Abstract
Over the last three decades, new concepts, strategies, frameworks and systems have been developed to tackle the sustainable development issue. This paper reviews the challenges, perspectives and recent advances in support of sustainable production operations decision-making. The aim of this review is to provide a holistic understanding of advanced scientific analysis methodologies for the evaluation of sustainability, to provide efficient decision support. Over 100 publications have been analysed, and a characterisation of state-of-the-art sustainability analysis methodologies has been produced, which includes life cycle assessment and multi-criteria decision analysis (MCDA), along with their applications to three key areas of production operations: sustainable design, sustainable manufacture and sustainable supply chain management. Distribution of existing work is discussed and future research directions are elicited from the literature. The paper finds three trends in supporting sustainable production operations decisions: (a) sustainability analysis has moved to whole life cycle assessment from single-stage assessment, (b) sustainability analysis has shifted away from single criterion to MCDA and (c) sustainability analysis has evolved from stand-alone approaches to integrated systematic methodologies. The paper concludes that integrated sustainability analysis can provide more efficient and effective support to complex decision-making in sustainable production operations.
1. Introduction
Sustainability, or sustainable development, was first defined by the World Commission on Environment and Development as the ‘development that meets the needs of present generations while not compromising the ability of future generations to meet their needs’ (WCED Citation1987). It is believed that the first consideration of sustainability can be traced back to practices of many ancient philosophers, although the concept of sustainability only entered modern literature in the 1970s (Linton et al. Citation2007). However, the definition of sustainability published by WCED (Citation1987) elevated it from a set of technical concepts into the political, and subsequently business, mainstream. Not surprisingly, it has since been recognised as one of the greatest challenges facing the world (Ulhoi Citation1995, Wilkinson et al. Citation2001, Bateman Citation2005, Espinosa et al. Citation2008).
Common values for achieving sustainability have been articulated in a recent review (Lindsey Citation2011). For development to be sustainable, it is essential to integrate environmental, social and economic considerations into the action of greening operations (i.e. the transformation processes that produce usable goods and services) (Handfield et al. Citation1997, Kelly Citation1998, Gauthier Citation2005, Lee and Klassen Citation2008), because operations have the greatest environmental impacts among all business functions of a manufacturer (Rao Citation2004, Nunes and Bennett Citation2010). In the context of sustainable development, operations have to be understood from a network perspective, in which operations include not only manufacturing, but also design and supply chain management (SCM) activities across products, processes and systems (Geldermann et al. Citation2007, Allesian et al. 2010). Without proper consideration of inter-relationships and coherent integration between different operations activities, sustainability objectives cannot be achieved (Sarkis Citation2003, Zhu et al. Citation2005). In the past, there was a lack of true integration between environmental and operations management, because environmental management was viewed simply as a narrow corporate legal function, primarily concerned with reacting to environmental legislation. Subsequently, managerial actions focused on buffering the operations function from external forces to improve efficiency, reduce cost and increase quality (Hill Citation2001, Taylor and Taylor Citation2009). More recently, green operations management has been investigated from a more integrative perspective, instead of a constraint perspective, in which environmental management is viewed as an integral component of an enterprise's operations systems (Yang et al. Citation2010). This means that the research foci have shifted to the exploration of the coherent integration of environmental and operations management through business managers' proactive decision-making (Liu et al. Citation2010).
Many decisions have to be made for green production operations in relation to sustainable design, manufacture and SCM (Sarkis Citation2003, Galasso et al. Citation2009). Slack et al. (Citation2010) classify strategic operations decisions into two main categories: structural and infrastructural. Structural decisions are those which primarily influence design activities, such as those relating to new product design and supply network design, whereas infrastructural decisions are those which influence manufacturing planning and control, and management improvements, such as inventory management and supplier development. Miltenburg (Citation2005) defines six production decision areas: production planning and control, organisation structure and control, human resource, facilities, sourcing and process technology. This classification scheme was also adopted by Choudhari et al. (Citation2010), and 54 decision types were further identified under the 6 decision areas with 113 decision choices suggested for the decision types. However, the papers did not provide discussion on how the optimal decision choice could be reached for each decision type.
To allow the optimal decision choice to be made, decision makers have to be able to define appropriate decision criterion or criteria for decision problems (Anderson et al. Citation2009). On some occasions, a single decision criterion can be used. For example, an economic objective (minimum cost or maximum profit) has been widely used as the sole criterion in traditional production operations decision-making. However, when environmental regulations are getting tougher and public awareness of the green issues is getting stronger, companies have to adopt other decision criteria aside from economic objective. The concept of the triple bottom line (3BL), which encompasses economic, environmental and social sustainability, has been widely recognised as offering general guidelines to sustainable development decision-making (Lu et al. Citation2007).
Multi-criteria decision-making is challenging. There is a broad consensus that decision evaluation holds the key to reaching an optional decision choice, especially under complex decision situations (Mehrabad and Anvari Citation2010). Decision evaluation enables decision makers to perform scientific analysis; to weigh, score and rank the alternatives against decision criteria and to assess the consequences of each decision alternative, so that optimal decision choices become more transparent to decision makers (Karsak and Ahiska Citation2008, Chituc et al. Citation2009). In recent years, sustainability analysis has emerged as an important decision aid to provide efficient and effective decision evaluation in all aspects of business (Hodgman Citation2006). Sustainability analysis is important to production research because decision-making in sustainable operations often has high complexity, cost and risk. Getting the decisions right will generate not only considerable economic value, but also great environmental and social impacts, which can sharpen a company's competitive edge (Noran Citation2010).
To the authors' best knowledge, there has, so far, been no comprehensive review that addresses sustainability analysis for complex decision-making in the sustainable operations context, although reviews on general green operations management have been available (Bloemhof-Ruwaard et al. Citation1995, Angell and Klassen Citation1999, Kleindorfer et al. Citation2005, Craighead and Meredith Citation2008). Therefore, this paper examines, for the first time, existing work in the area from a holistic point of view, discussing the sustainable operations decisions from both life cycle and multi-criteria analysis perspectives. Major contributions include: (a) producing a classification scheme to systematically link disparate work in sustainability analysis for green production operations decision-making, which will help researchers to better understand the current status of research in this area and (b) eliciting potential research trends that provide direction for future research. The paper focuses solely on production operations decision-making (Pun Citation2006, Bayraktar et al. Citation2007) and so does not cover service operations such as banking, education or medical services. The boundary of this work is life cycle assessment support for multi-criteria decision-making problems within production operations. Therefore, other eco-efficiency technologies such as environmental accounting, value analysis, eco-indicators, lifetime design strategies (LiDS) wheel and materials, energy, toxicity (MET) matrix are outside the scope of this paper. Decision-making situations where only a single decision criterion is required are also not within the scope of this review. The next section of the paper reviews advanced sustainability analysis methodologies. Section 3 examines how the sustainability analysis methodologies have been explored to support sustainable production operations decision-making. Distribution of existing work and future research directions are discussed in Sections 4 and 5, respectively, before conclusions are drawn in Section 6.
2. Sustainability analysis methodologies
Decision analysis would be theoretically straightforward if the key interacting variables and boundaries of responsibilities were well understood by decision makers (Mihelcic et al. Citation2003). Unfortunately, such situations are rare, whereas benefits from sustainability efforts have been elusive. Practitioners continue to grapple with how sustainability analysis should be undertaken, due to the complexities and uncertainties of the environmental systems involved and the imperfections of human reasoning (Allenby Citation2000, Hertwich et al. Citation2000). According to Hall and Vredenburg (Citation2005), innovating for sustainable development is usually ambiguous. When it is not possible to identify key parameters or when conflicting pressures are difficult to reconcile, such ambiguities make traditional risk assessment techniques unsuitable. Researchers further argue that sustainability analysis frequently involves a wide range of stakeholders, many of whom are not directly involved with the company's production operations activities. Decision makers are thus likely to have significant difficulties in reaching the right decisions if efficient support is not available. Powerful systematic analysis methodologies have great potential to guide decision makers to see through the complexities and ambiguities (Matos and Hall Citation2007). This section discusses two types of the most influential analysis methodologies that could facilitate efficient and effective sustainable production operations decision-making: life cycle analysis (LCA) and multi-criteria decision analysis (MCDA).
2.1 Life cycle analysis
Life cycle assessment or analysis (LCA) is regarded as a ‘cradle to grave’ technique that can support environmentally friendly product design, manufacture and management (Hunkeler et al. Citation2003, Verghese and Lewis Citation2007, Jose and Jabbour Citation2010). It can be used to assess the environmental aspects and potential impacts associated with a product, process or system (Matos and Hall Citation2007, Kim et al. Citation2010). It also allows decision makers to evaluate the type and quantity of inputs (energy, raw materials, etc.) and outputs (emissions, residues, other environmental impacts, etc.) of production operations to completely understand the context involving product design, production and final disposal (Fuller and Ottman Citation2004, Jose and Jabbour Citation2010). Standards for the application of LCA have been set up by International Standards Organisation (ISO). A four-stage LCA process has been defined (ISO Citation14040 1997), as illustrated in Figure .
Life cycle analysis can be conducted along two different dimensions: product life cycle (PLC) and operational life cycle (OLC) analysis. A new product progresses through a sequence of stages from development and introduction to growth, maturity and decline. This sequence is known as the PLC (Sarkis Citation2003, Bevilacqua et al. Citation2007, Gunendran and Young Citation2010). On the other hand, OLC includes stages of procurement, production, packaging, distribution, use, end-of-life (EOL) disposal and reverse logistics (Nunes and Bennett Citation2010, Yuan and Gao Citation2010). The nature of both of these analytical tools can generate important insights into environmentally conscious practices in organisations, and there are certainly interdependencies between PLC and OLC. For example, in the PLC introduction phase, procurement is more influential than production for sustainable practices, whereas in the maturity and decline stages of the PLC, efficient EOL and reverse logistics are more influential than distribution operations. It is also not difficult to understand that distribution decisions, such as facility locations and modes of transportation, will influence both the forward and the reverse logistics networks (Bayazit and Karpak Citation2007, Chan et al. Citation2010). However, it is widely acknowledged that environmental methods (LCA, PLC and OLC analysis) should be ‘connected’ with social and economic dimensions to help address the 3BL and that this is only meaningful if they are applied to support the decision-making process and are not just a ‘disintegrated’ aggregation of facts (Matos and Hall Citation2007). It is advantageous that both PLC and OLC analyses are collectively conducted to obtain a more holistic picture of the economic and ecological impacts of production operations (Neto et al. Citation2010). Figure shows the relationships between LCA, PLC and OLC, and how they can be used together to support sustainable production operations decision making.
2.2 Multi-criteria analysis
There is no doubt that sustainable production operations decision-making has multiple criteria to meet simultaneously, i.e. environmental, social and economic performance objectives. The production operations decisions can envelop both quantitative and qualitative, and tangible and intangible factors. The methodology that can empower decision makers to consider all the decision criteria and decision factors, resolve the conflicts between them and arrive at justified trade-offs is the well-known MCDA (Thawesangskulthai and Tannock Citation2008). Over the past three decades, different variants of MCDA have been developed. This section compares four important MCDA methods: analytical hierarchy process (AHP), analytic network process (ANP), fuzzy set theory and fuzzy AHP/ANP.
The AHP was introduced by Saaty (Citation1980) for solving unstructured problems. Since its introduction, AHP has become one of the most widely used analysis methods for MCDA. AHP requires the decision maker to provide judgements about the relative importance of each criterion and specify a preference for each decision alternative using each criterion. The output of AHP is a prioritised ranking of the decision alternatives based on the overall performance expressed by the decision maker (Lee Citation2009). The key techniques to successfully implement AHP include developing a goal-criteria-alternatives hierarchy, pairwise comparisons of the importance of each criterion and preference for each decision alterative, and mathematical synthesisation to provide an overall ranking of the decision alternatives. The strength of AHP is that it can handle situations in which the unique subjective judgements of the individual decision maker constitute an important part of the decision-making process (Anderson et al. Citation2009). However, its key drawback is that it does not take into account the relationships between the decision factors.
The ANP is an evolution of AHP (Saaty and Vargas Citation2006). Given the limitations of AHP such as sole consideration of one-way hierarchical relationships among decision factors, failure to consider interaction between the various factors and ‘rank reversal’, ANP has been developed as a more realistic decision method. Many decision problems cannot be built within the hierarchical constraints of AHP because of dependencies (inner/outer) and influences between and within clusters (goals, criteria and alternatives). ANP provides a more comprehensive framework to deal with decisions without making assumptions about the independence of elements between different levels and within the same level. In fact, ANP uses a network without the need to specify hierarchical levels (Sarkis Citation2003, Dou and Sarkis Citation2010) and allows both interaction and feedback within clusters of elements (inner dependence) and between clusters (outer dependence). Such interaction and feedback best captures the complex effects of interplay in sustainable production operations decision-making (Gencer and Gurpinar Citation2007). Both ANP and AHP derive ratio scale priorities for elements and clusters of elements by making paired comparisons on a common property or criterion. The disadvantages of ANP arise when the number of decision factors and respective inter-relationships increase, requiring increasing effort by decision makers. Saaty and Vargas (Citation2006) suggested the usage of AHP to solve the problem of independence between decision alternatives or criteria, and the usage of ANP to solve the problem of dependence among alternatives or criteria.
Both AHP and ANP share the same drawbacks: (a) with numerous pairwise comparisons, perfect consistency is difficult to achieve. In fact, some degree of inconsistency can be expected to exist in almost any set of pairwise comparisons. (b) They can only deal with definite scales in reality, i.e. decision makers are able to give fixed value judgements to the relative importance of the pairwise attributes. In fact, decision makers are usually more confident, giving interval judgements rather than fixed value judgements (Kahraman et al. Citation2010). Furthermore, on some occasions, decision makers may not be able to compare two attributes at all due to the lack of adequate information. In these cases, a typical AHP/ANP method will become unsuitable because of the existence of fuzzy or incomplete comparisons. It is believed that if uncertainty (or fuzziness) of human decision-making is not taken into account, the results can be misleading.
To deal quantitatively with such imprecision or uncertainty, fuzzy set theory is appropriate (Huang et al. Citation2009a, Citation2009b, Kahraman et al. Citation2010). Fuzzy set theory was designed specifically to mathematically represent uncertainty and vagueness and to provide formalised tools for dealing with the imprecision intrinsic to multi-criteria decision problems (Beskese et al. Citation2004, Mehrabad and Anvari Citation2010). The main benefit of extending crisp analysis methods to fuzzy techniques is that it can solve real-world problems, which have imprecision in the variables and parameters measured and processed for the application (Lee Citation2009).
To solve decision problems with uncertainty and vague information in which decision makers cannot give fixed value judgements, while also taking advantage of the systematic weighting system presented by AHP/ANP, many researchers have explored the integration of AHP/ANP and fuzzy set theory to perform more robust decision analysis. The result is the emergence of an advanced analytical method – fuzzy AHP/ANP (Huang et al. Citation2009a, Citation2009b, Sen et al. Citation2010). Fuzzy AHP/ANP is considered as an important extension of conventional AHP/ANP (Kahraman et al. Citation2010). A key advantage of fuzzy AHP/ANP is that it allows decision makers to flexibly use a large evaluation pool including linguistic terms, fuzzy numbers, precise numerical values and ranges of numerical values. Hence, it offers the ability to supply more comprehensive evaluations to provide more effective decision support (Bozbura et al. Citation2007).
The relationship between (or evolution of) the MCDA methods is diagrammatically shown in Figure . The two axes indicate the levels of interactions and uncertainty which the MCDA methods can deal with. Details of the key features, strengths and weaknesses of different MCDA methods are compared in Table .
Table 1 Comparison between the MCDA methods.
3. Application of sustainability analysis methodologies in green production operations
This section examines how sustainability analysis methodologies have been explored to support integrated decision-making in three key areas of the sustainable production operations: sustainable design, sustainable manufacturing and sustainable SCM.
3.1 Sustainability analysis to support sustainable design decisions
The growing interest in ‘sustainable development’ has led many companies to examine the ways in which they deal with environmental issues during their design of products, processes, systems and supply chains. Design for environment (DfE) has, therefore, become an increasingly important topic for academic research. DfE has been defined as the systematic consideration of design performance with respect to environment, health and safety objectives over the full PLC and OLC (Ray and Guzzo 1993, Ding et al. Citation2009, Kim et al. Citation2010). The aim of DfE is the reduction of a product's environmental impact without creating a negative trade-off with other design criteria, such as costs and functionality (Grote et al. Citation2007). In earlier years, DfE was very technically focused, but it has gradually evolved to affect every aspect of business and the entire supply chain. The evolution has taken three major phases (Johansson et al. Citation2007):
• | Phase 1 – start-up period in the early 1990s, during which DfE was introduced into companies through projects with specific focus on environmental issues. | ||||
• | Phase 2 – consolidation period in mid 1990s, during which environmental science and methodology formed the basis of the DfE activities. Even though technical activities were still at the core, an initial understanding was gained that the drivers for environmental concern should be analysed from a business perspective. | ||||
• | Phase 3 – business-integrated DfE. This phase acknowledges that management of DfE is the real key to success, i.e. the DfE efforts should be embedded into all business activities. |
The benefits of integrating environmental impacts into the design of products, processes, systems and supply chains at early development stage have been well identified. Such an approach helps in reducing emissions and waste, avoids excessive use of energy or non-renewable energy sources, offers proof of a sense of responsibility towards the consumer and improves the market position of the firm (Rahimifard and Clegg Citation2007). However, it is perceived that DfE ‘principles’ are without value unless considered within a specific context.
Most literature on sustainability analysis to support DfE decision-making discusses product design. MCDA techniques such as AHP and ANP have been widely used to support decisions at early product design stage such as product screening (Calantone et al. Citation1999) and preliminary design (Lee et al. 2010). Studies on the application of environmental principles and directives to sustainable design have been targeted at complex products such as automobiles, electrical and electronic equipment, and energy-using products (Grote et al. Citation2007, Johansson et al. Citation2007). Techniques such as LCA, PLC and OLC analyses have also been extensively used to assist in determining how to design a product to minimise environmental impact over its useable life and afterwards (Pennington et al. Citation2004, Linton et al. Citation2007). Gao et al. (Citation2010) explored the utilisation of function, cost and environmental performance as primary decision-making factors for scheme selection in green design. References are available for prescribing the process of designing environmentally friendly products, e.g. ISO/TR Citation14062 (2001). However, it appears that there has been little discussion on integrating both MCDA and LCA methods for sustainability analysis in product design (Bevilacqua et al. Citation2007).
There is relatively little existing work addressing the sustainable design decision-making issue in the design of supply chains, as most often the assumption is that product quality and environmental sustainability are not directly affected by supply chain designs. However, in some industries such as the consumer goods and food industry, especially in the context of shorter life cycle products, supply chain design is a key factor in product quality and environmental sustainability (Matthews et al. Citation2006). Thus, effective supply chain design decision-making is intrinsic to sustainable design success (van der Vorst et al. Citation2009).
A key decision issue in supply chain design is supply chain configuration, concerning the number of echelons required, the number of facilities per echelon, re-order policy to be adopted by the echelons, assignment of a market region to the locations and the selection of suppliers for materials, components and sub-assemblies. Recently, a network optimisation modelling framework and an MCDA algorithm have provided to compute solutions to sustainable supply networks design (Nagurney and Nagurney Citation2010). Analysis is centred on the evaluation of the effects of different configurations on the resulting total supply chain performance. Decision criteria that have been used include supply chains costs, the bullwhip effect, quality improvement initiatives, lead time reduction and environmental impacts (Bottani and Montannari Citation2010). MCDA is the predominant method used to support the green supply chain design decision-making, but there are some pilot investigations integrating MCDA and LCA for a more robust decision analysis. For example, a fuzzy AHP analysis has been successfully integrated with LCA by Lu et al. (Citation2007) to evaluate supply chain configuration alternatives.
3.2 Sustainability analysis to support sustainable manufacturing decisions
Rapid technology advancement in product development, coupled with consumer desire for newer product models, has resulted in shorter PLCs and premature disposal of products. As a result, many manufacturers are being forced to take back their products at the end of their useful life, driven by government legislation and increasing public awareness of environmental issues. This has led to sustainable, or environmentally conscious, manufacturing methods that have been receiving considerable research interest in recent years (Rawabdeh Citation2005). According to Gupta and Lambert (Citation2008), sustainable manufacturing is concerned with the development of manufacturing methods and technologies that comply with environmental legislation and requirements considering all phases in a product's life cycle. Jose and Jabbour (Citation2010) summarised the fundamental aims of sustainable manufacturing as ‘4Rs’ – reduce, reuse, recycle and remanufacture. These aims have been addressed to some extent through various manufacturing strategies, such as manufacturing processes and product disassembly.
Manufacturing processes were in the centre of the earliest sustainable manufacturing initiatives and programmes. Since the 1980s, governments and industries have tended to focus their environmental policies and programmes towards addressing process-related environmental impacts resulting from, for example, cutting, welding and painting processes (Kim et al. Citation2010). Decision making focuses on the optimisation of manufacturing processes to reduce waste (solid/liquid), energy use (electricity and water), air emissions and noise. As a result, environmentally questionable processes can be substituted (Rao Citation2004).
Recently strengthened environmental regulations, such as those relating to energy-using products and waste electrical and electronic equipment, have driven manufacturing companies to strive for improved environmentally conscious treatment of EOL products. Disassembly has been the focus of EOL discussion for some time. From a business perspective, environmentally conscious treatment induces additional cost, hence EOL treatment and disassembly decisions have to balance the criteria between environmental value and economic performance (Kang et al. Citation2010). To assess economic and environmental value, an EOL decision maker needs to find out the optimal, feasible processes by which a product can be disassembled and remanufactured.
To help decision makers evaluate the environmental consequences of alternative manufacturing processes, disassembly and remanufacturing choices, the effective sustainability analysis methods reviewed in Section 2, LCA and MCDA, have been explored. A quantitative PLC model for strategic decision-making in the remanufacturing sector has been presented in Hu and Bidanda (Citation2009). Kim et al. (Citation2010) used the LCA methodology to evaluate painting process alternatives for sustainable manufacturing decision-making, through a case study in forklift production. Similarly, Cabrera et al. (Citation2010) discussed decision support for processes in the automotive industry with a case study of rubber extrusion. In the case studies, the entire life cycle of the products, from raw material extraction to EOL disposition, was considered.
Fuzzy set theory has been widely used to support sustainable manufacturing decision-making because of the complex, uncertain and dynamic nature of the decision situations. Kulak and Kahraman (2005) applied fuzzy set theory to the decisions related to the acquisition of automated manufacturing systems. In Mehrabad and Anvari (Citation2010), provident measures are presented, which enable decision makers to consider not just the effect of current changes but also the effect of future changes in the decision-making process. An integrated methodology proposed by Rao (Citation2009) collectively used fuzzy AHP and LCA to address the environmental impact of the interrelated decisions that are made at various stages of product life. The integrated multi attribute decision-making methodology enabled the effective evaluation of sustainable manufacturing programmes for producing a given product. A wide range of decision criteria have been used in the above discussions: cost reduction (financial and ecological costs), energy and water conservation, and minimisation of overall output of waste. Most literature addressed the green production issues from the regulatory compliance point of view, but did not include employee health and safety as key decision factors.
3.3 Sustainability analysis to support sustainable SCM decisions
As the concept of ‘Sustainable Development’ was introduced by the WCED, researchers in SCM started to bind environmental sustainability to SCM (Lee et al. Citation1997, Mentzer et al. Citation2007, Chan et al. Citation2010). It was noted that ‘corporate environmental management becomes potentially fallacious without the contribution of SCM to accomplish superior performance’ (Wu and Dunn Citation1995). Many researchers have undertaken both theoretical and empirical studies to explore the concepts, models and frameworks for sustainable SCM (SSCM) (Jayaraman et al. Citation2007, Svensson Citation2007). Over time, SSCM has gone through many different names because it lacked a clear definition, such as environmental SCM and green SCM (Geoffrey et al. Citation2001, Tsoulfas and Pappis Citation2006). Consensus on the definition of SSCM was finally reached through the most influential work in the field by Carter and Rogers (Citation2008), in which they stated ‘SSCM is the strategic, transparent integration and achievement of an organisation's environmental, social, and economic goals in the systematic co-ordination of key inter-organisational business processes for improving the long-term economic performance of the individual company and its chains’. Researchers and industrial practitioners have learnt that the challenge of SSCM is to integrate the environmental dimension into the context of supply chain manager's decision-making; as Gonzalez-Benito and Gonzalez-Benito (Citation2006) noted, ‘almost all environmental improvements possibly undertaken by a company depend on the contribution of SCM to their execution (implementation of decisions)’.
SSCM is sometimes referred to as closed-loop SCM. Closed-loop supply chains are those supply chains in which care is taken of items once they are no longer desired or can no longer be used. A closed-loop supply chain consists of a forward chain and a reverse chain (Yuan and Gao Citation2010). In the forward chain, raw materials are transformed into new products, distributed to and used by customers. In the reverse chain, used products are recycled, reused, repaired or remanufactured (Hoek Citation1999, Simpson et al. Citation2007). Increasing legislation in the field of producer responsibility and take-back obligations, and setting up collection and recycling systems have led to a strong focus on closed-loop SCM. The primary objective of closed-loop supply chains is to improve the maximum economic benefit from the end-of-use products, whereas SSCM requires the co-ordination of the social, environmental and economic dimensions. However, closed-loop supply chains are regarded as environmentally friendly by mitigating the undesirable environmental footprint of supply chains. Therefore, closed-loop supply chains are assumed to be sustainable almost by definition (Huang et al. Citation2009a, Citation2009b, Neto et al. Citation2010). Nevertheless, to maximise the profit for a closed-loop supply chain and to manage the co-ordination of the social, environmental and economic performance objectives, decision-making in SSCM has been further complicated for both decentralised and centralised decision-making, which requires efficient support from advanced sustainability analysis.
Table summarises some of the most recent work, investigating sustainability analysis methodologies for SSCM decisions. From Table we can see that a wide range of SSCM decisions are addressed, including decisions on partner selection (Crispim and Sousa Citation2009), purchasing (insourcing and outsourcing) (Gunasekaran and Irani Citation2010), packaging (Verghese and Lewis Citation2007), transportation (Yang et al. Citation2005), upstream and downstream integration (Vachon and Klassen Citation2006) and reverse logistics (Meade and Sarkis Citation2002, Bottani and Rizzi Citation2006, Erol et al. Citation2010). Because of the various decision criteria considered, SSCM decisions are among the most complex multi-criteria decision issues that production operations can encounter. Therefore, both life cycle tools (such as LCA, PLC and OLC) and MCDA methods (e.g. AHP, fuzzy set theory, fuzzy AHP and ANP) have been widely explored to perform robust decision evaluation for effective decision making.
Table 2 Comparison of different MCDA methods used to support decision making in SSCM.
An under-addressed area in SSCM decision-making is the inclusion of ethics values as decision criteria so that principles of corporate actions and behaviour can be integrated into management alternative actions (Cruz and Matsypura Citation2009, Svensson Citation2009).
4. Discussion
This paper has surveyed and classified over 100 publications in sustainability analysis to support green production operations decision-making. Figure shows the classification scheme that is used in this section to discuss the distribution of existing work, to compare interests from different areas and to identify possible future research directions.
Figure 4 Classification of sustainability analysis methodologies and their applications (corresponding section numbers are in brackets).
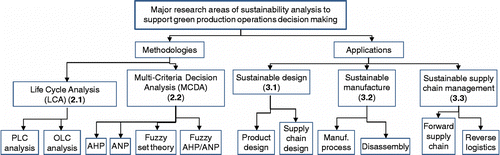
The first comparison has been undertaken through the application of sustainability analysis methodologies (i.e. LCA and MCDA) to the three areas (sustainable design, sustainable manufacture and SSCM) of sustainable production operations decision-making. The number of the publications is represented by the height of the column bars in Figure . The Figure shows that research on using both LCA and MCDA to support decision-making in all three areas has been actively carried out (with a significant number of publications in each area). However, LCA methodology is primarily explored for sustainable design (with 18 publications) and is rarely utilised for SSCM decision-making (with only two publications). MCDA is widely used in all three areas, especially in SSCM in which it has been extensively investigated as the main decision support methodology (39 publications using MCDA compared with 2 using LCA).
Figure illustrates the differentiation between the analysis methods within each category, i.e. LCA and MCDA. The majority of LCA work is on the OLC analysis. Among the 23 publications surveyed in relation to LCA, over half of them (13 out of 23) are on OLC. Only three publications (constituting 13%) collectively used both OLC and PLC analyses. Within the MCDA methodology (with 71 publications on the topic surveyed), ANP has received substantial attention with an impressive 25% (19 out of the 71) despite its relatively short history. AHP undoubtedly is the most widely used method, because of its long history and maturity as well as user friendliness (easy to implement). Fuzzy set theory and fuzzy AHP/ANP have also gained increasing research interests.
The second comparison is carried out between different journal categories that have actively published articles about green production operations. On the basis of the publications surveyed in this paper, the top three categories of journals with the most articles included are:
• | Forty-four publications from production research journals, represented by International Journal of Production Research, International Journal of Production Economics, Journal of Cleaner Production and International Journal of Sustainable Engineering. | ||||
• | Twenty-two publications from operations management journals, represented by International Journal of Operations and Production Management, Journal of Operations Management, Production and Operations Management and European Journal of Operational Research. | ||||
• | Ten papers from SCM journals, represented by SCM: an International Journal, International Journal of Logistics Management, and International Journal of Physical Distribution and Logistics Management. |
The third comparison is carried out between different publication times. Figure shows the distribution of the publications along the time line, including 104 publications that were published over the last decade. The trend in the figure clearly indicates that there has been increasing research interests in the topic in more recent years.
5. Future research directions
With the rapid development of green production operation theories and practices, and with the importance of holistic, integrated management decision-making, the requirements for decision support are changing. There is an urgent need for realistic and effective sustainability analysis methodologies to enable management decision makers to address the changes and take advantage of the opportunities presented by the changes. There has been active research to explore the key issues and methodologies to improve decision analysis for green production operations decision-making, with many research questions still open for discussion. Future research directions include (but are not limited to):
1. | As it is now commonly accepted that environmental concerns are global issues, green production operations should be understood from both network and multi-stakeholder perspectives. The outputs of green production operations should emphasise not only delivering high-quality products and services to customers and maximising profits for the owners and investors, but also reducing the impacts from discharge and used products to environment, and increasing social benefits to other stakeholders (public, employees, communities, etc.). Therefore, sustainability decision assessment should continue to be undertaken with the whole life cycle instead of within a single, isolated stage of the life cycle. The majority of the existing work on LCA has focused on either PLC or OLC analysis. Future research needs to investigate the coherent integration of both PLC and OLC analyses, as they are effectively two sides of the same coin. A key area could be to study the inter-relationships between the stages of the two life cycles. | ||||
2. | It is a known fact that green operations decisions are multi-criteria problems, so the exploration of MCDA in sustainable design, manufacture and SSCM should be expected to hold continued interest for future research. As the environmental objectives and performance measures have been diverse and elusive, future research in decision analysis needs to address the vagueness and uncertainties of green production operations situations, at the same time accommodating business manager's subjective preferences and judgements in the decision-making process. Although existing research has started to uncover the power of fuzzy AHP/ANP in dealing with these issues, future research needs to address the trade-offs between the decision maker's subjective influence and the scientific rigour of the methodologies, i.e. where the line should be drawn so that decision maker's preferences and values will not lead to unacceptably biased decisions. | ||||
3. | Future research on sustainability analysis should abandon the traditional, stand-alone, individual decision optimisation and pursue global integration and optimisation. In the 1990s and early 2000s, many firms turned inwards to focus on efficiency and integration of their sustainable design and manufacturing processes. SSCM initially emphasised local optimisation of supply chain activity. However, because of the dysfunctional consequences that local optimisation can have, there is a need for companies to balance their entire supply chain through holistic decision-making and strategic operations decisions. Subsequently, decision criteria should aim to consider net revenue maximisation, total emissions minimisation and total risk minimisation, not just for the focal operations within a company but also for the whole supply chain, i.e. to include the supplier's suppliers and customer's customers (Liu and Young Citation2004, Stonebraker and Liao Citation2004). To manage the complexity of global decision-making, it is suggested that both MCDA and LCA methodologies be explored coherently to improve the decision makers' scientific judgements. |
6. Conclusions
Sustainability analysis methodologies have been widely explored to provide effective, insightful and powerful support for complex decision-making in sustainable production operations. It is essential that an integrated approach is taken to tackle the sustainability issues from both life cycle and multi-criteria perspectives. Applying the LCA and MCDA methodologies to green production operations, decision-making has never been a straightforward issue, because decision makers can be easily swamped by the complexity of the decision situations and the minutiae of evaluation techniques for an accurate analysis.
This paper has reviewed over one hundred recent publications in three key areas of sustainable production operations: sustainable design, manufacture and SCM. The key contribution of this review is that it provides a holistic understanding of the key challenges, perspectives and recent advances of sustainability analysis methodologies in support of decision-making in green production operations. In particular, the key features, strengths and weakness of different sustainability analysis methodologies have been compared and contrasted. A classification of the key issues addressed by existing work using the LCA and MCDA methodologies has been produced. On the basis of a critical analysis of literature, future research directions have been suggested. It is highlighted that sustainable development principles and practices should be integrated into the holistic decision-making of production operations based on systematic sustainability analysis.
Additional information
Notes on contributors
Mike Leat
1Melanie Hudson Smith
2Notes
1. Email: [email protected]
2. Email: [email protected]
References
- Allenby , B.R. 2000 . Implementing industrial ecology: the AT&T matrix system . Interfaces , 30 ( 3 ) : 42 – 54 .
- Allesian , S. 2010 . Performance measurement in supply chains: new network analysis and entropic indexes . International Journal of Production Research , 48 ( 8 ) : 2297 – 2321 .
- Anderson , D.R. 2009 . An introduction to management science: quantitative approaches to decision-making , London : Cengage Learning EMEA .
- Angell , L.C. and Klassen , R.D. 1999 . Integrating environmental issues into the mainstream: an agenda for research in operations management . Journal of Operations Management , 17 : 575 – 598 .
- Bateman , N. 2005 . Sustainability: the elusive element of process improvement . Journal of Operations Management , 25 ( 3 ) : 261 – 276 .
- Bayazit , O. and Karpak , B. 2007 . An analytical network process-based framework for successful total quality management: an assessment of Turkish manufacturing industry readiness . International Journal of Production Economics , 105 : 79 – 96 .
- Bayraktar , E. 2007 . Evolution of operations management: past, present and future . Management Research News , 30 ( 11 ) : 843 – 871 .
- Beskese , A. , Kahraman , C. and Irani , Z. 2004 . Quantification of flexibility in advanced manufacturing systems using fuzzy concept . International Journal of Production Economics , 89 ( 1 ) : 45 – 56 .
- Bevilacqua , M. , Ciarapica , F.E. and Giacchetta , G. 2007 . Development of a sustainable product lifecycle in manufacturing firms: a case study . International Journal of Production Research , 45 ( 18–19 ) : 4073 – 4098 .
- Bloemhof-Ruwaard , J.M. 1995 . Interactions between operational research and environmental management . European Journal of Operational Research , 85 : 229 – 243 .
- Bozbura , F.T. , Beskese , A. and Kahraman , C. 2007 . Prioritisation of human capital measurement indicators using fuzzy AHP . Experts with Applications , 32 ( 4 ) : 1100 – 1112 .
- Bottani , E. and Montannari , R. 2010 . Supply chain design and cost analysis through simulation . International Journal of Production Research , 48 ( 15 ) : 2859 – 2886 .
- Bottani , E. and Rizzi , A. 2006 . A fuzzy TOPISIS methodology to support outsourcing of reverse logistics services . Supply Chain Management: An International Journal , 11 ( 4 ) : 294 – 308 .
- Byrne , M.R. and Polonsky , M.J. 2001 . Impediments to customer adoption of sustainable transportation: alternative fuel vehicles . International Journal of Operations and Production Management , 21 ( 12 ) : 1521 – 1538 .
- Cabrera , C.B. 2010 . A data-driven manufacturing support system for rubber extrusion lines . International Journal of Production Research , 48 ( 18 ) : 2219 – 2231 .
- Calantone , R.J. , Di Benedetto , C.A. and Schmidt , J.B. 1999 . Using the hierarchy analytic process in new product screening . Journal of Production Innovation Management , 16 ( 1 ) : 65 – 76 .
- Carter , C. and Rogers , D. 2008 . A framework of sustainable supply chain Management – moving toward new theory . International Journal of Physical Distribution and Logistics Management , 38 ( 5 ) : 360 – 387 .
- Chan , H.K. , Yin , S. and Chan , F.T.S. 2010 . Implementing just-in-time philosophy to reverse logistics systems: a review . International Journal of Production Research , 48 ( 21 ) : 6293 – 6313 .
- Chituc , C.M. , Azevedo , A. and Toscano , C. 2009 . Collaborative business frameworks comparison, analysis and selection: an analytic perspective . International Journal of Production Research , 47 ( 17 ) : 4855 – 4885 .
- Choudhari , S.C. , Adil , G.K. and Ananthakumar , U. 2010 . Congruence of manufacturing decision areas in a production system: a research framework . International Journal of Production Research , 48 ( 20 ) : 5963 – 5989 .
- Craighead , C.W. and Meredith , J. 2008 . Operations management research: evolution and alternative future paths . International Journal of Operations and Production Management , 28 ( 8 ) : 710 – 726 .
- Crispim , J.A. and Sousa , J.P. 2009 . Partner selection in virtual enterprises: a multi-criteria decision support approach . International Journal of Production Research , 47 ( 17 ) : 4791 – 4812 .
- Cruz , J.M. and Matsypura , D. 2009 . Supply chain networks with corporate social responsibility through integrated environmental decision-making . International Journal of Production Research , 47 ( 3 ) : 621 – 648 .
- Ding , L. , Davis , D. and McMahon , C.A. 2009 . The integration of lightweight representation and annotation for collaborative design representation . Research in Engineering Design , 19 ( 4 ) : 223 – 234 .
- Dou , Y. and Sarkis , J. 2010 . A joint location and outsourcing sustainability analysis for a strategic off-shoring decision . International Journal of Production Research , 48 ( 15 ) : 567 – 592 .
- Erol , I. 2010 . Exploring reverse supply chain management practices in Turkey . Supply Chain Management: An International Journal , 15 ( 1 ) : 43 – 54 .
- Espinosa , A. , Harnden , R. and Walker , J. 2008 . A complexity approach to sustainability – Stafford Beer revisited . European Journal of Operational Research , 187 : 636 – 651 .
- Fuller , D.A. and Ottman , J.A. 2004 . Moderating unintended pollution: the role of sustainable product design . Journal of Business Research , 57 ( 11 ) : 1231 – 1238 .
- Galasso , F. , Merce , C. and Grabot , B. 2009 . Decision support framework for supply chain planning with flexible demand . International Journal of Production Research , 47 ( 2 ) : 455 – 478 .
- Gao , Y. 2010 . Selection of green product design scheme based on multi-attribute decision-making method . International Journal of Sustainable Engineering , 3 ( 4 ) : 277 – 291 .
- Gauthier , C. 2005 . Measuring corporate social and environmental performance: the extended life-cycle assessment . Journal of Business Ethics , 59 ( 1–2 ) : 199 – 206 .
- Geldermann , J. , Treitz , M. and Rentz , O. 2007 . Towards sustainable production networks . International Journal of Production Research , 45 ( 18–19 ) : 4207 – 4224 .
- Gencer , C. and Gurpinar , D. 2007 . Analytical network process in supplier selection: a case study in an electronic firm . Applied Mathematical Modelling , 31 : 2475 – 2486 .
- Geoffrey , J.L.F. , Hagelaar , J.G. and van der Vorst , A.J. 2001 . Environmental supply chain management: using life cycle management to structure supply chains . International Food and Agribusiness Management Review , 4 : 399 – 412 .
- Gonzalez-Benito , J. and Gonzalez-Benito , O. 2006 . The role of stakeholder pressure and managerial values in the implementation of environmental logistics practices . International Journal of Production Research , 44 ( 7 ) : 1353 – 1373 .
- Grote , C.A. 2007 . An approach to the EuP Directive and the application of the economic eco-design for complex products . International Journal of Production Research , 45 ( 18–19 ) : 4099 – 4117 .
- Gunasekaran , A. and Irani , Z. 2010 . Modelling and analysis of outsourcing decisions in global supply chains . International Journal of Production Research , 48 ( 2 ) : 301 – 304 .
- Gunendran , A.G. and Young , R.I.M. 2010 . Methods for the capture of manufacture best practice in product lifecycle management . International Journal of Production Research , 48 ( 20 ) : 5885 – 5904 .
- Gupta , S.M. and Lambert , A.J.D. 2008 . Environmental conscious manufacturing , Boca Raton, FL : CRC Press, Taylor and Francis Group LLC .
- Hall , J. and Vredenburg , H. 2005 . Managing the dynamics of stakeholder ambiguity . MIT Sloan Management Review , 47 ( 1 ) : 11 – 13 .
- Handfield , R.B. 1997 . Green value chain practices in the furniture industry . Journal of Operations Management , 15 : 293 – 315 .
- Hertwich , E. , Hammitt , J. and Pease , W. 2000 . A theoretical foundation for life-cycle assessment . Journal of Industrial Ecology , 4 ( 1 ) : 13 – 28 .
- Hill , M.R. 2001 . Sustainability, greenhouse gas emissions and international operations management . International Journal of Operations and Productions Management , 21 ( 12 ) : 1503 – 1520 .
- Hodgman , K.L. 2006 . The sustainability analysis framework: an effective knowledge communication tool in a whole of government context . Systems, Cybernetics and Information , 5 ( 3 ) : 64 – 69 .
- Hoek , R.I. 1999 . From reversed logistics to green supply chains . Supply Chain Management , 4 ( 3 ) : 129 – 134 .
- Hu , G. and Bidanda , B. 2009 . Modeling sustainable product lifecycle decision support systems . International Journal for Production Economics , 122 ( 1 ) : 366 – 375 .
- Huang , C.T. 2009a . A fuzzy AHP-based performance evaluation model for implementing SPC in the Taiwanese LCD industry . International Journal of Production Research , 48 ( 18 ) : 5163 – 5183 .
- Huang , X.Y. , Yan , N.N. and Qiu , R.Z. 2009b . Dynamic models of closed-loop supply chain and robust H control strategies . International Journal of Production Research , 47 ( 9 ) : 2279 – 2300 .
- Hunkeler , D. 2003 . Life cycle management , Pensacola, FL : SETAC Publications .
- ISO 14040 . 1997 . Environmental management – life cycle assessment: principles and framework , Irvine, CA. Washington, DC : National Academy Press . Japan Workshop on Industrial Ecology, 1–3 March 1997
- ISO/TR 14062, 2001. Environmental management – integrating environmental aspects into product design and development. Distributed through American National Standards Institute (ANSI). ASIN: B000Y2TYPQ.
- Jayaraman , V. , Klassen , R. and Linton , J.D. 2007 . Supply chain management in a sustainable environment . Journal of Operations Management , 25 : 1071 – 1074 .
- Johansson , G. , Greif , A. and Fleischer , G. 2007 . Managing the design/environment interfaces: studies of integration mechanisms . International Journal of Production Research , 45 ( 18–19 ) : 4041 – 4055 .
- Jose , C. and Jabbour , C.J.C. 2010 . In the eye of the storm: exploring the introduction of the environmental issues in the production function in Brazilian companies . International Journal of Production Research , 48 ( 21 ) : 6315 – 6339 .
- Kahraman , C. , Beskese , A. and Kaya , I. 2010 . Selecting among ERP outsourcing alternatives using a fuzzy multi-criteria decision-making methodology . International Journal of Production Research , 48 ( 2 ) : 547 – 566 .
- Kang , C.M. 2010 . Automatic derivation of transition matrix for end-of-life decision-making . International Journal of Production Research , 48 ( 11 ) : 3269 – 3298 .
- Karsak , E.F. and Ahiska , S.S. 2008 . Improved common weight MCDM model for technology selection . International Journal of Production Research , 46 ( 24 ) : 6933 – 6944 .
- Kelly , K.L. 1998 . A systems approach to identify decisive information for sustainable development . European Journal of Operational Research , 109 : 452 – 464 .
- Kim , J. 2010 . Sustainable manufacturing: a case study of the forklift painting process . International Journal of Production Research , 48 ( 10 ) : 3061 – 3078 .
- Kleindorfer , P.R. , Singhal , K. and Wassenhove , L.N.V. 2005 . Sustainable operations management . Production and Operations Management , 14 ( 4 ) : 482 – 492 .
- Kulak , O. and Kahraman , C. 2005 . Multi-attribute comparison of advanced manufacturing systems using fuzzy vs. crisp axiomatic design . International Journal of Production Economics , 95 : 415 – 424 .
- Kumar , S. , Zampogna , P. and Nansen , J. 2010 . A closed loop outsourcing decision model for developing effective manufacturing strategy . International Journal of Production Research , 48 ( 7 ) : 1873 – 1900 .
- Lee , A.H.I. 2010 . An evaluation framework for product planning using FANP, QFD and multi-choice goal programming . International Journal of Production Research , 48 ( 13 ) : 3977 – 3997 .
- Lee , A.H. 2009 . A fuzzy AHP evaluation model for buyer-supplier relationship with the consideration of benefits, performance, costs and risks . International Journal of Production Research , 45 ( 15 ) : 4255 – 4280 .
- Lee , S.Y. and Klassen , R.D. 2008 . Drivers and enablers that foster environmental management capabilities in small- and medium-sized suppliers in supply chains . Production and Operations Management , 17 ( 6 ) : 573 – 586 .
- Lee , H. , Padmanabhan , V. and Whang , S. 1997 . Information distortion in a supply chain: the bullwhip effect . Management Science , 43 ( 4 ) : 546 – 558 .
- Lindsey , T.C. 2011 . Sustainable principles: common values for achieving sustainability . Journal of Cleaner Production , 19 ( 5 ) : 561 – 565 .
- Linton , J.D. , Klassen , R. and Jayaraman , V. 2007 . Sustainable supply chains: an introduction . Journal of Operations Management , 25 : 1075 – 1082 .
- Liu , S. 2010 . Integration of decision support systems to improve decision support performance . Knowledge and Information Systems – an International Journal , 22 ( 3 ) : 261 – 286 .
- Liu , S. and Young , R.I.M. 2004 . Utilizing information and knowledge models to support global manufacturing co-ordination decisions . International Journal of Computer Integrated Manufacturing , 17 ( 6 ) : 479 – 492 .
- Lu , L.Y.Y. , Wu , C.H. and Kuo , T.C. 2007 . Environmental principles applicable to green supplier evaluation by using multi-criteria decision analysis . International Journal of Production Research , 45 ( 18–19 ) : 4317 – 4331 .
- Matos , S. and Hall , J. 2007 . Integrating sustainable development in the supply chain: the case of life cycle assessment in oil and gas and agricultural biotechnology . Journal of Operations Management , 25 : 1083 – 1102 .
- Matthews , J. 2006 . A constraint-based approach to investigate the ‘process flexibility’ of food processing equipment . Journal of Computers and Industrial Engineering , 51 ( 4 ) : 809 – 820 .
- Meade , L. and Sarkis , J. 2002 . A conceptual model for selecting and evaluating third-party reverse logistics providers . Supply Chain Management: An International Journal , 7 ( 5 ) : 283 – 295 .
- Mehrabad , M.S. and Anvari , M. 2010 . Provident decision-making by considering dynamic and fuzzy environment for FMS evaluation . International Journal of Production Research , 48 ( 15 ) : 4555 – 4584 .
- Mentzer , J.T. , Myers , M.B. and Stank , T.P. 2007 . Handbook of global supply chain management , Thousand Oaks, CA : Sage Publications .
- Mihelcic , J.R. 2003 . Sustainability science and engineering: the emergence of a new meta-discipline . Environmental Science and Technology , 37 : 5314 – 5324 .
- Miltenburg , J. 2005 . Manufacturing strategy – how to formulate and implement a winning plan , Portland, OR : Productivity Press .
- Nagurney , A. and Nagurney , L.S. 2010 . Sustainable supply chain network design: a multi-criteria perspective . International Journal of Sustainable Engineering , 3 ( 3 ) : 189 – 197 .
- Neto , J.Q.F. 2010 . From closed-loop to sustainable supply chains: the WEEE case . International Journal of Production Research , 48 ( 15 ) : 4463 – 4481 .
- Noran , O. 2010 . A decision support framework for collaborative networks . International Journal of Production Research , 47 ( 17 ) : 4813 – 4832 .
- Nunes , B. and Bennett , D. 2010 . An environmental reports analysis and benchmarking study . Benchmarking: An International Journal , 17 ( 3 ) : 396 – 420 .
- Pennington , D.W. 2004 . Life cycle assessment, part 2, current impact assessment practice . Environment International , 30 : 721 – 739 .
- Pun , K.P. 2006 . Determinants of environmentally responsible operations: a review . International Journal of Operations and Production Management , 23 ( 3 ) : 279 – 297 .
- Rahimifard , S. and Clegg , A.J. 2007 . Aspects of sustainable design and manufacture . International Journal of Production Research , 45 ( 18 ) : 4013 – 4019 .
- Rao , P. 2004 . Greening production: a South-East Asian experience . International Journal of Operations and Production Management , 24 ( 3 ) : 289 – 320 .
- Rao , R.V. 2009 . An improved compromise ranking method for evaluation of environmentally conscious manufacturing . International Journal of Production Research , 47 ( 16 ) : 4399 – 4412 .
- Rawabdeh , I.A. 2005 . A model for the assessment of waste in job shop environments . International Journal of Operations and Production Management , 25 ( 8 ) : 800 – 822 .
- Ray , D.L. and Guzzo , L.R. 1993 . Environmental overkill: whatever happened to common sense? , Washington, DC and Lanham, MD: Regnery Gateway
- Saaty , T.L. 1980 . The analytical hierarchy process , New York : McGraw-Hill .
- Saaty , T.L. and Vargas , L.G. 2006 . Decision making with the analytic network process , New York : Springer .
- Sarkis , J. 2003 . A strategic decision framework for green supply chain management . Journal of Cleaner Production , 11 : 397 – 409 .
- Sen , C.G. , Sen , S. and Baslihil , H. 2010 . Pre-selection of suppliers through an integrated fuzzy analytic process and max-min technology . International Journal of Production Research , 48 ( 6 ) : 1603 – 1625 .
- Simpson , D. , Power , D. and Samson , D. 2007 . Greening the automotive supply chain: a relationship perspective . International Journal of Operations and Production Management , 27 ( 1 ) : 28 – 48 .
- Slack , N. , Chambers , S. and Johnston , R. 2010 . Operations management , 6th ed. , London : FT Prentice Hall .
- Stonebraker , P.W. and Liao , J. 2004 . Environmental turbulence, strategic orientation: modelling supply chain integration . International Journal of Operations and Production Management , 24 ( 10 ) : 1037 – 1054 .
- Svensson , G. 2007 . Aspects of sustainable SCM: conceptual framework and empirical example . Supply Chain Management – An International Journal , 12 ( 4 ) : 262 – 266 .
- Svensson , G. 2009 . The transparency of SCM ethics: conceptual framework and empirical illustrations . Supply Chain Management – an International Journal , 14 ( 4 ) : 259 – 269 .
- Taylor , A. and Taylor , M. 2009 . Operations management research: contemporary themes, trends and potential future directions . International Journal of Operations and Production Management , 29 ( 12 ) : 1316 – 1340 .
- Thawesangskulthai , N. and Tannock , J.D.T. 2008 . A decision aid for selecting improvement methodologies . International Journal of Production Research , 46 ( 23 ) : 6721 – 6737 .
- Tsoulfas , G. and Pappis , C.P. 2006 . Environmental principles applicable to supply chain design and operations . Journal of Cleaner Production , 14 : 1593 – 1602 .
- Ulhoi , J.P. 1995 . Coporate environmental and resource management: in search of a new managerial paradigm . European Journal of Operational Research , 80 : 2 – 15 .
- Vachon , S. and Klassen , R.D. 2006 . Extending green practices across the supply chain . International Journal of Operations and Production Management , 26 ( 7 ) : 795 – 821 .
- van der Vorst , J.G.A.J. , Tromp , S.Q. and van der Zee , D.J. 2009 . Simulation modelling for food supply chain re-design: integrated decision-making on product quality, sustainability and logistics . International Journal of Production Research , 47 ( 23 ) : 6611 – 6631 .
- Verghese , K. and Lewis , H. 2007 . Environmental innovation in industrial packaging: a supply chain approach . International Journal of Production Research , 45 ( 18–19 ) : 4381 – 4401 .
- Wang , Y.M. , Chin , K.S. and Leung , J.P.F. 2009 . A note on the application of the data envelopment analytic hierarchy process for supplier selection . International Journal of Production Research , 47 ( 11 ) : 3121 – 3138 .
- WCED . 1987 . Our common future: the world commission on environment and development , Oxford : Oxford University Press .
- Wilkinson , A. , Hill , M. and Gollan , P. 2001 . The sustainability debate . International Journal of Operations and Production Management , 21 ( 12 ) : 1492 – 1502 .
- Wu , H.J. and Dunn , S.C. 1995 . Environmental responsible logistics systems . International Journal of Physical Distribution and Logistics Management , 25 ( 2 ) : 20 – 38 .
- Yang , B. , Yang , Y. and Wijngaard , J. 2005 . Impact of postponement on transport: an environmental perspective . International Journal of Logistics Management , 16 ( 2 ) : 131 – 156 .
- Yang , C.L. 2010 . Mediated effect of environmental management on manufacturing competitiveness: an empirical study . International Journal of Production Economics , 123 ( 1 ) : 210 – 220 .
- Yuan , K.F. and Gao , Y. 2010 . Inventory decision-making models for a closed-loop supply chain system . International Journal of Production Research , 48 ( 20 ) : 6155 – 6187 .
- Zhu , Q. , Sarkis , J. and Geng , Y. 2005 . Green supply chain management in China: pressures, practices and performance . International Journal of Operations and Production Management , 25 ( 5 ) : 449 – 468 .