidence level of 95%.
5.1 Saturation effectiveness, pressure drop and energy consumption
Figure indicates that the cooling efficiency or saturation effectiveness for different pads decreased when the air velocity increased. Saturation effectiveness for Coconut coir type 2 (CC2) was the highest; it varied from 51.53 to 48.69% (the average cooling efficiency was 50.46%) compared with the commercial paper pad for which the saturation effectiveness varied from 49.17 to 46.29% (the average saturation effectiveness was 47.49%). The lowest saturation effectiveness was presented by Coconut coir type 1 (CC1) and varied from 45.66 to 44.07% (the average saturation effectiveness was 44.96%). It can be concluded that the coconut pads performed in a similar manner to the commercial paper pad which is an encouraging finding. The difference in cooling efficiency value between the three beds is due to the difference in the inlet conditions (see Table ): the lower the air dry‐bulb inlet temperature and humidity ratio, the cooler the cooling water temperature and the higher the pad cooling efficiency. When the velocity of air circulated through the cooling pad increases, the efficiency decreases because the static pressure drop increases, as shown in Figure . This effect is also illustrated in Figure which presents the pressure drop across the three pads versus the air face velocity, i.e. velocity of air at the pad inlets. Owing to its configuration, the coconut coir type 2 (CC2) pad exhibited the lowest pressure drop (average pressure drop of 1.5 Pa) for air face velocity ranging from 2.05 to 3.55 m s−1. For the coconut coir type 1 (CC1) pad the pressure drop increased from 2.9 to 8.1 Pa (average pressure drop of 5.1 Pa) when the air face velocity increased from 2.90 to 4.64 m s−1. The paper pad exhibited the highest pressure drop, varying between 9.1 and 12.4 Pa, for air face velocity ranging from 4.35 to 5.36 m s−1. From Table , it can be seen that to maintain the same air supply velocity for each pad configuration, the fan's electricity consumption was different. The commercial paper pad consumed the highest amount of electricity, followed by the coconut coir type 1 pad; the fan's electricity consumption was at its lowest for the coconut coir type 2 pad.
Figure 8 Variations of cooling efficiency across two coconut coir pads compared with paper pad versus air supply velocity.
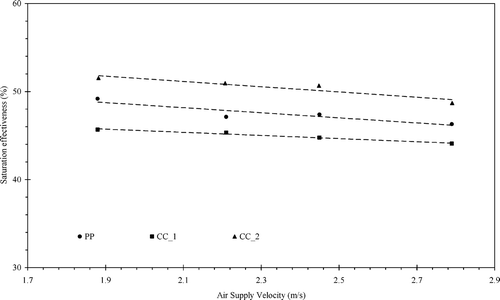
Figure 9 Variations of measured pressure drop across two coconut coir pads compared with paper pad versus air supply velocity.
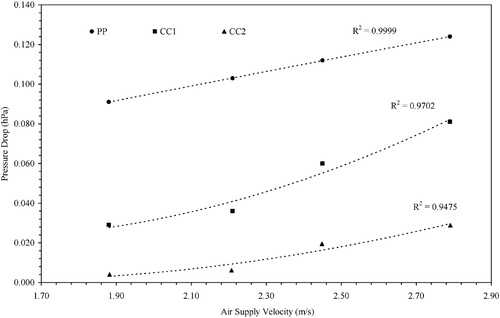
Figure 10 Variations of measured pressure drop across two coconut coir pads compared with paper pad versus air face velocity.
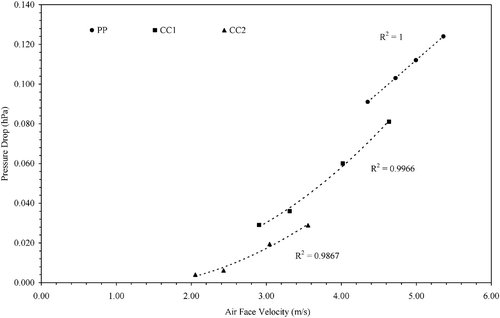
To conclude this section, and for the test conditions used here, increasing air velocity yields no significant gain in cooling efficiency. Coconut coir type 2 is the most appropriate to use as a wetted cooling pad as it presented the highest saturation effectiveness, the lowest pressure drop and the lowest fan electrical power consumption when compared with coconut coir type 1 and commercial paper pads. These experiments confirmed that coconut coir pads provide good performance when used as a wetted cooling media in a direct evaporative cooling pad.
5.2 Heat and mass transfer coefficients
Calculated heat (hH ) and mass (hM ) transfer coefficients for the two coconut coir pads and the paper pad for various air supplies are illustrated in Figures and respectively. Under operating air supply velocity varying from 1.88 to 2.79 m s−1 (the corresponding air flow rate varying between 0.027 and 0.040 m3 s−1), the hH and hM values of coconut coir pad type 2 were within the ranges of 179.9 to 246.2 W m−2 K−1 and 0.13 to 0.17 m s−1, respectively. The corresponding hH and hM values for coconut coir type 1 were within the ranges of 154.7 to 218.5 W m−2 K−1 and 0.11 to 0.14 m s−1, respectively. Those for the paper pad, determined using the same experimental arrangement, varied from 64.7 to 88.2 W m−2 K−1 and 0.05 to 0.07 m s−1, respectively.
Figure 11 Relationship between heat transfer coefficients and air supply velocity of two coconut coir pads and paper pad.
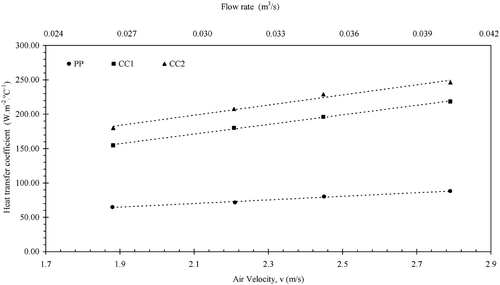
Figure 12 Relationship between mass transfer coefficients and air supply velocity of two coconut coir pads and paper pad.
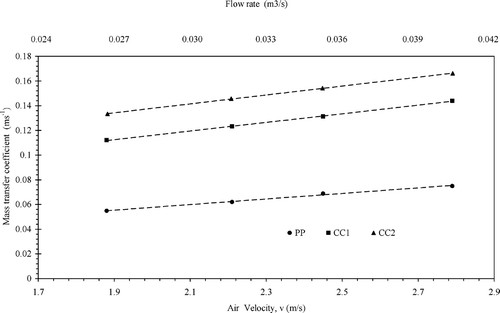
The air supply velocity obviously influences the heat and mass transfer rate which was approximately described by a linear expression. An increase in air velocity yielded better heat and mass transfer exchanges between air and the wetted pad. When comparing the three pads, the results showed that coconut coir type 2 (CC2) had the highest heat and mass transfer coefficients. It should also be pointed out that due to the pad configurations (we remind the readers that the dimensions of the three pads were the same), the contact surface area between air and the wetted surface of the coconut pads compared to the paper pad is much lower (about 60%). That explains why coconut pads have higher heat (hH ) and mass (hM ) transfer coefficients than the commercial paper pad.
5.3 Correlation of heat and mass transfer
Non‐dimensionalised heat and mass transfer data for the two coconut coir pads and paper pad are showed in Figures and , respectively. Using the experimental data, general empirical correlations for the heat and mass transfer coefficients dimensionless groups across the coconut coir pads in an evaporative cooling system are derived using simple mathematical regression techniques. These are presented in Table .
Table 2. Correlations of heat and mass transfer dimensionless groups.
The experimentally determined correlations for heat and mass transfer for the paper pad were compared with the results from Dowdy's experiment [Equations Equation(13) and Equation(14)
]. For the heat transfer correlation, it can be seen that the values for the constant C and exponent m are similar at 0.07 and 0.8 respectively. For the mass transfer correlation, the values for the constant C are fairly close, at 0.05 and 0.06, while the value for the exponent m is the same at 0.8. The small difference in C is probably due to the different testing conditions such as the difference in pad configuration and experimental arrangements. Despite this, it is reasonable to conclude that the analysis presented is valid and that the simple empirical correlation form used is acceptable.
As mentioned earlier, heat and mass transfer coefficients depend closely on the Reynolds numbers. The average values for the exponent m of coconut coir pads is approximately 0.85 and 0.6 for heat and mass correlation, respectively. The value for the constant C for coconut coir pads is higher than that for the paper pad which means that the heat and mass transfer rates are higher for coconut pads, as discussed earlier.
5.4 Friction factor for coconut coir pads
Pressure drop across wetted media in a direct evaporative cooling system is an important consideration in performance and system design. In fact, the air flow resistance of wetted media determines the air velocity through it and the resulting associated dynamic pressure decreases. Studying the effect of various configurations of media pad on pressure drop mechanism of the fluid flow requires a study of the friction factor due to wetted media whose characteristics are related to configuration and roughness of surface.
A plot of the friction factor as a function of Reynolds number is presented in Figure . The friction factor ranged from: 0.003 to 0.011; 0.024 to 0.030; and 0.074 to 0.046 respectively for coconut coir type 2, type 1 and paper pads. The friction factor for paper pads varied inversely with the Reynolds number whereas an increase in Reynolds number did not yield a significant effect on the friction factor of the coconut coir pads. This observation is due to the configuration of each pad. With its many corrugated layers, the paper pad has a higher frictional resistance than the coconut coir pads.
5.5 Analysis of the evaporative cooling potential of the coconut coir pad
To assess the potential of the coconut coir evaporative cooling pad its performance was simulated for various climatic conditions. The climatic data used were those for the central region in Thailand from 1997 to 2007 collected from 18 stations by the Thai Meteorological Department. Average monthly air temperature and relative humidity throughout the year were calculated (Table ). The following conditions were considered for coconut coir pad type 2 as the rigid media: a cooling efficiency of 50%; an air supply velocity at 1.88 m s−1; and heat and mass transfer coefficients of 180 W m−2 K−1 and 0.13 m s−1 respectively. The simulated conditions for air leaving a direct evaporative cooling system are presented in Table . The cooling path is schematically illustrated in a psychrometric chart in Figure . The black dots represent the average ambient climatic conditions of the month whereas the white ones represent those leaving the coconut coir pad. It can be seen that the reduction of air temperature varies between 23.4 and 28°C and this is an encouragement for commercial development.
Table 3. Average monthly climatic conditions throughout the year within the central region of Thailand and air conditions leaving the coconut coir pad type 2.
6. Conclusions
This experimental investigation confirmed that coconut coir demonstrated reasonable potential for use as a wetted media in evaporative cooling systems. Consequently, it creates the possibility of new sustainable engineering systems where either cooling and/or humidifying is required. Two different pad types were considered with some rows inclined at 45° and some at 6° (type 1 and type 2). When compared to commercial paper pads, under the test conditions used here, it was found that type 2 coconut coir pads had similar saturation effectiveness of about 50%, higher heat and mass transfer rates, lower pressure drop and consumed less electrical power. The accuracy and consistency of the measurements were considered to be satisfactory based on the measurement of air velocity, dry‐bulb temperature, relative humidity, and static pressure drop in transition. Correlations of dimensionless groups for heat and mass transfer coefficients expressed using Nusselt and Sherwood numbers were developed using a small evaporative cooling process arrangement. Simulation of the evaporative cooling potential of the coconut coir pad, using the average climatic conditions of the central region of Thailand, showed reasonable performance year‐round. Finally, this research showed that natural and cheap materials, such as coconut coir, can be used as a new alternative material for rigid media pads. However, although a promising alternative material it would be necessary to evaluate coconut pad production technology before proposing commercial product development.
Acknowledgements
The authors would like to thank the Thailand Research Fund (TRF) under the framework of Royal Golden Jubilee scholarship programme, the National Research Council of Thailand (NRCT) and the Commission on Higher Education (CHE) of the Ministry of Education for providing financial support to this study and to our research group.
References
- Agricultural Statistics of Thailand Crop Year . 2001/02 . Office of Agricultural Economics. . Available from: http://oae.go.th/statistic/yearbook/2001‐02/index.html
- Al‐Sulaiman , F. 2002 . Evaluation of the performance of local fibers in evaporative cooling. . Energy Conversion and Management , 43 : 2267 – 2273 .
- ASHRAE . 1983 . Fundamental handbook , New York : Evaporative Cooling and evaporative coolers, American Society of Heating Refrigeration and Air conditioning Engineer .
- ASHRAE Guideline, 2‐1986 (RA 90) . 1986 . Engineering Analysis of Experimental Data 16
- ASHRAE Standard, ANSI/ASHRAE 41.6‐1994 . 1994 . Method for measurement of moist air properties 28
- ASHRAE Standard, ANSI/ASHRAE 133‐2001 . 2001 . Method of testing direct evaporative air coolers 24
- Dowdy , J. A. and Karabash , N. S. 1988 . Experimental determination of heat and mass transfer coefficients in rigid impregnated cellulose evaporative media. . ASHRAE Transaction , 93, 2A : 382 – 394 .
- Dowdy , J. A. , Reid , R. L. and Handy , E. T. 1986 . Experimental determination of heat and mass transfer coefficients in aspen pad. . ASHRAE Transaction , 92, 2A : 60 – 70 .
- Holman , J. P. 1980 . Thermodynamics , 770 New York : McGraw‐Hill .
- Holman , J. P. 1992 . Heat transfer , 714 London : McGraw‐Hill . 7th ed
- Jain , D. and Tiwari , G. N. 2002 . Modeling and optimal design of evaporative cooling system in controlled environment greenhouse. . Energy Conversion and Management , 43 : 2235 – 2250 .
- Khedari , J. 2003 . Feasibility study of using agriculture waste as desiccant for air conditioning system. . Renewable Energy , 28, 192 : 1617 – 1628 .
- Kittas , C. , Bartzanas , T. and Jaffrin , A. 2003 . Temperature gradients in a partially shaded large greenhouse equipped with evaporative cooling pads. . Biosystems Engineering , 85 (1) : 87 – 94 .
- Ubonwat , J. 2001 . “ A feasibility study of using water hyacinth as wetted media in evaporative cooling system. ” . Master of Science Thesis, Energy Technology Program, King Mongkut's Institute of Technology Thonburi
- Watt , J. R. 1986 . Evaporative air conditioning handbook , 455 London : Chapman and Hall . 2nd ed
- Willits , D. H. 2003 . Cooling fan‐ventilated greenhouse: a modeling study. . Biosystems Engineering , 84 (3) : 314 – 329 .