Abstract
Solar cooker is an alternate device used for reducing the problems of energy supply for cooking needs, especially in rural households. Though solar cookers have been commercialised in rural and other domestic areas, further research is still required to enhance the efficiency of the solar cooker. Previous studies have helped to ascertain that the central annular cylindrical hole in the cooking vessel reduces the length of the heat-transfer path and provides higher heat-transfer rate to the water kept in the vessel. Convective heat transfer can be further increased by providing additional surface area in the cylindrical hole. This study proposes a new cooking vessel with central annular cavity and fins attachment that will help in increasing the heat-transfer rate to the cooking vessel. Experiments are conducted with three types of vessels, i.e. conventional cooking vessel, cooking vessel with central annular cavity and cooking vessel with rectangular fins in the central annular cavity. For the annular cavity with fins vessel, a peak temperature of 98°C is observed. The maximum temperature difference of 30°C has been observed between annular cavity vessel with fins and conventional vessel. As the height of the lug is also expected to play an important role, experiments are conducted to determine the effect of lug height on the heat transfer to the cooking vessel. This study indicates that a lug height of 9 mm is optimum for maximum heat transfer to the cooking vessel.
Nomenclature
φ | = |
diameter of central annular cavity |
LAT | = |
local apparent time |
V1 | = |
conventional cooking vessel |
V2 | = |
cooking vessel with central annular cavity |
V3 | = |
cooking vessel with central annular cavity and fins |
1. Introduction
Solar cooker has much potential for use in many regions throughout the world, especially in areas that experience a good intensity of solar radiation. In the last four decades, several types of solar cookers, such as box type, concentrator type and oven type, have been designed, developed and tested around the world. Schwarzer and da Silva (Citation2008) reviewed the basic characteristics and experimental procedures to test the different types of solar cookers and to compare the thermal performance of solar cookers. Of the different types of solar cookers, only box-type solar cookers have been disseminated at the mass level in India and in other developing countries. A significant amount of work has been carried out since the nineteenth century by several investigators on different designs of solar cookers to bring about improvement in their performance. A box-type solar cooker with a single reflector at the hood was designed by Tiwari and Yadav (Citation1986). To avoid the convection heat losses during the loading and unloading of the vessels in the oven, the lid could be situated at the base of the oven. By introducing the concept of the lid at the base of the oven, they solved the problems of preheating. Narasimha Rao et al. (Citation1988) investigated the effect of elongation (ratio of length/width of aperture) of rectangular apertures provided with one single booster mirror (hinged on the northern edge of the aperture) on the energy collection pattern and concluded that the elongation has a significant bearing on the total energy collection. Narasimha Rao et al. (Citation1991) made an analysis of the energy accumulation pattern of a box-type solar cooker with a plane mirror hinged on the northern edge. The effect of latitude on the energy contribution by the mirror onto the cooker aperture was investigated. It is concluded that the energy contribution by the mirror is more during the winter solstice, which is a desirable trend. The performance of a box-type solar cooker employing booster mirrors was studied by Tiwari et al. (Citation1990) who concluded that cooking period is reduced due to enhanced solar flux on cooking surface. Narasimha Rao et al. (Citation1989) conducted experiments by providing a single adjustable mirror booster hinged on one side pointing towards the south. The total energy incident on the cooker aperture has been calculated at latitude of 18° and at five different declinations of the sun. It has been found that, by intermittent and continuous adjustment of the booster mirror, the total energy collection has improved throughout the day. Thulasi Das et al. (Citation1994a) conducted experiments by considering the parameters such as the thickness and size of the absorber plate, emissivity of the vessel, insulation thickness and cooking time. These studies indicated that the cooking time was minimum for the plates of 0.5–1.0 mm in thickness. Thulasi Das et al. (Citation1994b) presented thermal models for the box-type solar cookers loaded with one, two or four vessels. The method of Taha and Eldighidy was utilised to estimate the enhanced solar irradiance on the cooker due to the flat reflector fitted to the cooker.
A transparent insulation material is made from polyester film to produce a stagnation-proof and cost-effective cooker that has a greater chance of adoption than less efficient cookers currently made (Turner et al. Citation1998). Kumar (Citation2004) conducted experiments on box-type solar cooker to evaluate the thermal performance of a cooker, i.e. top heat loss coefficient U tw for a water-loaded cooker. A correlation is developed for the variable U tw and which is a function of pot-water temperature, wind speed and ambient air temperature. Narasimha Rao and Subramanyam (Citation2003) suggested that the bottom of the vessel could be raised above the floor of the solar cooker by means of a few point supports (known as lugs) and the net area of heat transfer to the food could be increased significantly. It is also concluded that the area of heat transfer has nearly doubled due to the additional area at the bottom. Narasimha Rao and Subramanyam (Citation2005) conducted experiments on the cooking vessel with a central annular cavity kept on lugs using water and thermic fluid (high heat-transfer fluid) as a working medium. It is observed that the central cavity increases the effective area of heat transfer to the water and hence reduces the time of cooking. Arezki et al. (Citation2008) conducted experiments in a box-type solar cooker with fins on external lateral surface and it was observed that fins improve the heat transfer from the internal hot air of the cooker towards the interior of the vessel in which the food is to be cooked. Experiments were also conducted with different food materials in box-type solar cookers. Terres et al. (Citation2007) conducted experiments in a box-type solar cooker with honey, olive oil, milk and water and the results are compared with the numerical simulation results. A low-cost solar cooker was made, and energy and exergy efficiencies of the animal feed were experimentally evaluated by Panwar et al. (Citation2010). It is found that the energy efficiency of the cooker varies between 1.12 and 29.78%, while the exergy efficiency varies between 0.07 and 1.52% during the same period.
So far, only a maximum temperature of 80°C is achieved in commercial box-type solar cookers. From the literature, it is established that there is ample scope for an increase of heat transfer to the cooking vessel through innovative designs. Therefore, in this study, in continuation of the work of Narasimha Rao and Subramanyam (Citation2005), an attempt is made to design a cooking vessel with central annular cavity and attached fins to increase the heat-transfer rate to the water in the cooking vessel. As a part of student research project, experiments are also conducted to determine the optimum lug height on which the cooking vessel is kept.
2. Experimental set-up and experimentation
Figure shows the sketch of a box-type solar cooker. The cooker consists of a square aluminium tray constructed using a 0.55 mm-thick sheet. The sides and the bottom of the tray are enclosed in a box made of sheet metal. The gap between the tray and the casing is filled with the glass wool to provide thermal insulation. The tray is provided with a movable double glass cover hinged to the one side of the casing at the top. A plain glass mirror, encased in a sheet metal shell, is fitted to serve as a reflector.
The cooking vessels are cylindrical in shape (φ = 155 mm and height = 62.5 mm) and have a flat base made of aluminium. The vessels are provided with light-fitting flat covers. The tray and the outsides of the vessels are painted with dull black paint. Figure illustrates the conventional cooking vessel (V1). Figure illustrates the cooking vessel with central annular cavity (V2). The cooking vessel consists of two parts, (i) a container and (ii) a lid. The lid is a circular aluminium plate with its circular edge bent downwards. The plate has a hollow concentric cylindrical projection (φ = 55 mm) downwards at the centre. The container consists of a cylindrical vessel with an upward projecting hollow concentric cylinder (φ = 55 mm) at the centre of the vessel. The cooking vessel with central annular cavity with fins; V3 (12 fins of size 15 × 12 × 1 mm rectangular cross section) is designed as shown in Figure . The food material to be cooked can be loaded into the annular cavity between the inner wall of the vessel and the outer wall of the cylindrical projection of the vessel. The lid can be pressed to fit snugly over the outer edge of the vessel and on the cylindrical projection of the container of the vessel. Air can pass through the central cylindrical hole of the vessel right from bottom to top, when the lid is pressed fit to the container.
Initially, the conventional vessel is kept on the base of the cooker and is loaded with a fixed quantity of water. The temperature of the water in the vessel, ambient temperature and plate temperature at preset interval of time at local area (latitude 16°10′, longitude 80°16′) are noted with the help of calibrated copper–constantan thermocouples. Observations are recorded at time intervals of 30 min. Then, the cooking vessel is kept on three aluminium lugs of 7 mm height and 10 mm diameter placed at 120° spacing as shown in Figure . Similar sets of experiments are conducted with cooking vessels of other designs. All the above experiments are conducted simultaneously on different solar cookers in the same time intervals for the purpose of comparison. The readings are obtained for a period of 23 days and the average temperatures are considered for the analysis.
3. Results and discussions
3.1 Conventional vessel on the base of cooker
When the beam radiation impinges on the top cover of the cooker, it cannot heat the food because there is an air gap between the top cover and the top layer of the food. In this arrangement, the lid first gets heated and generates a current of hot air, which then, circulates inside the cooker. The heat carried by this air circulation eventually reaches the food through the sides of the vessel.
A typical temperature–time history of the water, plate and plate wall are shown in Figure . At the beginning of the experiment, the bottom plate temperature is at 43°C. It gradually reaches 59°C at 14:00 h then the temperature again drops to 48°C. The difference between water temperature and plate temperature is found to continuously increase with time. The maximum difference between water temperature in vessel and bottom plate temperature of 36°C is observed at a local time of 15:00 h. This indicates that the heat transfer to the vessel is just not due to direct solar radiation on the plate or vessel, but it is mostly due to convection currents of air present in the solar cooker.
3.2 Conventional vessel on lugs
When the conventional vessel is placed on the floor of the cooker, the top surface (the cover) does not efficiently transfer the heat to the food, as there is an air gap between the lid and the food. The bottom of the vessel is also ineffective in transferring the heat to the food, because the vessel kept on the floor of the cooker prevents the circulation of hot air. Therefore, the bottom of the vessel is raised above the floor of the solar cooker by means of a few point supports. This enables the hot air in the cooker to circulate in the space between the floor of the cooker and the bottom of the vessel. Thus, the net area of heat transfer to the food is increased significantly. For the present vessel size, the effective areas would be 243 cm2 for the peripheral area and 189 cm2 for the bottom. Through this arrangement, the area of heat transfer is increased by 80%. A typical temperature–time history of the water, plate and plate sidewall temperature is shown in Figure . A peak pot-water temperature of 88°C is observed as shown in Figure against the peak pot-water temperature of 86°C with conventional vessel as shown in Figure .
3.3 Annular cavity vessel on lugs
Consider a cooking vessel containing food material heated from the sides. After the initial phase when the excess or free water is evaporated or absorbed, the food material can be described as a semi-solid medium. In such a case, a temperature gradient is established in the food. The temperature will have a maximum value at the wall of the cooking vessel. It decreases as the distance from the wall increases and may reach a level that is insufficient to carry forward the cooking process. This deficiency can be overcome by providing a heat source from the opposite direction. The annular cavity provides such a heat source (Narasimha Rao and Subramanyam Citation2005).
In this vessel, air can pass through the central cylindrical hole of the vessel, right from bottom to top when the lid is fitted to the container. The central annular cylindrical hole reduces the length of the heat-transfer path and facilitates better heat transfer to the water kept in the vessel. When the cooking vessel with central annular cavity is placed on the lugs, the hot air circulates through the gap between the bottom surface and the floor of the cooker and also through the central cylindrical hole in the vessel. Figure shows the temperature variation with time for annular cavity vessel on lugs. The temperature of water in the vessel, on measurement, was found to increase significantly in comparison with the previous case. It could, thus, be concluded that the introduction of a central cylindrical hole reduces the length of the heat-transfer path and brings down the cooking time in the cooking vessel.
3.4 Cooking vessel with fins on lugs
The hot air flowing through the annular cavity influences the rate of heat transfer to the water. The circulation of the hot air through the annular hole is a result of density gradient that exists between the upper part and the bottom of the cooking vessel. The amount of heat transfer from the hot air to the water in the vessel is due to the combined convection and conduction mode of heat transfer. As per Newton's law of cooling, the convection heat transfer from the hot air to the vessel also depends upon the contact surface area. The attachment of fins in the annular space can, therefore, increase surface area. These fins provide additional surface area to enable the increase of convection heat transfer. Figure shows the temperature variation with time for annular cavity vessel with fins. It is observed that water temperature in the vessel is further increased when compared with the previous case.
The water temperatures in different types of cooking vessels are compared as shown in Figure . It is noted that cooking would take place faster in the vessel on lugs when compared with the conventional arrangement of vessels. The temperatures at any time during the experimentation are high with the annular cavity vessel (V2) in comparison with the conventional vessel. It is evident that the temperature of the water kept in the vessel containing central annular cavity with fins and supported on lugs achieves the peak temperature nearly 20–30 min earlier when compared to the vessel kept on the floor of the cooker. That means cooking time is reduced considerably. Also, the temperature difference between V1 and V3 is as high as 30°C. The reduction in cooking time is due to the following factors: (i) the vessel is kept on the lugs and (ii) the enhanced heat-transfer surface area is because of the central annular cavity with fins.
Experiments are conducted with central annular cavity vessel on 7 mm lug height and these findings are compared with the literature values (Narasimha Rao and Subramanyam Citation2005) for validation. There is a reasonable agreement between the results as shown in Figure .
Figure 10 Comparison of the temperature profile of annular cavity vessel with that in the literature.
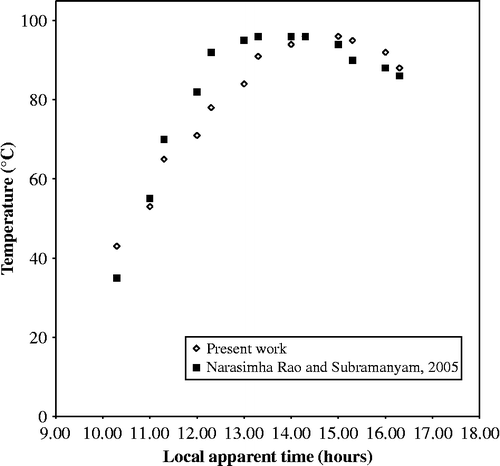
It has been established that annular cavity cooking vessel on lugs improves the performance of the cooking vessel significantly. During the experiments, the lug height was slowly increased and corresponding change in the temperatures of water was noted. As shown in Figure , with increase in lug height, the temperatures of pot water at all points of time were recorded and found to have increased continuously. This increase in the temperatures of water may be attributed to the increase in natural convection currents at the upper end and the lower end of the cooking vessel. The air near the absorber plate is hot with respect to air at the top glass cover. Due to this temperature difference, the air flows through the cylindrical hole of the vessel in addition to free convection of air inside the solar cooker. But at 10 mm of lug height, a sudden drop in the temperatures of water was observed. This phenomenon is because of reduction in temperature gradient above and below the cooking vessel as the cooking vessel is positioned close to the top glass cover due to increase in lug height. Therefore, from the present experimentation, it is recommended that a lug height of 9 mm is optimum for better heat transfer for commercial box-type solar cooker.
4. Conclusion
The hot-air circulation through the central annular cavity with fins improves the heat transfer to the water in the vessel and results in the reduction of the cooking time. The cooking vessel placed on lug helps to increase the heat transfer to the vessel; hence, it is recommended that a lug height of 9 mm is optimum for better heat transfer for commercial box-type solar cooker.
Notes
† Current address. Department of Mechanical Engineering, Gandhi Engineering College, Bhubaneswar, 752054, Orissa, India.
References
- Arezki , H. , Boukar , M. and Amar , M. 2008 . Experimental study of a double exposure solar cooker with finned cooking vessel . Solar Energy , 82 : 287 – 289 .
- Kumar , S. 2004 . Thermal performance study of box type solar cooker from heating characteristic curves . Energy Conversion and Management , 45 : 127 – 139 .
- Narasimha Rao , A.V. , Sitharama Rao , T.L. and Subramanyam , S. 1988 . Mirror boosters for solar cooker-I . Journal of Energy Conversion and Management , 28 ( 3 ) : 265 – 269 .
- Narasimha Rao , A.V. , Sitharama Rao , T.L. and Subramanyam , S. 1989 . Mirror boosters for solar cooker-II . Journal of Energy Conversion and Management , 29 ( 3 ) : 157 – 164 .
- Narasimha Rao , A.V. , Sitharama Rao , T.L. and Subramanyam , S. 1991 . Mirror boosters for solar cooker-III . Journal of Energy Conversion and Management , 32 ( 1 ) : 51 – 58 .
- Narasimha Rao , A.V. and Subramanyam , S. 2003 . Solar cookers – part I: cooking vessel on lugs . Solar Energy , 75 : 181 – 185 .
- Narasimha Rao , A.V. and Subramanyam , S. 2005 . Solar cookers – part II: cooking vessel with central annular cavity . Solar Energy , 78 : 19 – 22 .
- Panwar , N.L. , Kothari , S. and Kaushik , S.C. 2010 . Experimental investigation of energy and exergy efficiency of masonry-type solar cooker for animal feed . International Journal of Sustainable Energy , 29 ( 3 ) : 178 – 184 .
- Schwarzer , K. and da Silva , M.E.V. 2008 . Characteristic and design methods of solar cookers . Solar Energy , 82 : 157 – 163 .
- Terres , H. 2007 . “ Heating of bee honey, olive oil, milk and water in a solar cooker box type with internal reflectors ” . In Proceedings of the energy sustainability conference 1117 – 1122 . 27–30 June, Long Beach, California, USA. New York: ASME
- Thulasi Das , T.C. , Karmakar , S. and Rao , D.P. 1994a . Solar box-cooker: part I – modelling . Solar Energy , 52 ( 3 ) : 263 – 272 .
- Thulasi Das , T.C. , Karmakar , S. and Rao , D.P. 1994b . Solar box-cooker: part II – analysis and simulation . Solar Energy , 52 ( 3 ) : 273 – 282 .
- Tiwari , G.N. , Thakur , K. and Yadav , Y.P. 1990 . Evaluation of a solar cooker design with reflectors . International Journal of Sustainable Energy , 9 ( 2 ) : 93 – 99 .
- Tiwari , G.N. and Yadav , Y.P. 1986 . A new solar cooker design . Energy Conversion and Management , 26 ( 1 ) : 41 – 42 .
- Turner , M.T.A. , Marshall , R.H. and Brinkworth , B.J. 1998 . A transparent insulation material for solar cookers and collectors . International Journal of Sustainable Energy , 19 ( 4 ) : 251 – 262 .