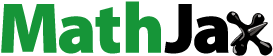
ABSTRACT
The worldwide biomedical polymeric waste (BPW) production from dialysis treatment is estimated to be reached about 1.6 million tons/year by 2025, dramatically raising financial, environmental, and ecological concerns. Thanks to new technologies, the waste is disinfected for being discarded as non-hazardous waste, incinerated or landfilled, making the disinfection process redundant and increasing the disposal costs. This study takes advantage of BPW to improve water sorptivity and electrical resistivity of concrete and lightens the way towards environmental-friendly disposal of BPW. Three Hybrid, Soft, and Hard BPW were added to concrete from 0% to 9% by weight of cement in 13 different mixtures. Afterwards, electrical resistivity, long-term capillary water absorption tests were conducted. Predictive models were then developed in three time periods. The addition of Hybrid and Hard BPW improved the capillary absorption and sorptivity by 28%, but, surprisingly, Soft BPW addition showed an adverse effect. Electrical resistivity also improved up to 22.1% after BPW addition. Considering the results, utilising Hybrid BPW in concrete eliminates the waste separating phase and can improve the concrete’s durability, saving time and costs.
1- Introduction
Environmental impacts of healthcare have been dramatically neglected up to recently despite climate scientists’ warnings for damaging effects of greenhouse and carcinogen gases generation and landfilling (Agar Citation2019; Barnes et al. Citation2009). Among healthcare practices, nephrology and particularly dialysis is of the most BPW producers, which about 1.5 to 8 kg BPW after each treatment (400 kg/patient/year) is usually discarded by landfilling or combusting [3]. The financial cost of biomedical waste disposal is high, from 2.7 to 21 USD, and it is estimated that the worldwide number of dialysis patients to be reached about 4 million by 2025 (Piccoli et al. Citation2015; Tarrass et al. Citation2010). In this regard, it is estimated that around 1.6 million ton/year BPW is generated globally from dialysis treatment by 2025. The mentioned numbers represent critical challenges to healthcare budgets and more dramatic environmental and ecological problems. Since most polymers are not biodegradable, they accumulate in landfills, and the combustion treatment of polymeric waste also raises air pollution concerns (Schecter et al. Citation2006). According to the literature, 49–60% of biomedical waste is incinerated, 20–37% is autoclaved, and 4–5% is treated by other technologies (Windfeld and Brooks Citation2015). Although autoclaving and microwaving are considered environment-friendly compared with incineration, the waste volume does not change after treatment, increasing the disposal cost and making the disinfection process redundant (Jang et al. Citation2006; Klangsin and Harding Citation1998).
Since recent decades, recycling waste materials in the forms of resins, aggregate, and fibre (M. Al-Tayeb et al. Citation2020; Nematzadeh, Tayebi, and Samadvand Citation2021; Seco et al. Citation2020; Stallings, Durham, and Chorzepa Citation2019; S. Xu et al. Citation2018) as well as pozzolanic materials (Golewski Citation2020a, Citation2020b, Citation2021; Szostak and Golewski Citation2020) in concrete is considered an environment-friendly, safe, and economical disposal approach among different waste management and recycling approaches. Several fresh and hardened properties of mortars and concrete containing different types of polymers have been researched. However, there is still a scarcity of knowledge concerning the durability of concrete containing polymeric waste (Jacob-Vaillancourt and Sorelli Citation2018; Mohammadhosseini and Tahir Citation2018). According to the literature, transport properties of concrete, namely, sorptivity, permeability, and diffusivity, are among the most important indicators for determining the durability and serviceability of concrete structures (Alderete, Zaccardi, and De Belie Citation2020; Koushkbaghi et al. Citation2019; Tibbetts et al. Citation2020). Moreover, the ability of concrete to withstanding the penetration of aggressive substances crucially depends on the pore structures and porosity of its matrix (Cantero et al. Citation2020; Li, Yang, and Yang Citation2019; Tahmouresi et al. Citation2019). In this regard, it is vital to investigate the contributing factors and understand the pore system of the new concrete. Furthermore, water absorption and electrical resistivity test methods are increasingly used for describing the transport properties of concrete (Cantero et al. Citation2020; Kurda, De Brito, and Silvestre Citation2019).
Previous studies (Albano et al. Citation2009; K. G. Babu and Babu Citation2004; Choi et al. Citation2009; Fraj et al. Citation2010; Marzouk, Dheilly, and Queneudec Citation2007; Saikia and Brito Citation2013; Silva, De Brito, and Saikia Citation2013) reported an increase in the water absorption of the lightweight concrete and mortars containing polymeric materials used as aggregate. They related it to insufficient mix and different size distribution of the waste from natural aggregate, resulting in a more porous matrix (Gu and Ozbakkaloglu Citation2016). Marzouk et al. (Marzouk, Dheilly, and Queneudec Citation2007) investigated the sorptivity of cement mortars containing waste polyethylene terephthalate as aggregate. They reported lower sorptivity after up to 50% waste substitution by volume of sand. Hannawi et al. (Hannawi, Kamali-Bernard, and Prince Citation2010) also resulted in a lower sorptivity of mortars containing polyethylene and polycarbonate aggregates, separately, and related it to the hydrophobic nature of the polymers. However, Choi et al. (Choi et al. Citation2009) resulted in a higher sorptivity than control mortar after replacing 50% and 75% of powder-coated Polyethylene terephthalate aggregate by weight of fine aggregate. Silva et al. (Silva, De Brito, and Saikia Citation2013) also reported an increase in water absorption by either immersion and capillarity. Regarding the resistivity also, Şimşek et al. (Şimşek et al. Citation2018) studied the effect of Dioctyl terephthalate on the electrical resistivity of concrete. They reported higher electrical resistivity than the normal weight concrete by utilising Dioctyl terephthalate in concrete containing polyethylene terephthalate added by 10% of fine aggregate.
1-2 Research significance
Minimising the sorptivity, which is the rate of absorbed water by a certain area of the test specimen, is vital to limit the ingress of sulphate or chloride contained water into concrete (Bai, Wild, and Sabir Citation2002; Shadmani et al. Citation2018). It is also crucial for inferring the degree of reinforcement protection by the cover of concrete due to the progressive penetration of water inside the concrete by capillary action (Aïssoun, Khayat, and Gallias Citation2016). Moreover, the transport of ions through the microstructure of concrete highly depends on its electrical resistivity. It also plays a dominant role to control the durability of concrete. As the electrical current is conveyed by ions that flow through the concrete pore solution, it can be a good indicator of concrete pore structures (Azarsa and Gupta Citation2017; Sengul Citation2014).
In this regard, this study aims to address the gap in the literature regarding the sorptivity and electrical resistivity of concrete containing BPW by conducting long-term capillary water absorption and sorptivity as well as electrical resistivity tests along with introducing new models for engineering purposes. It also paves the way towards a more sustainable construction industry, more durable concrete, and safe disposal of BPW.
2- Experimental Programme
2-1 Materials
2-1-1 Biomedical Polymeric Waste (BPW)
The disinfected BPW has been provided by Fresenius Medical Care Australia (Fresenius Medical Care Australia Citation2020) from dialysis treatment. illustrates the disinfection process by the steam steriliser machine. The waste is sterilised under high pressure saturated steam, helping the heat to kill the microorganisms. The waste is shredded after sterilisation and then is dried by compressed air.
For This study, the disinfected waste was delicately inspected, and hollow filter fibres have been removed because of their low strength by sieving and vacuuming. The purified BPW was then separated into three groups of Soft (polypropylene, low-density polyethylene, nylon, and silicon), Hard (polyvinyl chloride, high-density polyethylene), and Hybrid (an equivalent combination of hard and soft BPW). The sieve analysis based on the AS 1141.11.1 (Methods for sampling and testing aggregates Citation2009) was conducted on all groups. depict the results of sieve analysis and a general view of shapes (rounded, irregular, elongated, and flaky) and dimensions of BPW, respectively.
2-1-2 Natural Aggregate
The single size 14 mm crushed basalt aggregate, provided from Anakie quarry, Victoria, Australia, with particle density (SSD) of 2670 kg/m3 used to keep and help the uniformity of the concrete mixtures after adding BPW. The sand with the particle density (SSD) of 2590 kg/m3 was also used. All the aggregate selections were conducted considering the AS2758.1 and AS1141 (Methods for sampling and testing aggregates Citation2009; Standards Australia Citation2009) standards ( and ).
Table 1. Natural aggregate properties
2.1.3. Cement
The Eureka general-purpose (GP) Portland cement, according to the AS 3972 (General purpose and blended cements Citation2010) with a specific gravity of 3150 kg/m3 was used in this study ().
Table 2. Eureka general-purpose (GP) Portland cement composition (Independent Cement and Lime Pty Ltd Citation2020)
2-1-4 Mix Design
The concrete mixtures were designed according to the ACI-211 (ACI Committee 211, Citation1991) method with a water/cement ratio of 0.38 to meet the criteria of the concrete exposed to severe environments. Four percentages of 1.5, 3, 6, and 9 by weight of cement for each of the above mentioned three types of BPW were added to the mixtures ().
Table 3. Concrete mix proportions
Firstly, to cast the concrete, the coarse aggregate and half of the fine aggregate were mixed, and then BPW was added to the existing mixing materials. Then, 20% of water was added to wet the mixing aggregate. Afterwards, the other half of the fine aggregate and the cement were added. Finally, the remained water was added.
2-2 Test Methods
Thirteen mixtures (including 4 BPW Percentages × 3 combinations + 1 control) were cast and moulded in 52 of 100 × 200 mm cylindrical specimens and then cured in freshwater for 28 days based on the Methods of testing concrete Citation2014c). Afterwards, the slump test was carried out following Methods of testing concrete Citation2014b). The strength tests were also conducted by employing the GEO-CON compression loading frame with the maximum capacity of 3000 152 kN [64] and according to Methods of testing concrete Citation1998), Methods of testing concrete Citation2014a), AS 1012.10–2000 (AS Citation1012.10-2000 (R2014) 2014), and AS 1012.17–1997 (AS Citation1012.17 – 1997 2014) standards (). Capillary water absorption and electrical resistivity tests also were conducted according to ASTM C1585-13 (ASTM C1585 – 13, Citation2013) and ASTM G57 – 06 (ASTM G57 – 06, Citation2012). Finally, new regression-based relationships between the ER, water sorptivity, and BPW percentage were introduced for better engineering interpretations.
2-2-1 Capillary Water Absorption and Sorptivity
To conduct the capillary water absorption and sorptivity tests, concrete discs with 100 ± 6 mm diameter and 50 ± 3 mm height were prepared by cutting 100 × 200 mm cylindrical specimens. Before the start of the test procedure and for conditioning the samples, they were placed in the environmental chamber at a temperature of 50 ± 2°C and relative humidity of 80 ± 3 % for 3 days. Afterwards, they were placed in a desiccator inside an oven at a temperature of 50 ± 2°C for 3 days. Concrete specimens were then placed inside a sealable container and stored at 23 ± 2°C for 15 days to equilibrate the moisture distribution within the test specimens. The side surface of the discs was then sealed with duct tape, and the end of the discs was sealed by a loosely attached plastic sheet. The absorption test was then conducted at 23 ± 2°C by placing the discs on the supports placed in the containers with a tap water level of 1 to 3 mm. The weights of the discs were measured at different intervals based on the ASTM C1585-13 (ASTM C1585 – 13, Citation2013) standard. The standard test procedure is in two intervals of 6 hours (Initial) and 8 days (Secondary). For understanding more about the long-term sorptivity, the test was continued for 156 days. shows the concrete discs and the test apparatus.
2-2-2 Electrical Resistivity
Generally, measuring the electrical resistivity of concrete is based on imposing a voltage between two or more electrodes and recording the current or vice versa. Afterwards, employing a cell constant, depending on the electrodes’ configuration, to convert the recorded electrical resistance to electrical resistivity or R (Ω) to ρ (Ωm). Both alternating current (AC) and direct current (DC) can be employed to measure concrete’s electrical resistance. The electrical resistivity test was conducted using a Resipod resistivity metre () on the 100 × 200 mm saturated dry surface cylindrical specimens according to ASTM G57 – 06 (ASTM G57 – 06, Citation2012).
Figure 7. Apparatus for electrical resistivity measurement (PCTE, Citation2021)
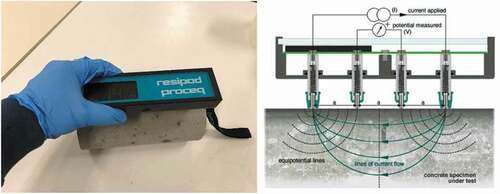
3- Results and Discussion
3-1 Workability and Mechanical Properties
The Workability and mechanical properties of BPW contained concrete are mentioned in . Generally, it is evident that adding BPW leads to a decrease in mechanical properties and the workability of concrete. However, the decrement can be neglected at lower percentages of BPW addition.
Table 4. Workability and mechanical properties of BPW contained concrete
3-2 Electrical Resistivity
The variation of electrical resistivity ratios of BPW contained concrete to the control concrete versus different added BPW percentages is shown in .
Figure 8. The electrical resistivity ratio of BPW contained concrete to control concrete versus BPW percentages
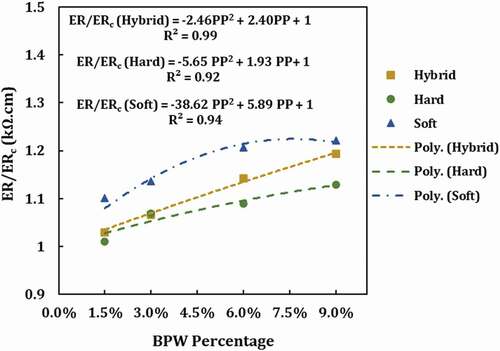
Clearly can be seen that the addition of BPW leads to an increase in the electrical resistivity of concrete which the most variation can be attributed to the soft BPW addition is followed by Hybrid and Hard BPW additions. Moreover, the introduced relationships are not only dependent on the BPW percentages but also strongly dependent on the type of added BPW.
Variations of compressive strength ratios versus the electrical resistivity ratios for BPW contained concrete to control concrete is also shown in . An increase in electrical resistivity accompanies the decrease in compressive strength. It is well proved that water-saturated concrete’s electrical resistivity depends on the microstructural properties of concrete, namely pore connectivity, pore solution, porosity, and tortuosity of pores (Liu, Presuel-Moreno, and Paredes Citation2015). Hence, the increase in the electrical resistivity may be due to less pore connectivity and more tortuosity of pores. However, the compressive strength of the concrete is decreased by adding BPW due to a weaker ITZ between BPW and cement paste (He et al. Citation2020; Rahimireskati et al. Citation2021; L. Xu, Deng, and Chi Citation2017).
3-3 Capillary Water Absorption and Sorptivity
The capillary water absorption test is a method to measure the capacity of concrete to absorb water through the capillaries due to the pressure gradient between the air and the water surface in the capillaries. This parameter also is among the most relevant factors to determine the durability performance of the concrete subjected to aggressive environments (Hilsdorf & Kropp Citation1995). The conducted capillary water absorption test results on concrete containing different percentages of Hybrid, Hard, and Soft BPW are shown in , respectively. According to the figures, concretes containing Hybrid and Hard BPW show the less absorbed volume of water and show a less sorptivity (the slope of the graph) or sorptivity compared to the control concrete. It is also notable to say that concrete containing Soft BPW has more sorptivity, although the concrete containing 1.5% of BPW absorption is slightly lower than the control concrete.
Furthermore, the averaged sorptivity values of BPW contained concrete versus different types and percentages of BPW addition are shown in . Clearly can be seen that Hybrid BPW addition, results in a lower sorptivity compared to control and other BPW contained mixtures. However, soft BPW addition leads to an increase in the water sorptivity of concrete. Generally, The lower sorptivity of Hybrid and hard BPW contained concrete could be attributed to, first, the hydrophobic nature of the BPW, reducing the imbibition of water (Courard, Zhao, and Michel Citation2020; Hannawi, Kamali-Bernard, and Prince Citation2010). Secondly, adding the BPW could change the pore structure and reduce the inner connectivity of the pores of the concrete matrix (Afroughsabet et al., Citation2018; D. S. Babu, Babu, and Tiong-Huan Citation2006). In contrast, the addition of soft BPW could increase the porosity and result in higher absorption (Mohammadhosseini and Tahir Citation2018).
Figure 13. The sorptivity of concrete containing different types and percentages of 1.5% (a), 3% (b), 6% (c), and 9% (d) BPW
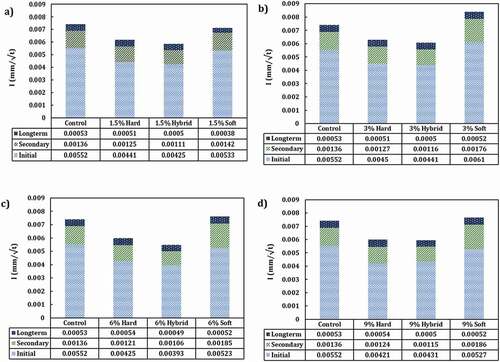
illustrates the mass change of saturated concrete containing BPW compared to their oven-dried condition. The lowest change can be attributed to the 6% Hybrid mixture followed by 1.5% BPW stating the less pore volume of the mixtures mentioned above (Kurda, De Brito, and Silvestre Citation2019).
Figure 14. Mass change of concrete specimens containing different types and percentages of BPW after saturation
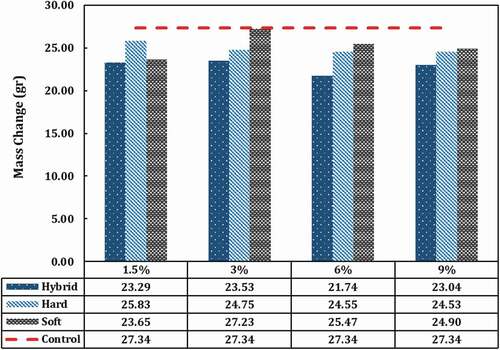
Besides, Due to the difficulty and time-consuming procedure of capillary water absorption test and easier electrical resistivity test procedure, three equations, based on the test results, can be proposed for engineering purposes. EquationEq.1Eq.1
Eq.1 , EquationEq.2
Eq.2
Eq.2 , and EquationEq.3
Eq.3
Eq.3 are regarding the initial, secondary, and long-term sorptivity of concrete containing BPW, respectively.
where
is the ratio of initial sorptivity of BPW contained concrete and control concrete.
is the ratio of secondary sorptivity of BPW contained concrete and control concrete.
is the ratio of long-term sorptivity of BPW contained concrete and control concrete.
is the ratio of 28-day electrical resistivity of BPW contained concrete and control concrete.
PP is the BPW percentage.
Considering the fact that concretes containing different types of BPW behave differently and in order to break down the error and to reach more accurate equations, correction factors calculated based on regression analysis for concrete containing Hybrid, Hard, and Soft BPW at all initial, secondary, and long-term periods as, respectively, shown in . It is also notable to say that as the long-term sorptivity does not linearly change with time, it is calculated from the parallel line’s slope at each measuring point.
shows the experimental versus predicted sorptivity values after applying the correcting factors. It is evident that the accuracy of predicted values improved for both initial and secondary periods.
Figure 18. Experimental versus predicted sorptivity values after applying the correcting factors ((a): Initial, (b): Secondary, and (c): Long-term)
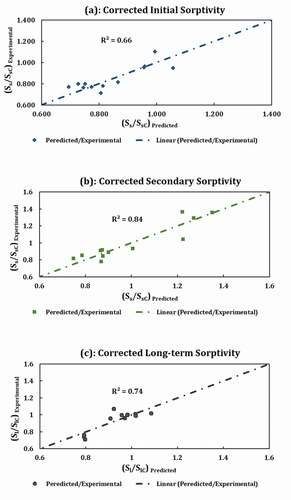
The results are also tabulated in .
Table 5. Initial sorptivity values
Table 6. Secondary sorptivity values
Table 7. Long-term sorptivity values
4- Conclusion
In order to pave the way towards utilising BPW in structural concrete as a sustainable way for biomedical waste disposal, water transport, and electrical resistivity tests were conducted on concrete containing Hybrid, Hard, and Soft BPW as additive materials. Afterwards, Predictive models were then introduced, taking advantage of electrical resistivity to predict the long-term sorptivity and capillary absorption of the concrete and address the current lack of knowledge in the literature.
The main findings and highlights of the results of this study are as follows:
The electrical resistivity is improved by adding all three types of Hybrid, Hard, and Soft BPW of up to 19.3%, 12.9%, and 22.1%, respectively, compared with the control concrete. It also follows an indirect trend with the compressive strength, which is related to more tortuosity of pores structure of the concrete matrix after BWP addition (higher resistivity) and more porous ITZ between BPW and cement paste (lower compressive strength).
Hybrid, Hard, and Soft BPW as additives to concrete cause the sorptivity to improve, respectively, by up to 28%, 23%, and 5.3% for initial and secondary periods comparing with the control concrete, attributing to the hydrophobic nature of the BPW and lower inner connectivity of pores of the concrete matrix.
The sorptivity follows a linear trend in the initial and secondary stages and then follows a logarithmic pattern in the long-term.
The lowest mass change of BPW contained concrete from the oven-dried stage to the saturated stage can be attributed to the 6% followed by 1.5% Hybrid BPW mixture.
Considering the difficulties and time-consuming procedure of the absorption and sorptivity tests, predictive models also proposed to predict the sorptivity of BPW contained concrete in three time periods of initial, secondary, and long-term by taking advantage of the electrical resistivity and the BPW percentage. Correction coefficients also obtained for breaking down the tolerance and reaching models with excellent accuracy.
Given that Hybrid BPW contained high strength concrete shows the lowest absorption and reasonably better resistivity than the control concrete, it reveals that Hybrid BPW can improve the durability of concrete without the need for separating the BPW, saving time and costs.
It is also recommended for future studies to investigate the durability of the concrete containing BPW in different harsh and aggressive environments along with utilising other industrial wastes as substitution for cement.
conflicts of interest
No potential conflict of interest was reported by the author(s).
Acknowledgments
Authors gratefully acknowledge Prof. John Agar (Barwon Health), Dr Katherine Barraclough (Melbourne Health), Mr Richard Knight (Barwon Health), Dr Susan Blair, and Fresenius Medical Care support for providing the BPW. We also acknowledge Deakin University’s support and assistance of the Structures laboratory technicians.
Correction Statement
This article has been republished with minor changes. These changes do not impact the academic content of the article.
Additional information
Notes on contributors
Saman Rahimireskati
Saman Rahimireskati is a Civil Structural, Water & Wastwater Engineer and PhD by research student at Deakin University. Along with working on different construction projects, he acted as a researcher at various top academic and industrial research centres and researched experimentally and analytically on innovative green construction materials, advanced sustainable concrete composites manufactured from waste, and the application of artificial intelligence in structural design.
Kazem Ghabraie
Kazem is an expert in topology and structural optimisation. His research interests also include using sustainable and recycled material in construction. Before joining Deakin in 2016, he was a Lecturer at USQ. He has also taught at RMIT and Victoria University. He completed his undergraduate and master's degree in Civil Engineering at the University of Tehran, Iran and obtained a PhD in civil engineering from RMIT, Melbourne in 2009.
Estela Oliari Garcez
Estela is a Civil Engineer with interest in cementitious composites, and reinforced concrete research. Her principal areas of expertise are advanced materials characterization and microstructure analysis applied to the development of sustainable and durable infrastructure materials. Estela has worked and published in the field of fibre reinforced concrete, development and optimization of high ductility cementitious composites, Reitveld refinement of powder X-ray diffraction of cements, and others Estela has previously worked as an Assistant Professor at University of Pelotas, Brazil from 2009 to 2016.
Riyadh Al-Ameri
Riyadh obtained his PhD in structural engineering from Cardiff University (UK) and contributed to the theoretical development of steel-concrete composite structures. Before joining Deakin University in 2010, Riyadh has engaged for five years in a senior role with the construction industry within the design and consultation space during which a professional international reputation has been established. Riyadh has worked as an academic in various universities internationally including the University of Baghdad and the University of Technology in Iraq. Riyadh expanded his research focus in collaboration with industry partners to cover innovative structural systems such as composite slim floors, cellular structures, and affordable housing. In his research, Riyadh is focusing on the durability of concrete and composite structures and investigates the steel/concrete corrosion in the harsh and marine environment. He also investigates sustainable construction materials and techniques aiming to extend the service life of new and existing structures, while incorporating various waste and industry by-products in concrete, such as epoxy treated chopped carbon fibres, waste plastic, waste glass, and fly ash.
References
- ACI Committee 211. (1991). ACI 211.1-91: Standard Practice for Selecting Proportions for Normal, Heavyweight, and Mass Concrete (Reapproved 2009). https://www.concrete.org/store/productdetail.aspx?ItemID=211191
- Afroughsabet, V., L. Biolzi, and P. J. M. Monteiro. 2018. “The Effect of Steel and Polypropylene Fibers on the Chloride Diffusivity and Drying Shrinkage of High-strength Concrete.” Composites Part B: Engineering 139: 84–96. doi:https://doi.org/10.1016/j.compositesb.2017.11.047.
- Agar, J. W. M. 2019. “Dialysis and the Environment: Seeking a More Sustainable Future.” Artificial Organs 43 (12): 1123–1129. doi:https://doi.org/10.1111/aor.13585.
- Aïssoun, B., K. Khayat, and J.-L. Gallias. 2016. “Variations of Sorptivity with Rheological Properties of Concrete Cover in Self-consolidating Concrete.” Construction and Building Materials 113: 113–120. doi:https://doi.org/10.1016/j.conbuildmat.2016.03.006.
- Albano, C., N. Camacho, M. Hernandez, A. Matheus, and A. Gutierrez. 2009. “Influence of Content and Particle Size of Waste Pet Bottles on Concrete Behavior at Different W/c Ratios.” Waste Management 29 (10): 2707–2716. doi:https://doi.org/10.1016/j.wasman.2009.05.007.
- Alderete, N. M., Y. A. V. Zaccardi, and N. De Belie. 2020. “Mechanism of Long-term Capillary Water Uptake in Cementitious Materials.” Cement and Concrete Composites 106: 103448. doi:https://doi.org/10.1016/j.cemconcomp.2019.103448.
- Al-Tayeb, M., M., . M. Zeyad, A. Dawoud, and B. A. Tayeh. 2020. “Experimental and Numerical Investigations of the Influence of Partial Replacement of Coarse Aggregates by Plastic Waste on the Impact Load.” International Journal of Sustainable Engineering 1–8. doi:https://doi.org/10.1080/19397038.2020.1774820.
- AS 1012.12.1. 1998. Methods of testing concrete - Determination of mass per unit volume of hardened concrete - Rapid measuring method. Australian Standard
- AS 1012.9:2014. 2014a. Methods of testing concrete - Compressive strength tests - Concrete, mortar and grout specimens. Australian Standard
- AS 1012.3.1:2014. 2014b. Methods of testing concrete - Determination of properties related to the consistency of concrete - Slump test. Australian Standard
- AS 1012.8.1:2014. 2014c. Methods of testing concrete - Method for making and curing concrete - Compression and indirect tensile test specimens. Australian Standard
- AS 1012.10-2000 (R2014). (2014). Methods of testing concrete Determination of indirect tensile strength of concrete cylinders (“Brasil” or splitting test). Standards Australia.
- AS 1012.17—1997. (2014). Determination of the static chord modulus of elasticity and Poisson’s ratio of concrete specimens. Standards Australia.
- ASTM C1585-13. (2013). Standard Test Method for Measurement of Rate of Absorption of Water by Hydraulic-Cement Concretes.
- ASTM G57-06. (2012). Standard Test Method for Field Measurement of Soil Resistivity Using the Wenner Four-Electrode Method.
- AS 2758. 2009. Aggregates and rock for engineering purposes Concrete aggregates. Standards Australia.
- Azarsa, P., and R. Gupta. 2017. “Electrical Resistivity of Concrete for Durability Evaluation: A Review.” Advances in Materials Science and Engineering, 2017. doi:https://doi.org/10.1155/2017/8453095
- Babu, D. S., K. G. Babu, and W. Tiong-Huan. 2006. “Effect of Polystyrene Aggregate Size on Strength and Moisture Migration Characteristics of Lightweight Concrete.” Cement and Concrete Composites 28 (6): 520–527. doi:https://doi.org/10.1016/j.cemconcomp.2006.02.018.
- Babu, K. G., and D. S. Babu. 2004. “Performance of Fly Ash Concretes Containing Lightweight EPS Aggregates.” Cement and Concrete Composites 26 (6): 605–611. doi:https://doi.org/10.1016/S0958-9465(03)00034-9.
- Bai, J., S. Wild, and B. B. Sabir. 2002. “Sorptivity and Strength of Air-cured and Water-cured PC–PFA–MK Concrete and the Influence of Binder Composition on Carbonation Depth.” Cement and Concrete Research 32 (11): 1813–1821. doi:https://doi.org/10.1016/S0008-8846(02)00872-4.
- Barnes, D. K. A., F. Galgani, R. C. Thompson, and M. Barlaz. 2009. “Accumulation and Fragmentation of Plastic Debris in Global Environments.” Philosophical Transactions of the Royal Society B: Biological Sciences 364 (1526): 1985–1998. doi:https://doi.org/10.1098/rstb.2008.0205.
- Cantero, B., I. F. S. Del Bosque, A. Matías, M. I. S. De Rojas, and C. Medina. 2020. “Water Transport Mechanisms in Concretes Bearing Mixed Recycled Aggregates.” Cement and Concrete Composites 107: 103486. doi:https://doi.org/10.1016/j.cemconcomp.2019.103486.
- Choi, Y. W., D. J. Moon, Y. J. Kim, and M. Lachemi. 2009. “Characteristics of Mortar and Concrete Containing Fine Aggregate Manufactured from Recycled Waste Polyethylene Terephthalate Bottles.” Construction and Building Materials 23 (8): 2829–2835. doi:https://doi.org/10.1016/j.conbuildmat.2009.02.036.
- Courard, L., Z. Zhao, and F. Michel. 2020. “Influence of Hydrophobic Product Nature and Concentration on Carbonation Resistance of Cultural Heritage Concrete Buildings.” Cement and Concrete Composites 115: 103860. doi:https://doi.org/10.1016/j.cemconcomp.2020.103860.
- Fraj,, A. Ben, M. Kismi, and P. Mounanga. 2010. “Valorization of Coarse Rigid Polyurethane Foam Waste in Lightweight Aggregate Concrete.” Construction and Building Materials 24 (6): 1069–1077. doi:https://doi.org/10.1016/j.conbuildmat.2009.11.010.
- Fresenius Medical Care Australia. (2020). https://www.fmc-au.com/
- General purpose and blended cements. (2010). AS 3972-2010. Australian Standard
- Golewski, G. L. 2020a. “Changes in the Fracture Toughness under Mode II Loading of Low Calcium Fly Ash (LCFA) Concrete Depending on Ages.” Materials 13 (22): 5241. doi:https://doi.org/10.3390/ma13225241.
- Golewski, G. L. 2020b. “Energy Savings Associated with the Use of Fly Ash and Nanoadditives in the Cement Composition.” Energies 13 (9): 2184. doi:https://doi.org/10.3390/en13092184.
- Golewski, G. L. 2021. “The Beneficial Effect of the Addition of Fly Ash on Reduction of the Size of Microcracks in the ITZ of Concrete Composites under Dynamic Loading.” Energies 14 (3): 668. doi:https://doi.org/10.3390/en14030668.
- Gu, L., and T. Ozbakkaloglu. 2016. “Use of Recycled Plastics in Concrete: A Critical Review.” Waste Management 51: 19–42. doi:https://doi.org/10.1016/j.wasman.2016.03.005.
- Hannawi, K., S. Kamali-Bernard, and W. Prince. 2010. “Physical and Mechanical Properties of Mortars Containing PET and PC Waste Aggregates.” Waste Management (New York, N.Y.) 30 (11): 2312–2320. doi:https://doi.org/10.1016/j.wasman.2010.03.028.
- He, W., X. Kong, Y. Fu, C. Zhou, and Z. Zheng. 2020. “Experimental Investigation on the Mechanical Properties and Microstructure of Hybrid Fiber Reinforced Recycled Aggregate Concrete.” Construction and Building Materials 261: 120488. doi:https://doi.org/10.1016/j.conbuildmat.2020.120488.
- Hilsdorf, H. & Kropp, J. (1995). Performance criteria for concrete durability. In CRC Press. E & FN Spon, London.
- Independent Cement and Lime Pty Ltd. 2020. EUREKA GP CEMENT®.. https://www.independentcement.com.au/product/eureka-gp-cement/
- Jacob-Vaillancourt, C., and L. Sorelli. 2018. “Characterization of Concrete Composites with Recycled Plastic Aggregates from Postconsumer Material Streams.” Construction and Building Materials 182: 561–572. doi:https://doi.org/10.1016/j.conbuildmat.2018.06.083.
- Jang, Y.-C., C. Lee, O.-S. Yoon, and H. Kim. 2006. “Medical Waste Management in Korea.” Journal of Environmental Management 80 (2): 107–115. doi:https://doi.org/10.1016/j.jenvman.2005.08.018.
- Klangsin, P., and A. K. Harding. 1998. “Medical Waste Treatment and Disposal Methods Used by Hospitals in Oregon, Washington, and Idaho.” Journal of the Air & Waste Management Association 48 (6): 516–526. doi:https://doi.org/10.1080/10473289.1998.10463706.
- Koushkbaghi, M., P. Alipour, B. Tahmouresi, E. Mohseni, A. Saradar, and P. K. Sarker. 2019. “Influence of Different Monomer Ratios and Recycled Concrete Aggregate on Mechanical Properties and Durability of Geopolymer Concretes.” Construction and Building Materials 205: 519–528. doi:https://doi.org/10.1016/j.conbuildmat.2019.01.174.
- Kurda, R., J. De Brito, and J. D. Silvestre. 2019. “Water Absorption and Electrical Resistivity of Concrete with Recycled Concrete Aggregates and Fly Ash.” Cement and Concrete Composites 95: 169–182. doi:https://doi.org/10.1016/j.cemconcomp.2018.10.004.
- Li, Q., K. Yang, and C. Yang. 2019. “An Alternative Admixture to Reduce Sorptivity of Alkali-activated Slag Cement by Optimising Pore Structure and Introducing Hydrophobic Film.” Cement and Concrete Composites 95: 183–192. doi:https://doi.org/10.1016/j.cemconcomp.2018.11.004.
- Liu, Y., F. J. Presuel-Moreno, and M. A. Paredes. 2015. “Determination of Chloride Diffusion Coefficients in Concrete by Electrical Resistivity Method.” ACI Materials Journal 112 (5): 5. doi:https://doi.org/10.14359/51687777.
- Marzouk, O. Y., R. M. Dheilly, and M. Queneudec. 2007. “Valorization of Post-consumer Waste Plastic in Cementitious Concrete Composites.” Waste Management (New York, N.Y.) 27 (2): 310–318. doi:https://doi.org/10.1016/j.wasman.2006.03.012.
- Methods for sampling and testing aggregates. (2009). AS 1141-2009. Standards Australia.
- Mohammadhosseini, H., and M. M. Tahir. 2018. “Durability Performance of Concrete Incorporating Waste Metalized Plastic Fibres and Palm Oil Fuel Ash.” Construction and Building Materials 180: 92–102. doi:https://doi.org/10.1016/j.conbuildmat.2018.05.282.
- Nematzadeh, M., M. Tayebi, and H. Samadvand. 2021. “Prediction of Ultrasonic Pulse Velocity in Steel Fiber-reinforced Concrete Containing Nylon Granule and Natural Zeolite after Exposure to Elevated Temperatures.” Construction and Building Materials 273: 121958. doi:https://doi.org/10.1016/j.conbuildmat.2020.121958.
- PCTE. (2021). “Concrete Resistivity Meter”. https://www.pcte.com.au/resipod-resistivity-meter
- Piccoli, G. B., M. Nazha, M. Ferraresi, F. N. Vigotti, A. Pereno, and S. Barbero. 2015. “Eco-dialysis: The Financial and Ecological Costs of Dialysis Waste Products: Is a ‘Cradle-to-cradle’model Feasible for Planet-friendly Haemodialysis Waste Management?” Nephrology Dialysis Transplantation 30 (6): 1018–1027. doi:https://doi.org/10.1093/ndt/gfv031.
- Rahimireskati, S., K. Ghabraie, E. O. Garcez, and R. Al-Ameri. 2021. “Prediction of the Mechanical Performance of High-Strength Concrete Containing Biomedical Polymeric Waste Obtained from Dialysis Treatment.” Applied Sciences 11 (5): 2053. doi:https://doi.org/10.3390/app11052053.
- Saikia, N., and J. Brito. 2013. “Waste Polyethylene Terephthalate as an Aggregate in Concrete.” Materials Research 16 (2): 341–350. doi:https://doi.org/10.1590/S1516-14392013005000017.
- Schecter, A., L. Birnbaum, J. J. Ryan, and J. D. Constable. 2006. “Dioxins: An Overview.” Environmental Research 101 (3): 419–428. doi:https://doi.org/10.1016/j.envres.2005.12.003.
- Seco, A., A. M. Echeverría, S. Marcelino, B. García, and S. Espuelas. 2020. “Durability of Polyester Polymer Concretes Based on Metallurgical Wastes for the Manufacture of Construction and Building Products.” Construction and Building Materials 240: 117907. doi:https://doi.org/10.1016/j.conbuildmat.2019.117907.
- Sengul, O. 2014. “Use of Electrical Resistivity as an Indicator for Durability.” Construction and Building Materials 73: 434–441. doi:https://doi.org/10.1016/j.conbuildmat.2014.09.077.
- Shadmani, A., B. Tahmouresi, A. Saradar, and E. Mohseni. 2018. “Durability and Microstructure Properties of SBR-modified Concrete Containing Recycled Asphalt Pavement.” Construction and Building Materials 185: 380–390. doi:https://doi.org/10.1016/j.conbuildmat.2018.07.080.
- Silva, R. V., J. De Brito, and N. Saikia. 2013. “Influence of Curing Conditions on the Durability-related Performance of Concrete Made with Selected Plastic Waste Aggregates.” Cement and Concrete Composites 35 (1): 23–31. doi:https://doi.org/10.1016/j.cemconcomp.2012.08.017.
- Şimşek, B., T. Uygunoğlu, H. Korucu, and M. M. Kocakerim. 2018. “Analysis of the Effects of Dioctyl Terephthalate Obtained from Polyethylene Terephthalate Wastes on Concrete Mortar: A Response Surface Methodology Based Desirability Function Approach Application.” Journal of Cleaner Production 170: 437–445. doi:https://doi.org/10.1016/j.jclepro.2017.09.176.
- Stallings, K. A., S. A. Durham, and M. G. Chorzepa. 2019. “Effect of Cement Content and Recycled Rubber Particle Size on the Performance of Rubber-modified Concrete.” International Journal of Sustainable Engineering 12 (3): 189–200. doi:https://doi.org/10.1080/19397038.2018.1505971.
- Szostak, B., and G. L. Golewski. 2020. “Improvement of Strength Parameters of Cement Matrix with the Addition of Siliceous Fly Ash by Using Nanometric CSH Seeds.” Energies 13 (24): 6734. doi:https://doi.org/10.3390/en13246734.
- Tahmouresi, B., M. Koushkbaghi, M. Monazami, M. T. Abbasi, and P. Nemati. 2019. “Experimental and Statistical Analysis of Hybrid-fiber-reinforced Recycled Aggregate Concrete.” Computers and Concrete 24 (3): 193–206.
- Tarrass, F., M. Benjelloun, O. Benjelloun, and T. Bensaha. 2010. “Water Conservation: An Emerging but Vital Issue in Hemodialysis Therapy.” Blood Purification 30 (3): 181–185. doi:https://doi.org/10.1159/000321485.
- Tibbetts, C. M., J. M. Paris, C. C. Ferraro, K. A. Riding, and T. G. Townsend. 2020. “Relating Water Permeability to Electrical Resistivity and Chloride Penetrability of Concrete Containing Different Supplementary Cementitious Materials.” Cement and Concrete Composites 107: 103491. doi:https://doi.org/10.1016/j.cemconcomp.2019.103491.
- Windfeld, E. S., and M. S.-L. Brooks. 2015. “Medical Waste management–A Review.” Journal of Environmental Management 163: 98–108. doi:https://doi.org/10.1016/j.jenvman.2015.08.013.
- Xu, L., F. Deng, and Y. Chi. 2017. “Nano-mechanical Behavior of the Interfacial Transition Zone between Steel-polypropylene Fiber and Cement Paste.” Construction and Building Materials 145: 619–638. doi:https://doi.org/10.1016/j.conbuildmat.2017.04.035.
- Xu, S., M. A. Malik, Z. Qi, B. Huang, Q. Li, and M. Sarkar. 2018. “Influence of the PVA Fibers and SiO 2 NPs on the Structural Properties of Fly Ash Based Sustainable Geopolymer.” Construction and Building Materials 164: 238–245. doi:https://doi.org/10.1016/j.conbuildmat.2017.12.227.