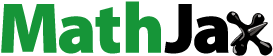
ABSTRACT
This paper aims at analysing the utilisation of biodiesel for the case of bus rapid transit system (BRTS) in Ahmedabad, India, through the social benefit cost analysis (SBCA) and life cycle cost analysis (LCCA) tools. The SBCA and the LCCA have been performed for diesel and biodiesel blends B0, B5, B10, B15, B20, B30, B50 and B100. The study reveals that the overall social benefit cost ratio is close to 0.7 for diesel-based transit and can be marginally improved to 0.77 by adding biodiesel in various proportions. Given the easy transition of diesel-based transit to biodiesel-based transit coupled with improvement in benefit cost ratio, it is suggested to transition from diesel to biodiesel blended fuel. However, since the benefit cost ratio is less than 1.0, a need for alternative financial model development is identified. It is also deducted that the fuel conversion is one way to sustainable development, however, looking to the nature of social benefits; the step to development of BRTS instead of conventional bus transport can also aid sustainable development in a major way.
Introduction
An alternative form of energy for the transport sector is biofuels, also referred as biodiesel which are derived from natural sources like crops which can be replenished more often than fossil fuel-based sources such as diesel and petrol. A variety of feedstock, for example, corn, wheat, sweet sorghum, cassava, sugarcane, soybean, rapeseed, Jatropha can be used individually or in a controlled form for the purpose of locomotion. The feedstock may be first generation (vegetable oils, corn, sunflower oil, etc.), second generation (agriculture and forest residues, grass, waste vegetables oil, Jatropha, etc.) or third generation (algal biomass) and depending on that their effects may vary. Being renewable and replenishable in nature, the biofuels appear lucrative from point of view of energy security and energy independence of the nation. With the advent of the National Policy on biofuels-2018, it is clear that the Government of India encourages biofuels as an alternative to crude oil. The highest consumption of imported and petro-based oil is found in the transportation sector and therefore an obvious indication is made to modify the current fossil fuel practices for transportation. A suggested 20% blending of biofuels is found in the policy but varies in practice at fuel stations in India. Enabling mechanisms to encourage biofuels such as minimum support price for biodiesel oil seeds, species identification, standards and certifications, surplus production to ensure availability and other financial, fiscal, and institutional frameworks in this direction were indicated in the policy document. The present paper aims at exploring the possibilities of using biodiesel in bus rapid transit system (BRTS), India, and to compute social benefit cost analysis (SBCA) and life cycle cost analysis (LCCA) of the same to find out the financial and technical feasibility of the project.
Literature review
Biodiesel as alternative fuel technology
The available literature on biofuels include technical, environmental and economic feasibility; energy and emission effects of biofuels; feedstock types, blends and fundamental performance effects; effect of nanoparticles and other additives on biofuels, and other sustainability effects. Gebremariam and Marchetti (Citation2018) in their paper on economics of biodiesel production clearly reflect that biodiesel is not a complete substitute of diesel, mainly because of its higher cost of production. Equipment cost is a major component of capital investment cost of the biodiesel plant, and cost of feedstock is a major component of operational cost of the biodiesel plant.
Economic and environmental benefits have been recommended by researchers through technology improvement. For instance, Caliskan (Citation2017) recommended the use of genetically modified biodiesel with an aim to have better enviro-economic gains. Technology improvement for India and other countries has also been suggested looking into other sustainability aspects. Dwivedi et al., (Citation2011) recognised Pongamia as a potential feedstock for biodiesel production in India given its various practical advantages such as non-edibility, ability to grow in all types of soil, requirement of small input and management, good productive life, high percentage yield and so forth. In another instance, Sharma and Singh (Citation2017) suggest microalgae as suitable feedstock for India to have less impact on the use of fertile land, competition with food crop, GHG emission reduction potential and international dependency. International practices of microalgae-based biodiesel productions have been reviewed by them and suitable technological evolvement identified.
An interesting temporal study by Naylor and Higgins (Citation2017) presents the last decade trend of biodiesel and predict the risky scenario for the coming decade. They establish the relative growth of biodiesel production in the decade of 2005–2015, at a good 23% per annum. It is indicated that this could be a result of high crude oil prices (which have gone down since 2014). It is also indicated that this dramatic biodiesel production increase has occurred due to strong policy directives, subsidies, trade policies, agricultural interest protection, rural economic development, energy security and climate targets. The authors present several country-specific case studies and predict the 2020 onward scenario as risky and market-wise uncertain due to large social costs dominating over policies. This indicates all the more reason to explore the social benefits and costs as opposed to the 16% fuel subsidy offered by the Government of India on biodiesel.
For the same decade spanning 2005 to 2016, Moreno-Pérez et al., (Citation2017) studied the business concentration of biodiesel industry in Brazil in particular and found that the period from 2005 to 2011 marked a deconcentration in Brazilian biodiesel industry whereas from 2011 to 2016 indicated stability. The research also illustrates that biodiesel is produced by fewer companies and plants with the passage of years and different business models exist among them. Similar potential and need for biodiesel has been identified for China but as reviewed by Xu et al., (Citation2016), the demand is not being met and continuous capital is required for the cause. The same nature of recommendations, such as raw materials’ production capacity boosting, regional dependencies, standards, tax policies and enforced applications was suggested for China as well. Similar reviews have been illustrated by other researchers on the shortfall of incentives and price reductions to boost biodiesel usage. For instance, a review of biodiesel potential for Serbia by Đurišić-Mladenović et al. (Citation2018) revealed there is sufficient production provision for oil seed biodiesel without hampering the food and fodder industry, alternatives for eliminating ‘food versus fuel’ debate were presented but moreover, it was identified that though potential exists, governmental incentives are not lucrative for biodiesel promotion in reality and therefore they need to be strengthened to fulfill the demand. Similarly, Drabik et al. (Citation2014) evaluated the effect of three different biodiesel interventions which were a biodiesel mandate, a tax exemption and an exogenous diesel price on the world biodiesel process. The analysis indicated that the tax exemption is more influential than the mandate and the regulation of diesel prices.
Further, Gracia et al., (Citation2018) identify attitude factors such as use, knowledge and self-identity for market acceptance and establish the role of convenience (like to fuel station availability) and price for enhancing biodiesel use. Although the attitude factors may be more sensitive to private vehicle users, the convenience and price variables influence ground-level decisions for BRTS as well. All these factors seem to have been integrated in the model by Hombach et al., (Citation2018) where the importance of combining political regulations with sustainability objectives has been recognised and for doing so a robust and sustainable biofuel supply chain model has been formed and applied to German biodiesel market. As part of this application, the decision-maker’s risk attitude is shown to play an important role.
Life cycle cost assessment for alternative fuel technologies
LCCA is a decision-making tool which can be effectively used to establish the economic feasibility of a project. Berawi et al. (Citation2016) employed it for the energy infrastructure of Indonesia on a timescale indicating a positive move towards transportation sector sustainable development. Much research and testing are being conducted worldwide exploring the alternative mechanisms for vehicle transportation. Many debates have been held, and views expressed on the topic of mobility will lead to lower local emissions, and is accepted as an environmentally friendly practice compared to diesel-based mobility, there have been concerns over energy security, as well as the environmental pollution caused by the fossil fuels used for transportation. Energy security can be enhanced by promoting more usage of renewable energy. One of the significant causes of the slow pace of the adoption is the economics involved. The sustainable development of infrastructure projects such as mass rapid transit systems (MRTS) is a challenge if the mode of the operation is renewable energy based, such as electric or biodiesel vehicles. Demands for such projects need to be studied and forecast. Husin et al. (Citation2015) also applied a system dynamic approach to forecast the demand on mega infrastructure projects.
Adheesh et al. (Citation2016) studied the feasibility of electric buses compared to conventional diesel buses in Bangalore, India. The initial investments in electric buses were found as much higher than for conventional diesel buses. The operation and maintenance costs of electric buses were also found as very high. A study on the total cost of ownership and externalities for conventional, hybrid, and electric vehicles by Mitropoulos et al., (Citation2017) suggests a LCCA method, which includes the external costs of emissions with more detail on the comparison with light-duty vehicles. As an outcome of the study, the researchers have a strong view on the dynamism of transport policies with respect to regional data. According to Helmers et al., (Citation2017), most of the attempts at LCA by past researchers have been on generic vehicles. Their work aimed at developing and quantifying LCA through the real-time data of vehicles in use globally, with data pertaining to energy consumption before and after the conversion of vehicles.
Nordelöf et al. (Citation2014) conducted a detailed literature review of the usefulness of the different methods that can be used for the life cycle assessment of the different electric vehicles. In addition, Steubing et al. (Citation2016) developed a modular LCA approach to optimise the vital parameters involved in and associated with the value chain in the sustainable transportation sector. Bauer et al. (Citation2015) made an assessment of the environmental performance of vehicles used at present, which was intended as used in future by computing the life cycle cost of all the available modes using a novel scenario analysis framework. Heidrich et al. (Citation2017) studied the effectiveness of the policies and mitigation strategies pertaining to climate change which might support the uptake and use of electric vehicles. Bubeck et al. (Citation2016) made an attempt to compute the TCO of electric vehicles in Germany. Their study highlighted that electric vehicles can be a feasible and attractive option for achieving the climate change mitigation targets of the EU and the German government. Furthermore, Jochem et al. (Citation2016) computed the external cost components that affect electric vehicles. These costs include those of accidents, congestion, environmental pollution, climate change and noise. They observed that in most cases these costs are lower than those incurred by internal combustion engine vehicles.
LCCA research work on diesel and electric public transport buses in the United States by Cooney et al. (Citation2013) made strong suggestions to focus on the electricity mix in the grid, while recommending the use of electric buses.
One of the challenges faced in the study is arriving at the monetisation of costs of air pollution, both for the use of diesel and for electricity generation. The external costs of emissions have been adopted from the research of Matthews et al., (Citation2001), who estimated the unit of social damage due to air pollution from the transportation sector. Karaaslan et al., (Citation2018) developed a hybrid input-output LCCA method to estimate the impacts on the environment, primarily greenhouse gas emissions, water consumption, and the energy consumption of sports utility vehicles. The hybrid LCCA model was supplemented by sensitivity analysis using Monte Carlo simulation. Moro and Helmers (Citation2017) developed a new hybrid method for reducing the gap between the well-to-wheel (WTW) method and LCCA to assess the greenhouse gas (GHG) emissions from vehicles on the road.
Benefit cost assessment techniques for alternative fuel technologies
Benefit cost analysis has historically been applied to evaluate alternative transport projects and aided in decision-making and policy formulations with considerations of equity, use of discounted cash flow techniques and sensitivity analysis for cost benefit study of the future (Barrell and Hills, Citation1972). Although more recently, the concept of total cost analysis over traditional cost benefit analysis for comparing transport projects has also been recommended (DeCorla-Souza et al., Citation1997) where all the monetizable cost components including ‘cost savings’ are aggregated (these were referred to as ‘benefits’ in traditional analysis techniques).
Many researchers have brought into discussion the challenges associated with cost benefit analysis as a technique and the capacity of CBA frameworks in decision-making. Shortfalls such as incomplete analysis, uncertainty and difficulties of effect estimations (Mouter et al., Citation2015), the sensitive question of uncertainties in the cost benefit assessments and their influence on public infrastructure investment decisions (Asplund and Eliasson Citation2016), the methodological issues and corresponding models for ex-ante project assessment of cost-benefit analysis for capital intensive infrastructures (Florio and Sirtori Citation2016), critical questions on the importance of qualitative impacts including social, human life, natural and built environment over quantitative assessment (Hickman and Dean Citation2017) and on the equity perspectives (Martens and Floridea Citation2017) have been addressed in the context of cost-benefit method for project appraisal.
Other researchers have commented on the CBA technique as applied to assess the economies on large scale and organisational changes in infrastructure projects such as public urban transit (Viton, Citation1993) and the microeconomic and macroeconomic analyses tools for transportation sector as an attempt for broader (regional) economic benefits of new transportation investment (Anas et al., Citation2016). A broader approach for the evaluation of economic benefits of urban infrastructure with an integrated four-pronged approach accounting for the effects of consumption investment, government purchase effect and external demand (Sun and Cui, Citation2018a) reflected the potential of coordinated development and the same was illustrated (Sun and Cui, Citation2018b) regarding economic, social and environmental benefits.
In the particular context of MRTS, it has been illustrated that the indirect social effect such as ridership can work towards better benefits (White et al., Citation1992) and, on the other hand, subsidies and public ownership models for mass transit (Pucher and Markstedt, Citation1983) can also lead to wasteful cost escalation. And it has also been recommended that cost-benefit analysis should be used for regional public transport planning in healthy economies as well (Johansson et al., Citation2017). The social, economic and environmental impacts of public transportation have also been reviewed for meeting sustainability goals of cities and regions, and recommendations on sustainable performance of public transit have been also made (Miller et al. Citation2016).
Reviewing the available literature, it has been observed that the research and pilot projects available in literature indicate either small-scale specific experimental technicalities like combustion performance of a biodiesel feedstock or large-scale economic evaluation at the scale of the nation’s demand supply potential. If the technology was to be applied, it would be required to have a sense of feasibility at project scale and evaluate the impact of technology on the proposed sustainable project. To address this gap, this paper analyses and presents a systematic case evaluation considering the life cycle socio-economic-environmental aspects of biodiesel project appraisal. The project being considered in this paper is the BRTS in Ahmedabad, India. Two methods of analyses, namely, social benefit cost assessment (SBCA) and life cycle cost assessment (LCCA) have been used to deduct the potential for switching over to biodiesel as a fuel for urban mass transit.
Problem statement
The objective of this research paper is to explore the feasibility of use of green fuel like biodiesel for public mode of transportation. Detailed analyses have been carried out by the application of two decision-making tools like SBCA and LCCA to find out the economic feasibility of switching over from convention mode (use of diesel) to green mode (use of biodiesel). The biodiesel feedstock represented herein is palm stearin, a first-generation biodiesel. The comparisons made in this study are for the present scenario of diesel fuel only and for various percentages of diesel substituted by biodiesel.
Methodology
The methodology adopted for this research work is primary data research where the primary data are collected from BRTS, Ahmedabad. This primary data pertaining to total daily vehicle kilometres for Ahmedabad BRTS; total number of buses; daily ridership; average ridership fare; advertisement tariff; mode split percentage of two-wheelers and four-wheelers; vehicle operation cost of diesel buses; average trip length in Ahmedabad; average speed of bus in mixed traffic; average speed of BRTS bus; average number of LCCA for BRTS operated with bio-diesel. The detailed methodology is highlighted through trips per day; fuel consumption or mileage of the BRTS bus. This data is used for computation of SBCA and the flowchart is presented in and . Sources of data include Ahmedabad Janmarg Limited (Citation2016, Citation2018), Delhi Metro Rail Corporation Ltd (Citation2014), the Automative Research Association of India (Citation2008), the Ministry of Road transport and Highways in India (Citation2015) and Ministry of Urban Development in India (Citation2016). Secondary data pertaining to monetisation and valuation of environmental and social benefits and biodiesel plant details have also been used. Research done by Lele (Citation2019), Mahadevia (Citation2013) and Murty et al. (Citation2006) have also been used as secondary data sources.
Case study and analysis
The case of Biodiesel- BRTS-Ahmedabad is evaluated for the purpose of looking at various quantifiable aspects of sustainability, and this is represented by SBCA. For this purpose, data pertaining to BRTS-Ahmedabad system and data pertaining to biodiesel fuel requirements (such as plant cost, operation cost, fuel cost, etc.) have been collected and presented in the next section. The present worth analysis in form of life cycle cost is also performed for the diesel and biodiesel situation to evaluate the impact. Many intangible parameters that remain silent in quantitative studies are beyond the scope of this paper but have been identified in the form of 20 indicators. The basic data for the Ahmedabad BRTS have been collected from various sources as listed at the end of the article. Further, transit calculations have been performed, and the following data used for SBCA and LCCA are presented in .
Table 1. Data used for LCCA and SBCA computations
The basic data collected and assumed for biodiesel implementation is presented in .
Table 2. Data for biodiesel implementation
The blends of diesel and biodiesel used in the analysis are stated in .
Table 3. Blends of diesel and biodiesel considered
illustrates the reduction in pollution and external costs associated with it in million INR for diesel bus and biodiesel bus functioning.
Table 4. Amount of pollution reduction and external costs of pollution
The total cost of pollution for various blends of diesel and biodiesel used for operation of the buses is illustrated in .
Table 5. External costs of pollution for various blends
Biodiesel plant cost
Biodiesel plant cost ( indicates the Biodiesel plant operation cost which will depend on the amount of biodiesel and per litre operation cost listed in the data section earlier.
Table 6. Biodiesel plant operation cost
Fuel benefits and costs
and respectively, indicate the amount of annual benefits and annual costs in a million INR accrued due to usage of biodiesel as fuel wholly or partially for BRTS operation.
Table 7. Benefits accrued due to biodiesel blending
Table 8. Costs due to biodiesel blending
Social benefit cost analysis (SBCA)
Benefits or costs accrued in the overall biodiesel BRTS Ahmedabad project may or may not be caused due to fuel conversion. For instance, reduction in the cost of accidents may be attributed to the construction of a corridor, and reduction in vehicle operation cost may be attributed to the inception of the BRT itself as opposed to environmental benefits attributed to fuel switchover from diesel to biodiesel. The present worth of benefits and costs is then computed using the relationships:
where
PWRB: Present worth of revenue benefits (million INR);
RR: Ridership revenue (million INR);
AR: Advertisement revenue (million INR);
i: Discount rate (%);
n: Life cycle (years);
PWC: Present worth of costs (million INR);
CDC: Corridor development cost (million INR);
CBP: Cost of bus procurement (million INR);
CBPI: Cost of biodiesel plant installation (million INR);
CIM: Cost of infrastructure maintenance (million INR);
CSO: Cost of transit system operation (million INR);
CSM: Cost of transit system maintenance (million INR);
CBPOM: Cost of biodiesel plant operation and maintenance (million INR);
CITMS: Cost of intelligent transport management system (million INR);
Litms: Life of ITMS replacement (years);
CBR: Cost of bus replacement (million INR);
Lb: Life of bus replacement (years);
FBCR: Financial benefit cost ratio;
FG: Financial gap (million INR);
PWVSB: Present worth of vehicle operation and fuel cost saving benefits (million INR);
VOSd: Vehicle operation cost savings of diverted vehicles (million INR);
FSd: Fuel consumption saving of diverted vehicles (million INR);
PWEB: Present worth of environmental benefits (million INR);
EBd: Environmental benefits due to diverted vehicles (million INR);
EBb: Environmental benefits due to buses (million INR);
PWSB: Present worth of social benefits (million INR);
SBtt: Social benefits due to travel time reduction (million INR);
SBa: Social benefits due to accident reduction (million INR);
SBCR: Social benefit cost ratio.
Identification of benefits with and without fuel switchover
Therefore, the benefits have been classified into two categories, (a) Incoming funds that are benefits not caused due to fuel conversion and (b) benefits caused due to fuel conversion. A sample calculation for B20 blend has been illustrated in .
Table 9. Sample calculation for b20 blend (benefits)
Identification of costs with and without fuel switchover
The costs have been classified into two categories: (a) Outgoing funds that are costs not caused due to fuel conversion and (b) costs caused due to fuel conversion. A sample calculation for B20 blend has been illustrated in .
Table 10. Sample calculation for b20 blend (costs)
Computation of overall SBCR and SBCR due to fuel switchover
indicates summary of present worth of all benefits and costs accrued with and without fuel switchover for all blends. indicates the SBCR of the overall project considering all benefits and costs for all blends, whereas indicates the SBCR of the project because of fuel conversion considering these particular benefits and costs for all blends.
Table 11. Summary of present worth of benefits and costs with and without fuel switchover
Table 12. SBCR of overall project for various blends
Table 13. SBCR considering the benefits and costs due to fuel conversion only
Life cycle cost analysis (LCCA)
Life cycle cost assessment (LCCA) of biodiesel BRTS Ahmedabad project has also been carried out using present worth analysis. It is quite likely that the bus operations and the plant operations may be governed by different regulating bodies, and therefore, the LCCA for both has been presented separately. The combined effect has been evaluated in the results and discussion section later. The cash flow diagrams pertaining to the overall biodiesel scenario, biodiesel bus operation and biodiesel plant operation scenario are indicated in .
Ca1 :Annual cost comprising diesel-biodiesel fuel blend, operation and maintenance, environmental and financing costs.
Ca2 :Annual cost of biodiesel plant operation and maintenance.
Ci :Initial cost of bus procurement and biodiesel plant set up.
Crbus :Cost of bus replacement
Ca :Annual cost comprising biodiesel fuel, operation and maintenance, environmental and financing costs.
Ci :Initial cost of bus procurement.
Crbus :Cost of bus replacement.
Ca :Annual cost comprising biodiesel plant operation and maintenance and financing costs.
Ci :Initial cost of biodiesel plant set up.
The life cycle cost is computed as per following relationship,
where
LCC: Life cycle cost (million INR);
PWcap: Present worth of capital costs (million INR);
PWann: Present worth of annual costs (million INR);
PWot: Present worth of one time costs (million INR);
PWfin: Present worth of financial costs (million INR).
Sample calculation for computation of SBCR and LCC is presented in Appendix 1.
Result and discussion
The life cycle cost of the process of using diesel fuel technology or biodiesel or biodiesel–diesel blends is about equal and to the order of 26,000 million INR for 25 years life at 12% discount rate. This represents about 101 million INR per kilometre of corridor for diesel transit to about 126 million INR per kilometre of corridor with biodiesel blending. The blending of biodiesel with diesel can significantly save the life cycle environmental cost which may amount to 2 to 12 million INR per kilometre depending on the percentage of diesel that is being replaced with biodiesel. The major life cycle cost component identified for both types of fuel technology is the financing cost of the transit system which amounts to about 40% to 45% of the total life cycle cost. The next major component of the life cycle cost is attributed to the cost of transit system operation and maintenance, amounting to about 28% to 42% of the total life cycle cost. The environmental cost savings accrued due to biodiesel fuel technology can amount from 2% to 10% of the life cycle cost depending upon the percentage of diesel being replaced by biodiesel.
illustrates the distribution of life cycle benefits of B20 blend biodiesel-based transit system over 25 years. As can be identified, various social benefits which are otherwise neglected constitute a major percentage in influencing the appraisal of the biodiesel system. Environmental benefits though expected contribute in an insignificant way in changing the benefit situation of the biodiesel transit.
illustrates the distribution of life cycle costs of diesel-based transit system. The life cycle cost components of a diesel BRTS consist of (a) capital cost of bus procurement at the starting of the project, (b) one-time costs of bus replacement at every 8 years, (c) annual costs of bus transit system operation and maintenance and (d) financing charges of the capital and annual costs. The major observation that can be made through the life cycle cost assessment of diesel BRTS is that although all costs are comparatively significant, the financial charges or costs are as high as the operation and maintenance (O&M) costs. Although the cost of diesel has been considered as same over the 25 years life cycle, present trends indicate that they are expected to rise in the near future.
illustrates the distribution of life cycle costs of B20 blend biodiesel-based bus transit system over 25 years. The life cycle cost components of a biodiesel-blended BRTS consist of (a) capital cost of bus procurement at the beginning, (b) one-time costs of bus replacement at every 8 years, (c) annual costs of bus transit system operation and maintenance, (d) capital cost of biodiesel plant, (e) annual cost of biodiesel plant operation and maintenance and (f) financing charges of the capital and annual costs. Similar observation can be made through the life cycle cost assessment of biodiesel BRTS on the high amount of the financial charges or costs accrued by both the biodiesel plant and the biodiesel transit system. However, as opposed to diesel, biodiesel fuel prices have been subsidised by the Government of India and are not expected to escalate in the near future. It may also be noticed that the subsidy on fuel is not sufficient to reduce the life cycle costing and more incentives especially for abating financial charges are required.
Whereas the high costs of transit system operation and maintenance may be difficult to avoid, the cost of financing the biodiesel transit system based on sustainability parameters is needed to be explored to bring a significant difference in life cycle costing of diesel and biodiesel blended fuel technologies. While shifting to the B15 blend to B85 blend, it can also be observed there is a significant rise in the cost of biodiesel plant operation and maintenance. This is attributed to the high cost of feedstock that forms the major sub-component of the biodiesel plant O&M cost. Therefore, there is a need to incentivise this part of the technology through the national biofuel policy.
The benefits for a diesel BRTS can be considered in two major categories: (a) economic and (b) social. The economic benefits are ridership and advertisement benefits, and vehicle operation cost-saving benefits. The vehicle operation cost-saving benefits are accrued because of ceasing of diesel buses and some percentage of diverted traffic reduced because of BRTS. The social benefits include accident reduction and travel time reduction. As opposed to that, the costs include (a) capital cost of corridor development and bus procurement, (b) annual cost of infrastructure maintenance, (c) one-time costs of bus replacement every 8 years and intelligent transport management system (ITMS) replacement every 7 years and (d) annual cost of system operation and maintenance.
illustrates the cash flow of all benefits and costs of the B20 blend of biodiesel BRTS over 25 years. The benefits for a biodiesel BRTS include environmental benefits of air pollution reduction in addition to economic and social benefits. The components of social benefits are the same as diesel BRTS. The economic cost component of biodiesel-based BRTS additionally includes the capital cost of biodiesel plant, biodiesel plant operation and maintenance cost and biodiesel transit system operation and maintenance cost. In addition, the biodiesel BRTS also offers some environmental benefits due to reduction of pollutant emissions.
The revenue model which is based on social marginal pricing of the infrastructure contributes to about 35% to 42% of the total benefits. A major share of benefits is accrued due to the advantages offered by BRTS alone, which amount to about 50% to 60% of the total benefits. The environmental benefits due to biodiesel blending amount to less than 4% and may be considered negligible. Similarly, whereas the costs associated with the implementation of the BRTS in general, such as corridor development, infrastructure maintenance cost, intelligent transport management system (ITMS) equipment replacement costs and system operation, and maintenance costs are significant and unavoidable, some additional costs of biodiesel plant may be expected. For the conversion of existing BRTS to biodiesel BRTS, since the costs of corridor and related infrastructure are already incurred, the investment regarding addition of biodiesel plant should be seen as a value addition to achieve environmental friendliness and energy security. However, for the new or proposed BRTS, the corridor cost incurred itself is more than 50% of the total costs.
The social benefit cost ratios of diesel-based transit are close to 0.7 and can go up to 0.77 with increasing blends of biodiesel. Although feasibility becomes a concern for less than 1.0 BCR, the existing situation of diesel will be improved by the use of biodiesel. By addressing the revenue model or alternative financial model, this figure can be improved. It can also be observed that the fuel conversion can contribute to the improvement of at least 20% of the BCR for B20 blend and increase to as much as 70% of the BCR with B100. Major contributions of benefits due to the existence of BRTS corridor itself have been observed owing to accident reduction and travel time savings.
Conclusion
The analysis carried out in this paper reflects upon the usage of biodiesel in different proportions for the scale of bus rapid transit in a metropolitan city in India. This paper mainly looks at the feasibility perspective on project scale through the instrument of social benefit cost ratio and life cycle cost assessment.
The national biofuel policy suggests the B20 blend as an indicative target. It may be noted that the state of Gujarat (as applicable to the case study), in particular, has registered very less number of biodiesel plants as well as biodiesel fuel stations.
The SBCR indicates an overall value of 0.72 for the presently recommended biodiesel blend (B20). This recommendation of B20 blend is based on the practical considerations of biodiesel availability and ease of technology transition. Use of B20 blend can be improved marginally by increasing proportion of biodiesel in the blended fuel. Since the SBCR falls short of unity, it is recommended that the biodiesel-based transit should be supported by more effective policies and incentives to promote the sustainability effect. The conversion of diesel to biodiesel B20 blend may be considered as an easy transition and should definitely be considered for the near future option for the existing BRTS. However, higher blends of biodiesel need to be evaluated prudently for the continuous availability on the scale of mass transit system before mandating. For a new or extended BRTS project, it is found that the major benefits of the system are due to the feature of the dedicated BRTS corridor and so, for the new projects and for farther future, other technologies such as electric BRTS may also be compared for viability before developing new infrastructure.
The life cycle cost assessment reveals that the only cost savings concerning external cost of environmental pollution are insufficient to compensate for the much larger costs of conversion. The most interesting revelation by the LCCA technique is the high amount of contribution that can be attributed to financing costs. Both in case of biodiesel bus operation and in case of biodiesel plant operation, it has been identified that other supporting financing mechanisms such as soft loans will be required if at all this technology transfer is to be implemented in the city. Further, it is envisaged that the regulatory authorities of biodiesel plant and bus operations will be different in which case a long time public–private partnership model may be imperative for sustained operations.
Scope for future research
It is a well-known fact that most of the biodiesel projects have not been approved by IPCC’s clean development mechanism (CDM). However, it is recommended that some qualitative value associated with this project be measured and a new method for evaluating such projects on factors in addition to emissions being developed and proposed for CDM consideration. It is also suggested that the evaluation be done at various scales of application to find an optimum trade-off for technology transfer.
Disclosure statement
No potential conflict of interest was reported by the authors.
Additional information
Notes on contributors
Anal Sheth
Dr. Anal Sheth is former PhD student of Department of Civil Engineering, School of Technology, Pandit Deendayal Petroleum University, Gandhinagar, India. She is also working as Adjunt Professor, Faculty of Technology, CEPT University, Ahmedabad, India. Her research interests include sustainable transportation, sustainable development and transportation engineering.
Debasis Sarkar
Dr. Debasis Sarkar has been working as Associate Professor and former HOD of Department of Civil Engineering, School of Technology, Pandit Deendayal Petroleum University, Gandhinagar, India. His research interests are sustainable development, sustainable transportation, built enviornment, Building Information Modelling (BIM), project management, project risk management and green building materials and technology.
Indrajit Mukhopadhyay
Prof. Dr. Indrajit Mukhopadhyay has been working as Professor in Department of Solar Energy, Pandit Deendayal Petroleum University, Gandhinagar, India. His area of interests are high energy density Li ion battery, solar hydrogen generation and thin film solar cells in the Solar Research and Development Center, PDPU.
References
- Adheesh, S. R., V. M. Shravanth and S. K. Ramasesha. 2016 “Air- Pollution and Economics: Diesel Bus Versus Electric Bus” Current Science. 858:862.
- Ahmedabad Janmarg Limited. 2016. “Socio Economic Costs Benefit Analysis of AhmedabadBRTS.” Accessed 03 July 2018. http://shodhganga.inflibnet.ac.in/bitstream/10603/189686/14/15_chapter6.pdf
- Ahmedabad Janmarg Limited. 2018. “Request for Proposal (RFP) for Selection of Bus Operator for Procurement, Operation and Maintenance of Midi Ac Fully Built Pure Electric Buses on Gross Cost Contract Basis.” Accessed 03 July 2018. http://www.ahmedabadbrts.org/web/documents/Vol-III_Bus%20Specifications_RFS%202.pdf
- Anas, R., O. Tamin, and S. Wibowo. 2016. “Applying Input–Output Model to Estimate Broader Economic Impact of Transportation Infrastructure Investment.” Journal of the Institution of Engineers (India): Series A-Civil, Architectural, Environmental and Agricultural Engineering 323: 331.
- Asplund, D., and J. Eliasson. 2016. “Does Uncertainty Make Cost-benefit Analyses Pointless?” Transportation Research Part A. Policy and Practice 195: 205.
- Automative Research Association of India. 2008. “Draft Report on Emission Factor Development for Indian Vehicles.” Central Pollution Control Board/Ministry of Environment and Forests. Assessed 15th July 2018. https://theicct.org/sites/default/files/publications/ICCT_IndiaRetrospective_2013.pdf
- Barrell, D. W. F. and P. J. Hills. 1972. “The Application of Cost- Benefit Analysis to Transport Investment Projects in Britain” Transportation. 29 :54.
- Bauer, C., J. Hofer, H.-J. Althaus, A. Del Duce, and A. Simons. 2015. “The Environmental Performance of Current and Future Passenger Vehicles: Life Cycle Assessment Based on a Novel Scenario Analysis Framework.” Applied Energy 871: 883.
- Berawi, M. A., P. B. Miraj, A. R. B. Silva, and F. Darmawan. 2016. “Towards Self –sufficient Demand in 2030: Analysis of Life-cycle Cost for Indonesian Energy Infrastructure.” International Journal of Technology 1445: 1454.
- Bubeck, S., J. Tomaschek and U. Fahl. 2016. “Perspectives of electric mobility: Total cost of ownership of electric vehicles in Germany” Transport Policy. 63:77
- Caliskan, H. 2017. “Environmental and Enviroeconomic Researches on Diesel Engines with Diesel and Biodiesel Fuels” Journal of Cleaner Production. 125 :129.
- Cooney, G., T. R. Hawkins and J. Marriott. 2013. “Life Cycle Assessment of Diesel and Electric Public Transportation Buses” Journal of Industrial Ecology. 689:699.
- DeCorla-Souza, P., J. Everett, B. Gardener and M. Culp. 1997. “Total Cost Analysis: An Alternative to Benefit-Cost Analysis in Evaluating Transportation Alternatives” Transportation. 107 :123.
- Delhi Metro Rail Corporation Ltd. 2014. “Detailed Project Report for Ahmedabad Metro Rail Project Phase I.” Accessed 15 July 2018. http://www.gujaratmetrorail.com/wp-content/uploads/2016/07/Ahmedabad-Metro-DPR-2014.pdf
- Đurišić-Mladenović, N., F. Kiss, B. Skrbic, M. Tomic, R. Micic, and Z. Predojevic. 2018. “Current State of the Biodiesel Production and the Indigenous Feedstock Potential in Serbia.” Renewable and Sustainable Energy Reviews 280: 291.
- Drabik, D., H. De Gorter, G. R. Timilsina 2014. “The Effect of Biodiesel Policies on World Biodiesel and Oil seed Prices” Energy Economics. 80 :88.
- Dwivedi, G., S. Jain, and M. Sharma. 2011. “Pongamia as a Source of Biodiesel in India.” Smart Grid and Renewable Energy 184: 189.
- Florio, M., and E. Sirtori. 2016. “Social Benefits and Costs of Large Scale Research Infrastructures.” Technological Forecasting and Social Change 65: 78.
- Gebremariam, S. N., and J. M. Marchetti. 2018. “Economics of Biodiesel Production: Review.” Energy Conversion and Management 74: 84.
- Gracia, A., J. Barreiro-Hurle, and L. P. Perez. 2018. “Overcoming the Barriers for Biodiesel Use in Spain: An Analysis of the Role of Convenience and Price.” Journal of Cleaner Production : 391:401.
- Heidrich, O., G. A. Hill, M. Neaimeh, Y. Huebner, P. T. Blythe and R. J. Dawson. 2017. “How do cities support electric vehicles and what difference does it make” Technological Forecasting and Social Change. 17 :23.
- Helmers, E., J. Dietz, and S. Hartard. 2017. “Electric Car Life Cycle Assessment Based on Real-world Mileage and the Electric Conversion Scenario.” International Journal of Life Cycle Assessment 15: 30.
- Hickman, R., and M. Dean. 2017. “Incomplete Cost – Incomplete Benefit Analysis in Transport Appraisal.” Transport Reviews. doi:10.1080/01441647.2017.1407377.
- Hombach, L. E. , C. Busing and G. Walther. 2018. “Robust and Sustainable Supply Chains Under Market Uncertainties and Different Risk Attitudes-A Case Study of the German Biodiesel Market” European Journal of Operational Research 302:312
- Husin, A. E., M. A. Berawi, S. Dikun, T. Ilyas, and A. R. B. Berawi. 2015. “Forecasting Demand on Mega Infrastructure Projects: Increasing Financial Feasibility.” International Journal of Technology 73: 83.
- Jochem, P., C. Doll and W. Fichtner. 2016. “External Costs of Electric Vehicles” Transportation Research Part D: Transport and Environment. 60 :76.
- Johansson, E., L. Hiselius, T. Koglin and A. Wretstrand. 2017. Evaluation of public transport: regional policies and planning practices in Sweden Urban Planning and Transport Research. 59:77
- Karaaslan, E., Y. Zhao, and O. Tatari. 2018. “Comparative Life Cycle Assessment of Sport Utility Vehicles with Different Fuel Options.” International Journal of Life Cycle Assessment 333: 347.
- Lele, S. 2019. “Biodiesel in India.” Accessed 01 July 2019. http://www.svlele.com/biodiesel_in_india.htm
- Mahadevia, D. (2013). “Inclusive Transport.” Accessed 03 July 2018. http://www.urbanmobilityindia.in/Upload/Conference/3aa6127f-2dd6-43a0-5be5a50ea32e.pdf
- Martens, K., and D. Floridea. 2017. “Travel Time Savings, Accessibility Gains and Equity Effects in Cost–benefit Analysis.” Transport Reviews 152: 169.
- Matthews, B. H. S., C. Hendrickson, and A. Horvath. 2001. “External Costs of Air Emissions from Transportation.” Journal of Infrastructure Systems 13: 17.
- Miller, P., A. G. De Barros, L. Kattan, and S. C. Wirasinghe. 2016. “Public Transportation and Sustainability: A Review KSCE.” Journal of Civil Engineering 1076: 1083.
- Ministry of Road Transport and Highways (India). 2015. “Road Accidents in India.” Accessed 03 July 2018. http://pibphoto.nic.in/documents/rlink/2016/jun/p20166905.pdf
- Ministry of urban development (India). 2016. “Ahmedabad Traffic Management and Informationcontrolcentreoperationsdocument.” Accessed 03 July 2018. http://www.sutpindia.com/skin/pdf/Ahmedabad_TMICC.pdf
- Mitropoulos, L. K., P. D. Prevedouros, and P. Kopelias. 2017. “Total Cost of Ownership and Externalities of Conventional, Hybrid and Electric Vehicle.” Transportation Research Procedia 267: 274.
- Moreno-Pérez, O. M., G. P. C. Marcossi, and D. Ortiz-Miranda. 2017. “Taking Stock of the Evolution of the Biodiesel Industry in Brazil: Business Concentration and Structural Traits.” Energy Policy 525: 533.
- Moro, A., and E. Helmers. 2017. “A New Hybrid Method for Reducing the Gap between WTW and LCA in the Carbon Footprint Assessment of Electric Vehicles.” International Journal of Life Cycle Assessment 4: 14.
- Mouter, N., J. A. Annema, and B. Van Wee. 2015. “Managing the Insolvable Limitations of Cost-benefit Analysis.” Results of an Interview Based Study, Transportation 277: 302.
- Murty, M., K. Dhavala, M. Ghosh, and R. Singh 2006. “Social Cost-Benefit Analysis of Delhi Metro.” Accessed 03 July 2018. http://www.iegindia.org/upload/publication/Workpap/wp273.pdf
- Naylor, R. L., and M. M. Higgins. 2017. “The Political Economy of Biodiesel in an Era of Low Oil Prices.” Renewable and Sustainable Energy Reviews 695: 705.
- Nordelöf, A., M. Messagie, A-M.. Tillman, M. Ljunggren Söderman and J. Van Mierlo. 2014. “Environmental Impacts of Hybrid, Plug-in Hybrid and Battery Electric Vehicles- What can we learn from Life Cycle Assessment?” The International Journal of Life Cycle Assessment. 1866 :1890. 11.
- Pucher, J. and A. Markstedt. 1983. Consequences of public ownership and subsidies for mass transit: Evidence from case studies and regression analysis Transportation. 323 :345.
- Sharma, Y. C., and V. Singh. 2017. “Microalgal Biodiesel: A Possible Solution for India’s Energy Security.” Renewable and Sustainable Energy Reviews 72: 88.
- Steubing, B., C. Mutel, F. Suter, and S. Hellweg. 2016. “Streamlining Scenario Analysis and Optimization of Key Choices in Value Chains Using a Modular LCA Approach.” International Journal of Life Cycle Assessment 510: 522.
- Sun, Y., and Y. Cui. 2018a. “Analysing Urban Infrastructure Economic Benefits Using an Integrated Approach.” Cities 124: 133.
- Sun, Y., and Y. Cui. 2018b. “Evaluating the Coordinated Development of Economic, Social and Environmental Benefits of Urban Public Transportation Infrastructure: Case Study of Four Chinese Autonomous Municipalities.” Transport Policy 116: 126.
- Viton, P. A. 1993. “How Big Should Transit Be? Evidence on the Benefits of Reorganization from the San Francisco Bay Area” Transportation. 35 :57.
- White, P. R., R. P. Turner and T. C. Mbara. 1992. “Cost Benefit Analysis of Urban Minibus Operations” Transportation. 59: 74
- Xu, Y. J., G. X. Li, and Z. Y. Sun. 2016. “Development of Biodiesel Industry in China: Upon the Terms of Production and Consumption.” Renewable and Sustainable Energy Reviews 318: 330.
Appendix 1.
Sample Calculations for Social benefit cost ratio (SBCR) and Life cycle costs (LCC)
Sample calculations for SBCR
Ridership revenue (RR) + Advertisement revenue (AR) = 511 million INR;
Discount rate, i = 12%;
Life cycle, n = 25 years;
Therefore, present worth of revenue benefits, PWRB = 4007.82 million INR.
Cost of corridor development, CDC = 31,042.55 million INR;
Cost of biodiesel plant, CBP = 1.9 million INR;
Cost of infrastructure maintenance, CIM = 384 million INR;
Cost of system operation and maintenance, CSOM = 729.5 + 790.6 = 1520 million INR;
Cost of biodiesel plant operation and maintenance, CBPOM = 119 million INR;
Cost of ITMS infrastructure replacement, CITMS = 1707 million INR;
Recurring period of replacement of ITMS infrastructure, Litms = 7 years;
Cost of replacement of buses, CBR = 2471 million INR;
Recurring period of replacement of buses, Lb = 7 years;
Therefore, present worth of costs, PWC = 50,043.23 million INR.
Therefore, financial benefit to cost ratio, FBCR = 0.08.
Therefore, financial gap, FG = 50,043.23 – 4007.82 = 46,035.41 million INR.
Vehicle operation cost saving, VOS = 766 million INR;
Fuel savings, FS = 2298 + 152 = 2450 million INR;
Therefore, present worth of vehicle operation and fuel savings benefit,
PWVSB = 19,215.6 million INR;
Environmental benefits, EB = 153.38 million INR;
Therefore, present worth of environmental benefits, PWEB = 1202.97 million INR;
Social benefits (accident reduction and travel time saving) = 600.22 + 288 = 888.22 million INR.
Therefore, present worth of social benefits = 6966.4 million INR.
Therefore, social benefit cost ratio, SBCR = 0.63.
Sample calculations for LCC
The life cycle cost is computed as per following relationship,
Present worth of capital costs (include cost of biodiesel plant installation and cost of bus procurement) = 2473 million INR.
Present worth of annual costs (include cost of biodiesel plant operation and maintenance, cost of infrastructure maintenance, cost of transit system maintenance and cost of transit system operation)= 15,867 million INR.
Present worth of one-time costs (include cost of replacement of ITMS infrastructure and cost of replacement of buses) = 3131 million INR.
Present worth of financial costs (include cost of interest on capital and annual financial charges) = 4745 million INR.
Life cycle cost = 2473 + 15,867 +3131 + 4745 = 26,217 million INR