Abstract
Mullite-based glass-ceramics were produced from aluminium hydroxide sludge resulting from the anodisation process and waste glasses. Phase development for crystallisation of amorphous mullite was investigated between 900°C and 1200°C. The kinetic parameters such as activation energy of crystallisation and Avrami exponent for the samples were evaluated from differential thermal analysis curves using various heating rates (5–30°C) and particle size ranges ( < 45, 80–125 and 355–400 μm). The growth morphology parameters ‘n’ and ‘m’ are in the range of 2.5–5.4 and 1.5–4.4, respectively, indicating that bulk nucleation is dominant in mullite crystallisation followed by two- to three-dimensional growth of mullite crystals controlled by diffusion from a varying number of nuclei.
1. Introduction
The issue of recycling and reuse of industrial wastes plays a vital role in contributing to the conservation of resources and providing economically favourable solutions as well as promoting principles of solid waste management in the society. Many communities throughout the world are striving to develop efficient and effective plans for managing their solid waste that are generated in large volumes and may be deposited over large areas. Therefore, in order to lessen environmental loading and effectively use resources, enhancement of recycling and reuse of industrial wastes is now one of the most important environmental concerns all over the world. In addition to the environmental advantage of immobilising wastes into materials with a generally high chemical resistance, e.g. glasses, a certain economic benefit may be found in entering the large market of construction materials. Therefore, the development of novel glass-ceramic products by reuse of industrial wastes leading to materials with excellent properties, e.g. mud from zinc hydrometallurgy plant and blast furnace slags, bottom ash of municipal solid waste (MSW) as well as fly ash from domiciliary waste incinerators and coal power plants, has been the subject of many investigations (Boccaccini et al. Citation1996, Romero et al. Citation1999, Barbieri et al. Citation2000, Fredericci et al. Citation2000, Francis et al. Citation2002a, Citation2002b, Francis Citation2005, Schabbach et al. Citation2011).
Because of its potentially favourable chemical and thermomechanical properties, mullite is a promising candidate for advanced structural and functional ceramics (Tummala Citation1991, Schneider et al. Citation1993, Osendi and Baudin Citation1996, Rhanim et al. Citation1997). Over the last decades, a number of different mullite synthesis techniques, starting either from naturally occurring aluminosilicates or organometallic precursors, have been reported in the literature (Pask and Tomsia Citation1991, Liu et al. Citation1994, Mackenzie et al. Citation1996, Chen et al. Citation2000). Aluminium hydroxide has also been used as alumina sources in aluminium silicate-based mullite forming mixtures (Viswabaskaran et al. Citation2002, Citation2003, Martins et al. Citation2004, Vieira et al. Citation2007). It was mainly obtained as by-product from aluminium industry, known as aluminium sludges produced by physicochemical treatment of waste water generated by electrochemical treatment of aluminium surfaces (Vieira et al. Citation2002).
Differential thermal analysis (DTA) has been employed to study the kinetic of mullite formation from aluminium silicate glass fibre (Wei and Rongti Citation1999), diphasic gels (Boccaccini et al. Citation1999, Campos et al. Citation2002) and kaolin ceramics (Castelein et al. Citation2001, Chen et al. Citation2004a, Citation2004b) by both isothermal and non-isothermal methods. However, to our knowledge, the crystallisation kinetics and growth mechanism of mullite formation from Al-rich sludge and waste glasses have not yet been considered in this regard. In Egypt, huge amounts of Al-rich sludges are currently produced and unfortunately no interesting applications were implemented up to now. On the other hand, the high alumina contents of such residue and its compositional constancy along the time make it very attractive for use as a major component for mullite or alumina refractory bodies as well as attempting to incorporate them in other products. The purpose of this work, therefore, was to develop mullite-based glass-ceramic, made by the glass-forming technique of Al-rich sludge and common waste glass materials.
2. Experimental
The raw materials were Al-rich sludge resulting from the anodising process, soda lime and pyrex waste glasses. The sludge is produced from the Al-surface treatment processes and is generated in the etching unit of an industrial plant in Egypt. The composition of the as-received Al-rich sludge, determined by X-ray fluorescence (PW 1410 X-ray spectrometer, Philips), is 66.1% Al2O3, 4.78% SiO2, 0.046% TiO2, 0.68% CaO, 0.17% P2O5, 0.36% Fe2O3, 0.017% MnO, 0.15% MgO, 0.026% Na2O and 27.8% loss of ignition. The XRD patterns of the as-received Al-sludge and heat-treated samples at 1300°C and 1400°C are shown in Figure . Bayerite is one of the four forms of naturally occurring aluminium trihydroxide crystallites, the others are polymorphic modifications known as gibbsite, nordstrandite and doyleite (Demichelis et al. Citation2008). The thermal treatment of Al-sludge at 1300°C and 1400°C reveals diffraction lines attributed mainly to corundum at 1300°C and to both corundum and aluminium silicate at 1400°C.
Figure 1 XRD powder diffraction patterns of the (a) as-received Al-sludge, (b) Al-sludge after treatment at 1300°C and (c) after treatment at 1400°C. B, designated diffraction lines corresponding to bayerite; C, denotes diffraction lines corresponding to corundum; D, Al2SiO5 (card No. 16-602).
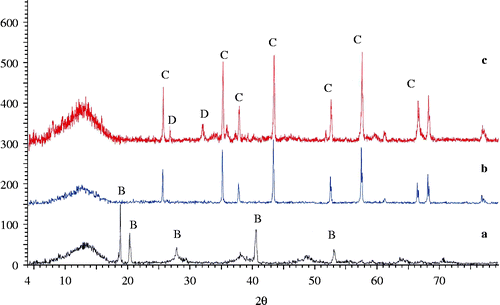
The formulation used in this study include an aluminium sludge as the main component (61%) and 39% of silica waste (Pyrex or soda lime). A glass with high alumina content is expected to exhibit a high viscosity, so additives such as CaF2 and H3BO3 should be added to facilitate the melting process. The mixtures were then melted in a graphite crucible at 1650°C for 1 h. The resulting parent glasses were cast in stainless steel mould and then subjected to crystallisation regime in a muffle furnace. The batch compositions used in this study are
(A) Al-rich waste+soda lime+20% H3BO3, | |||||
(B) Al-rich waste+Pyrex+5% CaF2+10% H3BO3, | |||||
(C) Al-rich waste+Pyrex +5% CaF2+5% H3BO3, | |||||
(D) Al-rich waste+Pyrex+10% CaF2. |
DTA was carried out on different glass compositions using a SETARAM TA 92 thermal analyser. The samples were heated from room temperature up to 1200°C at various heating rates of 5, 10, 20 and 30°C/min. The DTA scan was conducted in flowing air using platinum crucibles with calcined Al2O3 as a reference material. The morphology of the samples was observed by scanning electron microscopy (SEM) on a Jeol, JSM 5410 using an accelerating voltage of 200 kV. X-ray diffraction analysis of crystallised samples was carried out using model-Bruker AXS D8 advance with Ni-filtered Cu Kα radiation (λ = 1.54056 Å).
3. Results and discussions
Depending on the heating rate, DTA of batch (A), Figure (a), highlighted a well-defined glass transition in the range of 754–783°C followed by a marked exothermic peak over the temperature range of 878–921°C. It can be seen that all DTA traces are similar in general characteristics but with minor variations in peak positions and intensities. The exothermic peak shifts to higher temperature with increasing heating rate and this is consistent with previous work (e.g. Matsura and Suzuki Citation1979, Matusita and Sakka Citation1980).
Figure 2 DTA plots of a glass composition consisting of alumina waste+soda lime +20% boric acid (formulation A) during heating up to 1200°C (a) at different heating rate 5–30°C/min at constant particle size 80–125 μm (b) at constant heating rate 10°C/min of various particle sizes, < 45, 80–125 and 355–400 μm.
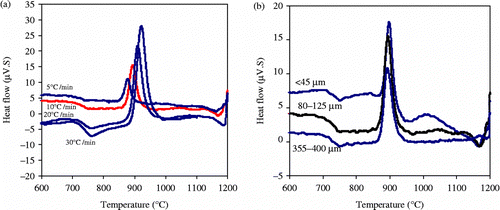
The peak crystallisation temperature as a function of the glass particle sizes ( < 45, 80–125, 355–400 μm) was monitored by heating the batch (A) through a constant heating rate of 10°C/min. There is no clear influence of particle size on the crystallisation behaviour for batch (A) composition, Figure (b). The slight variation in peak crystallisation temperature (892.2, 894.4 and 897.7°C) as a function of increasing the glass particle size reflects that the bulk crystallisation mechanism is the predominant one. Based also on the interpretation of Ozawa (Citation1971) that a broad crystallisation peak indicates a surface crystallisation, whereas a sharp one signifies bulk crystallisation, the DTA crystallisation exotherms confirm that a bulk crystallisation mechanism is predominant. It can be seen that there is a broad and clear shoulder area observed in the DTA plot with particle size ( < 45 μm), which imply that at least one different crystal phase would be formed during the heat treatment.
The evolution of crystalline phases along the crystallisation process in the temperature range of 1000–1100°C can be observed in Figure . At 1000°C, mullite has been formed, being one of the main crystalline phases, coexisting with cristobalite, α-alumina and kyanite which is a form of aluminium silicate (Al2O3√SiO2). Going up to 1100°C, the mullite phase became amorphous and the XRD pattern contained just barely detectable diffraction lines corresponding to cristobalite, α-alumina and kyanite.
Figure 3 XRD powder diffraction patterns of the formulation A at 1000°C and 1100°C for 2 h. M, mullite; K, kyanite; S, cristobalite and C, corundum.
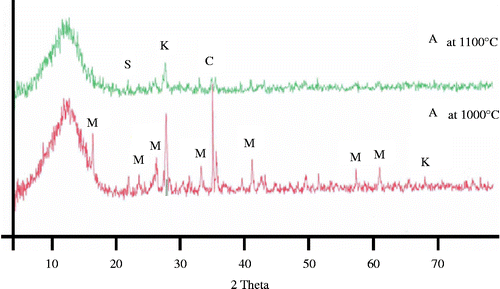
Considering the DTA crystallisation exotherms recorded at different heating rates for batch samples B and C, it is concluded that there is no clear difference between the exothermic peak positions for both batches. Figure (a),(b) also exhibits sharp peaks indicating the predominance of bulk crystallisation mechanism. It is also noticed that the well-defined exothermic peak temperature T p for different parent glass samples obeys the same trend and shifts to a higher temperature with increasing heating rate.
Figure 4 DTA plots of a glass compositions during heating up to 1200°C (a) Pyrex +5% CaF2 +10% boric acid (formulation B) at constant particle size 80–125 μm at different heating rate of 5–30°C/min (b) Pyrex +5% CaF2 +5% boric acid (formulation C) at different heating rate of 5–30°C/min at constant particle size 45–80 μm.
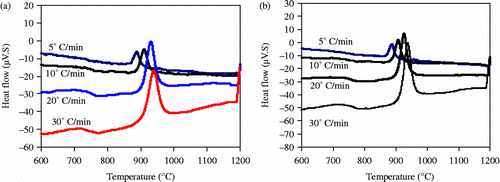
DTA for batch D in Figure highlighted the presence of two exothermic peaks, the first one occurring in the temperature range of 812–867°C and the second in the temperature range of 916–973°C. These two exothermic peaks correspond to the formation of two main crystalline phases kyanite (Al2O3√SiO2) and anorthite (CaO√Al2O3√2SiO2) as revealed in Figure . Previous work (Pena Citation1989) reported that the combination of alkaline oxides with the alumina originating from the dissociation of the mullite produces sodium alumino–silicate. Consequently, we could expect the formation of anorthite as a calcium alumino–silicate phase originating from batch D.
Figure 5 DTA plots of a glass compositions consisting of alumina waste + Pyrex +10% CaF2 (formulation D) during heating up to 1200°C at different heating rate of 5–30°C/min at constant particle size 80–125 μm.
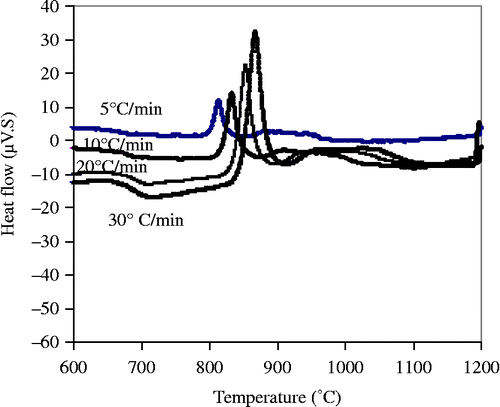
Figure 6 XRD patterns of the parent glass and various formulations treated at 1000°C for 2 h. M, mullite; K, kyanite; S, cristobalite; anor, anorthite and C, corundum.
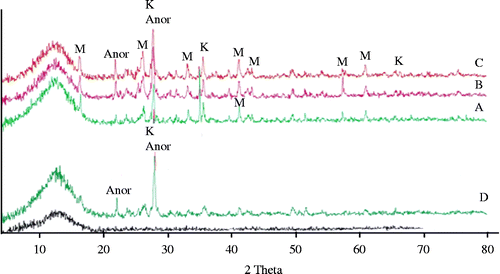
The four batch formulations were heat treated at 900°C and 1000°C (Figures and ) to evaluate the formation of mullite with other coexisting phases. The results reveal the presence of mullite and kyanite as main crystalline phases besides cristobalite. This indicates that alumina and silica wastes have been converted to mullite and kyanite under all of the heat treated and batch conditions. A main crystalline phase of mullite was obtained by heat treating the batch composition (C) at 900°C for 2 h as shown in Figure . This observation might confirm that the intensity of mullite phase increases at the expenses of kyanite as the additive glass phase-forming components decrease. The role of temperature in influencing the morphology of the crystalline phases has been illustrated in Figure , where the specimen (D) was thermal treated at 900°C and 1000°C. The morphology of the crystalline phases heat treated at 900°C for 2 h has a plate-like shape, forming a cluster of microcrystals while heat treated at 1000°C, Figure (b). We suggest that the variation in the shape of mullite crystals morphology might be due to the type of waste glass used (Pyrex, soda lime).
Figure 8 SEM micrographs of the glass-ceramic having D formulation at (a) 900°C and (b) 1000°C for 2 h.
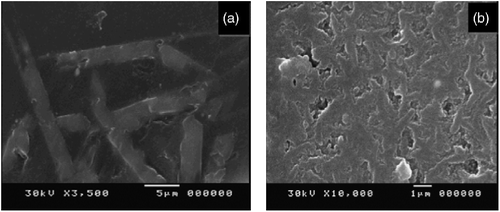
DTA has been employed for the study of the activation energy for crystallisation. The following equation proposed by Kissinger (Citation1956) was applied for this purpose:
Figure 9 Plot of Ln (Φ/T 2 p) versus 1/T p for the determination of activation energy of crystallisation of batch A at size 80–125 μm.
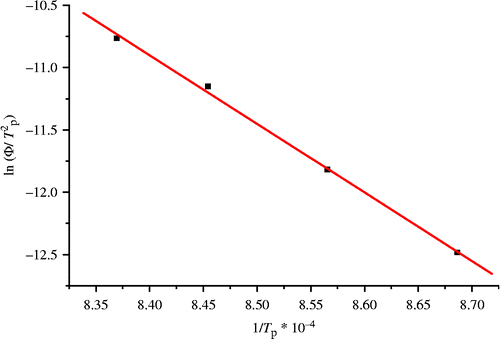
The value of the Avrami parameter ‘n’ which is a measure of the dimensionality of the transformation is determined using the Augis and Bennett equation (Citation1978):
Table summarises the activation energies E ck and corresponding activation energy calculated by the modified Kissinger model E c in the studied glasses. Vieira et al. (Citation2007) demonstrated that the non-isothermal activation energies for the phase transformations of the ratio 2:1 aluminium sludge-slate waste for primary and secondary mullite crystallisation were found equal to 431 and 454 kJ/mol, respectively. Others (Maciejewski Citation2000) suggested that the non-isothermal activation energy of mullite crystallisation in kaolin ceramics was 1182.3 kJ/mol, with an associated growth morphology parameter (n) of about 2, related to bulk nucleation and two-dimensional (2D) diffusion-controlled growth of plate-like mullite crystals.
Table 1 Kinetic parameters for crystallisation for Al–Si–oxides wastes.
The calculated activation energies for all batches are consistent with previous works (Boccaccini et al. Citation1999, Campos et al. Citation2002, Chen et al. Citation2004a, Citation2004b), while the average value of E c for batches B and C is equal to ∼388 kJ/mol according to the Kissinger model. The results of the ‘n’ values for the crystallisation peak at different heating rates are listed in Table . The growth morphology parameter ‘n’ is covering the range of 2.5–5.4 depending on the composition of the raw materials and the additives as well as the alkali impurities. These values indicate that bulk nucleation is dominant in mullite crystallisation followed by 2D to 3D growth of mullite crystals controlled by diffusion from a varying number of nuclei. However, the variation in the ‘n’ values with different formulations leads to a conclusion that the mullite crystals have different and irregular crystals forms. These E c values are compounded values corresponding to nucleation and growth processes or overlapping of two or more crystalline phases rather than specific to a single process. In this sense, it is important to note that the observations of mullite in Al–Si wastes in this study are on impure systems which are likely to be far from equilibrium so that simple equilibrium phase diagrams may not be relevant.
Table 2 Values of the Avrami exponent n obtained by the Augis and Bennett equation.
4. Conclusion
It is confirmed that both pyrex and soda lime glasses are suitable for producing mullite-based glass ceramics in the presence of additional glass-forming components. The results of this study are summarised as follows: (i) The mullite which is transformed from Al–Si–oxides wastes appeared at exothermic peak temperature range of 878–973°C. (ii) The non-isothermal activation energy of mullite crystallisation calculated by the modified Kissinger model is within the range of 429–768.2 kJ/mol. (iii) The value of growth morphology parameter ‘n’ indicates that bulk nucleation is dominant in mullite crystallisation followed by 2D to 3D growth of mullite crystals controlled by diffusion from a varying number of nuclei.
Acknowledgements
The authors are grateful to CMRDI (ASRT - Egypt ) and IPCMS (EGIDE - France) for supporting this work.
References
- Augis , J.A. and Bennet , J.D. 1978 . Calculation of the Avrami parameters for heterogeneous solid state reactions using a modification of the Kissinger method . Journal of Thermal Analysis , 13 ( 2 ) : 283 – 293 .
- Barbieri , L. , Corradi , A. and Lancellotti , I. 2000 . Alkaline and alkaline-earth silicate glasses and glass-ceramics from municipal and industrial wastes . Journal of the European Ceramic Society , 20 : 2477 – 2483 .
- Boccaccini , R.A. , Bucker , M. and Bossert , J. 1996 . Glass and glass-ceramics from coal fly-ash and waste glass . Tile and Brick International , 12 : 508 – 515 .
- Boccaccini , A.R. , Khalil , T.K. and Bücker , M. 1999 . Activation energy for the mullitization of a diphasic gel obtained from fumed silica and boehmite sol . Materials Letters , 38 : 116 – 120 .
- Campos , A.L. 2002 . Crystallization kinetics of orthorhombic mullite from diphasic gels . Journal of Non-Crystalline Solids , 304 : 19 – 24 .
- Castelein , O. 2001 . The influence of heating rate on the thermal behaviour and mullite formation from a kaolin raw material . Ceramics International , 27 : 517 – 522 .
- Chen , C.Y. , Lan , G.S. and Tuan , W.H. 2000 . Microstructural evolution during the sintering of kaolin powder compacts . Ceramics International , 26 : 715 – 720 .
- Chen , Y.-F. , Wang , M.-C. and Hon , M.-H. 2004a . Phase transformation and growth of mullite in kaolin ceramics . Journal of the European Ceramic Society , 24 : 2389 – 2397 .
- Chen , Y.-F. , Wang , M.-C. and Hon , M.-H. 2004b . Kinetics of secondary mullite formation in kaolin–Al2O3 ceramics . Scripta Materialia , 51 : 231 – 235 .
- Demichelis , R. 2008 . Ab initio quantum mechanical study of akdalaite (5Al2O3√H2O): structure and vibrational spectrum . Chemical Physics Letters , 465 : 220 – 225 .
- Francis , A.A. , Rawlings , D.R. and Boccaccini , R.A. 2002a . Glass-ceramics from mixtures of coal ash and soda lime glass by the petrurgic method . Journal of Materials Science Letters , 21 : 975 – 980 .
- Francis , A.A. , Rawlings , D.R. and Boccaccini , R.A. 2002b . Production of glass-ceramics from coal ash and waste glass mixtures . Key Engineering Materials , 206–213 : 2049 – 2052 .
- Francis , A.A. 2005 . Non-isothermal crystallization kinetics of a blast Furnace slag glass . Journal of the American Ceramic Society , 88 ( 7 ) : 1859 – 1863 .
- Fredericci , C. , Zanotto , E.D. and Ziemath , E.C. 2000 . Crystallization mechanism and properties of a blast furnace slag glass . Journal of Non-Crystalline Solids , 273 : 64 – 75 .
- Kissinger , H.E. 1956 . Variation of peak temperature with heating rate in differential thermal analysis . Journal of Research of the National Bureau of Standards , 57 ( 4 ) : 217 – 221 .
- Liu , K.-C. 1994 . Mullite formation in kaolinite–alumina . Acta Metallurgica et Materialia , 42 ( 2 ) : 489 – 495 .
- Maciejewski , M. 2000 . Computational aspects of kinetic analysis. Part B. The ICTAC Kinetics Project – the decomposition kinetics of calcium carbonate revisited, or some tips on survival in the kinetic minefield . Thermochimica Acta , 355 : 145 – 154 .
- Mackenzie , K. 1996 . The formation of mullite from kaolinite under various reaction atmospheres . Journal of the European Ceramic Society , 16 : 115 – 119 .
- Martins , I.M. 2004 . Manufacture of ceramic products using inertized aluminium sludges . Materials Science Forum , 455–456 : 822 – 826 .
- Matsura , M. and Suzuki , K. 1979 . Kinematical transformations of amorphous selenium by DTA measurement . Journal of Materials Science , 14 : 395 – 400 .
- Matusita , K. and Sakka , S. 1979 . Kinetic study of crystallization of glasses by differential scanning calorimeter . Physics and Chemistry of Glasses , 20 : 81 – 84 .
- Matusita , K. and Sakka , S. 1980 . Kinetic study on crystallization of glass by differential thermal analysis – criterion on application of Kissinger plot . Journal of Non-Crystalline Solids , 38-39 : 741 – 746 .
- Matusita , K. , Sakka , S. and Matsui , Y. 1975 . Determination of activation energy for crystal growth by differential thermal analysis . Journal of Materials Science , 10 : 961 – 966 .
- Osendi , M.I. and Baudin , C. 1996 . Mechanical properties of mullite materials . Journal of the European Ceramic Society , 16 : 217 – 224 .
- Ozawa , T. 1971 . Kinetics of non-isothermal crystallization . Polymer , 12 : 150 – 158 .
- Pask , J.A. and Tomsia , A.P. 1991 . Formation of mullite from sol–gel mixtures and kaolinite . Journal of the American Ceramic Society , 74 ( 10 ) : 2367 – 2373 .
- Pena , P. 1989 . Refractarios para zonasde contacto conel vidrio . Boletin de la Sociedad Española de Cerámica y Vidrio , 28 ( 2 ) : 89 – 96 .
- Rhanim , H. 1997 . Experimental characterization of high temperature creep resistance of mullite . Ceramics International , 23 : 497 – 507 .
- Romero , M. , Rawlings , D.R. and Rincón , J.Ma. 1999 . Development of a new glass-ceramic by means of controlled vitrification and crystallization of inorganic wastes from urban incineration . Journal of the European Ceramic Society , 19 : 2049 – 2059 .
- Schabbach , L.M. 2011 . Integrated approach to establish the sinter-crystallization ability of glasses from secondary raw material . Journal of Non-Crystalline Solids , 357 : 10 – 17 .
- Schneider , H. , Rodewald , K. and Eberhard , E. 1993 . Thermal expansion discontinuities of mullite . Journal of the American Ceramic Society , 76 ( 11 ) : 2896 – 2898 .
- Tummala , R.R. 1991 . Ceramic and glass-ceramic packaging in the 1990s . Journal of the American Ceramic Society , 74 ( 5 ) : 895 – 908 .
- Vieira , S.C. , Ramos , A.S. and Vieira , M.T. 2007 . Mullitization kinetics from silica- and alumina-rich wastes . Ceramics International , 33 ( 1 ) : 59 – 66 .
- Vieira , S. , Vieira , M.T. and Catarino , L. 2002 . “ Recovery of sludges from aluminium surface treatments ” . In Proceedings of the TMS fall 2002 extraction and processing division meeting on recycling and waste treatment in mineral and metal processing: technical and economic aspects 489 – 498 .
- Viswabaskaran , V. , Gnanam , F.D. and Balasubramanian , B. 2002 . Mullitization behaviour of south Indian clays . Ceramics International , 28 ( 5 ) : 557 – 564 .
- Viswabaskaran , V. , Gnanam , F.D. and Balasubramanian , B. 2003 . Mullitization behaviour of calcined clay–alumina mixtures . Ceramics International , 29 : 561 – 571 .
- Wei , P. and Rongti , L. 1999 . Crystallization kinetics of the aluminium silicate glass fiber . Materials Science and Engineering A , 271 : 298 – 305 .
- Xu , X.J. , Ray , C.S. and Day , D.E. 1991 . Nucleation and crystallization of Na2O√2CaO√3SiO2 glass by differential thermal analysis . Journal of the American Ceramic Society , 74 ( 5 ) : 909 – 914 .
- Yinnon , H. and Uhlmann , D.R. 1983 . Applications of thermoanalytical techniques to the study of crystallization kinetics in glass-forming liquids, part I: theory . Journal of Non-Crystalline Solids , 54 : 253 – 275 .