Abstract
The underground temperature at a depth of about 3–4 m is almost constant all the year round. In summer, the underground temperature is lower than the ambient temperature, but in winter it is vice versa. This potentiality is considered for greenhouse cooling and heating by using an earth-to-air heat exchanger (EAHE). This paper considers the effects of two parameters as independent variables including the area of greenhouse and the percentage of vegetation coverage inside the greenhouse on the performance of an EAHE system during both cooling and heating modes. The inside temperature, the thermal energy exchange and the coefficient of performance (COP) of the system were considered as dependent variables. The results showed that both greenhouse area and the percentage of vegetation coverage inside the greenhouse had significant effects on the performance of the EAHE system during both cooling and heating modes. However, the COP of the EAHE system was higher in the cooling mode (4.32) than during the heating mode (1.01). The percentage of vegetation coverage negatively affected the performance of the EAHE system in the cooling mode. However, the performance of the EAHE system improved with the increase in the percentage of vegetation coverage during the heating mode.
1. Introduction
Population growth and increasing food consumption are two important problems encountered in developing countries such as Iran. To overcome these limitations, an effective and efficient use of water and soil resources and manpower are important. To achieve gross domestic product growth and self-sufficiency in meeting the food requirements, it becomes important to have an in-depth knowledge of practical and economic processes and the application of the latest knowledge and technology used worldwide. Nowadays, the greenhouse industry has promisingly increased the efficient use of limited water and land resources, especially in arid and semi-arid areas.
Over the last two decades, there has been a manifold increase in the construction of agricultural greenhouses using renewable energy (RE) resources, e.g. solar energy. The primary objective of a greenhouse is to produce a higher yield outside the cultivation season, which can be achieved by maintaining optimal growth conditions at each stage of crop production. For this purpose, an appropriate heating (or cooling) system can be coupled with the greenhouse. This strategy may significantly affect cultivation time and the quality and quantity of agricultural products (Sethi and Sharma Citation2008). Seeking RE resources instead of fossil fuels is of great importance even for countries that are rich in fossil fuels such as Iran. This approach is mainly pursued due to environmental and/or economic constraints. At present, in Iran and other oil-producing nations, RE sources represent a low share and a high cost in electricity or heat production (Midilli, Dincer, and Ay Citation2006).
Recently, the Iranian government has announced its new policy on subsidising energy carriers, which is known as subsidies reform plan or targeted subsidies plan. The simple argument in the plan is that the price of oil by-products, including gasoline, has been posted at about 6 cents/L (in US currency) in Iran, whereas the global price of gasoline has been fixed at 70 cents/L or even more (Abbaspour-Fard, Gholami, and Khojastehpour Citation2011). After this plan had been launched, the price of petroleum products in Iran has become much closer to that in other parts of the world. Despite this sharp increase in the price of petroleum products, greenhouses in Iran continue to depend mostly on fossil fuel resources for their energy requirements. Consequently, this poses a major threat to the success of this growing industry in this country. Therefore, seeking and studying alternative RE resources is a necessity to overcome this challenge. One of the readily RE sources is the earth's energy. The so-called earth-to-air heat exchanger (EAHE) has been principally considered as an effective renewable, passive energy source for building space conditioning (Bansal, Sodha, and Bhardwaj Citation1983; Bharadwaj and Bansal Citation1983), which can also be a promising system for compensating at least a fraction of heating and cooling loads of agricultural industries, e.g. greenhouses and poultry production units (Santamouris et al. Citation1994). Fundamentally, this idea is not new and can be traced back to ancient times. Utilising the underground potential for greenhouse heating (and cooling) applications has gained increasing acceptance during the last few years. The temperature below the earth's surface at a depth of 3–4 m remains constant throughout the year. The huge underground mass of the earth can be used as a heat storage source. The EAHE basically consists of underground pipe(s) and an airflow system that forces the air through the pipe(s) (Figure ). The pipes usually run along the length of the greenhouse, with inlets and outlets for the circulating air at opposite sides.
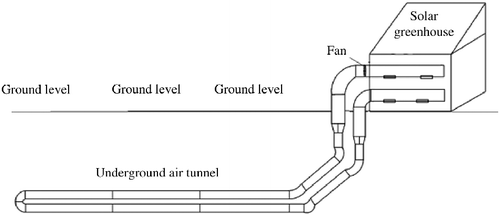
During the cold season, the cold air from inside the greenhouse is circulated through the underground pipe(s). Heat is transferred from the soil mass to the air stream and then returns to the greenhouse. The circulating process of the air gradually heats up the greenhouse. The same system can also be used for cooling the greenhouse during the warm season. Extensive research on the EAHE system has been conducted in different parts of the world. In this paper, some important representative applications of agricultural greenhouses using the EAHE system for heating purposes are reviewed and discussed.
Some of the important features examined include: material of the pipe; number of the pipes used; pipe diameter; depth at which the pipe(s) was installed; vertical distance kept between the pipes under the ground; area of the greenhouse; its location; type of the covering material used; flow rate through the pipes; and the performance of the system (Sethi and Sharma Citation2008). Ground-source or geothermal heat pumps are a highly efficient, RE technology for space heating and cooling. This technology relies on the fact that, at depth, the temperature of the earth is relatively constant, which is warmer than the air temperature in winter and cooler than that in summer. A geothermal heat pump can transfer the heat stored in the earth into a building during winter and transfer the heat out of the building during summer (Omer Citation2008). The performance of an EAHE system depends upon the temperature and moisture distribution in the ground as well as on climate conditions on the ground surface (Kumar, Kaushik, and Garg Citation2006).
In one earlier study, 44 plastic pipes were arranged in two rows and buried at depths of 50 and 90 cm, respectively, in a 1736 m2 glasshouse located at Yokohama, Japan (Kozai Citation1989). The diameter and total length of the pipes were 0.102 and 587.1 m, respectively. A total of eight electric fans (0.315 kW per fan; airflow rate: 54 m3 per min per fan) were used for heat exchange to force the air through the rigid PVC pipes. The average circulation speed of the air in the pipe was 4.7 m/s. Tomatoes were grown inside the greenhouse in a hydrophonic system. The use of the EAHE system reduced oil consumption by 28% when compared with a conventional greenhouse.
A greenhouse in Quebec (Canada) was equipped with a thermal blanket along with an EAHE system consisting of two rows of corrugated plastic pipes buried at depths of 0.45 and 0.75 m (Gauthier, Lacroix, and Bernier Citation1997). The air was circulated through the pipes with a velocity of 4 m/s. In spite of shallow burial, the EAHE system could increase the inside temperature of the greenhouse by 5–7°C when compared with the outside temperature that was below the freezing point.
A one-way EAHE system with cooling and heating modes was employed in Ahmedabad, India with an annual average temperature ranging between 23 and 43°C (Sharan and Jadhav Citation2002). The system consisted of a 50 m pipe of 0.10 m nominal diameter, 0.003 m thickness and 3 m burial depth. The air was pumped through a pipe with a velocity of 11 m/s using a 400 W electropump. Heating and cooling trials were performed during three consecutive days in January and May, respectively. Temperatures at the entrance, middle and end of the pipe were recorded. The system was able to increase the temperature by about 14°C in January and decrease it by the same value in May. The coefficient of performance (COP) of the EAHE system for the cooling and heating modes was 3.3 and 3.8, respectively.
Experimental tests for the heating potential of an EAHE system were performed in a 120 m2 greenhouse with a single PE cover at Kothara, India (Sharan, Prakash, and Jadhav Citation2003). A total of two sets of four mild steel pipes of diameter 0.1 m and length 23 m each were buried at depths of 2 and 3 m in a single-pass mode to heat the greenhouse during the winter season. An airflow rate of 7200 m3/h was used by means of a 7.5 hp motor blower assembly. The system could heat up the inside air temperature of the greenhouse from 9 to 22–23°C within half an hour during the cold winter nights of January, with the outside temperature during 24 h being 7–9°C on average.
In another study, an EAHE system was integrated with a greenhouse of floor area 8.58 × 4 m2 and oriented from the east to west direction located at IIT Delhi, India (Tiwari et al. Citation2006). The EAHE system consisted of a PVC pipe of length 39 m and diameter 0.06 m. The pipes were spread under the ground at a depth of 1 m in a serpentine manner. The length and spacing between the serpentine pipes were 4.8 and 0.5 m, respectively, with eight number of turns. A blower of 335 W with a capacity of 150 kg/h was fitted at the end of the suction pipe positioned in the south-west direction of the greenhouse. The correlation coefficient and the root-mean-square (RMS) percentage deviation were computed for each month for the validation of the thermal model. The respective computed values for the thermal model were 0.99 and 4.24% for the month of January. Statistical analysis revealed that there was a fair agreement between the predicted and experimental values. An effort was also made to optimise the working hours of the EAHE system to obtain a maximum heating/cooling potential. The non-operational hours of the EAHE system were 252 and 279 h for the months of February and March, respectively. The maximum values of the heating potential (11.55 MJ) and the cooling potential (18.87 MJ) were found during off-sunshine hours (8 pm–8 am) and peak sunshine hours (8 am–8 pm), for a typical day in the months of January and June.
Owing to a large number of graduate manpower in the agricultural sector and the severe limitations of soil and water resources, greenhouse and intensive indoor cultivation has become a growing industry in Iran, including its north-east region having a desert-like climate with a highly fluctuating daily temperature as well as a high annual temperature variation. Under such climatic conditions, the frequent heating (during nights) and cooling (during days) may be needed throughout the year. Much of these heating and cooling loads can be compensated by an EAHE system (Abbaspour-Fard, Gholami, and Khojastehpour Citation2011). Most probably, physiological activities of the plants may affect the performance of an EAHE system, as these activities can alter environmental conditions, mainly temperature and humidity, which are the most important operational parameters for the function of an EAHE system. To our knowledge, this aspect has not yet been considered elsewhere. In the present study, to evaluate the effect of evapotranspiration activity of existing plants in a greenhouse on the performance of an EAHE system, two parameters were considered as independent variables including: the area of the greenhouse and the percentage of vegetation coverage inside the greenhouse. The inside temperature, the thermal energy exchange and the COP of the system were considered as dependent variables in both cooling and heating modes.
2. Materials and methods
The effects of greenhouse area and the percentage of vegetation coverage inside the greenhouse were evaluated on the performance of an existing EAHE system. The EAHE system was constructed in the campus of the Ferdowsi University of Mashhad, which is the part of the Mashhad great plain. Mashhad is located at a latitude of 36.20°N and a longitude of 59.35°E and at 999.2 m above sea level. The region has about 250 mm of annual precipitation. Summers are typically hot and dry with an average temperature sometimes exceeding 35°C with the highest recording of about 43°C between the months of June and August. Winters are typically cold, with an overnight temperature routinely dropping below the freezing point. The air is driest during the month of August, when the relative humidity drops below 21%, and it is most humid around the month of January, with the relative humidity exceeding 89%. The area receives on average only 2800 h of sunshine annually (Abbaspour-Fard, Gholami, and Khojastehpour Citation2011). Table presents the details of the climatic conditions of the area.
Table 1 Climate data for the Mashhad region.
The EAHE system comprised a horizontal pipe of 0.1 m diameter with a total length of 18 m made up of galvanised mild steel pipes. This pipe was buried at a depth of 4 m in a flat land with unsaturated soil far above the water table. The two ends of the pipe were brought to the surface. The air was forced through the earth–air–pipe system using a blower, which was powered by a 550 W single-phase ELPROM electrical motor. The inlets of the galvanised pipes were connected to the blower. The outlets of the EAHE system were distributed uniformly by means of plastic tubes inside the greenhouse. The pipes were covered and insulated to reduce heat losses. The air temperature and velocity at the outlets were measured using a vane probe-type digital Prova AVM07 thermo-anemometer, ranging between 0.4 and 30.0 m/s with a least count of 0.3 m/s. The temperature accuracy and air velocity accuracy of this device were ± 1.0°C and 0.1 m/s, respectively, and it was equipped with an RS-232 interface for PC connection. The air temperature and the inlet temperature were measured using a digital thermometer with 0.1°C accuracy. The underground temperature was measured using the mercury thermometer with 0.5°C accuracy.
Adjacent to the EAHE system, three greenhouse units with metal frames being covered by plastic material (Figure ) and the floor area being 3 × 6 m2, middle height 2.5 m and side height 1.7 m were constructed. The greenhouse units were installed alongside each other to provide different floor areas of 3 × 3, 3 × 6 and 3 × 9 m2. To study the effects of vegetation coverage on the performance of the EAHE system in the greenhouse, grass was planted in the units in a sequential manner to provide three levels of the percentage of vegetation coverage: 0, 50 and 100%. In many instances, green grass has been used to calculate the reference evapotranspiration (Banayan et al. Citation2004). The grass used was a combination of 30% Lolium perenne Romco, 25% L. perenne Numan, 25% Poa pratensis Conni and 20% Festuca rubra Casanova varieties.
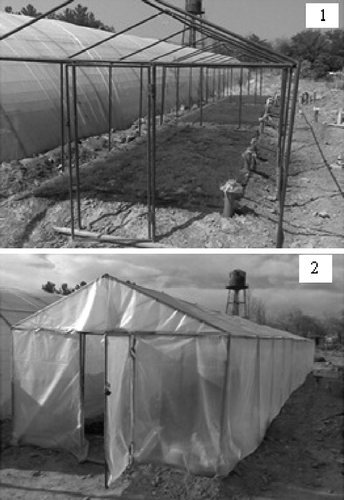
The experiments were conducted in two periods (summer cooling and winter heating). Therefore, grasses were planted during two months: mid-July for summer cooling and the end of January for winter heating. For creating identical conditions in the heating and cooling experiments, data recording commenced when the average length of the grass was 5 cm.
To evaluate the effect of greenhouse area, three different levels of floor area were set (9, 18 and 27 m2) by merging the smaller units with the larger ones. Subsequently, the effects of different levels of the percentage of vegetation coverage inside the greenhouse (0, 50 and 100%) were examined. A factorial experiment was conducted by using a randomised complete block design for evaluating the effects of the variables on the performance of the EAHE system. The experimental treatments are described as follows:
Percentage of vegetation coverage: D1 = 0%, D2 = 50% and D3 = 100%. | |||||
Floor area of the greenhouse: S1 = 9 m2, S2 = 18 m2 and S3 = 27 m2. | |||||
All treatments: D1S1, D1S2, D1S3, D2S1, D2S2, D2S3, D3S1, D3S2 and D3S3. |
The cooling mode experiment was performed in August 2010. During this period, data collection was conducted from day 9 to day 17. The heating mode trial was carried out in January and February 2011. During this period, data collection was performed from day 18 to day 6. Weather conditions were checked regularly via an accredited weather website to perform all tests under similar circumstances. During the experiments, the inside temperature of the greenhouse (TG), the control greenhouse (TGs), the ambient temperature (Ta), the air flow rate within the pipe, the inlet pipe (Ti), the outlet air temperature (To) and the soil temperature (Ts) were recorded. Subsequently, data analysis was performed using SPSS16 software.
The dependent variables were the temperature difference between the control greenhouse unit (with no EAHE) and the treatment greenhouse unit, and the COP of the system. The COP is a suitable criterion for the evaluation of the heat exchanger, which can be calculated as follows (Ashrae Citation1985):
3. Results and discussion
3.1 Cooling mode
In the first stage, the cooling mode experiment was performed in a closed-loop manner, i.e. the air inside the greenhouse was pumped through the pipes of the EAHE system and then back to the greenhouse. In other words, the air inside the greenhouse exchanged the heat with soil mass. However, due to a high relative humidity (generally above 85%) of the air inside the greenhouse (due to evaporation and transpiration of vegetation) as well as a lower soil temperature than the air temperature of the greenhouse, condensation occurred inside the pipes and subsequently the system was blocked by the condensed water. Hence, the closed-loop set-up was not feasible for carrying out the cooling mode experiment. So far, this phenomenon has not been reported by other studies. Therefore, an open-loop set-up was employed for the cooling mode experiment, i.e. the fresh ambient air was pumped into the EAHE system and the cooled air returned into the greenhouse. To avoid the increase in air pressure inside the greenhouse, a suitable outlet was installed on the greenhouse wall to allow the extra air to exit the unit.
At each hour during the day, the air temperature at the inlet and outlet of the EAHE system and the soil temperature were measured. The mean values of a 3-day-run cooling mode experiment conducted in an open-loop manner were obtained as shown in Figure .
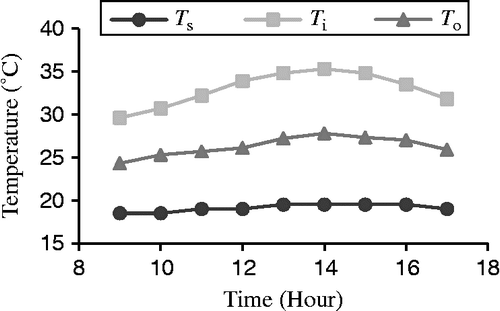
It was realized that during the day, the soil temperature in the cooling mode was almost constant, ranging from 19 to 20°C. Figure also shows that the inlet air of the EAHE system becomes colder at a temperature of about 6°C due to heat exchange with the soil mass. In addition, fluctuations in the outlet air temperature of the EAHE system are reduced and air with a uniform temperature enters into the greenhouse.
During the experiments, a similar greenhouse without the EAHE system was considered as the control unit. Figure shows the temperature differences between the treatment and control greenhouse units at the different levels of floor area. In Figure (a)–(c), Gs, D and S represent control greenhouse, vegetation coverage and floor area, respectively, and the numbers refer to the level of each variable.
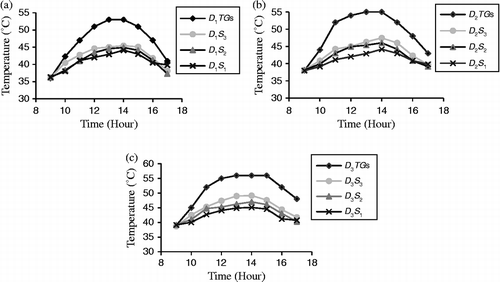
Temperature differences between the control and treatment greenhouse units are shown in Figure . Due to the constant airflow from the EAHE system into the greenhouse units, temperature differences were observed at the different levels of greenhouse floor area. Since the volume of the inside air increased in larger greenhouse units, more time was taken to receive the cooled air from the EAHE system. Therefore, smaller greenhouse units were cooler, as evident in Figure . In addition, there was a reduction in the peaks of the air temperature inside the greenhouse due to the cooling performance of the EAHE system. In the middle of the day, when cooling becomes very critical, the EAHE system caused a temperature difference of about 8–10°C between the control and treatment greenhouse units. Grass used as the reference crop has an evapotranspiration of 1500 mm per year (Banayan et al. Citation2004). Solar thermal energy is absorbed by greenhouse air due to the moisture resulting from the evapotranspiration of the grass. The humidity inside the greenhouse rises with increasing vegetation coverage. So, the temperature inside the greenhouse increases and hence a greater cooling load is needed.
3.2 Heating mode
In the heating mode, the condensation that occurs in the pipes of the EAHE system buried in the soil mass is not a problem as the temperature of the soil is higher than the temperature inside the greenhouse, so a closed-loop set-up was employed, i.e. the air inside the greenhouse was forced into the EAHE system and after heat exchange with the soil mass, the air returned into the greenhouse in a circulating manner. In the heating experiments, every 2 h, the ambient temperature and the soil temperature were measured during the night from 18 January to 6 February 2011. The mean values of 3-day measurements for the soil and ambient temperatures were obtained, as shown in Figure . During this time period of the year (in winter), the soil temperature was about 15.5–17°C in the night. The most effective factor of heat transfer to the air passing through the EAHE system is the temperature difference between the soil mass and the environment, as evident in Figure in the region under study.
During the experiments, a similar greenhouse without the EAHE system was considered as the control unit. Figure shows the temperature differences between the treatment and control greenhouse units at the different levels of floor area and vegetation coverage in the heating mode.
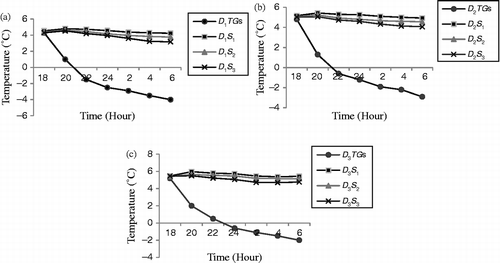
The temperature differences between the control and treatment greenhouse units are evident in Figure . At the beginning of the experiment, the temperatures between the control and treatment greenhouse units were almost equal. When the EAHE system begins to work, the temperature of the treatment greenhouse remains nearly constant, but that of the control greenhouse decreases with increasing time. In addition, there is a reduction of fluctuations in the air temperature of the greenhouse. During the night, the EAHE system caused a temperature difference of about 7°C between the control and treatment greenhouse units. The temperature required for some crops such as Calendula ranges from 4.5 to 7°C and that for Primrose ranges from 5.8 to 10°C during the night. Given the average temperature of 7°C in the greenhouse, suitable conditions can be partially provided for the two plants. However, for other plants, EAHE may be used to reduce the heating load of the greenhouse.
3.3 Statistical analysis of the experimental results
Statistical analysis of the experiments was performed using two factors, greenhouse floor area (S) and the percentage of vegetation coverage (D), each at three levels and with three replications in a factorial experiment based on a randomised complete block design. Furthermore, the analysis was performed in three ranges (9–11, 12–14 and 15–17) for the cooling mode and in two ranges (18–24 and 2–6) for the heating mode due to large temperature differences during the day and night.
For both cooling and heating modes, the effects of both greenhouse floor area and the percentage of vegetation coverage on temperature differences at all time intervals were significant, as summarised in Table . Furthermore, it can be observed that the mutual effects of these two parameters at the 1% level for all time intervals of the cooling mode were significant. However, in the heating mode, the mutual effects were significant at the 5% level for the range of 2–6, but they were not significant for the other range. This contrast was anticipated, because the evapotranspiration rate of the plant is much higher during the day than in the night when the cooling mode is active. These results indicate that the effective operation of an EAHE system is dependent on the size of the greenhouse. Moreover, as vegetation coverage strongly affects the performance of the EAHE system, it should be considered as a main design parameter and the system should be evaluated with full vegetation coverage.
Table 2 Summary of the temperature differences for the heating and cooling modes (mean square).
3.4 Heat transfer rate and the COP
Temperature difference shows only the maximum possible thermal gradient that can be obtained from an EAHE system. It does not exhibit the effective performance of the EAHE system. Therefore, in conjunction with this parameter, the heat transfer rate and the COP for the different treatments of the EAHE system were also compared. Table gives the details on the heat transfer rate and the COP for all the experimental treatments, using Equations 12(3).
Table 3 Heat transfer rate and COP of the different treatments of the EAHE system.
In general, from the viewpoint of heat transfer rate, the heating mode obtains a higher heat exchange than the cooling mode for all the treatments. However, on the basis of the COP, the cooling mode operates better than the heating mode. According to Table , the highest heat transfer rate of 6413 kJ/h was observed for the treatment D3S3 in the heating mode and that of 1502.2 kJ/h was found for the treatment D1S1 in the cooling mode, and the highest COPs of 1.01 and 4.32 were observed for the same treatments in both the heating and cooling modes, respectively. These results show the importance of greenhouse size and the percentage of vegetation coverage inside the greenhouse for the design of an EAHE system. In other words, although the increase in vegetation coverage during the cooling mode decreases the efficiency and performance of an EAHE system, it may be improved during the heating mode.
4. Conclusion
According to the results obtained from the experimental treatments and the control greenhouse, both percentage of vegetation coverage and greenhouse size significantly affect the performance and efficiency of an EAHE system. The highest temperature difference of 9.6°C was observed for a system with 100% vegetation coverage and 9 m2 floor area in the cooling mode and that of 7.3°C was found for a system with 50% vegetation cover and 9 m2 floor area in the heating mode. In general, although the heat transfer rate was higher during the heating mode, a better COP was observed during the cooling mode. Although the percentage of vegetation coverage negatively affected the cooling mode of the EAHE system, its performance was improved by the increase in vegetation coverage during the heating mode. This can be related to the evapotranspiration activity of the plants and the latent heat of the water vapour. The average COPs in the heating and cooling modes were equal to 0.91 and 3.67, respectively. In other words, the performance of the EAHE system was more than three times for the cooling mode than for heating. Although the heating mode had a much lower COP than the cooling mode, yet it is much better than any other conventional fossil fuel heating system such as unit heaters and central heating, from viewpoints of efficiency, energy balance, global heating and sustainability. On the other hand, it implies that the heating mode of the EAHE system is not reliable enough for greenhouse heating and should be used as an auxiliary system for conventional heating to reduce the heating load of the greenhouse.
Acknowledgement
The authors thank the Research Deputy of the Ferdowsi University of Mashhad (FUM) for financial and technical support.
Notes
1. Email: [email protected]
References
- Abbaspour-Fard, M. H., A.Gholami, and M.Khojastehpour. 2011. “Evaluation of an Earth-to-Air Heat Exchanger for the North-East of Iran with Semi-Arid Climate.” International Journal of Green Energy8: 499–510. 10.1080/15435075.2011.576289.
- Ashrae. 1985. Handbook of Application. Atlanta, GA: American Society of Heating Refrigerating and Air Conditioning Engineers. 30329 404-636-8400.
- Banayan, M., M.Jami Al-Ahmadi, B.Kamkar, A.Mahdavi Damghani, and M.Salehi. 2004. Principle of Tropical Agronomy. Mashhad: Ferdowsi University of Mashhad Press.
- Bansal, N. K., M. S.Sodha, and S. S.Bhardwaj. 1983. “Performance of Earth–Air Tunnel System.” International Journal of Energy Research7 (4): 333–341.
- Bharadwaj, S. S., and N. K.Bansal. 1983. “Temperature Distribution Inside Ground for Various Surface Conditions.” International Journal of Building and Environment16 (3): 183–192. 10.1016/0360-1323(81)90012-3.
- Gauthier, C., M.Lacroix, and H.Bernier. 1997. “Numerical Simulation of Soil Heat Exchanger Storage Systems for Greenhouses.” Journal of Solar Energy60: 333–346. 10.1016/S0038-092X(97)00022-4.
- Kozai, T.1989. “Thermal Performance of a Solar Greenhouse with an Underground Heat Storage System.” Journal of Acta Horticulture257: 169–182.
- Kumar, R., S. C.Kaushik, and S. N.Garg. 2006. “Heating and Cooling Potential of an Earth-To-Air Heat Exchanger Using Artificial Neural Network.” Renewable Energy31 (8): 1139–1155. 10.1016/j.renene.2005.06.007.
- Midilli, A., I.Dincer, and M.Ay. 2006. “Green Energy Strategies for Sustainable Development.” Energy Policy34 (18): 3623–3633. 10.1016/j.enpol.2005.08.003.
- Omer, M. A.2008. “Ground-Source Heat Pumps Systems and Applications.” Journal of Renewable and Sustainable Energy Reviews12: 344–371. 10.1016/j.rser.2006.10.003.
- Ozgener, L., and O.Ozgener. 2010. “An Experimental Study of the Exergetic Performance of an Underground Air Tunnel System for Greenhouse Cooling.” Journal of Renewable Energy35: 2804–2811. 10.1016/j.renene.2010.04.038.
- Santamouris, M., C. A.Balaras, E.Dascalaki, and M. V.Allindras. 1994. “Passive Solar Agricultural Greenhouses: A Worldwide Classification and Evaluation of Technologies and Systems Used for Heating Purposes.” Journal of Solar Energy53: 411–426. 10.1016/0038-092X(94)90056-6.
- Sethi, V. P., and S. K.Sharma. 2008. “Survey and Evaluation of Heating Technologies for Worldwide Agricultural Greenhouse Applications.” Journal of Solar Energy82: 832–859. 10.1016/j.solener.2008.02.010.
- Sharan, G., and R. Jadhav. 2002. “Soil Temperature Regime at Ahmedabad.” IIMA Working Paper No. WP2002-11-02, Research and Publication Department, Indian Institute of Management Ahmedabad.
- Sharan, G., H. Prakash, and R. Jadhav. 2003. “Performance of Greenhouse Coupled to Earth Tube Heat Exchanger in Closed-Loop Mode.” Paper presented at the XXX CIOSTA-CIGR V Congress, Vol. 2, Proceedings on Management and Technology Applications to Empower Agriculture And Agro-Food Systems, Turin, Italy, pp. 865–873.
- Tiwari, G. N., M. A.Akhtar, A.Shukla, and K. M.Emran. 2006. “Annual Thermal Performance of Greenhouse with an Earth–Air Heat Exchanger: An Experimental Validation.” Renewable Energy31 (15): 2432–2446. 10.1016/j.renene.2005.11.006.