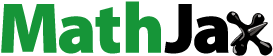
ABSTRACT
The potential reuse of different waste metallic particulates (aluminium, iron fillings and copper) from industrial operations in the development of conductive plastic composites was considered in this study. Polystyrene based resin was obtained from waste polystyrene by solvolysis and used for developing the composite. The density, voidage fraction, electrical conductivity and microstructure of the composites were evaluated. The conductivity and the density of composites increased with filler concentration with the aluminium based composites being the most conductive. The conductive ranges of composites with aluminium, iron fillings and copper were 7.6 × 10−8 to 7.3 × 10−7, 2.1 × 10−7 to 6.0 × 10−7 and 4.6 × 10−8 to 2.5 × 10−7 S/cm respectively which suggested that they can all be used as electrostatic dissipative materials. Microstructural analysis revealed that the particles were more homogeneously dispersed in the aluminium-polystyrene composite than the others. The conductive composite produced possess moderate electrical properties suitable for various industrial and electronic applications.
1. Introduction
A major technology in solid waste management is the development of composite materials for industrial applications. Composite refers to materials that typically consist of two or more components with significantly different physical and/or chemical properties (Adeniyi et al. Citation2019). In commercial production, low-cost metal particulate fillers are sought and mixed with plastics primarily to achieve lower processing cost and improved moulding characteristics (Adeniyi, Ighalo, and Onifade Citation2019). It is not just the Physico-chemical properties of the two components or the volume fraction of the filler which governs the properties and behaviour of the eventual composites, but shape, size, orientation and the extent of adhesion between the matrix and the filler (Fu et al. Citation2008).
Research has been carried out with metals in thermosets like epoxy but similar reports on thermoplastics like polystyrene are rather scarce (Ighalo and Adeniyi Citation2020). Adedayo and Onitiri (Citation2012) considered the reuse of waste material derived from the beneficiation of iron ore by reinforcing epoxy with iron ore tailings to produce a conductive composite. They observed that 30% volume content of 300 µm iron ore tailings gave the maximum Young’s modulus; 4.83% greater than that for pure epoxy. Osman and Mariatti (Citation2006) evaluated the mechanical and microstructural properties of aluminium filled epoxy composites. They observed a steep decrease in electrical resistivity and a pronounced decrease in tensile strength and elongation at break with an increase in the metal filler. Furthermore, thermal characterisation revealed that the addition of aluminium reduced the crystallinity of the polypropylene and slightly retarded its thermal degradation. Azghan and Eslami-Farsani (Citation2018) studied the effect of stacking sequence and thermal cycling on the mechanical properties of aluminium-epoxy-basalt/glass fibres-ternary composites. It was observed that flexural modulus was maximum for composites with basalt fibres while it was minimum for composites with glass fibres.
For polystyrene conductive polymers, Abdulkareem, Amosa, and Adeniyi (Citation2018) examined the crystallographic properties of polystyrene-aluminium composites besides other mechanical properties. The study found out that there are no chemical reactions between the metal particles and the polymer matrix during the curing process. The structural and other mechanical properties were also noticeably enhanced. Abdulkareem et al. (Citation2019) investigated hardness of the composites when aluminium and iron fillers are used. They observed that smaller metal particle sizes and greater filler loading led to an improvement in hardness while the thickness of the composite thickness had no significant effect on the hardness of the composites obtained. Aluminium-polystyrene composite was observed to be harder than aluminium-iron composite with the optimal value of the hardness number being 43.7 and 11 respectively. Abdulkareem and Adeniyi (Citation2019) evaluated the electrical properties when copper fillers are used. They observed a maximum conductivity of 2.53 × 10 − 7 S cm−1 with 40 wt% of copper loading.
As a continuation of this line of investigation, this study is aimed at comparatively evaluating the electrical and microstructural properties of conductive composites obtained from polystyrene matrix and metallic fillers (aluminium, iron fillings and copper) from solid waste streams. Furthermore, the density and voidage after curing will be examined which is also unreported in the open literature. The polystyrene is solvated by a novel low cold process initially reported by Abdulkareem and Adeniyi (Citation2017). It is an approach of reducing the large volume of PS waste into a small resinous volume of good adhesive and binding capacity. These waste metal particulates from the solid waste streams were used as fillers and PBR from recycled PS was employed as the matrix to produce conductive plastic composites of varying particulates contents at room temperature.
2. Methodology
2.1. Preparation of metallic particulates
Three different metallic particulates from different solid waste sources (shown in ) were obtained and dried in an oven for 18 h at 40°C to remove any free water present. The dried sample was graded to obtain the powder of 150 µm in size.
Table 1. Description of metallic particulates
2.2. Preparation of polystyrene based resin (PBR)
Polystyrene was sourced from the electronic waste dumpsite in the university community. It is usually used within packing for electronic gadgets to prevent damage from a collision in transit. The polystyrene-based resin (PBR) was produced according to the process described by S A Abdulkareem and Adeniyi (Citation2017) and possess a density of 855 kg/m3. It hardens on exposure to air at room temperature within 48 h curing time.
2.3. Preparation of conductive composites
Each metallic particulate type of 150 µm in size was mixed with solvated PBR in a mixer by simple mechanical stirring in the percentage projected in by weight. The mixing in each combination was done manually in 5 minutes to achieve uniform composition at all points. The obtained mixture was further pressed using a single roller on the metallic plate to eliminate the possible voidage and to achieve a uniform thickness of 3 mm. The conductive composite produced were then left to cure under ambient conditions (25 ± 2°C) for 7 days. Three types of conductive composites were produced in this sequence. The microstructural of the developed composites was observed at magnifications of 40× for each sample using an Olympus BX Metallurgical microscope.
Table 2. Composition of the conductive plastic composites
2.4. Measurement of electrical conductivity
The electrical conductivity of conductive composites was obtained by measuring the resistances using the standard four-point probe method (the conductivity is obtained as the inverse of resistivity). The apparatus used was Micro-ohmmeter (Model 580, Keithley Instruments, Inc., Cleveland, Ohio). Resistivity was obtained from the bulk resistance of the composite specimen. The narrow sides of the film were covered with aluminium foils to facilitate measurement with the four-point probe. The effect of different metallic fillers content in the PBR matrix on the resistivity of the conductive composites was observed. The film resistivity (R) was calculated by using the relation in EquationEquation 1(1)
(1) (Bhattacharya Citation1996).
where R (ohm) is the measured resistance, L (cm) is the length of the specimen, which is also the length of the glass slide, w (cm) is the width of the specimen, which corresponds to the gap between the two adhesive strips, and h (cm) is the thickness of the conductive paste. The product of width and thickness is the Area, A (cm). Four specimens were tested for each experimental condition. An average bulk resistivity for each sample was calculated for each sample. The conductivities were obtained as the simple inverse of resistivity.
2.5. Measurement of density
The experimental densities of all the metallic particles, PBR and respective composites were obtained using the laboratory-made density setup. The rule of mixture was employed to calculate the theoretical densities of the solvated polystyrene/metallic composites by using the densities of the solvated polystyrene and each metallic particulate. Theoretical densities of the composites were evaluated from EquationEquation 2(2)
(2) (Utracki Citation2002).
where and
are weight fraction of the metallic filler and PBR matrix respectively while
and
are densities of the metallic fillers and matrix respectively. The porosity in the composites was estimated by relating the theoretical and experimental densities to EquationEquation 3
(3)
(3) (Utracki Citation2002).
where is the theoretical density of the conductive plastic composites and
is the experimental density of the conductive plastic composites.
3. Results and discussions
3.1 Effect of metallic particulates on the bulk density of the composites
represents a comparison of the effects of the percentage of filler on the densities of the conductive composites. From the figure, it can be observed that as the concentration of filler in the matrix increases, the density of the developed composites increased for metal types. This is in agreement with the observations of a previous study on metal-plastic composites (Pargi et al. Citation2015). Furthermore, this effect is due to the higher densities of the metals in comparison to the polystyrene polymer. Any increase in the amount of the denser metals in the polymer matrix will invariably increase the overall density of the composite. It is equally evident from that the density of the metal type used for the composite affects the overall density of the composite. It can be observed as the iron-based conductive composites had the highest values of densities in all the combinations under study, followed by copper-based plastic composited and the lightest plastic composites produced is aluminium based. This is in agreement with the law of composite mixing (Al-Ramadhan Citation2008; Utracki Citation2002). A composite is a non-homogenous material with multiple phases. The combination of factors such as micro-mixing, filler-resin interfacial adhesion and filler-resin dispersion as well as the avoidance of voidage in the final composite structure has a greater effect on the bulk density of the composites compared to a factor like the inherent density of the fillers themselves. This is why the trend of the composite bulk density does not follow that of the density of the metallic fillers.
3.2. Effect of metallic particulates on the porosity of the composites
The effectiveness of mixing and the extent of interfacial adhesion in each of the conductive composite type is presented in terms of measurable porosity by comparing the experimental and theoretical densities. This is presented in . It can be generally observed that the porosity decreases as the percentage of metallic fillers increases in the PBR matrixes. Furthermore, the porosity was least in the aluminium composites than for copper and iron composites. This holds for all domain of filler concentration. This suggests that aluminium has the best affinity and interfacial adhesion to the polystyrene resin. This observation is in agreement with the findings from the microstructural analysis discussed in Sub-section 3.4.
3.3. Effects of metallic particulate on the electrical conductivity of the composites
Electrical conductivities of the different conductive polystyrene composites were determined through a four-point probe. shows the comparative electrical conduction behaviour of these conductive plastic composites with different metallic filler loadings (0–40%). The conductive ranges of composites with aluminium, iron fillings and copper were 7.6 × 10−8 to 7.3 × 10−7, 2.1 × 10−7 to 6.0 × 10−7 and 4.6 × 10−8 to 2.5 × 10−7 S/cm respectively. This suggests that they can all be used as electrostatic dissipative materials as previously confirmed in the works of Pargi et al. (Citation2015), Osman and Mariatti (Citation2006) and Gelinas, Chagnon, and Pelletier (Citation1999) for electrostatic dissipative composite. Aluminium based composites showed the highest conductivity in all percentage of fillers except at 10% whilst copper-based composites had the lowest conductivity (though still within the range of the projected application). The good conductivity of aluminium based conductive polymers can also be deduced to result from a better mixing and dispersion of the aluminium particles in the polystyrene matrix as observed from the microstructural analysis. The formation of a stable conductive network within the composites ensures a better flow of electric current through the material as the movement of electrons is permitted (Abdulkareem and Adeniyi Citation2019). The electrical conductivity of the composites does not show a trend with the inherent electrical conductivity of the metallic fillers. This is because the electrical conductivity of the composites depends on how well a conductive path is created (by a particle-to-particle contact) within the polymer resin. This is controlled by the extent of dispersion of the metal in the composite. It is however not surprising that the aluminium had the highest electrical conductivity as it was subsequently observed to have the best dispersion (from Section 3.4).
3.4. Micrograph of the metal-polystyrene composites
The optical reflection micrograph of the conductive polymers at 30% and 40x magnification are shown in . The particles appear homogeneously distributed at each metal-polystyrene composite type. However, there is evidence that the particles were more homogeneously dispersed in the aluminium-polystyrene composite than other which is a confirmation of its highest value of electrical conductivities. The continuous conducting paths in other metal-polystyrene composites are equally evident but at lower intensities. This also explains the effectiveness of the method employed in this product development approach.
4. Conclusion
The usage of different recycled metallic particles in the production of conductive composite has been successfully employed and the composites tested for the electrical properties, density and void fraction as a function of recycled metallic fillers. From the result obtained, it was observed that the electrical conductivity and density of composites increased with more addition of different metallic fillers with the aluminium based composites being the most conductive. The conductive ranges of composites with aluminium, iron fillings and copper were 7.6 × 10−8 to 7.3 × 10−7, 2.1 × 10−7 to 6.0 × 10−7 and 4.6 × 10−8 to 2.5 × 10−7 S/cm respectively which suggested that they can all be used as electrostatic dissipative materials. There was evidence from the optical micrographs that the particles were more homogeneously dispersed in the aluminium-polystyrene composite than other which is a confirmation of its highest value of electrical conductivities. This study has successfully utilised metallic fillers (aluminium, iron fillings and copper) and polystyrene from solid waste streams for the development of conductive polymers. The conductive composite produced possess moderate electrical properties suitable for various industrial and electronic applications.
Disclosure statement
No potential conflict of interest was reported by the authors.
Additional information
Notes on contributors
Adewale G. Adeniyi
Adewale G. Adeniyi is a senior lecturer in the Department of Chemical Engineering, University of Ilorin, Nigeria. He holds a PhD in Chemical Engineering from Ladoke Akintola University of Technology, Ogbomoso, Nigeria. He is interested in process and product development with a focus on computer-aided modelling and the optimisation of chemical process systems, biofuel production, and solid waste recycling.
Joshua O. Ighalo
Joshua O. Ighalo obtained his Bachelors’ Degree in Chemical Engineering in 2015 from the University of Benin, Nigeria. He also obtained a Masters’ Degree in Chemical Engineering in 2020 from the University of Ilorin, Nigeria. His research interests include computer-aided modelling and optimisation of chemical process systems, biofuel production, solid waste management, and environmental pollution control.
Sulyman A. Abdulkareem
Sulyman A. Abdulkareem is a professor of chemical engineering and the Current Vice-Chancellor of the University of Ilorin in Nigeria. Abdulkareem graduated from University of Detroit in the US in 1980, with BChE and MChE degrees in chemical engineering. In 1988 he was awarded a Ph.D. by the University of Louisville in the US. His areas of research interests are heterogeneous catalysis, reaction engineering and product development.
References
- Abdulkareem, S. A., and A. Adeniyi. 2017. “Production Of Particle Boards Using Polystyrene And Bamboo Wastes.” Nigerian Journal of Technology 36 (3): 788–793.
- Abdulkareem, S. A., and A. Adeniyi. 2019. “Recycling Copper and Polystyrene from Solid Waste Stream in Developing Conductive Composites.” The Journal of Solid Waste Technology and Management 45 (1): 39–44. doi:https://doi.org/10.5276/JSWTM.2019.39.
- Abdulkareem, S. A., M. K. Amosa, and A. Adeniyi. 2018. “Synthesis and Structural Analysis of Aluminium-filled Polystyrene Composites from Recycled Wastes.” Environmental Research, Engineering and Management 74 (2): 58–66.
- Abdulkareem, S. A., M. K. Amosa, A. G. Adeniyi, M. M. Magaji, and R. A. Ajibola. 2019. “Effect of Metallic Fillers on the Hardness of Polystyrene Composites: An Experimental Investigation.” Paper presented at the 1st International Conference on Sustainable Infrastructural Development, Convenant university, Nigeria.
- Adedayo, S. M., and M. A. Onitiri. 2012. “Tensile Properties of Iron Ore Tailings Filled Epoxy Composites.” The West Indian Journal of Engineering 35 (1): 51–59.
- Adeniyi, A. G., J. O. Ighalo, and D. V. Onifade. 2019. “Banana and Plantain Fiber Reinforced Polymer Composites.” Journal of Polymer Engineering. 39 (7): 597–611. doi:https://doi.org/10.1515/polyeng-2019-0085.
- Adeniyi, A. G., D. V. Onifade, J. O. Ighalo, and A. S. Adeoye. 2019. “A Review of Coir Fiber Reinforced Polymer Composites.” Composites Part B: Engineering 176: 107305. doi:https://doi.org/10.1016/j.compositesb.2019.107305.
- Al-Ramadhan, Z. 2008. “Effect of Nickel Salt on Electrical Properties of Polymethylmethacrylate.” Journal of College of Education, Al-Mustansiriyah University 3: 1-7.
- Azghan, M. A., and R. Eslami-Farsani. 2018. “The Effects of Stacking Sequence and Thermal Cycling on the Flexural Properties of Laminate Composites of Aluminium-epoxy/basalt-glass Fibres.” Materials Research Express 5 (2): 025302.
- Bhattacharya, S. K. 1996. Metal Filled Polymers, (Properties and Applications). New York: Markel Dekker.
- Fu, S. Y., X. Q. Feng, B. Lauke, and Y. W. Mai. 2008. “Effects of Particle Size, Particle/matrix Interface Adhesion and Particle Loading on Mechanical Properties of Particulate-polymer Composites.” Composites Part B: Engineering 39 (6): 933–961. doi:https://doi.org/10.1016/j.compositesb.2008.01.002.
- Gelinas, C., F. Chagnon, and S. Pelletier. 1999. Development of an Iron-Resin composite material for soft magnetic applications. Advances in Powder Metallurgy and Particulate Materials.,6: 20-33.
- Ighalo, J. O., and A. G. Adeniyi. 2020. “Utilization of Recycled Polystyrene and Aluminum Wastes in the Development of Conductive Plastic Composites: Evaluation of Electrical Properties.” In Handbook of Environmental Materials Management, edited by C. M. Hussain, 1–9. Switzerland: Springer Nature.
- Osman, A. F., and M. Mariatti. 2006. “Properties of Aluminum Filled Polypropylene Composites.” Polymers & Polymer Composites 14 (6): 623–633.
- Pargi, M. N. F., P. L. Teh, S. Hussiensyah, C. K. Yeoh, and S. A. Ghani. 2015. “Recycled-copper-filled Epoxy Composites: The Effect of Mixed Particle Size.” International Journal of Mechanical and Materials Engineering 10 (1): 3. doi:https://doi.org/10.1186/s40712-015-0030-2.
- Utracki, L. A. 2002. Polymer Blends Handbook. Dordrecht: Springer.