Abstract
Increasing cost of fossil fuels, environmental threats from exhaust emissions and their depleting nature have generated intense international interest in developing renewable and alternative fuels for internal combustion engines. This study investigates the suitability of different non-edible-derived biodiesels such as cottonseed oil methyl ester (COME), honne oil methyl ester (HnOME) and honge oil methyl ester (HOME) to four-stroke, single-cylinder compression ignition (CI) engine. Engine tests were conducted to study the effect of fuel injection timing (IT), fuel injector opening pressure (IOP) and injector nozzle geometry on the performance, combustion and emission characteristics of COME, HnOME and HOME in the modified CI engine. IT was varied from 19° to 27° before top dead centre (bTDC) in steps of 4° bTDC; IOP was varied from 205 to 240 bar in steps of 10 bar. Nozzle injectors of three to five holes, each of 0.3 mm size, were selected for the study. It was concluded that a retarded IT of 19° bTDC increased IOP of 230 bar, and four-hole nozzle injector of 0.3 mm size resulted in overall better engine performance with increased brake thermal efficiency and reduced hydrocarbon and carbon monoxide smoke emissions for the fuels tested.
1. Introduction
All nations in the world are confronted with the problem of energy shortage. There is an ever-increasing demand for energy in the industrial, transport and domestic sectors. Sustainable sources of energy are essential for economic and social development. The existing energy sources with fossil fuels may not be adequate to meet the ever-increasing energy demands. The diminishing and continuous increasing cost of petroleum products associated with their alarming pollution levels from diesel engines has caused an interest in finding renewable, alternative and sustainable fuels to fossil diesel. India's share in global oil reserves is about 0.5%, whereas its share in global consumption is about 3%. India ranks among the top 10 largest oil-consuming countries importing about 70% of its oil needs. The total oil consumption of India is about 2.5–2.8 million barrels per day. The demand for fossil fuel will rise higher and higher with every passing decade and is expected to reach an amount of nearly 250 million tons by the year 2024. In view of this, to reduce the huge amount of import bill on crude oil and become a self-reliant on energy sector, India has initiated renewable energy use in the country since three decades (Agarwal Citation2006).
Renewable fuels have advantages in terms of their renewability, biodegradability, energy security, environmental concerns, foreign exchange savings and socio-economical issues compared with fossil fuels. The use of renewable energy from non-edible oils reduces green house gas emissions and gives better food and energy security to the nation and ensures sustainable development (Banapurmath et al. Citation2005; Murugesan et al. Citation2009). Therefore, renewable fuels such as biofuels can be used predominantly as fuel for both the transportation and power generation sectors (Atadashi, Aroua, and Abdul Aziz Citation2010; Basha, Raja Gopal, and Jebaraj Citation2009; Banapurmath et al. Citation2005).
A number of vegetable oils have been used for biodiesel production and for diesel engine applications. These include biodiesels derived from Jatropha, honge (karanja), honne, palm, rubber seed, rape seed, mahua and neem seed oils (Banapurmath, Tewari, and Hosmath Citation2008, Citation2009; Bari, Lim, and Yu Citation2002; Gajendra Babu, Kumar, and Das Citation2006; Karnwal et al. Citation2010; Morshed et al. Citation2011; Onga et al. Citation2011; Raheman and Phadatare Citation2004; Ramadhas, Muraleedharan, and Jayaraj Citation2005a, Citation2005b; Sahoo et al. Citation2007, Citation2009; Venkanna and Reddy 2009; Yang et al. Citation2011). Biodiesel is a clean burning alternative fuel, produced from domestic, renewable resources. Biodiesel is simple to use, biodegradable, non-toxic and essentially free of sulphur and aromatics. Biodiesel improves lubricity and reduces premature wearing of fuel pumps. All biodiesels do not give the same performance when used in diesel engines. This is because each vegetable oil has different fatty acid composition with different molecular weight. Also each biodiesel has varying physico-chemical properties. Therefore, they behave differently when they are used as fuel in diesel engines. Comprehensive studies on their use as suitable alternative fuels to diesel engines are essential to ascertain their feasibility as diesel engine fuels. HOME, honne oil methyl ester (HnOME) and cottonseed oil methyl ester (COME) are also available in India at different places.
Feasibility of COME and HOME and their blends in diesel engine applications has been reported by several researchers. Lower carbon monoxide (CO), carbon dioxide (CO2), NOx, smoke emissions and lower brake thermal efficiency (BTE) of COME and their blends than those of diesel fuel have been reported (Nabi, Rahman, and Akhter Citation2009; Naga Sarada et al. Citation2010; Fan et al. Citation2008). Lower and medium percentages of COME can be partially substituted for the diesel fuel without any modifications of the diesel engine (Aydin and Bayindir Citation2010; Martin and Edwin Citation2011). The effects of using neat COME biodiesel on the combustion behaviour of a standard, high-speed, direct injection have been reported (Rakopoulos et al. Citation2010). It has been reported that ignition delay was not much affected, the fuel injection pressure diagrams were very slightly advanced accompanied with higher injection pressures, maximum cylinder pressures remained the same with the vegetable oil and slightly increased with the biodiesel and the cyclic irregularity was not affected with the biofuels remaining at the acceptable levels (Rakopoulos et al. Citation2010). HOME and their blends gave, higher CO, NOx, smoke emissions and lower BTE compared to diesel fuel (Banapurmath, Tewari, and Hosmath Citation2008, Citation2009; Gajendra Babu, Kumar, and Das Citation2006; Sahoo et al. Citation2009).
The production, performance and emission of palm oil, Jatropha curcas and Calophyllum inophyllum biodiesels have been reviewed (Onga et al. Citation2011). Palm oil is one of the most efficient oil-bearing crops in terms of oil yield, land utilisation, efficiency and productivity. However, competition between edible and non-edible oil sources as food and fuel makes edible oil not an ideal feedstock for biodiesel production. Therefore, attention is focused on non-edible oils derived from J. curcas and C. inophyllum. C. inophyllum oil as they can be transesterified and considered as potential biodiesel fuel. Compared with the use of palm oil and Jatropha in biodiesel industry, the use of biodiesel from C. inophyllum is still in a nascent state. Therefore, long-term endurance research and tribological studies need to be carried out before C. inophyllum oil base biodiesel can become an alternative fuel in future. Performance of a direct injection (DI) diesel engine typically used in the agricultural sector with diesel and neat honne oil (H100) has been reported in the literature. Lower BTE, higher hydrocarbon (HC), than those when using smoke and lower NOx diesel have been reported (Venkanna and Venkataramana Reddy Citation2011). A two-step process to produce biodiesel from C. inophyllum oil has been reported in the literature (Selvabala et al. Citation2011). Central composite design and response surface methodology were utilised to determine the best operating condition for the pre-treatment step. Biodiesel produced by this process was tested for its fuel properties.
Performance tests on compression ignition (CI) engine with different vegetable oils operated at different injection pressures and injection timings (ITs) have been carried out (Gajendra Babu Citation2007). Changes in ITs change the position of the piston and cylinder pressure and temperature at the injection. Retarded ITs showed significant reduction in diesel NOx and biodiesel NOx (Banapurmath, Tewari, and Hosmath Citation2008, Citation2009; Bari, Yu, and Lim Citation2004; Gajendra Babu, Kumar, and Das Citation2006; Hountalas et al. Citation2001; Roy Citation2009; Tao et al. Citation2005). Cylinder pressures and temperatures gradually decrease when ITs are retarded (Roy Citation2009). The effect of fuel IT on waste cooking oil as fuel in DI diesel engine with advanced IT by 4° before top dead centre (bTDC) gave better efficiency, reduced CO and higher NOx emissions (Bari, Yu, and Lim Citation2004). Vegetable oils for diesel engine applications operated at different injection pressures have also been reported (Banapurmath, Tewari, and Hosmath Citation2009; Gajendra Babu Citation2007; Puhan et al. Citation2009; Rosli, Semin, and Ismail Citation2008; Roy Citation2009). Better performance, higher peak cylinder pressure and temperature were observed at increased injection pressure (Rosli, Semin, and Ismail Citation2008; Roy Citation2009).
From the literature survey, it follows that not much work has been done to investigate the effect of IT, injector opening pressure (IOP) and nozzle geometry on the performance, combustion and emission characteristics of COME/HnOME/HOME-fuelled diesel engine.
This study reports the effect of fuel IT, fuel IOP and injector nozzle geometry on the performance and combustion characteristics of CI engine fuelled with selected biodiesels.
2. Fuels used in the experiment
Table shows fatty acid profiles of the vegetable oils used in the study. The properties of biodiesels, viz. COME, HnOME and honge oil methyl ester (HOME) are given in Table . The respective properties were determined in the author's college laboratory.
Table 1 Fatty acid composition of different vegetable oils.
Table 2 Properties of non-edible-derived biodiesels.
The transesterification method used to produce respective biodiesels is explained below.
Honge seed oil biodiesel production has already been reported in detail by the authors in their previous publications. However, the production of biodiesel derived from cottonseed and honne oils has been suitably explained.
Cottonseed oil biodiesel production was done as per the following procedure: 1 litre of raw cottonseed oil was heated and maintained at a temperature of 65°C for 30 min in order to the remove moisture content. The oil was then titrated against NaOH solution, which indicated about 1% free fatty acid (FFA) contained in oil. One litre of moisture-free cottonseed oil was heated to a temperature of 55°C in the flask. Then, a mixture of 200 ml methanol and 4 g NaOH was added. The resulting mixture is maintained at that temperature and stirred at speed of 400 rpm. After 1 h, the mixture was allowed to settle in a separating funnel for 8 h. Finally, the heavier layer of glycerine was separated out to get biodiesel, which was washed three or four times with 10 ml acetic acid+hot water solution to remove moisture and other sediments. A clean COME was thus obtained. The resulting ester was about 94%.
Honne seed oil biodiesel production was carried out using a three-stage transesterification process. The first stage involves use of 1 litre of raw honne oil, heated and maintained at a temperature of 65°C for 30 min in order to remove moisture content. The oil was titrated against NaOH solutions, which indicated 8% FFA. The second stage involves acid esterfication of 1 litre moisture-free honne oil, by heating to 60°C in a flask. Then a mixture of 150 ml of methanol and 1.5 ml of sulphuric acid was added to the oil; the mixture was maintained at that temperature and was stirred at a speed of 450 rpm for 1 h. The resultant solution was allowed to settle in a separating funnel for a period of 8 h, and subsequently the top layer of acid was removed and the residue oil was tested for FFA content which was found to be 5.6%. Because of high FFA, it was again given acid treatment with a solution of 100 ml of methanol and 1 ml of sulphuric acid, at 60°C with 450 rpm for 1 h duration. On repeating the above procedure, the residue oil showed 2.8% FFA. The third stage involves heating of residue oil at 60°C in the flask. Then a mixture of 100 ml methanol and 7.5 g of NaOH was added. The resulting mixture was maintained at that temperature with a stirrer speed of 450 rpm. After 1.5 h, the mixture was allowed to settle in a separating funnel. After 8 h, glycerine being heavier was separated out and obtained biodiesel of honne oil was further washed three to four times with 10 ml acetic acid and hot water solution to remove moisture and other sediments and to obtain clean HnOME. The resulting ester was about 86%.
3. Experimental set-up
Experiments were conducted on a Kirloskar TV1, four-stroke, single-cylinder, water-cooled diesel engine. Figure shows the schematic experimental set-up used in the study. Eddy current dynamometer was used for loading the engine. The engine was operated at a rated constant speed of 1500 rpm. The emission characteristics were measured using HARTRIDGE smoke meter and five gas analysers during the steady-state operation. The specification of the CI engine is given in Table . Figure shows three- to five-hole injectors with 0.3 mm orifice diameter. Specifications of exhaust gas analyser and smoke meter are shown in Tables and , respectively.
Table 3 Specifications of CI engine.
Table 4 Specifications of exhaust gas analyser.
Table 5 Specifications of smoke meter.
The experiments were carried out without any major engine modification. The main aim of this study was to suggest minimum modifications required to operate the engine with biodiesel and at the same time to optimise the biodiesel engine performance.
4. Results and discussions
Here, the effect of Injection Timing (IT), Injector opening pressure (IOP) and nozzle geometry on the performance of diesel engine fuelled with COME is presented in the following sections.
4.1 Effect of IT
Studies on engine performance were conducted at three ITs of 19°, 23° and 27° bTDC, and IOPs were varied from 210 to 240 bar in steps of 10 bar. An injector of three to five holes, each having 0.3 mm diameter orifices, was selected for the engine study.
4.1.1 Brake thermal efficiency
The effect of IT on BTE of CI engine operation with HnOME, COME and HOME at three ITs is shown in Figure . For 80% load, highest BTE of 27.57% was obtained with diesel at a static IT of 23° bTDC. The optimum IT for standard diesel was found to be 23° bTDC, which also matches with the manufacturer's specification. BTEs were lower for the biodiesels than for diesel operation for all the three ITs. The decrease in BTE for these biodiesels might be attributed to lower energy content of the fuel, higher viscosity and density and higher fuel consumption for the same power output. Due to high viscosity of all biodiesels used, the formation of the mixture and subsequent combustion were poorer than those of diesel. However, by retarding the IT by 4° crank angle, there was an improvement in BTE for all the biodiesels tested. Retarding the IT lowers the ignition delay period and results in higher heat release rate for the uncontrolled or premixed combustion phase. Lower IT results in better combustion.
Also higher peak pressure and heat release rate with retarded IT for all biodiesels could be the reason for this observed trend (Figures and ). Therefore, it is concluded that retarded IT of 19° bTDC will favour the biodiesels injected inside the engine cylinder. BTE with HnOME, COME and HOME operation at 19° bTDC and 80% load obtained was found to be 21.90, 21.68 and 21.25%, respectively, compared with 27.57% for diesel operation at 23° bTDC. HnOME performance was better than COME and HOME, because of its higher calorific value, Cetane number and lower viscosity.
4.1.2 HC and CO emissions
Figures and show the effect of IT on HC and CO emissions for standard diesel and COME, HnOME and HOME operation. HC emissions in diesel engines are caused due to lean mixture during delay period and under-mixing of fuel leaving the fuel injector nozzle at lower velocity. The general trend of increased HC and CO emissions was observed for HnOME, COME and HOME operation than for diesel operation for all the three ITs. The decreased combustion efficiency because of improper spray pattern and higher viscosity of all biodiesels could be the reason for this trend. The poor spray characteristics of HnOME, COME and HOME operation result in poor mixing, and consequently poor combustion may be responsible for this trend. The HC emission for HnOME, COME and HOME operation at 19° bTDC and 80% load obtained was 64, 68 and 72 ppm, respectively, compared with 29 ppm at 23° bTDC for diesel operation. Cetane number of the fuel plays an important role in the ignition process and accordingly the cetane number of HnOME was higher than that of COME and HOME.
CO is a toxic by-product and its presence is a clear indication of incomplete combustion of the pre-mixed mixture. From Figure , it is observed that CO levels were lower at the optimum IT of 19° bTDC for all biodiesels tested. The CO emission for HnOME, COME and HOME operation at 19° bTDC and 80% load was 0.21, 0.24 and 0.30%, respectively, compared with 0.1% at 23° bTDC for diesel operation. It may be concluded that improved combustion, increased cylinder pressure and temperature at 19° bTDC result in the lowest un-burnt and in-complete combustion by-products such as HC and CO.
4.1.3 NOx emissions
The effect of IT on emission of NOx with load for diesel, and for HnOME, COME and HOME operation is shown in Figure . In general, retarded injection results in substantial reduction in NOx emissions. As the IT is retarded, the combustion process gets retarded. NOx concentration levels were lower as peak combustion temperature was lower. The NOx emission levels for HnOME, COME and HOME operation at 19° bTDC and 80% load obtained were 542, 540 and 535 ppm, respectively, compared with 790 ppm at 23° bTDC for diesel operation. NOx levels were higher at the ITs of 23° and 27° bTDC as they lead to a sharp premixed heat release due to higher ignition delay. For all biodiesels operation, NOx emissions were slightly lower than those for diesel fuel operation. From these results, the best IT was taken as 19° bTDC for all biodiesels.
4.1.4 Combustion analysis
Figures and show the effect of IT on the pressure variation and heat release rate at 80% engine operation for HnOME. For this, IOP of 230 bar and four-hole injector with 0.3 mm diameter were considered.
Lower delay period was observed with HnOME followed by COME and HOME. Higher calorific value and Cetane number of HnOME are responsible for this trend.
Higher heat release rate during the premixed combustion phase with HnOME followed by COME and HOME were observed, whereas minor effect on the mixing controlled combustion phase for all the biodiesels was observed.
4.2 Effect of IOP
The IOPs were varied from 205 to 210–240 bar (21.0–24.0 MPa) in steps of 10 bar. Also, nozzle geometry (three- to four-hole nozzle, each 0.3 mm diameter) was varied against these pressures. The part load (80% load) readings were only reported at these injection pressures.
4.2.1 Standard diesel and biodiesel operation
4.2.1.1 Performance parameters
Engine was operated only at the manufacturer's specified IOP of 205 bar, optimum start of injection of 23° bTDC and maximum compression ratio of 17.5. It was observed that the maximum BTE at 80% load complies with the specified conditions for diesel operation and was found to be 27.57%.
The effect of brake power on BTE at different IOPs and different nozzle geometry such as three, four and five holes is shown in Figures . Amongst all the IOPs tested, the highest BTE occurred at 230 bar for a nozzle geometry of four holes with a orifice diameter of 0.3 mm each. This is because at higher injection pressures atomisation, spray characteristics and mixing with air are better, which result in improved combustion. Too high at IOP of 240 bar will lead to delayed injection neglecting the gain due to higher IOP.
The BTE is found to be 24.95% and the maximum value is obtained with four-hole nozzle at an IOP of 230 bar. The BTE reported for three- and five-hole nozzles was 24.50 and 24.25%, respectively, at 230 bar.
In view of this, the results revealed that the BTE was found to be more with the four-hole nozzle geometry and IOP of 230 bar amongst all IOPs and nozzle geometry studied. The IOP for other two nozzles (four and five holes) was also found to be optimum at 230 bar.
Increase of the number of holes has no much effect on ignition delay, but the fuel–air mixing rate increases. Also, heat release rates during premixed combustion and mixing-controlled combustion phases increase with increase of the number of holes on the injector.
4.2.1.2 Emission parameters
HC emission: Figures show the effect of brake power on HC emission at different IOPs and different nozzle geometry with COME engine operation. A significant drop in HC emission is observed at 230 bar IOP with the four-hole nozzle geometry because of better combustion. Enhanced atomisation will also lead to a lower ignition delay. This will enhance the performance of the engine with vegetable oils, which normally have a higher ignition delay on account of their higher viscosity. An improvement in the spray will lead to a lower physical delay. The improved spray will also lead to better combustion and thermal efficiency at full load. HC emission reduced from 90 to 80 ppm, after increasing the IOP from 210 to 230 bar at full load. The highest IOP of 240 bar leads to an increase in the HC level to 85 ppm probably because it leads to a reduction in the BTE. Also a very high IOP will lead to a considerable portion of the combustion occurring in the diffusion phase on account of the small ignition delay. Too high an IOP (240 bar) will lead to delayed injection, negating the gain due to higher IOP.
HC emissions were found to be lower at IOP 230 bar for four-hole followed by three-hole and five-hole nozzle geometry, respectively. This concludes that un-burnt HCs are less for the four-hole nozzle and IOP of 230 bar operation and are due to improved atomisation and proper combustion of all biodiesels tested.
CO emission: Figures show the effect of brake power on CO emission. Observed trends for CO emissions were similar to those for HC emissions, with lower CO emissions at 230 bar IOP and four-hole injector. This concludes that CO emissions are less for the four-hole nozzle and IOP of 230 bar operation which are due to improved atomisation and proper combustion of all biodiesels.
NOx emission: Nitric oxide emissions increase with the increase in IOP due to faster combustion and higher temperatures reached in the cycle as shown in Figures . However, at 240 bar, the NOx emissions reduced due to lowered combustion efficiency at this pressure.
Higher NOx emissions with all biodiesels were observed with increased number of holes. The four-hole injector provides better air and fuel mixing and hence higher premixed combustion occurs leading to higher NOx emissions.
4.3 Combustion analysis
Figures and show the effect of injection pressure on the pressure variation and heat release rate with crank angle at 80% load engine operation for the HnOME operation.
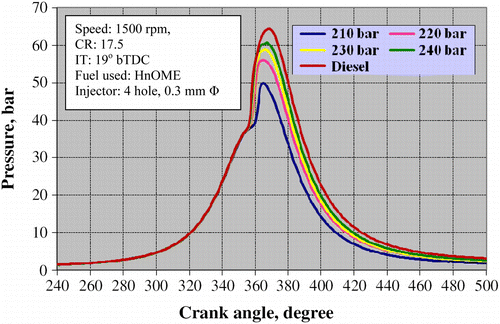
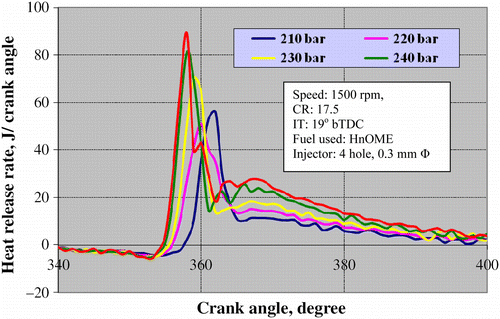
From Figures and , increasing IOP from 205 to 230 bar increases both peak pressure and heat release rate associated with lower delay period for HnOME. Increasing IOP beyond 230 bar reduces both peak pressure and heat release rate due to negating the gain in pressure.
5. Conclusions
From the exhaustive study on the feasibility of different biodiesels as alternative fuel to diesel engine applications, it is found that their performance is inferior to diesel engine operation. By suitable variations in the engine parameters such as IT and IOP and nozzle geometry, its performance can be suitably improved. With these adjustments, biodiesel performance approaches closer to diesel engine operation.
Retarding IT from 23° to 19° bTDC showed encouraging results for the biodiesels. Increasing injection pressure from 205 to 230–240 bar for biodiesels favoured the engine performance with reduced emissions. Increasing the number of nozzle holes in the injector improved the biodiesel engine performance appreciably.
It can be concluded that operating the existing diesel engine with slightly higher injection pressure, more number of holes in the fuel injector and retarding IT will improve the performance of the biodiesel-fuelled engine considerably.
REFERENCES
- Agarwal, A. K.2006. “Biofuels (Alcohols and Biodiesel) Applications as Fuels for Internal Combustion Engines.” Progress in Energy and Combustion Science33: 233–271.
- Atadashi, I. M., M. K.Aroua, and A.Abdul Aziz. 2010. “High Quality Biodiesel and Its Diesel Engine Application: A Review.” Journal of Renewable and Sustainable Energy Reviews14 (7): 1999–2008.
- AydinHüseyin, and HasanBayindir. 2010. “Performance and Emission Analysis of Cottonseed Oil Methyl Ester in a Diesel Engine.” Renewable Energy35: 588–592.
- Banapurmath, N. R., P. G. Tewari, Y. H. Basavarajappa, and V. S. Yaliwal. 2005. “Performance of Honge (Pongamia pinnata) Oil Blends in a Diesel Engine.” Paper presented at the XIX NCICEC, Annamalai University, Chidambaram, India, 1 – 6.
- Banapurmath, N. R., P. G.Tewari, and R. S.Hosmath. 2008. “Combustion and Emission Characteristics of a Direct Injection, Compression–Ignition Operated on Honge Oil, HOME and Blends of HOME and Diesel.” International Journal of Sustainable Engineering1 (2): 80–93.
- Banapurmath, N. R., P. G.Tewari, and R. S.Hosmath. 2009. “Effect of Biodiesel Derived from Honge Oil and Its Blends with Diesel when Directly Injected at Different Injection Pressures and Injection Timings in Single-Cylinder Water-Cooled Compression Ignition Engine.” Proceedings [universal] of Institute of Mechanical Engineers IMechE.Part A: Journal of Power and Energy223: 323–332.
- Bari, S., T. H.Lim, and C. W.Yu. 2002. “Effect of Preheating of Crude Palm Oil (CPO) on Injection System, Performance and Emission of a Diesel Engine.” Renewable Energy27: 339–351.
- Bari, S., C. W.Yu, and T. H.Lim. 2004. “Effect of Fuel Injection Timing with Waste Cooking Oil as a Fuel in Direct Injection Diesel Engine.” Proceedings [universal] of Institute of Mechanical Engineers IMechE.Part D, Journal of Automobile Engineering218: 93–104.
- Basha, Syed Ameer, K.Raja Gopal, and S.Jebaraj. 2009. “A Review on Biodiesel Production, Combustion, Emissions and Performance.” Renewable and Sustainable Energy Reviews13: 1628–1634.
- Fan, Xiaohu, XiWang, FengChen, P.Daniel Geller, and Peter, J.Wan. 2008. “Engine Performance Test of Cottonseed Oil Biodiesel.” The Open Energy and Fuels Journal1: 40–45.
- Gajendra Babu, M. K.2007. “Studies on Performance and Exhaust Emissions of a CI Engine Operating on Diesel and Diesel Biodiesel Blends at Different Injection Pressures and Injection Timings.” Society of Automotive Engineers, Paper No.: 2007-01-0613.
- Gajendra Babu, M. K., ChandanKumar, and L. M.Das. 2006. Experimental Investigations on a Karanja Oil Methyl Ester Fuelled DI Diesel Engine. Society of Automotive Engineers, Paper No.: 2006-01-0238.
- Hountalas, D. T., D. A.Kouremenos, K. B.Binder, A.Raab, and M. H.Schnabel. 2001. “Using Advanced Injection Timing and EGR to Improve DI Engine Efficiency at Acceptable NO and Soot Levels.” Society of Automotive Engineers, Paper No.: 2001-01-0199.
- Karnwal, A., NaveenKumar, M. M.Hasan, R.Chaudhary, A. N.Siddiquee, and Z. A.Khan. 2010. “Production of Biodiesel from Thumba Oil: Optimization of Process Parameters, Iranica.” Journal of Energy & Environment1 (4): 352–358.
- Martin, Leenus Jesu M., and GeoEdwin. 2011. “Effect of Diesel Addition on the Performance of Cottonseed Oil Fuelled DI Diesel Engine.” International Journal of Energy and Environment2 (2): 321–330.
- Morshed, Mahbub, KanizFerdous, M. R.Khan, M. S. I.Mazumder, M. A.Islam, and Md. T.Uddin. 2011. “Rubber Seed Oil as a Potential Source for Biodiesel Production in Bangladesh.” Fuel90: 2981–2986.
- Murugesan, A., C.Umarani, R.Subramanian, and N.Nedunchezhian. 2009. “Bio-Diesel as an Alternative Fuel for Diesel Engines – A Review.” Renewable and Sustainable Energy Reviews13 (3): 653–662.
- NabiNurun Md., MustafizurRahman Md., and Shamim Md.Akhter. 2009. “Biodiesel from Cotton Seed Oil and Its Effect on Engine Performance and Exhaust Emissions.” Applied Thermal Engineering29: 2265–2270.
- Naga Sarada, S., M.Shailaja, A. V.Sita Rama Raju, and K.Kalyani Radha. 2010. “Optimization of Injection Pressure for a Compression Ignition Engine with Cotton Seed Oil as an Alternate Fuel.” International Journal of Engineering, Science and Technology2 (6): 142–149.
- Onga, H. C., T. M. I.Mahlia, H. H.Masjukia, and R. S.Norhasyimab. 2011. “Comparison of Palm Oil, Jatropha curcas and Calophyllum inophyllum for Biodiesel.” Renewable and Sustainable Energy Reviews15 (3): 501–3515.
- PuhanSukumar, R.Jegan, K.Balasubbramanian, and G.Nagarajan. 2009. “Effect of Injection Pressure on Performance, Emission and Combustion Characteristics of High Linolenic Linseed Oil Methyl Ester in a DI Diesel Engine.” Renewable Energy34: 1227–1233.
- Raheman, H., and A. G.Phadatare. 2004. “Diesel Engine Emissions and Performance from Blends of Karanja Methyl Ester and Diesel.” Biomass and Bioenergy27: 393–397.
- Rakopoulos, C. D., D. C.Rakopoulos, E. G.Giakoumis, and A. M.Dimaratos. 2010. “Investigation of the Combustion of Neat Cottonseed Oil or Its Neat Bio-Diesel in a HSDI Diesel Engine by Experimental Heat Release and Statistical Analyses.” Fuel89 (12): 3814–3826.
- Ramadhas, A. S., C.Muraleedharan, and S.Jayaraj. 2005a. “Performance and Emission Evaluation of a Diesel Engine Fueled with Methyl Esters of Rubber Seed Oil.” Renewable Energy30: 1789–1800.
- Ramadhas, A. S., C.Muraleedharan, and S.Jayaraj. 2005b. “Characterization and Effect of Using Rubber Seed Oil as Fuel in the Compression Ignition Engines.” Renewable Energy30: 795–803.
- Rosli, Abu Bakar, Semin, and Abdul RahimIsmail. 2008. “Fuel Injection Pressure Effect on Performance of Direct Injection Diesel Engines Based on Experiment.” American Journal of Applied Sciences5 (3): 197–202.
- Roy, M. M.2009. “Effect of Fuel Injection Timing and Injection Pressure on Combustion and Odorous Emissions in DI Diesel Engine.” Journal of Energy Resources Technology, ASME Transactions131: 1–8.
- Sahoo, P. K., L. M.Das, M. K. J.Babu, and S. N.Naik. 2007. “Biodiesel Development from High Acid Value Polanga Seed Oil and Performance Evaluation in a CI Engine.” Fuel86: 448–454.
- Sahoo, P. K., L. M.Das, M. K. J.Babu, P.Arora, V. P.Singh, N. R.Kumar, and T. S.Varyani. 2009. “Comparative Evaluation of Performance and Emission Characteristics of Jatropha, Karanja and Polanga Based Biodiesel as Fuel in a Tractor Engine.” Fuel88: 1698–1707.
- Selvabala, V. S., Dinesh K.S., Jalagandeeswaran, K., Premkumar, M.P., and SivanesanS.2011. “Two-Step Biodiesel Production from Calophyllum inophyllum Oil: Optimization of Modified b-Zeolite Catalyzed Pre-Treatment.” Bioresource Technology102: 1066–1072.
- Tao, F., Y.Liu, B. H.Rempel Ewert, D. E.Foster, R. D.Reitz, D.Choi, and P. C.Miles. 2005. “Modelling the Effect of EGR and Retarded Injection on Soot Formation in a High Speed Diesel Injection (HSDI) Diesel Engine Using a Multi Step Phenomenological Soot Model.” Society of Automotive Engineers, Paper No.: 2005 -01-0121.
- Venkanna, B. K., and C.Venkataramana Reddy. 2011. “Performance, Emission and Combustion Characteristics of Direct Injection Diesel Engine Running on Calophyllum inophyllum Linn oil (Honne oil).” International Journal of Agricultural & Biological Engineering4 (1): 26–34.
- Yang, Ru, MengxingSu, JianchunZhang, FuqiangJin, ChunhongZha, MinLi, and XinminHao. 2011. “Biodiesel Production from Rubber Seed Oil Using Poly (sodium acrylate) Supporting NaOH as a Water-Resistant Catalyst.” Bioresource Technology102: 2665–2671.