Abstract
Phosphorus-containing moieties can be added to the ninth and/or tenth positions of a fatty chain of a methyl ester utilising an epoxide route. In this route, methyl oleate is epoxidised, and then ring opened using dibutyl phosphate to yield – among others – a dioxaphospholane-containing derivative of methyl octadecanoate. Progress of the reaction was determined by gas chromatography-mass spectrometry and compounds were identified by nuclear magnetic resonance spectroscopy. Addition of a titanium and zirconium oxide catalyst increased the yield of dioxaphospholane from 27%, observed without catalyst, to 37%. A similar reaction using bis-2-ethylhexyl phosphate gave 22% and 29% yield. However, an aryl phosphate, diphenyl phosphate, was not active in these reactions. The aliphatic compounds obtained are likely to be useful sulphur and metal-free extreme pressure lubricant additives which are highly compatible with vegetable oil-based lubricants.
Introduction
Society has always had the need for lubrication (Booser Citation1995) in which biobased oils (Hwang and Erhan Citation2002, Wan Nik et al. Citation2005, Erhan et al. Citation2008) with their friction reducing properties (Biresaw et al. Citation2003) have attracted special attention. Oils that have been subjected to chemical modification (Schneider Citation2006, Erhan et al. Citation2008, Sharma et al. Citation2008a, Citation2008b), such as epoxidation (Adhvaryu and Erhan Citation2002), hydrogenation (King et al. Citation2001, Martinelli et al. Citation2005) and thermal modification (Wang and Erhan Citation1999), have all proven useful in the production of new products.
The lubricant additive, zinc dialkyldithiophosphate (ZDDP), has been used for years in almost every application in which high loads are encountered. It is highly effective in the reduction of wear caused by metal-to-metal contact between parts. The mechanism of action (Spedding and Watkins Citation1982, Watkins Citation1982) involves the decomposition of the thiophosphate and subsequent formation of iron oxide and iron sulphide layers. Computer simulations show the possible formation of a pressure-induced cross-linked structure of zinc phosphates (Mosey et al. Citation2005) which may contribute to the performance as well.
As an additive, ZDDP has been shown to work well in vegetable oil-based lubricants as well (Asadauskas et al. Citation2010), although the additive was used at a level of 20% by weight in that study.The use of any additive at this concentration is in contrast to sustainable industry. Typically, anti-wear additive loadings are ∼2%. In addition, ZDDP is also no longer allowed in many applications due to its high sulphur content. These drawbacks leave the task of achieving this effectiveness at lower loading in a sulphur-free system, which is an attractive motivation to those wishing for sustainable industry. By covalently attaching the phosphate compound directly to the lubricant, it is hypothesised that a more effective use of phospate can be achieved. If successful, this approach would yield the additional benefit of an effective additive which is also sulphur and metal free. There is an even wider range of potential applications for such a compound, for example, as a monomer to produce fire resistant polymers (Derouet et al. Citation1996, Citation2001).
Herein, we report the synthesis of butyl dioxaphospholane and 2-ethylhexyl dioxaphospholane starting from methyl oleate, Scheme , through an epoxide route. The resultant product is expected to be a biobased lubricant additive with favourable blending characteristics for use in biobased lubricants.
Experimental
Materials
Methyl oleate (Nu check Prep, Elsyian, MN, USA, >99%), hydrogen peroxide (Sigma-Aldrich, St Louis, MO, USA, ACS Reagent, 30% Solution), formic acid (Sigma-Aldrich, 96%, ACS reagent), n-heptane (EMD, Gibbstown, NJ, USA, Omnisolve), sodium chloride (Fisher, Fairlawn, NJ, USA, ACS reagent), sodium bicarbonate (Fisher, ACS reagent), sodium hydroxide (Fisher, ACS reagent), acetonitrile (Fisher, HPLC grade), 1-butanol (Fisher, ACS reagent), titanium (IV) butoxide (Sigma-Aldrich, 97%), zirconium (IV) butoxide (Sigma-Aldrich, 80%), dibutyl phosphate (Sigma-Aldrich, 97%), bis-2-ethylhexyl phosphate (Sigma-Aldrich, 97%), diphenyl phosphate (Sigma-Aldrich, 99%) were used as received.
Synthesis of titanium/zirconium catalyst
The catalyst employed in one of the reactions reported herein was synthesised from a reaction of titanium (IV) butoxide and zirconium (IV) butoxide which were dissolved in butanol in desired molar ratios, ∼25 g total oxide in 20 ml of 1-butanol, and stirred for 20 min. Next, 50 ml of deionised water was added to yield a white precipitate. Precipitation was allowed to continue for 4 h and then the solutions were filtered and the precipitate was dried at 150°C for 96 h. Afterwards, the catalysts were then calcined at 400°C for 8 h and then cooled under nitrogen. The catalyst's Brunauer–Emmett–Teller-specific surface area was characterised by nitrogen absorption in a relative pressure range from 0.05 to 0.98 using a Quantachrome NOVA 2200e instrument (Quantachrome Instruments, Boynton Beach, FL, USA). The average pore size distributions were calculated using the Barrett–Joyner–Halenda method. All adsorption and desorption isotherms were obtained at a temperature of 77 K. A sample of 1.0 g was used for nitrogen adsorption. Before each measurement, all samples were degassed at 300°C for 3 h under vacuum to evacuate the physisorbed moisture.
Acidity was measured by temperature programmed desorption of ammonia on a CHEMBET-3000 instrument (Quantachrome Instruments, Boynton Beach, FL, USA). Approximately 0.2 g of each sample was treated around 300°C for 50 min under constant flow of helium (80 cm3 m− 1) before NH3 adsorption at 150°C. After sample purge, the spectrum was obtained under a temperature increase from 100°C up to 850°C, at a rate of 15°C min− 1. The desorbed NH3 was detected by a thermal detector (Table ). Further characterisation including X-ray diffraction, thermogravimeteric analysis and FT-IR spectroscopy was carried out as well, agreeing with the surface data. Titania and zirconia were much more crystalline than the mixed oxide. Details of further characterisation of these catalysts are available as supplementary material.
Table 1 The surface area and acidity of zirconium and titanium catalysts used in the catalytic reaction.
Gas chromatography-mass spectrometry
The gas chromatograph-mass spectrometer used was an Agilent (Santa Clara, CA, USA) 7890A gas chromatograph equipped with a 7683B series injector and a 5975C quadrapole mass detector. A Windows XP-equipped HP-Compaq DC7700 computer with a 3.39 GHz Pentium D processor and using Agilent MSD Enhanced Chemstation Version E01.00.237 controlled the instrument and acquired the processed data. The GC column used was an HP-5MS (Agilent), 30 m × 0.25 mm with film thickness 0.25 μm. A helium flow rate of ∼0.8 ml min− 1, an injection volume of 0.1 μl and a 50:1 split ratio were all used. The temperature conditions were as follows: inlet 220°C, detector 220°C, auxiliary transfer line 250°C and MSD 150°C. The initial temperature of 150°C was held for 2 min and then ramped to 280°C at 15°C min− 1 in which it was held for 20 min. The detector was run in the electron impact mode and set to scan for m/z ratios from 50 to 500 Da.
Nuclear magnetic resonance
Nuclear magnetic resonance (NMR) was carried out on a Bruker (Boston, MA, USA) Avance 500 NMR spectrometer operating at 500 MHz for 1H, 125 MHz for 13C and 202 MHz for 31P. Bruker Icon NMR software was used on a HP × 1100 Pentium 4 workstation. Peaks in the 1H and 13C spectra were referenced to tetramethyl silane (TMS) 0.00 ppm and to phosphoric acid in the 31P experiments. Simulations of 13C NMR spectra were done by ACD/Labs 6.00 ACD/CNMR predictor software, running on a Gateway Pentium 4 CPU with a 2.53 GHz processor, or on ChemDraw Ultra 11, version 11.0.1, running on a Windows XP Dell Optiplex 760 with an Intel Core 2 Duo 2.99 GHz processer.
Synthesis of epoxidised methyl oleate
Epoxidised methyl oleate (EMO) was synthesised according to prior literature methods (Findley et al. Citation1945, Schmits and Wallace Citation1954, Bunker and Wool Citation2002, Doll and Erhan Citation2005). In short, methyl oleate was placed into a round-bottomed flask and 4 equiv. of formic acid was added. The reaction mixture was cooled in an ice bath, and 2 equiv. of 30% hydrogen peroxide solution was added dropwise, over about 5 min, with continuous stirring of the solution. The ice bath was removed and the reaction was allowed to proceed. The temperature was maintained below 30°C. Reaction progress was monitored by taking aliquots, and dissolving in heptane and injecting into the GC-MS. After the reaction was done, ∼1 volume of heptane was added to help layer the solution and a separation funnel was used to remove the acid/peroxide layer. Sodium bicarbonate solution was added, shaken with the product layer and then removed. This was repeated until the solution was no longer acidic as measured by pH paper. A saturated sodium chloride solution was shaken with the product layer, removed and the product was dried on a Buchi (Flawil, Switzerland) model R-215 rotary evaporator.
Synthesis of phosphorus-containing compounds
In a small dried reaction vial, 625 mg of EMO was added to either 650 mg bis-2-ethylhexyl phosphate or 450 mg of dibutyl phosphate, depending on desired product. In the reactions where catalyst was used, it was added at this time. The reaction mixture was capped and a conical magnetic stir bar matched to the reaction vial was used. The reaction mixture was heated to the reaction temperature, 95°, 120° or 140°C, and it was allowed to run for 24–72 h. The reaction mixture was filtered using a syringe filter and further analysis was done. Reactions of twice this scale were also carried out.
Analysis of aliquots from the reaction mixture
The analyses of the reaction solutions were done by taking ∼20 μl of sample and diluting into 1 ml heptane. These solutions were injected into the GC-MS as described above. The areas of the total ion count chromatographs were used to determine the extent of the reaction.
The identification of the products was done by their mass spectra. In addition, the NMR was taken of the dioxaphospholane compound purified by the preparative high performance liquid chromatography (HPLC) method, detailed in the Separation of Products section. The 1H NMR was not assigned due to the number of signals. The 13C NMR at (125 MHz, CDCl3, δ from TMS) δ 174 (carbonyl carbon), δ 84, (carbon atoms in dioxophospholane), δ 51 (methoxy carbon), δ 64 (first carbon of butyl chain phosphate), δ 14 (two signals, end carbon of fatty chain and phosphate butyl chain), δ 20–35 (multiple signals) was expected from the simulation. A small amount of ketone was also identified in this purified product by NMR signals at δ 211 (ketone carbon) and δ 43 (carbon next to ketone). The 31P NMR (202 MHz, CDCl3, δ from phosphoric acid) at δ 18.19 and δ 18.14 corresponds to the two stereoisomers of dioxaphospholane.
Separation of products
From the material from one of the larger scale reactions, separation was accomplished by preparative HPLC. One gram sample of the mixed product was dissolved in 5 ml of acetonitrile and then injected into the chromatograph using a standard injection valve and loop. The size of column used was 375 × 16 mm, and it was packed with C18 reverse phase silica gel. Acetonitrile (100%), with a flow rate of 1 ml min− 1, was used as an eluent and fractions were collected. The eluted fractions were collected and the solvent was removed by rotary evaporation yielding materials which were analysed by GC-MS and NMR.
Results and discussion
Catalyst-free synthesis
The reaction was carried out without solvent, by mixing EMO with a series of phosphates in a 1:1 molar ratio at 120°C for 72 h. The products, as shown in Scheme , were identified by MS. The observed products in the dibutyl phosphate system closely parallel to those in the study of a similar reaction using 4,5-epoxyoctane as a substrate. Because the epoxy group of EMO is predominately in the cis configuration, butyl dioxaphospholane is observed as the main product over a possible β-hydroxy butyl phosphate. The olefinic and ketone products are the result of the loss of water and/or a facile phosphate leaving group. Also present was butyl group containing reaction products, both as a headgroup (butyl ester 1) or in the mid-chain (butyl ethers 2 and 3). The first is the expected product of a transesterification reaction between the methyl ester and the butyl ester of the phosphate. The ethers could be formed either initially at the ring opening or by a subsequent decomposition reaction of the β-hydroxy butyl phosphate. This was not investigated further. Overall, the yield of the dioxaphospholane product was 27% by area, Figure . The use of higher temperature, 140°C, lower temperature, 95°C, or 24-h reaction time did not cause any significant deviations from the original yields.
Scheme 2 The products tentatively identified by mass spectrometry from the reaction of EMO with dibutyl phosphate at 120°C for 72 h.
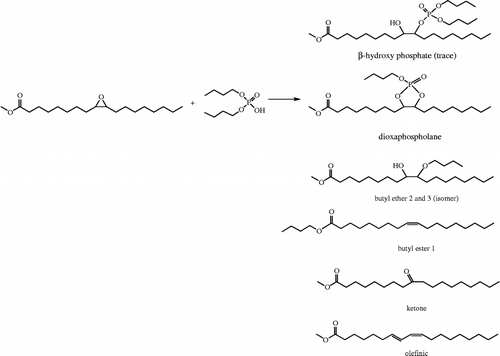
Figure 1 The distribution of the major products, shown in Scheme , from the reaction of EMO with dibutyl phosphate at 120°C for 72 h.
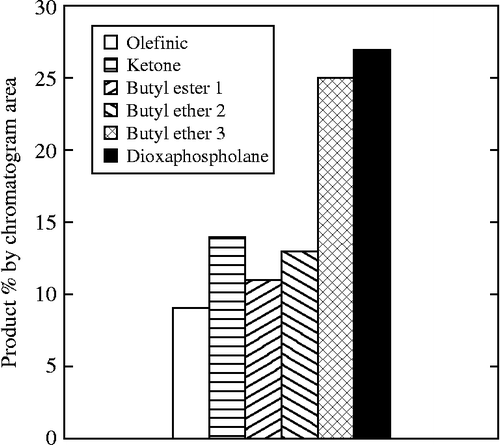
An analogous reaction was carried out using bis-2-ethylhexyl phosphate with similar results, yielding 22% of the dioxophospholane. However, we also found that under both catalytic and catalyst-free conditions, use of diphenyl phosphate as a reactant did not produce any phosphorus-containing oleochemical product, but instead produced only the olefinic and ketone products. This is perhaps due to the much higher pKa (Kumler and Eiler Citation1943, Kirby and Varvoglis Citation1967) of that phosphate.
Synthesis using a heterogeneous catalyst
Heterogeneous catalysis, due to its effectiveness and ease of product separation, has been shown to be a useful method for many reactions including the ring opening of epoxides (Fási et al. Citation1997, Bandgar et al. Citation2007, Maheswara et al. Citation2008). A series of titanium (IV) oxide and zirconium (IV) oxide catalysts were found to increase the content of the phosphorus-containing products in the observed mixture. As shown in Figure , a marginal success was realised in reactions in which the catalysts with the higher surface area, Table , were more effective. The 50/50 mol % titanium/zirconium catalyst provided the highest yield of 37%.
Further work
Further work is needed before industrial applications of the dialkyl phosphates in necessary. First, even the higher yield is not sufficient for industrial synthesis. Improvements in catalytic efficiency and reduction of side reactions are necessary to produce cost-effective additives. In addition, the effectiveness of the extreme pressure additives needs further work including studies that determine effective usage levels of the additive. Finally, potentially beneficial anti-oxidant effects of the new phosphate additive need to be studied.
Conclusions
The ring opening reaction of EMO was carried out using dialkyl phosphates. The expected heterocyclic reaction product dioxaphospholane was the dominant phosphate-containing product whereas the β-hydroxy phosphate was not observed in isolable quantity. An overall yield of 27% was obtained using butyl phosphate, and that could be improved to 37% with the use of heterogeneous titanium/zirconium catalysts.
Acknowledgements
We would like to acknowledge Jennifer R. Koch for GC-MS analysis, Daniel A. Knetzer for HPLC work and Karl E. Vermillion for NMR analysis and interpretation. This work was a part of the in-house research of the Agricultural Research Service of the United States Department of Agriculture.
Notes
†Mention of trade names or commercial products in this publication is solely for the purpose of providing specific information and does not imply recommendation or endorsement by the US Department of Agriculture. USDA is an equal opportunity provider and employer.
References
- Adhvaryu , A. and Erhan , S.Z. 2002 . Epoxidized soybean oil as a potential source of high-temperature lubricants . Industrial Crops and Products , 15 ( 3 ) : 247 – 254 .
- Asadauskas , S.J. , Biresaw , G. and McClure , T.G. 2010 . Effects of chlorinated paraffin and ZDDP concentrations on boundary lubrication properties of mineral and soybean oils . Tribology Letters , 37 ( 2 ) : 111 – 121 .
- Bandgar , B.P. 2007 . Regioselective ring opening of epoxides with thiols under solvent free and mild conditions using heterogeneous catalyst . Catalysis Communications , 8 ( 7 ) : 1065 – 1069 .
- Biresaw , G. , Adhvaryu , A. and Erhan , S.Z. 2003 . Friction properties of vegetable oils . Journal of the American Oil Chemists' Society (JAOCS) , 80 ( 7 ) : 697 – 704 .
- Booser , E.R. 1995 . “ Lubrication and lubricants ” . In Lasers to mass spectrometry , Edited by: Kroschwitz , J.I. and Howe-Grant , M. 463 – 517 . New York, NY : Wiley .
- Bunker , S.P. and Wool , R.P. 2002 . Synthesis and characterization of monomers and polymers for adhesives from methyl oleate . Journal of Polymer Science, Part A: Polymer Chemistry , 40 : 451 – 458 .
- Derouet , D. , Morvan , F. and Brosse , J.C. 1996 . Chemical modification of epoxy resins by dialkyl(or aryl) phosphates: evaluation of fire behavior and thermal stability . Journal of Applied Polymer Science , 62 ( 11 ) : 1855 – 1868 .
- Derouet , D. , Morvan , F. and Brosse , J.-C. 2001 . Chemical modification of 1,4-polydienes by di(alkyl or aryl)phosphates . European Polymer Journal , 37 ( 7 ) : 1297 – 1313 .
- Doll , K.M. and Erhan , S.Z. 2005 . Synthesis of carbonated fatty methyl esters using supercritical carbon dioxide . Journal of Agricultural and Food Chemistry , 53 ( 24 ) : 9608 – 9614 .
- Erhan , S.Z. 2008 . Lubricant base stock potential of chemically modified vegetable oils . Journal of Agricultural and Food Chemistry , 56 ( 19 ) : 8919 – 8925 .
- Fási , A. , Notheisz , F. and Bartók , M. 1997 . Transformation of cis- and trans-2,3-dimethyloxiranes on a Cu/SiO2 catalyst . Journal of Catalysis , 169 ( 1 ) : 114 – 119 .
- Findley , T.W. , Swern , D. and Scanlan , J.T. 1945 . Epoxidation of unsaturated fatty materials with peracetic acid in glacial acetic acid solution . Journal of the American Chemical Society , 67 : 412 – 414 .
- Hwang , H.-S. and Erhan , S.Z. 2002 . “ Lubricant base stocks from modified soybean oil ” . In Biobased industrial fluids and lubricants , Edited by: Erhan , S.Z. and Perez , J.M. 20 – 34 . Champaign, IL : AOCS Press .
- King , J.W. 2001 . Hydrogenation of vegetable oils using mixtures of supercritical carbon dioxide and hydrogen . Journal of the American Oil Chemists' Society (JAOCS) , 78 ( 2 ) : 107 – 113 .
- Kirby , A.J. and Varvoglis , A.G. 1967 . The reactivity of phosphate esters. Monoester hydrolysis . Journal of the American Chemical Society , 89 ( 2 ) : 415 – 423 .
- Kumler , W.D. and Eiler , J.J. 1943 . The acid strength of mono and diesters of phosphoric acid. The n-alkyl esters from methyl to butyl, the esters of biological importance, and the natural guanidine phosphoric acids . Journal of the American Chemical Society , 65 ( 12 ) : 2355 – 2361 .
- Maheswara , M. , Rao , K.S.V.K. and Do , J.Y. 2008 . Regioselective ring-opening of epoxides with amines using Zn(ClO4)2–Al2O3 as a heterogeneous and recyclable catalyst . Tetrahedron Letters , 49 ( 11 ) : 1795 – 1800 .
- Martinelli , M. 2005 . Castor oil hydrogenation by a catalytic hydrogen transfer system using limonene as hydrogen donor . Journal of the American Oil Chemists' Society (JAOCS) , 82 ( 4 ) : 279 – 283 .
- Mosey , N.J. , Muser , M.H. and Woo , T.K. 2005 . Molecular mechanisms for the functionality of lubricant additives . Science , 307 ( 5715 ) : 1612 – 1615 .
- Schmits , W.R. and Wallace , J.G. 1954 . Epoxidation of methyl oleate with hydrogen peroxide . Journal of the American Oil Chemists Society , 31 : 363 – 365 .
- Schneider , M.P. 2006 . Plant-oil-based lubricants and hydraulic fluids . Journal of the Science of Food and Agriculture , 86 ( 12 ) : 1769 – 1780 .
- Sharma , B.K. , Doll , K.M. and Erhan , S.Z. 2008a . Ester hydroxy derivatives of methyl oleate: tribological, oxidation and low temperature properties . Bioresource Technology , 99 ( 15 ) : 7333 – 7340 .
- Sharma , B.K. 2008b . One-pot synthesis of chemically modified vegetable oils . Journal of Agricultural and Food Chemistry , 56 ( 9 ) : 3049 – 3056 .
- Spedding , H. and Watkins , R.C. 1982 . The antiwear mechanism of zddp's. Part I . Tribology International , 15 ( 1 ) : 9 – 12 .
- Wang , C. and Erhan , S. 1999 . Studies of thermal polymerization of vegetable oils with a differential scanning calorimeter . Journal of the American Oil Chemists' Society (JAOCS) , 76 ( 10 ) : 1211 – 1216 .
- Wan Nik , W.B. 2005 . Rheology of bio-edible oils according to several rheological models and its potential as hydraulic fluid . Industrial Crops and Products , 22 ( 3 ) : 249 – 255 .
- Watkins , R.C. 1982 . The antiwear mechanism of zddp's. Part II . Tribology International , 15 ( 1 ) : 13 – 15 .