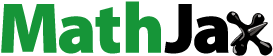
ABSTRACT
To minimise the food waste, this paper applied the antimicrobial plates produced from poly(butylene succinate) (PBS)/geraniol blend which was processed into two different microstructures. Experimental work was separated into three major parts. The first one was the determination of a suitable geraniol concentration by minimum inhibitory concentration and inhibitory clear zone techniques. After that a selected PBS/geraniol composition was then processed into the solid and porous antimicrobial plates of 15 mm in diameter and 2 mm in thickness. Finally, the solid and porous antimicrobial plates were inserted in bread package and evaluated the shelf-life extension performances. Experimental results indicated that a suitable geraniol composition was 8 wt%. Migration test confirmed that geraniol released from both solid and porous plates by more than 30 days. Shelf-life evaluation by counting the microorganisms indicated that applying antimicrobial plates in bread package was found to extend by around 5 and 10 days with solid and porous antimicrobial plates, respectively. In summary, both antimicrobial plates are possibly applied for bread shelf-life extension which easily inserts inside any food container. This is a practical use for retail and household stages.
1. Introduction
Food is normally transported from manufacturer to consumer through food processing, distribution, retailing and household consumption in a domino-like manner in the food supply chain. Simultaneously, consumers pay their money for food and it transfers to producers and farmers as the reverse process. Each process generates a large amount of food loss and waste (FLW). FLW is recognised as a terrible intimidation to environmental sustainability, economy and food security (Abiad and Meho Citation2018; Ishangulyyev, Kim, and Lee Citation2019; Vázquez-Rowe et al. Citation2020). Around 30% of food or approximately 1.3 billion tons are lost and wasted along supply chain every year (Ishangulyyev, Kim, and Lee Citation2019). Moreover, 12% of this food waste comes from bread products. The economic values of this amount of FLW are predicted by more than 900 billion USD. Contents of FLW are estimated to relieve by around 12.5% of the world’s population, inversely with the increased demand around 150–170% of the current demand by 2050 (FAO Citation2009) which makes pressure on the food supply chain to supply more foods. These problems have drawn much attention from both industrial and academic sectors to alleviate the FLW problems (Gustavsson et al. Citation2011; Le Mouël and Forslund Citation2017; Ishangulyyev, Kim, and Lee Citation2019).
Bread is one of the most widely consumed around the world, and this generates large amounts of waste every year. The Waste and Resources Action Programme (WRAP) has forecasted that around 10% of food waste accumulated in the UK is bread (WRAP, H., Link Consumer Strategies, Campden BRI Citation2011; Brancoli, Bolton, and Eriksson Citation2020). In Sweden, Brancoli et al. (Citation2019) have predicted that around 80,000 tons of bread is wasted annually. These losses of bread can occur in three levels e.g., 1–2% loss in the manufacturing stage, 3–7% loss in the retail stage and 18% loss from consumer or household stage.
In the retail and household stages, the deterioration of food is generally caused by the growth of microbial organism and expire on-shelf before selling or consuming. Nowadays, there are many technologies provided to avoid food from the unpleasant deterioration without the addition of food preservatives. The most widely known and used technique is the application of antimicrobial food packaging. Antimicrobial packaging technology is generally known as a packaging material incorporated with active compound which provides some interactions between food and package. These active compounds release and reduce or obstruct the growth of microorganisms related to food spoilage or pathogens which delays the deterioration of food. The method of manufacturing of this antimicrobial packaging is the blend or coat of antimicrobial agent into the polymer package during process (Petchwattana and Naknaen Citation2015; Motelica et al. Citation2020). A widely used technique is the use of essential oils which liberate the volatile to inhibit the microbial growth via various mechanisms such as i) prohibition of the syntheses of protein, ii) nucleic acid synthesis and cell wall and iii) disruption of cell membrane function and block the metabolisms pathways of microorganisms (Khaneghah, Hashemi, and Limbo Citation2018; Hoseinnejad, Jafari, and Iman Katouzian Citation2018). The application of this kind of packaging was found in many food products such as breads, fruits, seafood or meats (Cavalcante et al. Citation2019; Guijarro-Fuertes et al. Citation2019; Mesa, Manjarres-Pinzon, and Rodriguez-Andoval Citation2019).
Many researchers have reported some progresses of the food shelf-life extension by using antimicrobial packaging prepared from polymer/essential oils blends. In poly(butylene succinate) (PBS) film, thymol essential oil was added as an active antimicrobial agent. Release test of thymol indicated that the antimicrobial activity was the most effective over 15 days which suitable for the short shelf-life food packaging application (Petchwattana and Naknaen Citation2015). Gao et al. (Citation2017) produced poly(lactic acid) (PLA) with antimicrobial properties induced by allyl isothiocyanate (AIT). At the storage condition of 4 and 10°C, AIT could delay the spoilage of fresh pakchoi for 15 days. Campos-Requena et al. (Citation2015) prolonged the storage duration of fresh strawberries stored in an active packaging produced from low-density polyethylene (LDPE), thymol and carvacrol essential oil blends. This antimicrobial package can extend the life of strawberry by about two times compared with that conventional package. Ramos et al. (Citation2013) incorporated thymol-antimicrobial compound in bioplastic film. They found that the shelf-life of bakery product was increased by around 7 days. Although numerous literatures had shown the successful of food shelf-life extension of the applied essential oils-based antimicrobial packages. However, the release of the essential oil occurs over both inside and outside of the package which reduces antimicrobial efficiency. Moreover, the strong odour of some types of essential oils interferes with other products placed nearby.
Therefore, this research aims to apply two different microstructures, e.g. solid and porous, antimicrobial plates for the shelf-life extension of bread. These antimicrobial plates were fabricated to have the inside diameter around 15 mm which is easily inserted inside any food container. It is a practical use for retail and household stages food waste minimisation. A bio-PBS was applied as polymer matrix due to its low melting temperature suitable for the antimicrobial plate processing and its complete-biodegradability. Geraniol was applied as an antimicrobial agent due to its safety and efficiency. To produce the antimicrobial plate with the porous structures, sodium bicarbonate (SBC) was used as a foaming agent in PBS/geraniol blend. An investigation of the antimicrobial performance on the Bacillus cereus (Gram-positive) and Escherichia coli (Gram-negative) inhibition was observed together with some physical and thermal properties and the mass transfer estimation . Further investigations on the shelf-life extension performed on white bread slice stored with and without antimicrobial plate were also made.
2. Materials processing and testing
2.1 Raw materials
A bio-PBS (FZ91PM, PTT MCC Biochem) was employed as a polymer matrix. It has a melt-temperature of 115°C and density of 1.26 g/cm3. A 98% geraniol (trans-3,7-dimethyl-2,6-octadien-1-ol) (Sigma-Aldrich) was incorporated as an active agent to inhibit the growth of both B. cereus and E. coli. It has a molecular weight, density at 20°C and boiling point of 154.25 g/mol, 0.897 g/cm3 and 230°C, respectively. For the porous antimicrobial plate, SBC was further added in PBS/geraniol composition as a foaming agent. It liberates CO2 gas via endothermic reaction shown in EquationEquation (1)(1)
(1) by around 100 ml/g at 130–180°C.
In this paper, white bread is selected to represent a short-shelf-life food. It is widely consumed in every part of the world. The process of making bread mostly contacts with human through the production process. This is possible to contaminate with pathogenic bacteria during production, packaging, transportation and selling. These bacteria possibly stimulated the bread deterioration which possibly become food waste. White bread was obtained from a contract manufacturer who exactly knows the compositions, production and expiration dates. The white bread formulation is clearly listed in .
Table 1. Composition of white bread production
2.2 Experimental procedure and production process of antimicrobial plate
2.2.1 Blending PBS/geraniol for minimum geraniol concentration determination
To determine the minimum geraniol concentration for the production of antimicrobial plate, PBS was dry- and melt-blended with various geraniol concentrations of 4 to 10 wt% by using a twin screw extruder (Chareontut, CTE-D16L32). It was operated at the barrel temperatures of 115/120/130/130/130°C for the heating zones 1–5, respectively. The extrudate was then cut into a small piece with 2.0 mm in diameter. To evaluate the antimicrobial performance of each composition, B. cereus and E. coli were chosen to measure their viability by agar diffusion method due to their possibility of contaminating into bread, from human, during production process. An inhibition clear zone diameter was measured after the antimicrobial plate which was set on the agar surface and incubated at 37°C for 24 h.
2.2.2 Antimicrobial plate production process
After selecting a suitable PBS/geraniol composition in section 2.2.1, antimicrobial plate was produced by separating into two different microstructures.
For the solid antimicrobial plate, a selected PBS/geraniol composition was melt blended by a twin screw extruder (Chareontut, CTE-D16L32) at the identical processing condition addressed in section 2.2.1. The extrudate was then compressed by a compression moulding machine (Chareontut, PR2D-W300L350-PM-WCL-HMI) to obtain a solid antimicrobial plate of 2.0 mm in thickness and 15 mm in diameter, as shown in ).
For the porous plate, PBS and geraniol were melt-extruded with 2 wt% SBC (based on the previous work (Petchwattana and Covavisaruch Citation2011)). The extrusion temperature was set to avoid the decomposition of SBC while maintaining the PBS melting. The PBS/geraniol/SBC composition was compressed to obtain a round plate with the same dimension of the solid plate as shown in ). The compression moulding condition was designed to allow SBC to decompose and generate the foam structure in antimicrobial plate at a closed-moulding temperature of 180°C.
2.3 Packaging procedure
To control the initial microbial amount in the packaging system, a LDPE zipped lock bag was dried in a hot air oven at 65°C for 30 min. After that a 15 mm thick white bread slice, bought from a local store in Nakhon Nayok, Thailand, was packed individually in 16 × 22 cm LDPE bag without and with solid and porous antimicrobial plates. They were stored at 27 ± 2°C in an endeavour to simulate a real commercialisation condition of bread products. All the test samples were produced at the same lot and expired in the same day.
2.4 Testing and characterisations
2.4.1 Selecting a suitable geraniol concentration
E. coli (Gram-negative) and B. cereus (Gram-positive), concentration of 108 CFU/ml, were selected to determine the minimal inhibitory concentration (MIC). A 24-h-old colony of bacteria was cultured in liquid culture media and kept at 37°C. After that, it was diluted with culture media to reduce the concentration and then kept at 37°C for 24 h again to check MIC results.
To determine a suitable geraniol concentration for antimicrobial plate production, B. cereus and E. coli were chosen to measure their viability by agar diffusion method. A clear zone diameter was measured after the PBS/geraniol compositions were set on the agar surface and incubated at 37°C for 24 h.
2.4.2 Suitable processing temperature for SBC
To estimate the suitable processing temperature and foaming condition of SBC, a simultaneous thermal analyser (TA instruments, SDT Q600) was employed to monitor the thermagavimetric analysis (TGA) and differential scanning calorimetry (DSC) behaviours. This was operated at a thermal scan of 10°C/min in N2 gas environment. The quantity of SBC used was 10 mg. The TGA and DSC test was operated from room temperature to the maximum temperature of 400°C.
2.4.3 Microscopic observation
The fracture morphology of the neat PBS, solid PBS/geraniol and porous PBS/geraniol blends was observed by a SEM (JEOL, JSM-IT300). Each sample was cryogenic fractured in liquid N2, then placed on aluminium stub and coated with gold.
2.4.4 Headspace release of geraniol form solid and porous plates
The headspace release of geraniol from PBS was measured from 0 to 30 days of storage. Measurement was conducted at room temperature using a gas chromatography (GC, Nexis GC-2030) installed with a flame ionisation detector. The sample, 2 ml, was directly injected onto the column with a 10 ml syringe. The GC oven temperature was set at a ramp rate of 10°C/min from 80°C to 230°C. Helium was used as a carrier gas with the volumetric flow rate of 32 ml/min operating at an injector and detector temperatures of 230°C. The preparation of geraniol (98%) standard solution was done by dissolving 1 ml of each compound in 100 ml ethanol.
2.4.5 Shelf-life extension of bread
An evaluation of the antimicrobial efficiency was investigated by applying antimicrobial plates in white bread slice at the storage duration of 0 to 30 days. The total plate count (PCA medium, 30°C) and yeast and mould counts (YGC medium, 25°C) were determined following the FDA-BAM Chapters 3 and 18 (2001).
2.4.6 Statistical analysis
The antimicrobial and bread shelf-life test reports were obtained from the average of three replicated samples. Data were analysed using an analysis of variance (ANOVA) with the statistical significance calculated at P < 0.05.
3. Results and discussions
3.1 Suitable geraniol concentration determination
The MIC results, shown in , indicated that B. cereus required 1562 µg/ml to inhibit the bacterial growth, while E. coli did at 3125 µg/ml. In comparison, B. cereus was more responsive to geraniol than that of E. coli due to the cell wall of Gram-positive bacteria.
Table 2. Minimal inhibitory concentration (MIC) of B. cereus and E. coli.
To determine a suitable geraniol concentration, the blend compositions of PBS and geraniol were microbiologically measured via agar diffusion method. shows the inhibition clear zones detected from PBS/geraniol blends, at 0–10 wt%, against B. cereus and E. coli. Their quantitative data are clearly presented in . In , geraniol was effectively inhibited the foodborne pathogens growth against both B. cereus and E. coli used in this study. However, geraniol appeared to have less effectiveness against E. coli with lower inhibition clear zone. Previous reports informed that the cell wall of Gram-positive bacteria is mostly comprised of peptidoglycan which allows the hydrophobic molecules to conveniently penetrate and disrupt their cells. On the other hand, Gram-negative bacteria have an outer membrane which comprises of a double-layer of phospholipids and lipopolysaccharides (Antunes et al. Citation2017). This makes the Gram-negative bacteria has more geraniol resistance than that of Gram-positive bacteria (Wiburanawong, Petchwattana, and Covavisaruch Citation2014; Petchwattana and Naknaen Citation2015; Antunes et al. Citation2017).
Table 3. Inhibition zone diameter of the PBS/geraniol blends
Figure 2. Antimicrobial activities of PBS/geraniol blends against B. cereus: (a) neat PBS, (b) 4 wt% (c) 6 wt%, (d) 8 wt%, (e) 10 wt%, and E. coli (f) neat PBS, (g) 4 wt%, (h) 6 wt%, (i) 8 wt% and (j) 10 wt% geraniol
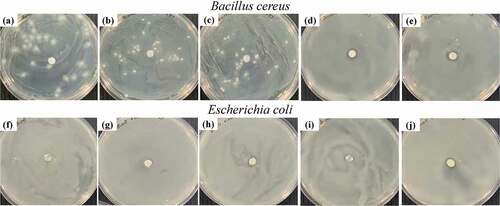
Based on the above results, the incorporation of 8 wt% geraniol in the PBS was an appropriate amount for the growth inhibition of both Gram-positive and Gram-negative bacteria in this study. In a thermoplastic starch/carvacrol essential oil and montmorillonite films, the strong antimicrobial properties against E. coli were found at 15 wt% carvacrol (Pilati et al. Citation2013). Blending cinnamon essential oil with sago starch films revealed a very good antimicrobial efficiency against E. coli, Salmonella typhimurium, and Staphylococcus aureus (Arezoo et al. Citation2020).
3.2 Decomposition temperature of SBC
illustrates the TGA and DSC thermograms of SBC which exhibited a broad thermal decomposition ranging from 130 to 180°C. From TGA thermogram, the amount of gas yielded by around 25% was achieved. DSC thermogram confirmed that the decomposition of SBC is truly endothermic. Its decomposition took place at the identical range found in TGA. The decomposition data was applied as a compression moulding condition of PBS/geraniol/SBC, at 180°C, to maximise the amount of gas yielded from the decomposition.
3.3 Microscopic observations
The SEM micrograph, in , was employed to compare the microstructure among neat PBS, solid PBS/geraniol plate and porous PBS/geraniol plate. ) shows a rather smooth fracture surface related to rapid crack propagation during the test preparation. With the addition of 8 wt% geraniol, ), the fracture surface seemed rougher due to the plasticisation effect derived by essential oil. This phenomenon was previously reported in other polymer/essential oil blends (Wiburanawong, Petchwattana, and Covavisaruch Citation2014; Petchwattana and Naknaen Citation2015; Liu et al. Citation2016). In ), the porous structure of PBS with the closed cells was observed. This indicated that geraniol was completely encapsulated in the foam structure of PBS which possibly prolong and increase the diffusion rate of geraniol.
3.4 Release of geraniol
illustrates the headspace concentration of geraniol which plotted the release versus storage time of the solid and porous antimicrobial plates from day 0 to day 30. At the initial state, the amount of geraniol released was found to increase rapidly during the storage time from 0 to 10 days with the released volatile of around 180–190 ppm. There was no significant difference between geraniol released from solid and porous plates. After 15 days, the porous plate seemed to be more effective than that of the solid plate. The release of the porous plate reached the equilibrium value at around 250 ppm while the solid plate did at around 200 ppm. This is mainly due to the higher amount of geraniol encapsulated in antimicrobial plate structures as evidenced in . For the solid plate, there was a possibility of migration of geraniol to its surface due to the chain mobility of PBS which was previously reported in many literatures on polymer/essential oils blend systems (Quintavalla and Vicini Citation2002; Suppakul et al. Citation2003; Kuorwel et al. Citation2013; Requena, Vargas, and Chiralt Citation2019). For the porous plate, geraniol was possibly encapsulated in two possible locations, e.g. free volumes and inside foam cells. The amounts of geraniol released from both plates were directly correlated to the antimicrobial activity.
3.5 Shelf-life extension of bread
Measurements of the total microbial and yeast and mould colonies applied on bread slice, with and without antimicrobial plate, during the storage period of 30 days are clearly illustrated in . Overall, the total plate count and yeast and mould counts of bread slice were increased with the storage period. For the control sample, the total microbial and yeast and mould counts reached the limit of spoilage, regulated by Thai-FDA, before 10 days of storage. For the solid antimicrobial plate, the total plate count indicated that bread samples reached the spoilage limit of 104 CFU/g at day 15 while the porous plate did at around day 20. Yeast and mould counts confirmed that the bread stored with porous plate had much lower colonies of yeast and mould than that stored with solid plate. Based on the antimicrobial test data, it can be summarised that the shelf-life of bread was extended by around 5 and 10 days, compared with that control sample, with the use of solid and porous plates, respectively. This achievement was mainly due to the release of geraniol volatile in the bread slice package. These results are in good correlation with release test results in and visual appearance of bread in . The control sample was found to show the green and grey mould since around day 20, while the bread with both antimicrobial plates showed the appearance identity of mould at day 30. For this case, Inouye, Takizawa, and Yamaguchi (Citation2001) concluded that, in the gaseous state, geraniol showed its antibacterial performance against various pathogens, which include Haemophilus influenzae, Staphylococcus aureus, Streptococcus pneumonia and Streptococcus pyogenes. Geraniol was also found to reduce the germ count by 64% when in the gas state (Sato, Krist, and Buchbauer Citation2007). A report by Hui et al. (Citation2017) indicated that geraniol and other essential oils are the multi-targeted antimicrobial agents. The primary mechanism is the cell membrane disruption through the enveloping of lipophilic segments of bacterial cell membrane. The secondary mechanism is a prohibition of the 1-deoxy-D-xylulose 5-phosphate reductoisomerase function. For the retail-stage food waste minimisation point of view, the current antimicrobial plate increases the shelf-life and allows food to sell out before their expiry dates. This is a practical way of the sustainable food waste minimisation.
4. Conclusions
In this paper, the solid and porous PBS/geraniol antimicrobial plates were successfully prepared. Experimental works indicated that the MIC of B. cereus and E. coli were 1562 and 3125 µg/ml, respectively. This is related to the geraniol concentration of 8 wt% in PBS matrix . Release test confirmed that the release time was more than 30 days. Shelf-life evaluation by counting the microorganisms indicated that bread shelf-life was increased by around 5 and 10 days with the incorporation of solid and porous antimicrobial plates, respectively. In summary, both antimicrobial plates are possibly applied for long-term food shelf-life extension which easily inserts inside any food container which is practical for retail-stage food waste minimisation. From an economic point of view, the cost of antimicrobial plate (estimated from raw materials and processing costs) was around 0.015 USD. This is very small compared to the benefits obtained from the increased shelf-life.
Acknowledgments
The current work was financially granted by the Mid-career research grant from the collaboration of the National Research Council of Thailand (NRCT) (Contract no. NRCT5-RSA63020-01) and Srinakharinwirot University (Contract no. 053/2564).
Disclosure statement
No potential conflict of interest was reported by the author(s).
Additional information
Funding
Notes on contributors
Nawadon Petchwattana
Dr. Nawadon Petchwattana received a doctoral degree in Chemical Engineering from Chulalongkorn University in 2010. He is now an associate professor at Srinakharinwirot University. His field of expertise is biodegradable polymers, food packaging and circular economy.
Phisut Naknaen
Dr. Phisut Naknaen received a doctoral degree in Food Technology from Prince of Songkla University in 2010. He is now an assistant professor at Srinakharinwirot University. His field of expertise is food chemistry and food packaging.
Kamonchai Cha-Aim
Dr. Kamonchai Cha-aim received a doctoral degree in Biotechnology from Yamaguchi University. He is now an assistant professor at Srinakharinwirot University. His field of expertise is yeast genetics and food microbiology.
Jakkid Sanetuntikul
Dr. Jakkid Sanetuntikul received a doctoral degree in Energy Science and Engineering from Daegu Gyeongbuk Institute of Science and Technology. He is now an associate professor at King Mongut's University of Technology North Bangkok. His field of expertise is energy technology and materials technology.
References
- Abiad, M. G., and L. I. Meho. 2018. “Food Loss and Food Waste Research in the Arab World: A Systematic Review.” Food Security 10 (2): 311–322. doi:https://doi.org/10.1007/s12571-018-0782-7.
- Antunes, M. D., G. Da Silva Dannenberg, A. M. Fiorentini, V. Z. Pinto, L. T. Lim, E. Da Rosa Zavareze, and A. R. Guerra Dias. 2017. “Antimicrobial Electrospun Ultrafine Fibers from Zein Containing Eucalyptus Essentialoil/cyclodextrin Inclusion Complex.” International Journal of Biological Macromolecules 104: 874–882. doi:https://doi.org/10.1016/j.ijbiomac.2017.06.095.
- Arezoo, E., E. Mohammadreza, M. Maryam, and M. N. Abdorreza. 2020. “The Synergistic Effects of Cinnamon Essential Oil and Nano TiO2 on Antimicrobial and Functional Properties of Sago Starch Films.” International Journal of Biological Macromolecules 157: 743–751. doi:https://doi.org/10.1016/j.ijbiomac.2019.11.244.
- Brancoli, P., K. Bolton, and M. Eriksson. 2020. “Environmental Impacts of Waste Management and Valorisation Pathways for Surplus Bread in Sweden.” Waste Management 117: 136–145. doi:https://doi.org/10.1016/j.wasman.2020.07.043.
- Brancoli, P., M. Lundin, K. Bolton, and M. Eriksson. 2019. “Bread Loss Rates at the Supplier-retailer interface–Analysis of Risk Factors to Support Waste Prevention Measures.” Resources, Conservation & Recycling 147: 128–136.
- Campos-Requena, V. H., B. L. Rivas, M. A. Pérez, C. R. Figueroa, and E. A. Sanfuentes. 2015. “The Synergistic Antimicrobial Effect of Carvacrol and Thymol in Clay/polymer Nanocomposite Films over Strawberry Gray Mold.” LWT - Food Science and Technology 64 (1): 390–396. doi:https://doi.org/10.1016/j.lwt.2015.06.006.
- Cavalcante, R. B. M., M. A. Morgano, M. B. A. Glória, M. M. Rocha, A. A. M. Araújo, and R. S. R. Moreira-Araújo. 2019. “Mineral Content, Phenolic Compounds and Bioactive Amines of Cheese Bread Enriched with Cowpea.” Food Science and Technology 39 (4): 843–849. doi:https://doi.org/10.1590/fst.11718.
- FAO. 2009. “FAO’s Director-general on How to Feed the World in 2050.” Population and Development Review 35 (4): 837–839. doi:https://doi.org/10.1111/j.1728-4457.2009.00312.x.
- Gao, H., X. Fang, H. Chen, Y. Qin, F. Xu, and T. Z. Jin. 2017. “Physiochemical Properties and Food Application of Antimicrobial PLA Film.” Food Control 73: 1522–1531. doi:https://doi.org/10.1016/j.foodcont.2016.11.017.
- Guijarro-Fuertes, M., M. J. Andrade-Cuvi, J. Bravo-Vásquez, L. Ramos-Guerrero, and M. G. Vernaza. 2019. “Andean Blueberry (Vaccinium Floribundum) Bread: Physicochemical Properties and Bioaccessibility of Antioxidants.” Food Science and Technology 39 (Suppl. 1): 56–62. doi:https://doi.org/10.1590/fst.30317.
- Gustavsson, J., C. Cederberg, U. Sonesson, R. Van Otterdijk, and A. Meybeck. 2011. Global Food Losses and Food Waste. Rome, Italy: FAO.
- Hoseinnejad, M., A. M. Jafari, and I. Iman Katouzian. 2018. “Inorganic and Metal Nanoparticles and Their Antimicrobial Activity in Food Packaging Applications.” Critical Reviews in Microbiology 44 (2): 161–181. doi:https://doi.org/10.1080/1040841X.2017.1332001.
- Hui, X., G. Yan, F.-L. Tian, H. Li, and W.-Y. Gao. 2017. “Antimicrobial Mechanism of the Major Active Essential Oil Compounds and Their Structure–activity Relationship.” Medicinal Chemistry Research 26 (2): 442–449. doi:https://doi.org/10.1007/s00044-016-1762-0.
- Inouye, S., T. Takizawa, and H. Yamaguchi. 2001. “Antibacterial Activity of Essential Oils and Their Major Constituents against Respiratory Tract Pathogens by Gaseous Contact.” Journal of Antimicrobial Chemotherapy 47 (5): 565–573. doi:https://doi.org/10.1093/jac/47.5.565.
- Ishangulyyev, R., S. Kim, and S. H. Lee. 2019. “Understanding Food Loss and Waste-why are We Losing and Wasting Food?” Foods 8 (8): 297. doi:https://doi.org/10.3390/foods8080297.
- Khaneghah, A. M., S. M. B. Hashemi, and S. Limbo. 2018. “Antimicrobial Agents and Packaging Systems in Antimicrobial Active Food Packaging: An Overview of Approaches and Interactions.” Food and Bioproducts Processing 111 (1–19): 2018. doi:https://doi.org/10.1016/j.fbp.2018.05.001.
- Kuorwel, K. K., M. J. Cran, K. Sonneveld, J. Miltz, and S. W. Bigger. 2013. “Migration of Antimicrobial Agents from Starch-based Films into a Food Simulant.” LWT – Food Science and Technology 50 (2): 432–438. doi:https://doi.org/10.1016/j.lwt.2012.08.023.
- Le Mouël, C., and A. Forslund. 2017. “How Can We Feed the World in 2050? A Review of the Responses from Global Scenario Studies.” European Review of Agricultural Economics 44 (4): 541–591. doi:https://doi.org/10.1093/erae/jbx006.
- Liu, D., H. Li, L. Jiang, Y. Chuan, M. Yuan, and H. Chen. 2016. “Characterization of Active Packaging Films Made from Poly(lactic Acid)/poly(trimethylene Carbonate) Incorporated with Oregano Essential Oil.” Molecules 21 (6): 695. doi:https://doi.org/10.3390/molecules21060695.
- Mesa, E., K. Manjarres-Pinzon, and E. Rodriguez-Andoval. 2019. “Gluten-free Cheese Bread from Frozen Dough: Effect of Modified Cassava Starch.” Food Science and Technology 39 (Suppl. 2): 654–661. doi:https://doi.org/10.1590/fst.30118.
- Motelica, L., D. Ficai, A. Fica, O. C. Oprea, D. A. Kaya, and E. Andronescu. 2020. “Biodegradable Antimicrobial Food Packaging: Trends and Perspectives.” Foods 9 (10): 1438. doi:https://doi.org/10.3390/foods9101438.
- Petchwattana, N., and S. Covavisaruch. 2011. “Influences of Modified Chemical Blowing Agents on Foaming of Wood Plastic Composites Prepared from Poly(vinyl Chloride) and Rice Hull.” Advanced Materials Research 306-307: 869–873. doi:10.4028/www.scientific.net/AMR.306-307.869.
- Petchwattana, N., and P. Naknaen. 2015. “Utilization of Thymol as an Antimicrobial Agent for Biodegradable Poly(butylene Succinate).” Materials Chemistry and Physics 163: 369–375. doi:https://doi.org/10.1016/j.matchemphys.2015.07.052.
- Pilati, F., M. Degli Esposti, M. Bondi, R. Iseppi, and M. Toselli. 2013. “Designing of Antibacterial Plastics: Thymol Release from Photocured Thymol-doped Acrylic Resins.” Journal of Materials Science 48 (12): 4378–4386. doi:https://doi.org/10.1007/s10853-013-7253-5.
- Quintavalla, S., and L. Vicini. 2002. “Antimicrobial Food Packaging in Meat Industry.” Meat Science 62 (3): 373–380. doi:https://doi.org/10.1016/S0309-1740(02)00121-3.
- Ramos, M., A. Beltrán, A. Valdés, M. A. Peltzer, A. Jimenez, M. C. Garrigos, and G. E. Zaikov. 2013. “Carvacrol and Thymol for Fresh Food Packaging.” Journal of Bioequivalence & Bioavailabilit 5 (4): 154–160. doi:https://doi.org/10.4172/jbb.1000151.
- Requena, R., M. Vargas, and A. Chiralt. 2019. “Eugenol and Carvacrol Migration from PHBV Films and Antibacterial Action in Different Food Matrices.” Food Chemistry 277: 38–45. doi:https://doi.org/10.1016/j.foodchem.2018.10.093.
- Sato, K., S. Krist, and G. Buchbauer. 2007. “Antimicrobial Effect of Vapours of Geraniol, (R)-(−)-linalool, Terpineol, γ-terpinene and 1, 8-cineole on Airbone Microbes Using an Air Washer.” Flavour and Fragrance Journal 22 (5): 435–437. doi:https://doi.org/10.1002/ffj.1818.
- Suppakul, P., J. Miltz, K. Sonneveld, and S. W. Bigger. 2003. “Active Packaging Technologies with an Emphasis on Antimicrobial Packaging and Its Applications.” Journal of Food Science 68 (2): 408–420. doi:https://doi.org/10.1111/j.1365-2621.2003.tb05687.x.
- Vázquez-Rowe, I., J. Laso, M. Margallo, I. Garcia-Herrero, D. Hoehn, F. Amo-Setién, A. Bala, et al. 2020. “Food Loss and Waste Metrics: A Proposed Nutritional Cost Footprint Linking Linear Programming and Life Cycle Assessment.” The International Journal of Life Cycle Assessment 25 (7): 1197–1209. doi:https://doi.org/10.1007/s11367-019-01655-1.
- Wiburanawong, S., N. Petchwattana, and S. Covavisaruch. 2014. “Carvacrol as an Antimicrobial Agent for Poly(butylene Succinate): Tensile Properties and Antimicrobial Activity Observations.” Advanced Materials Research 931-932: 111–115. doi:10.4028/www.scientific.net/AMR.931-932.111.
- WRAP, H., Link Consumer Strategies, Campden BRI. 2011. Reducing Household Bakery Waste. Banbury, UK: WRAP, Waste and Resource Action Programme.