ABSTRACT
Light is a critical environmental cue for plant growth and development. Plants actively monitor surrounding environments by sensing changes in light wavelength and intensity. Therefore, plants have evolved a series of photoreceptors to perceive a broad wavelength range of light. Phytochrome photoreceptors sense red and far-red light, which serves as a major photomorphogenic signal in shoot growth and morphogenesis. Notably, plants also express phytochromes in the roots, obscuring whether and how they perceive light in the soil. We have recently demonstrated that plants directly channel light to the roots through plant body to activate root phytochrome B (phyB). Stem light facilitates the nuclear import of phyB in the roots, and the photoactivated phyB triggers the accumulation of the photomorphogenic regulator ELONGATED HYPOCOTYL 5 in modulating root growth and gravitropism. Optical experiments revealed that red to far-red light is efficiently transduced through plant body. Our findings provide physical and molecular evidence, supporting that photoreceptors expressed in the underground roots directly sense light. We propose that the roots are not a passive organ but a central organ that actively monitors changes in the aboveground environment by perceiving light information from the shoots.
Light is not only an energy source for photosynthesis but also an important signal of monitoring environmental changes in plants.Citation1,2 Light affects virtually all developmental and physiological events in plants, covering from germination, stem and leaf growth, flowering to senescence, and responses to environmental stresses.Citation1,3 A series of photoreceptors that recognizes specific wavelengths of light have been discovered. Ultraviolet B light (UVB) is sensed by UVB-RESISTANCE 8, and blue light is perceived by cryptochromes and phototropins.Citation4-6 The red/far-red light photoreceptor phytochromes have been most extensively studied in plants. In red light, the red light-absorbing form of phytochromes (Pr) is converted to the far-red light-absorbing form (Pfr), which is an active form in transducing light signals to downstream signaling components.Citation7,8 It is well-established that phytochromes recognize red to far-red ratio to modulate plant growth and development.Citation9,10
Light cannot penetrate more than a few millimeters into soil layers, and thus most studies have been focused on the light responses of aboveground organs. Interestingly, plants express phytochromes not only in the shoots but also in the roots.Citation11,12 Histochemical β-glucuronidase (GUS) staining using transgenic plants expressing GUS gene under the control of phytochrome A (phyA) or phyB gene promoter have shown that the phyA and phyB genes are expressed in both light- and dark-grown roots.Citation13 In particular, the phytochrome genes are expressed specifically in the vascular bundles of the roots, raising a question as to the physiological role of root phytochromes.
To elucidate the function of root phytochromes, root phenotypes have been examined in Arabidopsis mutants that are defective in phytochrome or chromophore biosynthesis.Citation12,14-16 It has been reported that the rate of primary root elongation is reduced in phyB mutant but not in phyA mutant.Citation14 The phyB mutant is also defective in auxin transport from the shoots to the roots, resulting in reduced lateral root production.Citation11 Gravitropic responses of the roots are also regulated by phytochromes.Citation17,18 Light-mediated root gravitropism is significantly reduced in phyA phyB double mutant.Citation14 In chromophore-defective mutant, root sensitivity to jasmonic acid is altered,Citation15 suggesting that phytochromes are also involved in phytohormone signaling in the roots. However, most of the previous studies have been performed using light-grown roots, and thus it is still unclear whether root phytochromes are functional in the dark soil.
More than 30 y ago, it has been suggested that light is conducted through the stem and root tissues.Citation19 Recent studies also support the channeling of light through the vascular tissues.Citation20,21 While the direct transduction of light through stems is widely perceived, it has not been explored how the stem-piped light influences root growth and morphology until our recent studies.Citation13 With an aim of elucidating the physiological role of stem-piped light, we employed molecular, photochemical, and physical tools.
We established a controlled plant growth system, in which the shoots or the roots are selectively exposed to light. We performed RNA sequencing analysis using RNA samples extracted from the roots of plants that were treated with light in the controlled growth system. Bioinformatic analysis revealed that a group of light-responsive genes, including those encoding HY5 and its downstream targets CHALCONE SYNTHASE, and PHOTOLYASE 1, were induced in the roots under both stem light and root light conditions. Gene expression assays using the roots of photoreceptor mutants grown under shoot light conditions showed that phyB is responsible for the induction of HY5 gene and its downstream target genes in the roots under shoot light conditions.
We also employed micrografting tools to generate chimeric plants harboring phyB mutation in either the shoots or the roots. Grafting of wild type plants with phyB mutant or transgenic plants expressing a constitutively active phyB revealed that root phyB is necessary for the induction of root genes in response to aboveground light. In addition, chimeric plants having hy5 mutation in the roots exhibited defective root gravitropism, indicating that phyB-mediated sensing of stem-piped light modulates root gravitropism.
It is well known that phyB is nuclear-localized upon exposure to light to facilitate HY5 protein accumulation.Citation22,23 We generated transgenic plants expressing green fluorescent protein (GFP)-fused phyB or HY5 fusions to tract their subcellular localization and accumulation patterns. It was found that exposure of the shoots to light triggers the nuclear import of phyB and accordingly accumulation of HY5 in the nucleus. The phyB nuclear translocation and HY5 accumulation still occurred even when soil-penetrating light is completely blocked, excluding the possibility of direct sensing of light by the roots in triggering the cellular events and suggesting that light is channeled through the stems.
We employed optical methods to directly examine the channeling of light through the stems. Light was applied to the upper part of stem-root segments using optical fiber, and a highly sensitive light detector was positioned at the other end of the stem-root segments. We found that light in the red to near infrared wavelength range was efficiently conducted through the segments. Light in the green to red wavelength range was also detected, although the transduction efficiency was much lower than that of red to near infrared light. In addition, direct application of red and far-red light to the roots rapidly activated phyB and its downstream signals, indicating that stem-piped light activates phyB in the roots.
Collectively, our observations demonstrate that aboveground light is directly transduced through plant body to the roots and activates root phyB in mediating root growth and gravitropism. Meanwhile, it has been suggested that light triggers the synthesis of chemicals and proteins in the shoots, which migrate to the roots.Citation12,24,25 The root-targeted signaling molecules affect root growth and nitrate transport in the roots. It is thus likely that both light and mobile molecules transmit aboveground information to the roots to achieve optimal root growth and development under fluctuating environmental conditions (). It is notable that responses of the roots to the stem-piped light occur earlier than those of mobile molecules,Citation13,24,25 suggesting that light and signaling molecules have different roles in root biology.
Figure 1. Schematic modes of stem-to-root light signal transduction. Aboveground light is either directly transduced via vascular tissues to the roots (mode 1) or triggers the production of signaling molecules, such as mobile chemicals and HY5 transcription factor, which migrate to the roots (mode 2). In mode 1, stem-piped light activates root phyB, which stabilizes HY5. The root phyB-HY5 module mediates root growth and gravitropism. In mode 2, the mobile signaling molecules, including auxin and HY5, move from the shoots to the roots, where they regulate root growth and nitrate uptake.
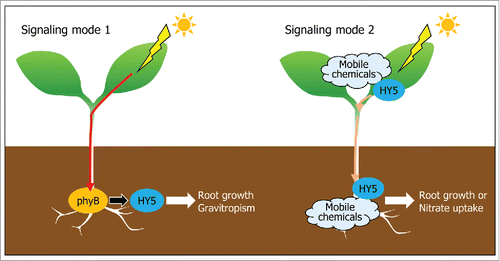
The roots are thought to be a passive organ compare with the shoots. Previous studies have shown that the shoots are major site of photosynthesis, circadian clock function, and perception of environmental cues.Citation2,3,26 Our findings indicate that the roots actively monitor aboveground environment by directly sensing stem-piped light and provide strong evidence, supporting that the roots are not just a passive organ but a central organ in shaping physiological events in response to environmental changes.
While our data support the role of stem-piped light in modulating root growth and gravitropism, it is still unclear how light is conducted through plant body. It has been shown that vascular tissues are effective light conductors in woody and herbaceous plants.Citation20,21 Together with optical properties of water, which efficiently guides light transduction,Citation27 it seems that xylem is a primary route for light transduction. Further works using plants with altered xylem development and architecture would be useful to examine this hypothesis.
Abbreviations
GFP | = | green fluorescent protein |
GUS | = | β-glucuronidase |
HY5 | = | ELONGATED HYPOCOTYL 5 |
Pfr | = | far-red light absorbing form of phytochrome |
phyA | = | phytochrome A |
phyB | = | phytochrome B |
Pr | = | red light absorbing form of phytochrome |
UVB | = | ultraviolet B |
Disclosure of potential conflicts of interest
No potential conflicts of interest were disclosed.
Funding
This work was supported by the Human Frontier Science Program (RGP0002/2012). It was also supported in part by the Leaping Research (NRF-2015R1A2A1A05001636) and Global Research Lab (NRF-2012K1A1A2055546) Programs provided by the National Research Foundation of Korea and the Next-Generation BioGreen 21 Program (Plant Molecular Breeding Center No. PJ0111532016) provided by the Rural Development Administration of Korea.
References
- Jiao Y, Lau OS, Deng XW. Light-regulated transcriptional networks in higher plants. Nat Rev Genet 2007; 8:217-30; PMID:17304247; http://dx.doi.org/10.1038/nrg2049
- Chenu A, Scholes GD. Coherence in energy transfer and photosynthesis. Annu Rev Phys Chem 2015; 66:69-96; PMID:25493715; http://dx.doi.org/10.1146/annurev-physchem-040214-121713
- Galvão VC, Fankhauser C. Sensing the light environment in plants: photoreceptors and early signaling steps. Curr Opin Neurobiol 2015; 34:46-53; PMID:25638281; http://dx.doi.org/10.1016/j.conb.2015.01.013
- Cashmore AR, Jarillo JA, Wu YJ, Liu D. Cryptochromes: blue light receptors for plants and animals. Science 1999; 284:760-5; PMID:10221900; http://dx.doi.org/10.1126/science.284.5415.760
- Christie JM. Phototropin blue-light receptors. Annu Rev Plant Biol 2007; 58:21-45; PMID:17067285; http://dx.doi.org/10.1146/annurev.arplant.58.032806.103951
- Rizzini L, Favory JJ, Cloix C, Faggionato D, O'Hara A, Kaiserli E, Baumeister R, Schäfer E, Nagy F, Jenkins GI, et al. Perception of UV-B by the Arabidopsis UVR8 protein. Science 2011; 332:103-6; PMID:21454788; http://dx.doi.org/10.1126/science.1200660
- Smith H. Phytochromes and light signal perception by plants-an emerging synthesis. Nature 2000; 407:585-91; PMID:11034200; http://dx.doi.org/10.1038/35036500
- Xu X, Paik I, Zhu L, Huq E. Illuminating progress in phytochrome-mediated light signaling pathways. Trends Plant Sci 2015; 20:641-50; PMID:26440433; http://dx.doi.org/10.1016/j.tplants.2015.06.010
- Mathews S. Phytochrome-mediated development in land plants: red light sensing evolves to meet the challenges of changing light environments. Mol Ecol 2006; 15:3483-503; PMID:17032252; http://dx.doi.org/10.1111/j.1365-294X.2006.03051.x
- Franklin KA, Quail PH. Phytochrome functions in Arabidopsis development. J Exp Bot 2010; 61:11-24; PMID:19815685; http://dx.doi.org/10.1093/jxb/erp304
- Sharrock RA, Clack T. Patterns of expression and normalized levels of the five Arabidopsis phytochromes. Plant Physiol 2002; 130:442-56; PMID:12226523; http://dx.doi.org/10.1104/pp.005389
- Salisbury FJ, Hall A, Grierson CS, Halliday KJ. Phytochrome coordinates Arabidopsis shoot and root development. Plant J 2007; 50:429-38; PMID:17419844; http://dx.doi.org/10.1111/j.1365-313X.2007.03059.x
- Lee HJ, Ha JH, Kim SG, Choi HK, Kim ZH, Han YJ, Kim JI, Oh Y, Fragoso V, Shin K, et al. Stem-piped light activates phytochrome B to trigger light responses in Arabidopsis thaliana roots. Sci Signal 2016; 9:ra106; PMID:27803284; http://dx.doi.org/10.1126/scisignal.aaf6530
- Correll MJ, Kiss JZ. The roles of phytochromes in elongation and gravitropism of roots. Plant Cell Physiol 2005; 46:317-23; PMID:15695459; http://dx.doi.org/10.1093/pcp/pci038
- Costigan SE, Warnasooriya SN, Humphries BA, Montgomery BL. Root-localized phytochrome chromophore synthesis is required for photoregulation of root elongation and impacts root sensitivity to jasmonic acid in Arabidopsis. Plant Physiol 2011; 157:1138-50; PMID:21875894; http://dx.doi.org/10.1104/pp.111.184689
- Dyachok J, Zhu L, Liao F, He J, Huq E, Blancaflor EB. SCAR mediates light-induced root elongation in Arabidopsis through photoreceptors and proteasomes. Plant Cell 2011; 23:3610-26; PMID:21972261; http://dx.doi.org/10.1105/tpc.111.088823
- Mandoli DF, Tepperman J, Huala E, Briggs WR. Photobiology of diagravitropic maize roots. Plant Physiol 1984; 75:359-63; PMID:16663626; http://dx.doi.org/10.1104/pp.75.2.359
- Feldman LJ, Briggs WR. Light-regulated gravitropism in seedling roots of maize. Plant Physiol 1987; 83:241-3; PMID:11539030; http://dx.doi.org/10.1104/pp.83.2.241
- Mandoli DF, Briggs WR. Optical properties of etiolated plant tissues. Proc Natl Acad Sci U S A 1982; 79:2902-6; PMID:16593186
- Sun Q, Yoda K, Suzuki M, Suzuki H. Vascular tissue in the stem and roots of woody plants can conduct light. J Exp Bot 2003; 54:1627-35; PMID:12730266; http://dx.doi.org/10.1093/jxb/erg167
- Sun Q, Yoda K, Suzuki H. Internal axial light conduction in the stems and roots of herbaceous plants. J Exp Bot 2005; 56:191-203; PMID:15533879; http://dx.doi.org/10.1093/jxb/eri019
- Kircher S, Kozma-Bognar L, Kim L, Adam E, Harter K, Schafer E, Nagy F. Light quality-dependent nuclear import of the plant photoreceptors phytochrome A and B. Plant Cell 1999; 11:1445-56; PMID:10449579; http://dx.doi.org/10.1105/tpc.11.8.1445
- Osterlund MT, Hardtke CS, Wei N, Deng XW. Targeted destabilization of HY5 during light-regulated development of Arabidopsis. Nature 2000; 405:462-6; PMID:10839542; http://dx.doi.org/10.1038/35013076
- Sassi M, Lu Y, Zhang Y, Wang J, Dhonukshe P, Blilou I, Dai M, Li J, Gong X, Jaillais Y, et al. COP1 mediates the coordination of root and shoot growth by light through modulation of PIN1- and PIN2-dependent auxin transport in Arabidopsis. Development 2012; 139:3402-12; PMID:22912415; http://dx.doi.org/10.1242/dev.078212
- Chen X, Yao Q, Gao X, Jiang C, Harberd NP, Fu X. Shoot-to-root mobile transcription factor HY5 coordinates plant carbon and nitrogen acquisition. Curr Biol 2016; 26:640-6; PMID:26877080; http://dx.doi.org/10.1016/j.cub.2015.12.066
- James AB, Monreal JA, Nimmo GA, Kelly CL, Herzyk P, Jenkins GI, Nimmo HG. The circadian clock in Arabidopsis roots is a simplified slave version of the clock in shoots. Science 2008; 322:1832-5; PMID:19095940; http://dx.doi.org/10.1126/science.1161403
- McCluney R. Color-rendering of daylight from water-filled light pipes. Sol Energ Mat 1990; 21:191-206; http://dx.doi.org/10.1016/0165-1633(90)90053-4