ABSTRACT
Root-knot nematodes possess a major threat to agricultural production of various crops worldwide. The intensive use of chemical nematicides to control plant parasitic nematodes has adverse effects on our environment and human health. Owing to the importance of developing new strategies, an experiment was conducted to reveal the influence of arbuscular mycorrhizal fungus, Rhizoglomus fasciculatum and nematophagous fungus, Paecilomyces lilacinus alone or in combination with various organic amendments such as superphosphate, green and organic manure to control the infection of root-knot, nematode Meloidogyne incognita in a vegetable crop Capsicum annuum. These two fungi along with soil amendments significantly improved plant growth and fruit yield and effectively controlled infection of M. incognita. The dual inoculation of P. lilacinus and R. fasciculatum reduced the number of galls and egg masses, thereby revealing the controlled proliferation of M. incognita infection in C. annuum roots. The beneficial effect of these fungi further increased on supplementation of soil with organic or green manures. Inoculation of C. annuum with these two fungi showed a significant increase in egg parasitization; however, maximum effect was detected on dual inoculation. Amongst the soil amendments, the best response was obtained in case of green manure along with mycorrhizal fungus and P. lilacinus. Present study revealed that nematophagous and AM fungi, in combination with green manure were effective in controlling M. incognita, thus suggesting the use of such agents for biocontrol of plant parasitic nematodes in agriculture.
Introduction
Plant parasitic nematodes, the unseen enemies, are a diverse group of obligate pathogens obtaining nutrition from the cytoplasm of living cells of the host. All major field crops, most vegetables, certain cash crops, ornamental plants and many weeds or grasses are susceptible to one or more species of root-knot nematodes [Citation1]. An estimate of $157 billion has been attributed to the damages occurred by plant parasitic nematodes [Citation2], which is more significant than the damage caused by invasive insects, which is approximately $70 billion [Citation3]. The full extent of worldwide nematode damage is likely to be miscalculated, since growers are often unaware of their presence because the symptoms caused in the plant are often nonspecific [Citation4,Citation5].
Nematodes are usually small soil-borne pathogens feeding on the different parts of a plant via roots, stems, leaves, flowers and seeds, although most species feed on roots [Citation6]. They feed through a protrusible stylet that penetrates the plant cells [Citation4,Citation7]. Based on their feeding habitats, plant-parasitic nematodes are categorized as either ectoparasitic or endoparasitic. Sedentary endoparasitic nematodes consist of the root-knot (Meloidogyne spp.) and cyst nematodes (Heterodera and Globodera spp.), which are the most common nematodes in terms of crop losses [Citation4].
Root-knot nematodes disrupt the normal processes of plant root growth and its soil exploration capacity for both water and nutrients; consequently, the infected plant shows above ground symptoms, such as stunting, yellowing, excessive wilting and reduced yield [Citation8].
So far, several strategies have been developed for the control of nematode in agriculture [Citation9]; however, strategies associated with biocontrol are proposed as a much safer alternative and highly practicable for plant-parasitic nematodes management [Citation10]. Paecilomyces lilacinus has been proven as an effective potential biocontrol agent for root-knot and cyst nematodes under both greenhouse and field conditions [Citation11]. P. lilacinus displays nematophagous capacity through different mechanisms of action, traps several fungi and phytopathogenic bacteria, as well as acts as bio-stimulants [Citation11–13]. P. lilacinus treated plants exhibit reduced root galling with increased plant health and crop yield in the soils infested by root–knot nematodes [Citation14]. Inoculation of plants with P. lilacinus results in the reduction in the hatching of egg masses as well as the final juvenile population of nematodes in soil [Citation15].
Interaction between M. incognita and arbuscular mycorrhizal fungi showed promising results against nematode pathogens, but application of arbuscular mycorrhizal fungi for biocontrol of plant pathogens remains insignificant. Recent literature suggests that the contribution of mycorrhizal fungi through mycorrhiza-mediated enhancement of plant nutrition, competition with the pathogen for resources and space, plant morphological changes and barrier formation, changes in biochemical compounds are related with plant resistance response, alleviation of physical stresses and changes in antagonist and deleterious microbes’ population in the mycorrhizosphere features biological control of root borne plant pathogens [Citation16].
Soil amendments mainly manures, crop residues, composts, oil cakes, ash, animal and human wastes are by products and wastes from agriculture or other activities. The nematicidal effects of some of these soil amendments have been recognized for some time and reviewed extensively [Citation17–18]. Addition of organic amendments to the soil stimulates microbial activity as evidenced by increased population of algae, nematode-trapping fungi, bacteria, microbivores nematodes, and other soil antagonists that destroy plant parasitic nematodes [Citation20]. Proliferation of microorganisms results in increased enzymatic activities of the amended soil. They promote decomposition of residues resulting in the soils of specific compounds that could be nematicidal [Citation8,Citation19].
Several members of Solanaceae including, Capsicum annuum are affected by root-knot nematode attack and severely reducing their yields worldwide. The chemical control of root-knot nematodes has not been effective; therefore, an alternative strategy to overcome the problem of nematodes management could be use of biocontrol agents. Hence, the present study aimed to understand the effect of nematophagous fungus Paecilomyces lilacinus and mycorrhizal fungus, Rhizoglomus fasciculatum alone or in combination along with different soil amendments in the biocontrol of root-knot nematode M. incognita.
Materials and methods
Plants and soil materials
The vegetable crop plant Capsicum annuum var. California Wonder belonging to family Solanaceae was selected as experimental plant and their seeds were procured from NBPGR, Indian Agricultural Research Institute, New Delhi. The garden soil and sand (2:1) was mixed properly, hereafter referred as soil. The soil was air-dried, passed through a 2-mm sieve and analyzed by preparing an extract as suggested by Citation21. The soil had the following properties: loam texture; pH (H2O) 7.5, EC 1.0 (dS/m), Organic C (%) 1.15, Total N (%) 0.49, Available P and K 11.1 and 55 mg kg−1, respectively. Soil was sterilized thoroughly using 0.1% formaldehyde (Excelar, Qualigen) and covered with black polythene for a week and eventually exposed for aeration. The process was repeated twice. Earthenware pots of size 28 cm × 25 cm (4.5 kg capacity) were filled with this sand-soil potting mixture. Seedlings were raised in the same soil. After 4 weeks, five seedlings were transplanted in each pot.
Inoculum production
The pure culture of pathogen M. incognita Kofoid and White was obtained from the Department of Nematology, Indian Agriculture Research Institute, Delhi, maintained and multiplied on the roots of Brinjal cv Pusa purple long. Inoculum was prepared according to the method of Hussey and Barker’s [Citation22] and the eggs of nematodes were isolated from infected roots and made to hatch for producing inoculum [].
Arbuscular Mycorrhizal fungus, Rhizoglomus fasciculatum (Thaxt.) Sieverd., G.A. Silva & Oehl comb. nov. (Syn. Glomus fasciculatum (Thaxt.) Gerd. and Trappe, Rhizophagus fasciculatus (Thaxt.) C. Walker and A. Schüßler) was obtained from our laboratory culture collection, which was isolated from the soil of botanical garden of University of Delhi, India. The soil-based culture of R. fasciculatum was maintained and multiplied on Sorghum bicolor and Trigonella foenum-graecum in garden soil, alternatively. The inoculum consisted of thoroughly mixed rhizosphere soil samples containing spores, hyphae and mycorrhizal root fragments [ b, c & d]. To standardize the number of spores per gram soil, spores were isolated with a wet sieving and decanting method [Citation23] and counted. A 100 g of soil containing 140 AM fungal spores was added to each pot. Non-Mycorrhizal plants received the same quantity of non-inoculated soil to ensure the same microflora.
Paecilomyces lilacinus (Thom.) Samson was maintained and multiplied on Potato Dextrose Agar (PDA) at 26 ± 2°C. For bulk inoculum, nine different agro-based carrier substrates were tried, out of which Chicken feed + Sand (3:1) and Rice bran + rice (2:1) were used in the present investigation. The 100 g of Chicken feed + Sand was placed in each of twenty-five 250 ml Erlenmeyer flasks and autoclaved for 15 min at 121°C, 15 psi. Spore suspension of fungus was prepared by adding sterile distilled water to the 7-days-old culture, and agar surface was gently rubbed to release the fungal spores [ e & f]. The 5 ml of spore suspension was used to inoculate cooled, autoclaved carrier substrate present in each flask. The infested carrier substrate was incubated at 26 ± 2°C for 21 days resulting in a fungal inoculum level of about 4.7 × 108/g of substrate. Chicken feed medium was used as carrier substrate. Similar procedure was employed to inoculate carrier substrate Rice bran + rice and fungal inoculum level of 4.5 × 108/g of substrate was raised. A 20 g of inoculated carrier substrate was added to each pot. Non-fungus treatments received equal amounts of non-inoculated carrier substrate to ensure similar soil conditions.
Figure 1. Eggs of M. incognita with fungal mycelium (a &b); Colonization of R. fasciculatum in the root cortical cells of C. annuum (c) and showing variously shaped intracellular vesicles of R. fasciculatum (d); Isolated egg parasite from rhizosphere of Capsicum annuum (e); Conidiophore of Paecilomyces lilacinus showing conidia in chain(f).
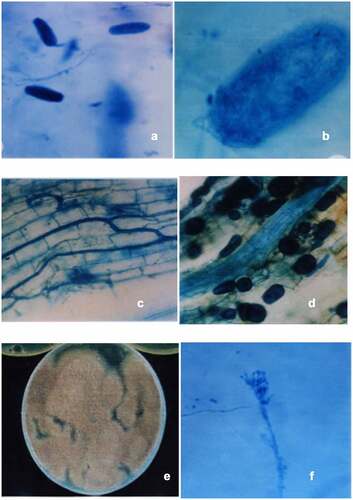
Soil amendments
To evolve an efficient and inexpensive cultural practice (soil amendment) due to its nematicidal properties and not just to reduce nematode menace but also to enhance effectivity of biocontrol agent and to improve the soil health, three different soil amendments were tested in the present study. Their type, application rate and time of application are presented in .
Table 1. Fungal treatments supplemented with different soil amendments
Experimental setup
Experiment with five treatments lasting 60 days (after nematode inoculation) was carried out. Following treatments were employed: (1) Control (C) (2) N (nematode Meloidogyne incognita) (3) N + F (NF, M. incognita with P. lilacinus) (4) N + M(NM, M. incognita with R. fasciculatum) (5) N + M + F (M. incognita (N) + R. fasciculatum (M) + P. lilacinus (F) (NMF), with the following soil amendments (a) Control (Cs, without amendment), (b) Superphosphate (Sp), (c) Organic manure (OM) (commercially prepared containing 0.7% N, 0.15% P and 0.4% K), (d) Green manure (GM) (leaves, and tender shoots were collected and finely chopped, weighed and then added to each pot. NPK composition in % dry weight C 38–40, N 4–9, P 0.69 and K 1.22).
Each treatment was repeated 5 times in a factorial design. Pots were planted with 4-week-old Capsicum annuum seedlings which were allowed to establish for 1 week before nematode juveniles were pipetted with water into holes made around the roots. The soil amendments were incorporated earlier (3 weeks) to transplanting. Two fungi, Paecilomyces lilacinus and Rhizoglomus fasciculatum were added simultaneously during transplantation alone or in combination with nematodes.
Harvest and analysis
Plants were harvested at the end of 60 days (5 replicates per treatment), which potentially allowed for the development of at least two life cycles of nematodes. Plants were dug out carefully, washed to get free of soil without damaging the roots and screened. Root and shoot were measured, and dry weights of shoot and root were taken separately after oven drying when the stable weight was achieved. Mature fruits were harvested and weighed in flushes. Total fruit yield per treatment was calculated.
To assess root knot infection, plant roots were carefully washed under tap water and were stained using sodium hypochlorite acid fuchsin method [Citation24]. Numbers of galls/root system and egg masses/root system were determined by visual observation. Ten egg masses were randomly collected from infected roots and each egg mass was squashed in a drop of 0.01% sodium hypochlorite solution and observed under 45x and quashed 100x. The presence of fungal mycelium in the egg masses was taken as an indicator of confirmed infection. Infected egg masses were examined to estimate the number of eggs destroyed. Presence of fungal hyphae and spores inside eggs and vacuolation of embryos were taken as a measure of egg destruction. Apparently, healthy eggs were also counted. Empty eggs were presumed to be hatched, escaping infection, therefore counted as healthy eggs for the purpose of computing percent eggs parasitized. The percent parasitization was determined according to the formula given by Citation25. Three such readings were taken and average percent parasitization was calculated.
On termination of the experiment, P. lilacinus was isolated from the soil. Soil samples were taken from each treatment with fungus amendment and stored in plastic vials in a refrigerator for a week and air dried at room temperature for 24 h. Stock was prepared by adding 10 g soil to 100 ml sterile distilled water which was further diluted to 103. The 1 ml of aliquot of the dilution was transferred to each of four petri plates followed by 15 ml of cooled P. lilacinus semi-selective medium in each of the petri plate. The petri plates were gently swirled to distribute the sample in the medium. Petri plates were incubated at 25 ± 2°C for 10 days. At harvest, after shoots were cut at the crown, roots were washed carefully under running tap water. Roots were cleared with 10% KOH and stained with 0.1% trypan blue (w/v) in lactophenol as described by [Citation26]. The roots were cut into small segments of 1 cm length. About 100 segments were mounted and observed under stereomicroscope (MAGNUS) to examine mycorrhizal colonization. A root segment was considered to be mycorrhizal, showing presence of mycorrhizal fungal hyphae. Percent mycorrhizal colonization in roots was calculated following Nicolson’s formula, where percent root colonization is equal to the number of segment with mycorrhizal/total number of segments) × 100.
Statistical analysis
Treatment effects were determined by one-way analysis of variance (ANOVA) using a factorial design. Differences between the treatments were confirmed by Duncan’s Multiple range test (DMRT). A significant level of 0.05 was applied.
Results
Fungal colonization
Plant growth parameters were observed at the end of 60 days to examine the effect of different treatments alone or in combination in respect to different soil amendments. Furthermore, the effect of plant parasitic nematode, M. incognita and different soil amendments was studied on the percent colonization of arbuscular mycorrhizal fungus, Rhizoglomus fasciculatum and amount of CFU/g by P. lilacinus [ a & b] in the rhizosphere of C. annuum plants. The highest mycorrhizal colonization was detected in the soil amended with organic and green manure. An opposite trend was observed in the colony formation of P. lilacinus. The colonization of P. lilacinus was higher in the case of control and superphosphate amendments and lower in GM and OM amendments. The effect of dual inoculation was greater to both the cases, which showed that the presence of R. fasciculatum along with P. lilacinus and M. incognita significantly supported both mycorrhizal or fungal colonization.
Effect on growth parameters
In the present study, the influence of mycorrhizal fungus, R. fasciculatum and P. lilacinus was rectilinear on the growth of C. annuum grown in M. incognita infected soil, amended with green manure, organic matter and superphosphate. The inoculation of C. annuum plants with mycorrhizal fungus and P. lilacinus either alone or in combination showed a significant increase in root and shoot length under all types of soil amendments as compared to the uninoculated control plants infected by M. incognita [ & b]. The M. incognita parasitization indeed had a negative impact on plant shoot and root lengths; however, the inoculation with mycorrhizal fungus R. fasciculatum and P. lilacinus significantly lessened the damage caused by nematode, and had a positive impact on root and shoot lengths of plant under all soil amendments. Noteworthy was that plants inoculated with the dual inoculum of both fungi (NMF) had highest root and shoot length and showed up to 35.72% increase over nematode (N) infected plants. Among soil amendments, the best growth of root and shoot length was recorded in case of green manure followed by organic manure and superphosphate; however, the latter showed least biocontrol effect [ & b].
The effect of nematode infection and fungal colonization was observed on the root and shoot fresh weight of C. annuum plants. Both the fungi had a profound effect on the root and shoot fresh weight of C. annuum under all types of soil amendments; however, the amendment of soil with green manure and organic manure showed greater increase in the root and shoot fresh weight than that of the soil amended with superphosphate. Compared to the control set, the fresh weight of root and shoot was higher in case of superphosphate but lower than the GM and OM soil amendments. Root and shoot fresh weight significantly increased in plants inoculated with R. fasciculatum and P. lilacinus alone or in combination. The dual inoculation of both the fungi showed the highest increase in root and shoot fresh weight [].
The deteriorating effect of M. incognita was also observed on root and shoot dry biomass. Root and shoot dry biomass of C. annuum significantly reduced due to M. incognita infection (in case of OM (25.34%) and in case of Sp (66.87%) [] in comparison to the uninfected healthy plants, but this decrease in plant biomass significantly controlled on inoculation of C. annuum plants with R. fasciculatum and P. lilacinus either alone or in combination. The best increment in the root (up to 22.38%) and shoot dry biomass (up to 57.10% Sp) was recorded in case of NMF treated plants in all soil amendments. In all the cases, infected plants inoculated with R. fasciculatum (up to 49.72% for shoot and 13.4% for root) showed better biocontrol effect than P. lilacinus (up to 32.60% for shoot and 3.60% for root) treated plants in terms of dry biomass. This observation significantly varies along all soil amendments, in fact, organic and green manure exhibited greater effect on diminishing the impact of M. incognita as compared to control set or superphosphate soil amendments.
The effect of R. fasciculatum and P. lilacinus inoculation alone or in combination was studied on the number of galls per root system [] formed by parasitic nematodes. The number of galls per root system was significantly reduced by the dual inoculation of C. annuum with R. fasciculatum and P. lilacinus. The R. fasciculatum colonized plants showed significantly similar biocontrol effect to the plants inoculated with P. lilacinus in all soil amendments, except organic manure. Soil with green manure and organic manure had a lower number of galls per root system than the soil with superphosphate, which showed a higher number of galls/root system.
The two fungi exhibited synergistic effect on fruit yield/plant; however, maximum effect was detected on the dual inoculation (NMF) of C. annuum plants grown in green and organic manure supplemented as compared to control set and superphosphate supplemented soils []. The M. incognita-infected plants colonized by R. fasciculatum showed similar biocontrol effect in terms of fruit yield to infected plants inoculated with P. lilacinus.
The M. incognita infection showed a sharp increase in the number of egg masses of nematodes per root system irrespective of all soil amendments; however, the highest egg masses were detected in the control (Cs) set [], which gradually decreased in the case of Sp, OM and GM soil amendments. The number of egg masses significantly reduced when C. annuum plants were treated with combined inoculum of R. fasciculatum and P. lilacinus (NMF). In fact, M. incognita-infected plants colonized with R. fasciculatum alone resulted in a similar number of egg masses, to the infected plants treated with P. lilacinus, thereby showing a similar biocontrol effect.
Egg parasitization was maximum in NMF-treated plants []. The C. annuum plants growing in the green manure amended soil showed higher egg parasitization than that of the other soil amendments, although this difference was insignificant when compared to organic manure soil amendment. There was a very marginal difference in egg parasitization between infected plants colonized with R. fasciculatum and infected plants treated with P. lilacinus alone. However, the NM- and NF-treated plants showed significantly less egg parasitization as compared to the NMF-treated plants.
Figure 2. Effect of different soil amendments and treatments alone or in combinations on the percentage of mycorrhizal colonization (a), amount of CFU per gram X 103 (b) of C. annuum plants infected with M. incognita. Within columns values of each parameter with the same superscript are not significantly different at (P = 0.05) Duncan’s multiple range test (DMRT). Soil amendments, Cs – Control Set, Sp – Superphosphate, GM – Green Manure, OM – Organic Manure, and Soil treatments, C – Control, M – R. fasciculatum, NM – M. incognita with R. fasciculatum, NMF – M. incognita with R. fasciculatum and P. lilacinus.
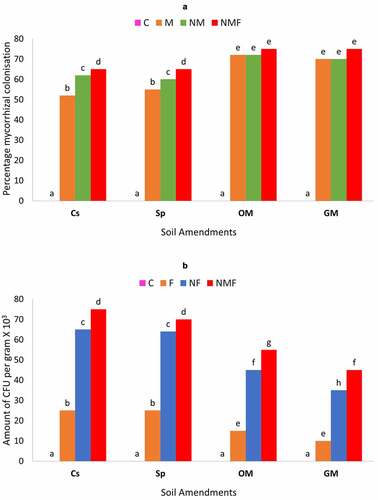
Figure 3. Effect of different treatments and soil amendments alone or in combination on the shoot length (a), root length (b), shoot fresh weight (c), root fresh weight (d), shoot dry weight (e) and root dry weight (f) of C. annuum plants infected with M. incognita. Within columns values of each parameter with the same superscript are not significantly different at (P = 0.05) Duncan’s multiple range test (DMRT). Soil amendments, Cs – Control Set, Sp – Superphosphate, GM – Green Manure, OM – Organic Manure, and Soil treatments, C – Control, N – M. incognita, NF – M. incognita with P. lilacinus, NM – M. incognita with P. lilacinus, NMF – M. incognita with R. fasciculatum and P. lilacinus.
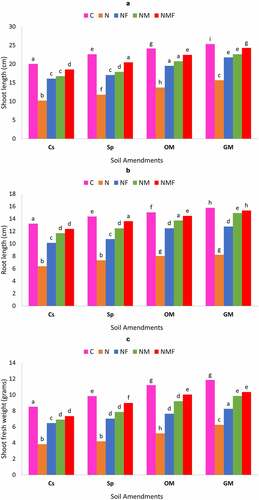
Figure 4. Effect of different treatments and soil amendments alone or in combination on number of galls per root system (a), number of egg masses per root system (b), percent egg parasitization (c) and fruit yield (d) of C. annuum plants infected with M. incognita. Within columns values of each parameter with the same superscript are not significantly different at (P = 0.05) Duncan’s multiple range test (DMRT). Soil amendments, Cs – Control Set, Sp – Superphosphate, GM – Green Manure, OM – Organic Manure, and soil treatments, C – Control, N – M. incognita, NF – M. incognita with P. lilacinus, NM – M. incognita with P. lilacinus, NMF – M. incognita with R. fasciculatum and P. lilacinus.
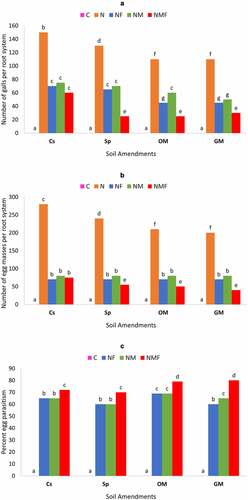
Discussion
Due to their nematophagous properties, Paecilomyces spp. have been used in a variety of biological formulations in agricultural crop protection [Citation27]. Among them, the egg parasite P. lilacinus potentially acts as a nematicidal agent, particularly against M. incognita as it drastically suppresses the growth and multiplication of this root gall forming nematode [Citation28–32,]. The mycorrhizal fungi, which could colonize more than 90% of terrestrial plant species, have also been found to have a profound effect on reducing growth and proliferation of plant pathogens, especially root borne pathogens like M. incognita [Citation16]. In the present study, the egg parasite, P. lilacinus and obligate root symbiont, R. fasciculatum alone or in combination effectively reduced the proliferation of root-knot nematode, M. incognita in the crop plant C. annuum. The individual inoculation of C. annuum with P. lilacinus (NF) remarkably reduced the number of galls and egg masses/root system in M. incognita-infected soil, thereby reducing the plant damage due to M. incognita (N). Results of present experimentation corroborate the findings of previous studies in black pepper and tomato revealing that the reduction in the root-knot population attributes to the fungal attack of larvae, or causing death of females before egg laying, thereby reducing their fecundity and rapid colonization and destruction of eggs formed [Citation33,Citation34]. The characterization of infection-related enzymes in tomato plants further revealed the effectiveness of P. lilacinus in controlling M. incognita [Citation35–38].
An increase in the number of galls and egg masses/root system in the plants amended with superphosphate in comparison to the control set plants suggests a correlation between phosphate and root-knot development and vindicate the assumption that the higher P-level increases root-knot development and egg production thereby enhancing susceptibility of hosts to the nematode attack [Citation39]. In the present study, not much difference was recorded among superphosphate, organic and green manure amendments; indeed, there was significantly lesser number of galls and egg masses/root system in the dual inoculated (NMF) or single inoculation of C. annuum plants with either of the two fungi (NF and NM). The two fungi drastically reduced the number of galls and egg masses of nematodes/root systems in comparison to that of the M. incognita (N) infected plants. Significantly lesser galls/root system in the roots of plants colonized by mycorrhizal fungi indeed concurs with the studies done on wheat and tomato [Citation35,Citation40]. The role of phosphate in mycorrhizal-nematode interaction remains incompletely understood thus requires additional research. The present study suggests that increased superphosphate in soil along with P. lilacinus and R. fasciculatum had a positive role against nematode M. incognita [Citation41].
Inoculation of C. annuum with R. fasciculatum indeed reduced the effect and severity of M. incognita more than that detected in the case of P. lilacinus when used alone, attributing to the lower plant damage. Mycorrhizal symbiosis significantly improved root length and shoot length and their biomass in M. incognita infected soil. The mycorrhiza-mediated alteration in root morphology and increased plant growth under stress conditions is a well-established characteristic phenomenon of arbuscular mycorrhiza, which has been reported in several plants [Citation16,Citation42]. Increased root growth, particularly elongation zone and lateral root formation sites are prone to be penetrated by sedentary parasites that may be due to leakage of root exudate in these areas, however increased root growth in fungi-inoculated M. incognita infested plants did not show susceptibility to nematode infection in the present study. The development and reproduction of nematodes was indeed inhibited in the mycorrhizal roots in comparison to non-mycorrhizal roots, which may be due to a nonspecific defense response [Citation43] and alteration of root morphology and physiology by mycorrhizal symbiosis [Citation44]. Mycoparasitism potential of arbuscular mycorrhizal fungi remains unclear, but indirect effects of mycorrhizal symbiosis on the root parasites have been proposed as well. Mycorrhizal fungi penetrate plant roots through root hairs and make arbuscules and vesicles in the cortical cells and help the host plant in acquiring mineral nutrition from soil, in turn, obtain photosynthate/food from host plant. Similarly, M. incognita also depends for food and shelter on their host plant; therefore, the competition between these heterotrophs for carbon source, space and indeed infection sites could limit M. incognita population growth in the mycorrhizal plants, and seems a potential arbuscular mycorrhiza-mediated biocontrol mechanism [Citation45].
In the present study, different soil amendments were applied and it was observed that the growth of P. lilacinus (in terms of CFU/g) was higher in plants amended with green and organic manure as compared to superphosphate and control sets that underlines the importance of soil amendment and P. lilacinus against root-knot nematode. The performance of P. lilacinus further increased when present in combination with R. fasciculatum (NMF), may be due to synergistic effect of both the fungi, thus controlling the infection and reducing the ability of M. incognita to reproduce in soil, which could further improve with soil amendments [Citation46]. The individual inoculation either with P. lilacinus or R. fasciculatum significantly enhanced the growth of Capsicum plants and suppressed the nematode population in both plant roots and soil in all soil amendments, however, the combined inoculum of two fungi was more effective in the suppression of nematode infection [Citation47].
The inoculation of plants with fungi along with organic matter amendments have shown significant reduction in gall development and reproduction of M. incognita [Citation19]. Present study showed that P. lilacinus proliferates more extensively on nematode damaged roots, may be due to excessive leakage of root exudates from the galled roots, and the presence of egg mass on the root surface. This direct relation between leakage of nutrients from the roots and the ability of the fungus to colonize rhizosphere and rhizoplane has also been studied in tomato [Citation48], which could be a great advantage for preventing the growth and development of plant parasites, thus signifying P. lilacinus as a biocontrol agent [Citation49]. Such a relationship could decrease dependency on other soil factors and therefore increase the reliability of P. lilacinus as a biocontrol agent [Citation8].
Adult female nematodes preparing for egg production require a considerable amount of nutrients, thus competing with the host for the pool of nutrients in the roots. The increased metabolic activity of massive cells of roots on which the nematode feeds stimulates mobilization of photosynthate from shoot to root tissues [Citation50]. The mobilization and accumulation of substances reaches a maximum when the adult females commence egg laying and declines thereafter. In this study, despite comparable colonization of P. lilacinus in the rhizosphere (in terms of CFU/g) of NF- and NMF-treated plants, the percent egg parasitization by P. lilacinus was different. A differential percent egg parasitization between NF and NMF treatments can be substantiated by the above hypothesis. Greater egg parasitization in NMF-treated plants may be due to smaller size gall formation, which in turn could be attributed to the cumulative inhibitory effect of R. fasciculatum and P. lilacinus on the development and reproduction of M. incognita. There are various reports revealing the influence of mycorrhizal fungi on their host plants grow under nematode infested soils [Citation36,Citation39,Citation51]; however, the presence of R. fasciculatum and P. lilacinus alone (NF and NM) did not result in a significant egg parasitization except in case of GM amendment.
In conclusion, the effect of two fungi in association with soil amendments revealed the potential applications of R. fasciculatum and P. lilacinus along with the supplementation of soil with green manure to control the growth and infection of M. incognita, and thereby the damage caused to vegetable crop plant like C. annuum.
Availability of data and materials
All data generated or analyzed during this study are included in this published article.
Author contribution
The authors confirm the contribution to the paper as follows: study conception and design: RR, BG, GS; data collection: RR; analysis and interpretation of results: BG, AS, PM, QSW; draft manuscript preparation: GS; BG, AS, PM, QSW, RR. All authors reviewed the results and approved the final version of the manuscript.
Ethics approval
No specific approval was required for this study.
Acknowledgments
The authors are thankful to the NBPGR and the Department of Nematology, Indian Agricultural Research Institute, New Delhi for providing seeds and M. incognita culture respectively.
Disclosure statement
No potential conflict of interest was reported by the author(s).
References
- Forghani F, Hajihassani A. Recent advances in the development of environmentally benign treatments to control root-knot nematodes. Front Plant Sci. 2020;11:1125.
- Singh S, Singh B, Singh AP. Nematodes: a threat to sustainability of agriculture. Procedia Environ Sci. 2015;29:215–216.
- Bradshaw CJA, Leroy B, Bellard C, et al. Massive yet grossly underestimated global costs of invasive insects. Nat Commun. 2016;7(1):12986.
- Jones TJ, Haegema A, Danchin EG, et al. Top 10 plant-parasitic nematodes in molecular plant pathology. Mol Plant Pathol. 2013;14(9):946–961.
- Siddique S, Grundler FM. Parasitic nematodes manipulate plant development to establish feeding sites. Curr Opin Microbiol. 2018;46:102–108.
- Janati S, Houari A, Wifaya A, et al. Occurrence of the root-knot nematode species in vegetable crops in souss region of Morocco. Plant Pathol J. 2018;34(4):308–315.
- Mejias J, Truong NM, Abad P, et al. Plant proteins and processes targeted by parasitic nematode effectors. Front Plant Sci. 2019;10:970 (1–10).
- Poveda J, Abril-Urias P, Escobar C. Biological control of plant-parasitic nematodes by filamentous fungi inducers of resistance: Trichoderma, Mycorrhizal And Endophytic Fungi. Front Microbiol. 2020;11:992.
- Zhang S, Gan Y, Xu B, et al. The parasitic and lethal effects of Trichoderma longibrachiatum against Heterodera avenae. Biol Control. 2014;72:1–8.
- Xiang N, Lawrence KS, Donald PA. Biological control potential of plant growth-promoting rhizobacteria suppression of Meloidogyne incognita on cotton and Heterodera glycines on soybean: a review. J Phytopathol. 2018;166(7–8):449–458.
- Moreno-Gavíra A, Huertas V, Diánez F, et al. Paecilomyces and its importance in the biological control of agricultural pests and diseases. Plants. 2020;9(12):1746.
- Saxena G. Nematophagous fungi as biocontrol agents of plant-parasitic nematodes. In: Saxena G, Mukerji KG, editors. Management of nematode and insect-borne plant diseases. New York: The Haworth Press, Taylor and Francis Group; 2007. p. 165–194.
- Saxena G. Biological control of root-knot and cyst nematodes using nematophagous fungi. Giri B, Prasad R, Varma A editors. Root biology, soil biology 52. Switzerland: Springer Int. Pub. AG, part of Springer Nature. 2018. 221–237. DOI:10.1007/978-3-319-75910-4_8.
- Mittal N, Saxena G, Mukerji KG. Integrated control of root-knot disease in three crop plants using chitin and Paecilomyces lilacinus. Crop Prot. 1995;14(8):647–651.
- Hano P, Khan MR. Evaluation of fungal (Paecilomyces lilacinus) formulations against root knot nematode infecting tomato. Bangladesh J Bot. 2016;45(5):1003–1013.
- Schouteden N, De Waele D, Panis B, et al. Arbuscular mycorrhizal fungi for the biocontrol of plant-parasitic nematodes: a review of the mechanisms involved. Front Microbiol. 2015;6:1280.
- Muller R, Gooch PS. nematological reviews: Organic amendments in nematode control. an examination of literature. Nematropica. 1982;1:319–326.
- Nikolleta N, Maria KD, Nikolaos M, et al. Nematicidal amendments and soil remediation. Plants. 2020;9(4):429.
- Rodriguez-Kabana R, Morgan-Jones G, Chet I. Biological control of nematodes: soil amendments and microbial antagonists. Plant Soil. 1987;100(1):237–247.
- Kanwar RS, Patil JA, Yadav S. Prospects of using predatory nematodes in biological control for plant parasitic nematodes – a review. Biol Control. 2021;160:104668.
- Adams F, Burmester C, Hue NV, et al. A comparison of column-displacement and centrifuge method for obtaining soil solutions. Soil Sci Soc Am J. 1980;44(4):733–735.
- Hussey RS, Barker KR. A comparison of methods of collecting inocula of Meloidogyne spp., including a new technique. Plant Disease Reporter. 1973;57:1025–1028.
- Gerdemann JW, Nicolson TH. Spores of mycorrhizal Endogone species extracted from soil by wet sieving and decanting. Trans Br Mycol Soc. 1963;46(2):235–244.
- Bybd DW, Kirkpatrick T, Barker KR. An improved technique for clearing and staining plant tissues for detection of nematodes. J Nematol. 1983;15(1):142–143.
- deLeij FAAM, Kerry BR, Dennehy JA. Verticillium chlamydosporium as a biocontrol agent for Meloidogyne incognita and M. hapla in pot and microplot tests. Nematologica. 1993;39(1–4):115–126.
- Phillips JM, Hayman DS. Improved procedures for clearing roots and staining parasitic and vesicular-arbuscular mycorrhizal fungi for rapid assessment of infection. Trans Br Mycol Soc. 1970;55(1):158–161.
- Dong LQ, Yang JK, Zhang KQ. Cloning and phylogenetic analysis of the chitinase gene from the facultative pathogen Paecilomyces lilacinus. J Appl Microbiol. 2007;103(6):2476–2488.
- Cabanillas E, Barker KR. Impact of Paecilomyces lilacinus inoculum level and application time on control of Meloidogyne incognita on Tomato. J Nematol. 1989;21(1):115–120.
- Favre-Bonvin J, Ponchet M, Djian C, et al. Acetic acid: a selective nematicidal metabolite from culture filtrates of Paecilomyces lilacinus (Thom) Samson and Trichoderma longibrachiatum Rifai. Nematologica. 1991;37(1–4):101–112.
- Nesha R, Siddiqui ZA. Effects of Paecilomyces lilacinus and Aspergillus Niger alone and in combination on the growth, chlorophyll contents and soft rot disease complex of carrot. Sci Hortic. 2017;218:258–264.
- Siddiqui ZA, Akhtar MS. Effects of antagonistic fungi and plant growth-promoting rhizobacteria on growth of tomato and reproduction of the root-knot nematode, Meloidogyne incognita. Australas Plant Pathol. 2009;38(1):22–28.
- Walters SA, Barker KR. Efficacy of Paecilomyces lilacinus in suppressing Rotylenchulus reniformis on tomato. J Nematol. 1994;26:60.
- Subhagan SR, Dhalin D, Kumar AK. Microbial control: a potential tool for management of plant parasitic nematodes in black pepper (Piper nigrum L.). J Entomol Zool Studies. 2020;8:1168–1175.
- Sharma A, Trivedi PC. Influence of inoculum levels of fungus Paecilomyces lilacinus {Thorn.) Samson on the biocontrol of root-knot nematode, Meloidogyne incognita (Chitwood). Int Nematol Network Newsletter. 1989;6(2):27–29.
- Al-Raddad AM. Interaction of Glomus mosseae and Paecilomyces lilacinus on Meloidogyne javanica of tomato. Mycorrhiza. 1995;5(3):233–236.
- Bonants PJ, Fitters PF, Thijs H, et al. A basic serine protease from Paecilomyces lilacinus with biological activity against Meloidogyne hapla eggs. Microbiology. 1995;141(4):775–784.
- Padgham JL, Sikora RA. Biological control potential and modes of action of Bacillus megaterium against Meloidogyne graminicola on rice. Crop Prot. 2007;26(7):971–977.
- Sharma W, Trivedi PC. Concomitant effect of Paecilomyces lilacinus and vesicular arbuscular mycorrhizal fungi on root-knot nematode infested okra. Annals Plant Prot Sci. 1997;5(1):70–84.
- Carling DE, Roncadori RW, and Hussey RS. Interactions of arbuscular mycorrhizae, Meloidogyne arenaria, and phosphorus fertilization on peanuts. Mycorrhiza. 1996;6(1):9–13.
- Begum N, Qin C, Ahanger MA, et al. Role of arbuscular mycorrhizal fungi in plant growth regulation: implications in abiotic stress tolerance. Front Plant Sci. 2019;10:1068.
- Kohler J, Caravaca F, Azcón R, et al. The combination of compost addition and arbuscular mycorrhizal inoculation produced positive and synergistic effects on the phytomanagement of a semiarid mine tailing. SciTotal Environ. 2015;514:42–48.
- Gutjahr C, Paszkowski U. Multiple control levels of root system remodeling in arbuscular mycorrhizal symbiosis. Front Plant Sci. 2013;4:204.
- Azcón-Aguilar C, Barea JM. Arbuscular mycorrhizas and biological control of soil-borne plant pathogens – an overview of the mechanisms involved. Mycorrhiza. 1997;6(6):457–464.
- Pinochet J, Calvet C, Camprubí A, et al. Interactions between migratory endoparasitic nematodes and arbuscular mycorrhizal fungi in perennial crops: a review. Plant Soil. 1996;185(2):183–190.
- Berruti A, Lumini E, Balestrini R, et al. Arbuscular mycorrhizal fungi as natural biofertilizers: let’s benefit from past successes. Front Microbiol. 2016;6:1559.
- Rizvi R, Singh G, Safiuddin ARA, et al. Sustainable management of root-knot disease of tomato by neem cake and Glomus fasciculatum. Cogent Food Agric. 2015;1(1):1008859.
- El-Hadad ME, Mustafa MI, Selim S, et al. The nematicidal effect of some bacterial biofertilizers on Meloidogyne incognita in sandy soil. Braz J Microbiol. 2011;42(1):105–113.
- Gamalero E, Glick BR. The use of plant growth-promoting bacteria to prevent nematode damage to plants. Biology (Basel). 2020;9(11):381.
- Saxena G. Biocontrol of nematode-borne diseases in vegetable crops. In: Mukerji KG, editor. Fruit and vegetable diseases; disease management of fruits and vegetables: Vol 1 Kluwer academic publishers. Dordrecht: The Netherlands; 2004. p. 387–450.
- Castillo P, Navas-Cortés JA, Gomar-Tinoco D, et al. Interactions Between Meloidogyne artiellia, the cereal and legume root-knot nematode, and Fusarium oxysporum f. sp. ciceris Race 5 in Chickpea. Phytopathology. 2003;93(12):1513–1523.
- Bagyaraj DJ, Manjunath A, Reddy DDR. Interaction of vesicular arbuscular mycorrhiza with root knot nematodes in tomato. Plant Soil. 1979;51(3):397–403.