Abstract
Emissions of nitrous oxide (N2O) from aquatic systems such as rivers and estuaries are enhanced as a result of human activities on land resulting in enhanced nitrogen availability in aquatic systems. These human activities include agricultural activities such as fertilizer use, as well as industrial activities resulting in nitrogen (N) losses to the environment. In this article, we analyze past and future trends in global emissions of N2O from rivers and estuaries. We calculate aquatic N2O emissions from trends in the export of nitrogen to coastal waters by world-wide rivers. These trends in riverine N exports are from the Global NEWS models, which are global, regionally explicit models developed in the NEWS (Nutrient Export from WaterShed) framework. The NEWS models calculate nutrient exports from land to coastal waters, taking into account different human activities on the land, as well as biological N2 fixation and different ways in which nitrogen is retained in watersheds, including the effect of dams. We present global total emissions of N2O for the years 1970, 2000, and for four scenarios for 2050, as well as regional patterns.
1. Introduction
Nitrous oxide (N2O) is an important greenhouse gas. Emissions of N2O are enhanced as a result of human activities. The increase in atmospheric N2O is largely associated with food production (Kroeze et al. Citation1999). The change in land as well as fertilizer usage tends to enhance biological formation of N2O during nitrification and denitrification in agricultural soils (Bouwman et al. Citation2002). Only part of the nitrogen (N) used in agriculture is taken up by crops. The remainder is lost to the environment, through leaching and runoff, or through gaseous losses (Mosier et al. Citation1998). This nitrogen is giving rise to enhanced N2O formation in natural areas, including aquatic systems such as rivers and estuaries. Other sources of increased nitrogen loading to aquatic systems include industrial activities and sewage systems. In this article, we focus on N2O emissions from rivers and coastal waters.
We analyze past and future trends in emissions of N2O from rivers and estuaries and compare these to emissions from other aquatic systems such as continental shelves and the deep ocean. To estimate aquatic N2O emissions from rivers and estuaries, we first calculate trends in the export of nitrogen to coastal waters by world-wide rivers. These trends in riverine N export are from the Global NEWS models, which are global, regionally explicit models developed in the NEWS (Nutrient Export from WaterShed) framework (http://marine.rutgers.edu/global news/). The NEWS models calculate nutrient export from land to coastal waters, taking into account different human activities on the land, as well as biological N2 fixation and different nitrogen-retention processes in watersheds, including effects of dams. NEWS models for different forms of N have been developed (Beusen et al. Citation2005; Dumont et al. Citation2005; Harrison et al. Citation2005). Recently, these models have been updated and combined into one unified framework (Mayorga et al. Citation2010; Seitzinger et al. in press). The NEWS models have been implemented for past years (1970 and 2000) and for the millennium ecosystem assessment scenarios up to the year 2050.
The NEWS model outputs are used here to estimate associated trends in aquatic emissions of N2O following earlier approaches to estimate emissions. We present global totals, as well as regional patterns.
2 Method for estimating aquatic emissions of N2O
We calculate N2O emissions as a function of N export by rivers. First, we estimate N2O emissions from rivers and estuaries for the year 2000, using different approaches. N2O formation in rivers depends on the N loading of rivers. However, existing approaches differ with respect to the form of N included in their equations. Some estimate N2O from dissolved inorganic N only (Seitzinger and Kroeze Citation1998; Kroeze et al. Citation2005). Others estimate N2O from total N inputs to rivers, arguing that other forms of N are also biologically available (De Klein et al. Citation2007). Here, we use two different approaches: we calculate N2O from rivers and estuaries either as a function of total dissolved inorganic and organic N (DN) or as a function of total N including particulate forms of N (TN). This may give an indication of the implication of different approaches to estimate aquatic N2O emissions.
The emission factors to be used for aquatic sources of N2O have been an issue of debate (Mosier et al. Citation1998; Kroeze et al. Citation2005; De Klein et al. Citation2007), and default emission factors suggested in the IPCC Guidelines for National Greenhouse Gas Inventories have been updated recently (De Klein et al. Citation2007). The IPCC default emission factor for rivers (0.0025 kg N2O‒N per kg of N inputs to rivers) has been revised downwards compared to earlier versions of the IPCC Guidelines (0.0075 kg N2O‒N per kg of N inputs to rivers). Both values are within the range of uncertainties (Mosier et al. Citation1998). The current version of the IPCC Guidelines recognizes that the current default value may be too low for some rivers. Here, we therefore apply two approaches for emission factors: (1) the current IPCC default, to which we refer as the low case, and (2) an alternative approach largely following Seitzinger and Kroeze (Citation1998) where we apply a higher emission factor (0.025) to rivers with relatively high N loadings (>10 kg N per km2 of watershed), to which we refer as the high case. The IPCC emission factors are typically developed for total N fluxes, but also applied to dissolved N fluxes as indicated above. Here, we do both. The resulting low and high cases should not be interpreted as the lower and upper bounds of the uncertainty range in estimates of global N2O emissions from aquatic systems. Rather, they reflect the differences between various suggested default emission factors.
We use N export rates from the Global NEWS models (Mayorga et al. Citation2010; Seitzinger et al. in press). The Global NEWS models can be used to calculate nutrient export by rivers to coastal seas for more than 6000 rivers in the world. The models not only calculate N export, but also river export of carbon (C), phosphorus (P) and silica. Here, we use river export of dissolved N (DN) and total N (TN) as calculated by Global NEWS to calculate N2O emissions from aquatic sources. This N export by river is calculated as a function of hydrology, basin characteristics and human activities on the land. Hydrology includes river discharge and takes into account human water consumption, damming of rivers and the effects of climate change. Basin characteristics include biophysical aspects that are important for nutrient retention in rivers, and nutrient inputs to rivers. And human activities range from agricultural activities in the watersheds, to sewage connection and treatment. Details on these model inputs and how they change over time can be found in several papers describing the Global NEWS model input (Bouwman et al. Citation2009; Van Drecht et al. Citation2009; Fekete et al. in press).
We calculate emissions for the years 1970, 2000, and 2050 for the four Millennium Ecosystem Assessment (MEA) scenarios. These scenarios are named Global Orchestration (GO), Order from Strength (OS), Technogarden (TG), and Adapting Mosaic (AM). The scenarios have been described in detail elsewhere (Alcamo et al. Citation2006). In short, they differ in the way markets will develop (globalization or regionalization) and the attitude towards environmental issues and ecosystem management (proactive or reactive):
Globalization: GO and TG. | |||||
Regionalization: OS and AM. | |||||
Reactive ecosystem management: GO and OS. | |||||
Pro-active ecosystem management: TG and AM. |
The MEA scenarios were interpreted to prepare the input data for the Global NEWS models (Bouwman et al. Citation2009; Van Drecht et al. Citation2009; Fekete et al. 2010). These input data range from human population densities, to agricultural parameters (land use, fertilizer use, manure excretion, crop production, etc), sewage system characteristics (fraction of people connected to sewage systems, sewage treatment, etc), and hydrology (basin discharge, human water consumption, damming, etc). In general, in the scenarios assuming a pro-active approach towards ecosystem management (TG and AM) there is more nitrogen efficient agriculture, and more advanced sewage control. These scenarios also assume more hydropower.
3 Estimates of present-day emissions from aquatic sources
We first calculate N2O emissions from rivers and estuaries for the year 2000, using four different approaches ().
Table 1. Global N2O emissions from rivers and estuaries as estimates from total N (TN) or dissolved N (DN) in rivers, and using low or high emission factors.
Our estimates for 2000 (0.3–2.1 Tg N2O‒N) are in line with earlier estimates of 1.3 Tg N2O‒N for 1990 (Seitzinger et al. Citation1998) and 1.5 Tg N2O‒N for 2000 (Kroeze et al. Citation2005). The differences with these earlier estimates can be explained by the emission factors used, and the fact that Seitzinger and Kroeze (Citation1998) and Kroeze et al. [Citation2005] only considered dissolved inorganic N (DIN) in rivers as a source of N2O.
We may compare our estimates for current river and estuarine N2O emissions (0.3–2.1 Tg N2O‒N in 2000) to estimates for emissions from continental shelves (0.6 Tg N2O‒N in 1990 (Seitzinger et al. Citation1998)) and recent estimates for open ocean sources, partly induced by atmospheric N deposition (5 Tg N2O‒N at present (Duce et al. Citation2008)). This would total the aquatic source of N2O to 6–8 Tg N2O‒N, or 30–40% of the 19 Tg global emissions needed to explain the increase in atmospheric N2O (Kroeze et al. Citation1999).
Which of the four approaches is most appropriate is difficult to say. One may argue that calculating N2O from dissolved N, while ignoring particulate forms of N, may underestimate N2O emissions because part of the particulate N is biologically available and may be denitrified, giving rise to increased N2O emissions. Also, using the low emission factor (0.0025 kg N2O‒N per kg of N inputs to rivers) may result in an underestimation, as indicated in the IPCC Guidelines and earlier studies (Seitzinger et al. Citation1998; De Klein et al. Citation2007). On the other hand, estimating N2O emissions from total N using high emission factors may result in an overestimation, because not all particulate N is biologically available, and because the high emission factor may be chosen too high for some rivers.
In the following, we use the case DN high (calculating emissions from dissolved N, using high emission factors for rivers with high N loadings) for analyses of future trends. We chose this case because it is an intermediate case, not because we consider high emission factors the best. The N2O emission estimates based on case DN high are in the middle of the range of estimates of global N2O emissions. Moreover, the NEWS model predictions of DN are validated with more measurements than those of PN, so we may consider DN estimates more appropriate.
4 N2O from rivers and estuaries: past and future trends
shows global N2O emissions from rivers and estuaries as calculated for past and future years (1970, 2000, and 2050).
Figure 1. Global emissions of nitrous oxide (N2O) from rivers and estuaries in 1970, 2000, and four scenarios for 2050.
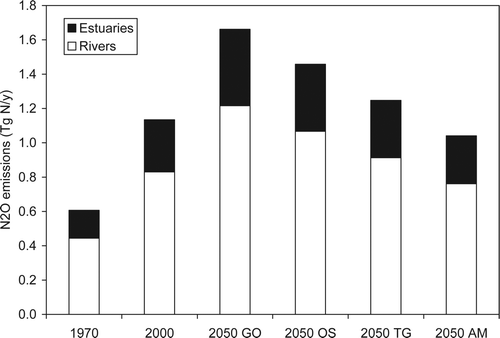
Global emissions have almost doubled between 1970 and 2000, from 0.6 Tg N2O‒N /y to 1.1 Tg N2O‒N /y. This is largely caused by agricultural activities and to some extent by sewage inputs of N into rivers.
Between 2000 and 2050, N2O emissions from rivers and estuaries may continue to increase or decrease, depending on the scenario. It is interesting to note that future increases are smaller than the past increase. This may seem surprising, given the increased population growth and economic development in this period, and associated trends in food production. We calculate the largest increase for the period 2000–2050 for the GO scenario (almost 50% increase to 1.7 Tg N/y); the globalized scenario assuming reactive ecosystem management. The lowest emissions are calculated for the AM scenario, in which 2050 emissions are in fact 8% lower than in 2000 (1 Tg N2O‒N/y). In general, in the scenarios assuming a pro-active attitude (TG and AM) towards environmental management, N2O emissions are lower than in the scenarios assuming a reactive attitude (GO and OS). The relatively moderate increases in the future are the effect of human activities on the land, and hydrological trends, including the effects of human consumption of river water, increased damming of rivers, and the effects of climate change on river runoff.
Even though future increases in N2O emissions from rivers and estuaries may seem moderate compared to past trends, and compared to the expected future population and economic growth, any increase in emissions to more than the current 1.1 Tg N2O‒N/y is a reason for concern, considering the policy targets to reduce greenhouse-gas emissions.
The trends in aquatic N2O emissions also reflect the trends in N inputs to coastal waters. Coastal eutrophication is a worldwide problem (Heil et al. Citation2005; Diaz and Rosenberg Citation2008; Selman et al. Citation2008). Recent inventories indicate that dead zones in the coastal oceans spread exponentially, and started to approximately double every 10 years starting in the 1960s (Diaz and Rosenberg Citation2008). River inputs of nutrients are generally considered a major cause of coastal eutrophication (Glibert et al. Citation2008). An increase in river export of nutrients may result in additional coastal eutrophication.
Global trends alone may not be the best indicator for local or regional trends. In fact, the NEWS model results indicate that local and regional trends may differ largely from global trends (Seitzinger et al. Citation2005b; Seitzinger et al. 2010). Therefore, we also present continental trends here (, ). The relative share of continents in global emissions changes over time. In 1970, about one-third of the emissions were from Asia, and another one-third from the Americas. In 2000, almost 50% of the emissions are from Asia. And in 2050, 40–50% of the emissions are from Asia, depending on the scenario.
Figure 2. Global emissions of nitrous oxide (N2O) from rivers and estuaries in 1970, 2000, and four scenarios for 2050 by continent. NB: Oceania includes Australia.
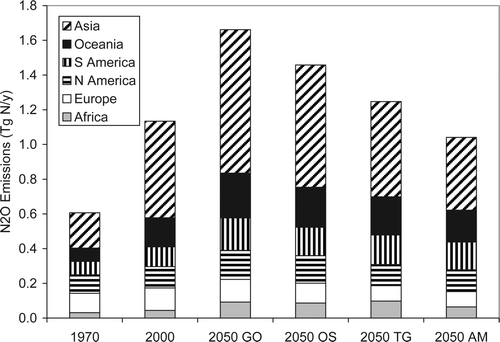
Table 2. Past (1970–2000) and future (2000–2050) trends in global emissions of nitrous oxide (N2O) from rivers and estuaries by continent.
The continental trends differ considerably from global trends (). For the period 1970–2000, we calculate increasing N2O emissions for all continents. Largest increases are calculated for Oceania and Asia where emissions roughly double and triple, respectively. Lowest increases are calculated for North America and Europe. These differences in trends can be explained by differences in population growth and economic activities.
For the period 2000–2050, we also calculate large differences in trends among scenarios (). The differences are most pronounced for Asia, where emissions may increase by almost 50% (GO scenario) or decrease by 25% (AM scenario). This large difference between the GO and AM scenario can be largely explained by the assumed attitude towards environmental management. In the AM scenario, the N is used more efficient in agriculture than in the GO scenario, effectively reducing the N loading of rivers. Other than the TG scenario, the AM scenario assumes local solutions to environmental problems, such as closing of nutrient cycles at the local scale. We also calculate reductions in emissions for Europe (all scenarios except GO) and North America (TG and AM scenarios). For Europe, this is associated with trends in agriculture aiming at reducing nutrient inputs to the environment. We calculate relatively large increases for South America in all scenarios.
It should be noted, that our calculations do not consider the additional amount of N2O that is produced on continental shelves due to river N that is either exported from estuaries or discharged directly by large rivers to continental shelves (Seitzinger et al. Citation2005a). This additional N2O could considerably exceed the amount of N2O that is produced in estuaries. As mentioned above, we also do not consider N2O emissions from the deep ocean, which may have increased as a result of atmospheric N deposition (Duce et al. Citation2008).
5 Conclusions
On the basis of the method to estimate emissions, we had found current N2O emissions from rivers and estuaries at 0.3–2.1 Tg N2O‒N in 2000. The total aquatic source (including continental shelves and the deep ocean) could total 6–8 Tg N2O‒N, or 30–40% of the 19 Tg global emissions needed to explain the increase in atmospheric N2O.
Global N2O emissions from rivers and estuaries have almost doubled between 1970 and 2000. For the period 2000–2050, we calculate increasing and decreasing trends in aquatic N2O emissions, depending on the scenario. The largest increase (almost 50%) is calculated for the GO scenario. This is a smaller increase than past increase. In the AM scenario, global N2O emissions even decrease by 8% relative to 2000. This slow down in the growth rate of aquatic N2O emissions contrasts with the increased population growth and economic development in this period. The relatively moderate increases in future emissions are the net effect of human activities on the land and hydrological trends. Nevertheless, we consider these increases reasons for concern given the policy targets to reduce greenhouse-gas emissions.
Acknowledgements
This study was performed as part of the International Global Nutrient Export from Watershed(S) (NEWS) activity: http://marine.rutgers.edu/globalnews. Global NEWS has been co-funded by UNESCO-IOC and is a project under LOICZ.
References
- Alcamo , J , Van Vuuren , D , Cramer , W , Alder , J , Bennett , E , Carpenter , S , Christensen , V , Foley , J , Maerker , M , Masui , T Morita , T . 2006 . “ Changes in ecosystem services and their drivers across the scenarios. Vol. 2 ” . In Millennium ecosystem assessment Edited by: Carpenter , S , Pingali , P , Bennet , E and Zurek , M . Island Press (WA): Scenarios. p. 297–373
- Beusen , A HW , Dekkers , A LM , Bouwman , A F , Ludwig , W and Harrison , J . 2005 . Estimation of global river transport of sediments and associated particulate C, N, and P . Global Biogeochem Cycles. , 19 : GB4S05
- Bouwman , A F , Boumans , L JM and Batjes , N H . 2002 . Modeling global annual N2O and NO emissions from fertilized fields . Global Biogeochem Cycles. , 16 : 28 – 21 .
- Bouwman , A F , Beusen , A HW and Billen , G . 2009 . Human alteration of the global nitrogen and phosphorus soil balances for the period 1970–2050 . Global Biogeochem Cycle. , 23 : GB0A04 doi:10.1029/2009GB003576
- De Klein , C , Novoa , R SA , Ogle , S , Smith , K A , Rochette , P , Wirth , T C , McConkey , B G , Mosier , A , Rypdal , K Walsh , M . 2007 . “ Chapter 11: N2O emissions from managed soils, and CO2 emissions from lime and urea application ” . In 2006 IPCC guidelines for national greenhouse gas inventories [cited 2010 Jun 24]. Available from: http://www.ipcc-nggip.iges.or.jp/
- Diaz , R J and Rosenberg , R . 2008 . Spreading dead zones and consequences for marine ecosystems . Science. , 321 : 926 – 929 .
- Duce , R A , LaRoche , J , Altieri , K , Arrigo , K R , Baker , A R , Capone , D G , Cornell , S , Dentener , F , Galloway , J Ganeshram , R S . 2008 . Impacts of atmospheric anthropogenic nitrogen on the open ocean . Science. , 320 : 893 – 897 .
- Dumont , E , Harrison , J A , Kroeze , C , Bakker , E J and Seitzinger , S P . 2005 . Global distribution and sources of DIN export to the coastal zone: results from a spatially explicit, global model . Global Biogeochem Cycles. , 19 : GB4S02
- Fekete , B M , Wisser , D , Kroeze , C , Mayorga , E , Bouwman , A F , Wollheim , W M and Vörösmarty , C J . in press . Millennium ecosystem assessment scenario drivers (1970–2050): climate and hydrological alterations . Global Biogeochem Cycles. , doi:10.1029/2009GB003593
- Glibert , P M , Mayorga , E and Seitzinger , S . 2008 . Prorocentrum minimum tracks anthropogenic nitrogen and phosphorus inputs on a global basis: application of spatially explicit nutrient export models . Harmful Algae , 8 : 33 – 38 .
- Harrison , J A , Caraco , N and Seitzinger , S P . 2005 . Global patterns and sources of dissolved organic matter export to the coastal zone: results from a spatially explicit, global model . Global Biogeochemical Cycle. , 19 GB4S03, doi:10.1029/2004GB002357
- Heil , C A , Glibert , P M and Fan , C . 2005 . Prorocentrum minimum (Pavillard) Schiller: a review of a harmful algal bloom species of growing worldwide importance . Harmful Algae , 4 : 449 – 470 .
- Kroeze , C , Dumont , E and Seitzinger , S P . 2005 . New estimates of global emissions of N2O from rivers, estuaries and continental shelves . Environ Sci. , 2 ( 2–3 ) : 159 – 167 .
- Kroeze , C , Mosier , A and Bouwman , A F . 1999 . Closing the global N2O budget: a retrospective analysis 1500–1994 . Global Biogeochem Cycle. , 13 : 1 – 8 .
- Mayorga , E , Seitzinger , S P , Harrison , J A , Dumont , E , Beusen , A HW , Bouwman , A F , Fekete , B , Kroeze , C and Van Drecht , G . 2010 . Global nutrient export from watersheds 2 (NEWS 2): model development and implementation . Environ Model Software , 25 : 837 – 853 .
- Mosier , A , Kroeze , C , Nevison , C , Oenema , O , Seitzinger , S and van Cleemput , O . 1998 . Closing the global N2O budget: nitrous oxide emissions through the agricultural nitrogen cycle . Nutrient Cycl Agroecosystems. , 52 : 225 – 248 .
- Seitzinger , S , Emilio Mayorga , E , Bouwman , A F , Kroeze , C , Beusen , A HW , Billen , G , Van Drecht , G , Dumont , E , Fekete , B M and Garnier , J . in press . Global river nutrient export: a scenario analysis of past and future trends . Global Biogeochem Cycle , doi:10.1029/2009GB003587
- Seitzinger , S , Harrison , J , Bo , J K , Bouwman , A F , Lowrance , R , Peterson , B , Tobias , C and Van Drecht , G . 2005a . Denitrification across landscapes and waterscapes: a synthesis . Ecol Appl. , 16 : 2064 – 2090 .
- Seitzinger , S P and Kroeze , C . 1998 . Global distribution of nitrous oxide production and N inputs in freshwater and coastal marine ecosystems . Global Biogeochem Cycle. , 12 : 93 – 113 .
- Seitzinger , S P , Harrison , J A , Dumont , E , Beusen , A HW and Bouwman , A F . 2005b . Sources and delivery of carbon, nitrogen, and phosphorus to the coastal zone: an overview of global nutrient export from watersheds (NEWS) models and their application . Global Biogeochem Cycle. , 19 : GB4S01 doi:10.1029/2005GB002606
- Selman , M , Greenhalgh , S , Diaz , R and Zachary , S [internet] . 2008 . Eutrophication and hypoxia in coastal areas: a global assessment of the state of knowledge. WRI Policy Note 1. World Resources Institute. 2007. EarthTrends: Environmental Information , Washington DC : World Resources Institute . Available from: http://earthtrends.wri.org
- Van Drecht , G , Bouwman , A F , Harrison , J and Knoop , J M . 2009 . Global nitrogen and phosphate in urban waste water for the period 1970–2050 . Global Biogeochem Cycles , 23 : GB0A03 doi:10.1029/2009GB003458