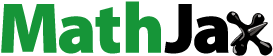
ABSTRACT
The objective of the current research is to study the resistance of cone (prepared from recycled coarse aggregate (RAC) and sand with the fractional substitution of cement by mineral slag) to freezing-thawing cycles. Compressive strength, length change, mass loss, ultrasonic pulse velocity, durability factor, and relative dynamic modulus of concrete mixtures were evaluated. In general, the incorporation of a 25% slag improved the compressive strength of the new concrete and had a favourable effect on them after freezing-thawing cycles. The freezing-thawing resistance was reduced due to the utilisation of recycled aggregates. The results showed a direct relationship between the resistance reduction and the recycled aggregates content. The introduction of a 25% slag was found to improve the frost resistance in addition to improving sustainability.
1. Introduction
The term ‘green concrete’ has gained extensive attention recently due to its impact on environmental sustainability. The amounts of construction wastes are increasing annually and their use as recycled materials can be an appropriate solution to produce greener concrete, save our natural resources and reduce the solid waste problem. Recycled aggregate obtained after demolishing and crushing concrete structures after the end of their service lives, has currently obtained increasing interest all over the world, for its possible utilisation as an environmentally-friendly building material (Ann et al. Citation2008). Many studies were conducted to investigate the properties and performance of RAC (Haque et al. Citation2014; Pečur, Štirmer, and Milovanović Citation2014; Al-Sanad and El-Hawary Citation2010; El-Hawary and Al-Otaibi Citation2010; Pedro, De Brito, and Evangelista Citation2015; Silva, De Brito, and Dhir Citation2015; Al-Otaibi, El-Hawary, and Abdul-Jaleel Citation2010). Conducted research also include behaviour of confined recycled aggregate concrete (Munir et al. Citation2020). Those and other studies showed that recycled aggregates may be used as full or partial replacement of conventional aggregates, in the production of good quality concrete.
Supplementary cementing materials (SCM) can be utilised as a fractional substitution for cement (Neville Citation2000). GGBS can be utilised in concrete for many applications (Barnett et al. Citation2006; ACI Committee 233 Citation2003; Bijen Citation1996). Work on the effect of slag on hydration and durability of concrete was performed by Kim (Kim et al. Citation2018). Slag may be also used as partial cement replacement to improve properties of RAC and make it more environmentally friendly (Al-Yaqout et al. Citation2020).
Subjecting concrete to cycles of freezing and thawing has a serious detrimental influence on concrete, especially in cold weather (Medina et. al. Citation2013). Resistance of concrete to cycles of freezing and thawing is considered a good measure of concrete durability. The factors affecting freeze-thaw resistance include freeze-thaw cycle time, temperature, surface conditions of concrete, and rate of freezing (Xiao, Lu, and Ying Citation2013). Many researchers investigated the freeze and thaw resistance of concrete containing recycled aggregates. Liu et al. (Citation2016) examined the freezing-thawing resistance of recycled aggregate concrete. This type of concrete with RA exhibited better freeze-thaw resistance. In contrast, the non-air entrained concrete with RA showed low frost resistance. Richardson, Coventry, and Bacon (Citation2011) examined the freeze-thaw test of RAC and NAC using polypropylene fibres and air entrainment to provide durability. Results showed that using additives combined with RA enhances the freezing-thawing resistance compared with normal concrete. Bogas, De Brito, and Ramos (Citation2016) searched the impact of utilising high and normal strength concrete of fine RAC on the resistance to freeze-thaw. A slight enhancement was obtained by using air-entraining on the concrete of high-strength. Moreover, it was found that fine RAC harmed freezing-thawing resistance. Concrete mixes containing RAC were tested by Medina et al. (Citation2013) to determine the freeze-thaw resistance of concrete. A direct relationship was found between RA content and freezing-thawing resistance. The influence of recycled aggregate on freezing-thawing resistance of self-compacting concrete (SCC) was investigated (Tuyan, Mardani-Aghabaglou, and Ramyar Citation2014). A direct relationship between the freezing-thawing resistance of SCC and RAC ratio was determined. Zaharieva, Buyle-Bodin, and Wirquin (Citation2004) found that using RAC in structures results in a reduction in frost resistance. This was attributed to the high porosity, high w/c ratio, and low mechanical features of RAC. Gokce et al. (Citation2004) showed that RAC made from non-air entrained had low resistance to freezing-thawing due to the available mortar which affects new mortar. Moreover, it was found that reducing non-air-entrained adhered mortar would reduce the freeze-thaw resistance after a long time. The effects of treatment of recycled aggregates on the mechanical behaviour (Kazmi et al. Citation2019) and on the durability of recycled aggregate concrete (Kazmi et al. Citation2020) were also investigated.
Due to the discrepancies and variations in results, more research is necessary to better comprehend circumstances that influence the resistance of RAC to freeze-thaw cycles. As the properties of recycled aggregates depend on exposure of the parent concrete, the work presented herein was conducted using recycled aggregates produced locally in Kuwait. Special freeze-thaw system was designed and installed with special freeze-thaw cycle durations. This study aims to investigate methods for improving the durability of RAC and reports the effect of adding mineral slag on the freeze resistance for recycled concrete with different proportions of recycled aggregates, and hence, can provide basic data and reference for the application of RAC.
2. Materials
2.1. Natural aggregates
Two types of aggregates were utilised: coarse fraction (Gabbro) of three varied sizes of 3/8, 1/2, and 3/4 inches (9.5, 12.7 and 19 mm), and a fine fraction (sand) having grains < 4 mm. indicates the grading distribution and physical properties of natural aggregates.
Table 1. Characteristics of natural and recycled aggregates
2.2. Recycled aggregates
The aggregate, obtained from a Kuwaiti Recycled Aggregates factory, was procured from demolished concrete and available in Kuwait commercially. illustrates the characteristics of the coarse aggregates. Saturated surface dry aggregates were used. The absorption and specific gravity were determined according to ASTM C 127 and ASTM C 128.
2.3. Cement
Ordinary Portland cement (Type I) was used in all blends. This cement was provided by KPCC. The cement was manufactured according to ASTM C150. The characteristics of the cement are illustrated in .
2.4. Ground Granulated Blast-furnace Slag
GGBS was obtained from the Kuwaiti British Ready-Mix Company (KBRC) as per ASTM C 989 specifications. A ratio of 25% of Portland cement was replaced by this slag. The characteristics of Portland cement and GGBS as received from the manufacturer are given in .
2.5. Batching water
Mixing water quality is of great importance on concrete strength, time of setting, and other properties related to strength such as concrete durability. In this work, regular tap water was used in all mixtures that meet the acceptance criteria of ASTM C 94.
2.6. Chemical admixtures
KUT PLAST SP-400 super-plasticising, high range, a water-reducing agent was utilised in all mixtures. The material fulfils the specifications of ASTM C494 Type F.
2.7. Concrete mixtures
Six different mixtures were utilised to prepare the concrete samples. The w/b ratio (water/binder) was reserved at 0.35 in all samples. The name of the samples was designated to reflect its properties. For example, a concrete mixture I, designated as (RA0%) is the reference mix with 0% recycled aggregate and 100% Ordinary Portland Cement. Recycled coarse aggregates were used in mixes II and III as a replacement of 50% and 100% of conventional coarse aggregate. The subsequent three mixes were prepared similar to the previous mixes, however, 25% GGBS was utilised as cement substitutes. For example, mixture Ⅳ, designated as (RA0% S) is a reference mix with 0% recycled aggregate and 25% slag as a cement substitute. The mixture proportions are illustrated in . The 25% slag ratio was selected as it is in the range usually used in Kuwait. Slag is not produced locally in Kuwait and hence it is more expensive than cement. The use of higher ratios, although used in other countries, will not be feasible. Smaller ratios will not have a noticeable effect on the behaviour of produced concrete. Fine tuning of the ratio may be performed in a coming study.
Table 2. Mix proportions
2.8. Specimens preparation and curing
The test specimens were produced in line with ASTM C192. For each mixture, 3 concrete cylinders (150 mm- diameter and 300 mm- height); for compressive strength test at 28 days, and three 75x75x285 mm prisms for freeze-thaw resistance (following ASTM C666 – Procedure A) was prepared. Ultrasonic pulse velocity (UPV), weight losses, and length changes were measured for the concrete prisms, during freeze-thaw cycles. Samples were covered with wet burlap and polyethylene sheets to decrease water evaporation. The workability of the samples was evaluated following the slump cone test (ASTM C143). All samples were initially cured for one day. The samples were then removed from the moulds and retained in water for curing at 20(±2) º C until the designated test ages.
3. Experiments
3.1. Compressive strength test
The compressive strength was measured utilising concrete cylinders (diameter of 150 mm and a height of 300 mm) (for 28-days following ASTM C39). The shortening is according to ASTM C 469 using a compressometer. The compression load was exercised with the use of a compression machine for a maximum load of 3000 kN (5.4 kN/s).
3.2. Freeze and thaw test
Three specimens (75 mm×75 mm×285 mm) were tested for length changes, weight losses, and relative dynamic modulus of elasticity. Following ASTM C666 – Procedure B, the prism samples were placed in boxes containing water up to 3 mm above the samples as shown in . The freezing-thawing test was initiated by retaining the samples in the water at the start of the thawing period. The duration of each freeze-thaw cycle was 4 hours. Weight, length, and dynamic modulus of elasticity were measured after every completed 30 freeze-thaw cycles. and show the detailed procedure of every freeze-thaw cycle. The test was stopped when the samples had been through 300 cycles or until attaining 60 % of the initial modulus, whatsoever occurs first.
Table 3. Phases of freezing-thawing cycles
3.2.1. Weight loss, length change and durability factor measurements
The weight change during the freeze-thaw test was evaluated by determining the weights of the sample after removing them from the system, in thawed condition, every 30 cycles.
Change of length and fundamental transverse frequency were also measured. Two metal pins were installed on the surface of the samples during casting (keeping a distance 200 mm). The measurement of length was obtained using a Comparator. Changes in length are calculated using EquationEq. (1)(1)
(1) .
The change in weight was calculated using EquationEq. (2)(2)
(2) .
RDME was calculated numerically as follows:
Where the pulse velocities, Vc and Vo were measures using the ultrasonic test as shown in .
DF was calculated by EquationEq. (4)(4)
(4) .
4. Results and discussion
4.1. Workability
Workability was assesses based on the slump results. The slump values of all mixtures investigated are illustrated in . An inverse relationship was noticed between the concrete workability and RCA content. This may be attributed to the increase in water demand which is not easy to foretell. This agrees with the results of previous studies (Qasrawi Citation2014; Park Citation1999; Buck Citation1977; Hansen and Narud Citation1983; Chen, Yen, and Chen Citation2003). Analogous results were noticed with GGBS mixes. The utilisation of slag as a fractional substitution of Portland cement in RCA did not improve concrete workability.
Table 4. Air content and slump values
also illustrates the air content effect for the different mixes. Generally, the air content increased when recycled coarse aggregates were increased. The air proportion was up to double the anticipated value. The rise of air content may be a result of the mortar tied up to the recycled aggregates (Qasrawi Citation2014). It was noticed that mixes including 25% slag as a fractional cement substitution, showed close and similar results of air content as for the original mixes.
4.2. Compressive strength
The compressive strength at the age of 28 days for concrete containing different proportions of recycled aggregates, with and without slag, is shown in . The concrete without slag showed compressive strengths between 35 and 42 MPa, whereas those for concrete samples including slag were between 35 to 54 MPa depending on the proportion of recycled aggregates. A reduction of compressive strength when using RCA was noticed. This reduction has a direct relationship with the ratio of RCA in the mixture. This agrees with many previous investigations (Munir et al. Citation2020). It was observed that the compressive strength of OPC concrete samples (having 100% RA at 28 days) was the lowest, and replacements of 50% and 0 % RA caused corresponding compressive strength increases of 19% and 6%, respectively. The results show that the compressive strength for 50% RA is more than that containing 100% RA by about 11%. Limbachiya, Meddah, and Ouchagour (Citation2012) related these variations in concrete properties to the various properties and characteristics of the RCA that influence the characteristics of concrete. The quantity and properties of old mortar available on the RCA greatly affect the properties and behaviour of the produced concrete ([Limbachiya, Meddah, and Ouchagour Citation2012).
also shows that the utilisation of GGBS as fractional substitution of PC increased the strength of the mixes incorporating RCA. The increase in strengths was found to be 1.41% and 45% for the RA100%S and RA 0%S mixtures, respectively, as compared to the mixtures with identical RA ratios, without GGBS. The strength reduction was found to be below 16% for the RA50% S mixture compared to the RA50% mixture. Due to secondary pozzolanic reactions, better results are expected at higher ages for mixes with slag (Song and Saraswathy Citation2006; Tan and Pu Citation1998; Park, Noh, and Park Citation2005; Aldea Citation2000). The same trend was found by Berndt (Citation2009) and Qasrawi (Citation2014).
Due to the high voids ratio in recycled aggregates, recycled aggregates are usually weaker than normal aggregates which usually results in weaker concrete. However, due to the high water absorption, resulting concrete will be more wet during test which will increase apparent strength. Absorbed water will also cause internal curing to take place and hence increase strength with time (El-Hawary and Alsulily Citation2020). Those two opposite actions may be the reason for the higher strength achieved for the 50% recycled aggregate concrete.
In general, results show that the compressive strength for concrete containing recycled aggregates is analogues and very close to that of conventional concrete. Due to the large variations in the properties of recycled aggregates and the numerous interacting factors affecting the behaviour of recycled aggregates concrete, it is very difficult to accurately predict the compressive strength of recycled aggregates concrete. The effect of introducing GGBS in the mix on the compressive strength is minimal compared to its effect on conventional concrete.
4.3. Freeze and thaw test
The prisms were retained in the curing area till the test date. The accelerated freeze-thaw test was carried out in an automatic environmental cabinet. Each cycle lasted for 4 hours as explained before.
4.3.1. Weight loss
The changes in the weight of concrete specimens are illustrated in . The weight of the concrete specimens was found to increase as the number of freezing-thawing cycles increases, up to 270 cycles; then a slow decrease was observed up to 300 cycles in all samples. This may be explained by water absorption and crystallisation in the early cycles followed by the observed scaling in the latter cycles.
shows the change of weight of recycled aggregates concrete samples after 300 freezing-thawing exposure cycles test. Generally, the weight loss of concrete samples increases as the quantity of recycled aggregates increases when exposed to freeze-thaw cycles. The results showed that after 300 cycles, weight loss of concrete specimens for RA100% mix was less than that for RA50% mix by 22%, while it is more than that for RA0% mix by 67%. The values of the weight loss for all mixes, however, are minimal. The values were not more than 0.15%, 0.32% and 0.25% for RA0%, RA50% and RA100% mixes respectively after 300 cycles. This indicates that the weight loss of RAC may be tolerated.
On the other hand, the weight loss for slag mixes increased as the amount of recycled aggregates increased. When RA reaches 100%, the weight loss is not more than 0.68%, while it is 0.65% for 50% RA and 0.3% for the conventional mix. The weight loss may be accepted for slag mixes after 300 freezing-thawing cycles. The introduction of 25% GGBS was found not to be enough to decrease the weight loss of recycled aggregates concrete specimens.
4.3.2. Relative dynamic modulus of elasticity
The relative dynamic modulus of elasticity values were evaluated as explained before. The effect of applying 300 freeze-thaw cycles on RDME is shown in . The test was terminated when the number of freeze-thaw cycles reaches 300 cycles or when RDME value approach 60 % of its original value, as specified (ASTM C666). The results show that RA0% and RA100% without slag satisfied the frost requirements, 60% RDME, up to 300 cycles of freezing-thawing. RDME value for RA50% mix, however, fell under 60% RDME at 210 cycles. The best performance for frost resistance was observed for RA0%, followed by the performance of RA100% which was slightly lower. According to results, the use of 100% recycled aggregates is preferred over the use of 50% as the resulting concrete achieved higher resistance to freezing and thawing and hence higher durability.
For mixes containing 25% slag, however, RDME of slag mixes RA0%SL and RA50%SL was above 60% up to 300 cycles. The performance of RA0%SL was slightly better than that for RA50%SL in resisting freeze-thaw cycles. As can be seen, these two mixes exhibit a higher frost resistance compared to mixes without slag, containing the same recycled aggregates replacement level. On the other hand, the RA100%SL mix fell below 60% at 120 cycles and shows the lowest performance in regards to RDME. This mix exhibited the lowest frost resistance and highest deterioration due to freeze-thaw cycles among all mixes. The introduction of 25% slag as cement replacement, therefore, improved the freeze-thaw resistance and durability for mixes containing no recycled aggregates or 50% recycled aggregates, while reducing durability and performance for mixes containing 100% recycled aggregates.
4.3.3. Durability factor
shows the Durability Factor (DF) for all mixtures calculated as explained before after the termination of the freezing-thawing cycles test. As explained before, the test was terminated after the samples are subjected to 300 cycles of freezing and thawing or after the RDME approaches 60% of its original value.
Table 5. Durability factor for each mix
For mixes without slag, the highest DF was obtained for both RA0% and RA100%, while the lowest DF was observed for RA50% which did not satisfy the minimum requirements of ASTM C666 (ACI Committee 233 Citation2003) concerning the DF. As for mixes containing slag, however, the durability factor was improved for mixes containing no recycled aggregates and mixes containing 59% recycled aggregates. The DF was reduced for the mix containing 100% recycled aggregates as a result of introducing 25% slag. The results follow the same trend noticed for the RDME evaluation test.
5. Conclusions
The results of the current research lead to the following conclusions:
Compressive strengths of RAC (after 28 days) are less than that of the control samples and maybe improved using 25% GGBS.
The weight loss of normal concrete increases as the amount of recycled aggregates increases after freezing-thawing cycles.
An inverse relation was determined between weight loss of slag mixes and the amount of recycled aggregates. It is obvious that using 25% GGBS is not enough to reduce the weight loss of recycled aggregates concrete samples.
Using low or high amounts of recycled aggregates produces concrete that has a higher resistance to freezing-thawing cycles in comparison to the medium amount of recycled aggregate. To produce higher resistance concrete, 50% of recycled aggregates is not advisable.
The concrete may exhibit lower frost resistance and increased deterioration due to freeze-thaw cycles when using 25% GGBS combined with 100% RA and is expected to exhibit higher durability and better frost resistance when combined with 0 and 50% RA.
For normal mixes, the highest DF was obtained for both RA0% and RA100%, while the lowest DF was noticed for RA50% which does not satisfy the requirements of ASTM C 666.
An inverse relationship was found between the durability factor for concrete mixes that contain slag and the amount of recycled aggregates.
List of symbols
Acknowledgments
This work received financial support from Kuwait University (Grant RE01/14). Kuwait University is gratefully appreciated. The authors would also like to show their gratitude to the College of Science.
Disclosure statement
No potential conflict of interest was reported by the authors.
Additional information
Funding
Notes on contributors
Moetaz El-Hawary
Moetaz El-Hawary is an Associate Professor at Kuwait University. He is a member in many ACI committees. He is the past president and current vice president for ACI-Kuwait Chapter. He has over 180 publications varying between journal papers, conferences proceedings and technical reports. He received his BS from King Saud University, Saudi Arabia and his MS and PhD from the University of California, Davis. His research interests include Concrete Technology and Concrete Sustainability.
Anwar Al-Yaqout
Anwar Al-Yaqout is an Associate Professor at Kuwait University, Kuwait. He received his BS from the University of Miami, Coral Gables, FL, and his MS and PhD from the University of Florida, Gainesville, FL. His research interests include geotechnical, environmental, waste management and recycling of construction materials.
Khallad Elsayed
Khallad Elsayed is a Research Assistant at Kuwait University, where he received his bachelor's degree in Civil Engineering in 2013 and his MS in 2016. His research interests include concrete materials.
References
- ACI Committee 233. 2003. Slag Cement in Concrete and Mortar, ACI 233R-03. Mich: American Concrete Institute, Farmington Hills.
- Aldea, C. M., F. Young, K. Wang, and S. P. Shah. 2000. “Effects of Curing Conditions on Properties of Concrete Using Slag Replacement.” Cement and Concrete Research 30 (3): 465–472. doi:https://doi.org/10.1016/S0008-8846(00)00200-3.
- Al-Otaibi, S., M. El-Hawary, and A. Abdul-Jaleel. 2010. “Recycling Crushed Concrete Fines to Produce Lime–silica Bricks.” Proceedings of the Institution of Civil Engineers-Waste and Resource Management 163 (3): 123–127. doi:https://doi.org/10.1680/warm.2010.163.3.123.
- Al-Sanad, S., and M. El-Hawary. 2010. “Recycling and Waste Management of Building Rubbles in Kuwait.” 25th International Conference on Solid Waste Technology and Management, Windsor University, Philadelphia, PA.
- Al-Yaqout, A., M. El-Hawary, K. Nouh, and P. Khan. 2020. “Corrosion Resistance of Reinforced Recycled Aggregate Concrete Incorporating Mineral Slag.” ACI Materials Journal 117 (3): 111–122.
- Ann, K. Y., H. Y. Moon, Y. B. Kim, and J. Ryou. 2008. “Durability of Recycled Aggregate Concrete Using Pozzolanic Materials.” Waste Management 28 (6): 993–999. doi:https://doi.org/10.1016/j.wasman.2007.03.003.
- Barnett, S. J., M. N. Soutsos, S. G. Millard, and J. H. Bungey. 2006. “Strength Development of Mortars Containing Ground Granulated Blast-furnace Slag: Effect of Curing Temperature and Determination of Apparent Activation Energies.” Cement and Concrete Research 36 (3): 434–440. doi:https://doi.org/10.1016/j.cemconres.2005.11.002.
- Berndt, M. L. 2009. “Properties of Sustainable Concrete Containing Fly Ash, Slag and Recycled Concrete Aggregate.” Construction and Building Materials 23 (7): 2606–2613. doi:https://doi.org/10.1016/j.conbuildmat.2009.02.011.
- Bijen, J. 1996. Blast Furnace Slag Cement for Durable Marine Structures. Netherlands: Stichting Beton Prisma.
- Bogas, J. A., J. De Brito, and D. Ramos. 2016. “Freeze–thaw Resistance of Concrete Produced with Fine Recycled Concrete Aggregates.” Journal of Cleaner Production 115: 294–306. doi:https://doi.org/10.1016/j.jclepro.2015.12.065.
- Buck, A. D. 1977. “Recycled Aggregate as a Source of Aggregate.” ACI Journal 74: 212–219.
- Chen, H., T. Yen, and K. Chen. 2003. “The Use of Building Rubbles in Concrete and Mortar.” Journal of the Chinese Institute of Engineers 26 (2): 227–236. doi:https://doi.org/10.1080/02533839.2003.9670773.
- El-Hawary, M., and S. Al-Otaibi. 2010. “Durability Assessment of Concrete Containing Recycled Aggregates.” The International Conference on Future Concrete, Doha, Qatar, 10.
- El-Hawary, M., and A. Alsulily. 2020. “Internal Curing of Recycled Aggregates Concrete.” Journal of Cleaner Production, Vol. 275.
- Gokce, A., S. Nagataki, T. Saeki, and M. Hisada. 2004. “Freezing and Thawing Resistance of Air-entrained Concrete Incorporating Recycled Coarse Aggregate: The Role of Air Content in Demolished Concrete.” Cement and Concrete Research 34 (5): 799–806. doi:https://doi.org/10.1016/j.cemconres.2003.09.014.
- Hansen, T. C., and H. Narud. 1983. “Strength of Recycled Concrete Made from Crushed Concrete Coarse Aggregate.” Concrete International 5 :79–83.
- Haque, M., A. Al-Yaqout, V. Sreekala, and M. El-Hawary. 2014. “Characteristics of Recycled Aggregate Concretes Produced in Kuwait: A Case Study.” Journal of Engineering Research 2 (4): 15–31. doi:https://doi.org/10.7603/s40632-014-0022-z.
- Kazmi, S., M. Munir, Y. Wu, I. Patnaikuni, Y. Zhou, and F. Xing. 2019. “Influence of Different Treatment Methods on the Mechanical Behaviour of Recycled Aggregate Concrete: A Comparative Study.” Cement and Concrete Composites, Vol. 104.
- Kazmi, S., M. Munir, Y. Wu, I. Patnaikuni, Y. Zhou, and F. Xing. 2020. “Effect of Recycled Aggregate Treatment Techniques on the Durability of Concrete: A Comparative Evaluation.” Construction and Building Materials, Vol. 264.
- Kim, Y., A. Hanif, M. Usman, M. Munir, S. Kazmi, and S. Kim. 2018. “Slag Waste Incorporation in High Early Strength Concrete as Cement Replacement: Environmental Impact and Influence on Hydration and Durability Attributes.” Journal of Cleaner Production, Vol. 172.
- Limbachiya, M., M. S. Meddah, and Y. Ouchagour. 2012. “Use of Recycled Concrete Aggregate in Fly-ash Concrete.” Construction and Building Materials 27 (1): 439–449.
- Liu, K., J. Yan, Q. Hu, Y. Sun, and C. Zou. 2016. “Effects of Parent Concrete and Mixing Method on the Resistance to Freezing and Thawing of Air-entrained Recycled Aggregate Concrete.” Construction and Building Materials 106: 264–273. doi:https://doi.org/10.1016/j.conbuildmat.2015.12.074.
- Medina, C., M. I. S. de Rojas, and M. Frías. 2013. “Freeze-thaw Durability of Recycled Concrete Containing CeramicAggregate.”JournalofCleanerProduction40:151–160. doi:https://doi.org/10.1016/j.jclepro.2012.08.042.
- Munir, M., S. Kazmi, Y. Wu, I. Patnaikuni, Y. Zhou, and F. Xing. 2020. “Stress Strain Performance of Steel Spiral Confined Recycled Aggregate Concrete.” Cement and Concrete Composites, Vol. 130.
- Neville, A. M. 2000. Properties of Concrete. 4th ed. Longman, England.
- Park, C. K., M. H. Noh, and T. H. Park. 2005. “Rheological Properties of Cementitious Materials Containing Mineral Admixtures.” Cement and Concrete Research 35 (5): 842–849. doi:https://doi.org/10.1016/j.cemconres.2004.11.002.
- Park, S. G. 1999. “Recycled Concrete Construction Rubble as Aggregate for New Concrete.” Study Report No 86 (BRANZ): 16.
- Pečur, I. B., N. Štirmer, and B. Milovanović. 2014. “Durability Properties of Recycled Aggregate Concrete.” In RILEM International workshop on performance-based specification and control of concrete durability, Zagreb, Croatia.
- Pedro, D., J. De Brito, and L. Evangelista. 2015. “Performance of Concrete Made with Aggregates Recycled from Precasting Industry Waste: Influence of the Crushing Process.” Materials and Structures 48 (12): 3965–3978. doi:https://doi.org/10.1617/s11527-014-0456-7.
- Qasrawi, H. 2014. “The Use of Steel Slag Aggregates to Enhance the Mechanical Properties of Recycled Aggregate Concrete and Retain the Environment.” Construction and Building Materials 54: 298–304. doi:https://doi.org/10.1016/j.conbuildmat.2013.12.063.
- Richardson, A., K. Coventry, and J. Bacon. 2011. “Freeze/thaw Durability of Concrete with Recycled Demolition Aggregate Compared to Virgin Aggregate Concrete.” Journal of Cleaner Production 19 (2–3): 272–277. doi:https://doi.org/10.1016/j.jclepro.2010.09.014.
- Silva, R. V., J. De Brito, and R. K. Dhir. 2015. “Prediction of the Shrinkage Behavior of Recycled Aggregate Concrete: A Review.” Construction and Building Materials 77: 327–339. doi:https://doi.org/10.1016/j.conbuildmat.2014.12.102.
- Song, H. W., and V. Saraswathy. 2006. “Studies on the Corrosion Resistance of Reinforced Steel in Concrete with Ground Granulated Blast-furnace Slag—an Overview.” Journal of Hazardous Materials 138 (2): 226–233. doi:https://doi.org/10.1016/j.jhazmat.2006.07.022.
- Tan, K., and X. Pu. 1998. “Strengthening Effects of Finely Ground Fly Ash, Granulated Blast Furnace Slag, and Their Combination.” Cement and Concrete Research 28 (12): 1819–1825. doi:https://doi.org/10.1016/S0008-8846(98)00158-6.
- Tuyan, M., A. Mardani-Aghabaglou, and K. Ramyar. 2014. “Freeze–thaw Resistance, Mechanical and Transport Properties of Self-consolidating Concrete Incorporating Coarse Recycled Concrete Aggregate.” Materials & Design 53: 983–991. doi:https://doi.org/10.1016/j.matdes.2013.07.100.
- Xiao, J., D. Lu, and J. Ying. 2013. “Durability of Recycled Aggregate Concrete: An Overview.” Journal of Advanced Concrete Technology 11 (12): 347–359. doi:https://doi.org/10.3151/jact.11.347.
- Zaharieva, R., F. Buyle-Bodin, and E. Wirquin. 2004. “Frost Resistance of Recycled Aggregate Concrete.” Cement and Concrete Research 34 (10): 1927–1932. doi:https://doi.org/10.1016/j.cemconres.2004.02.025.