Abstract
Energy security, once solely a local matter, has evolved into several scales of consideration – household, workplace, nation-state, and global systems. In result, energy policies addressing energy security must evolve as well. Based on discussions at a two-day workshop in Singapore, this paper summarizes four of the most important. First, in order for energy policies to embrace both efficacy and equity, they must be considered in terms of their scalar effects; second, energy security at the national level demands that each country initiate a diversity of energy supplies and an abundance of routes for energy delivery; third, establishing proper and effective policies for energy security must closely consider context if they are to be adequate and useful; fourth, energy security requires a non-linear approach to policy formation if threats and challenges are to be adequately conceptualized and addressed. All future research into energy security must explore the complex relationship between scale and energy security.
Introduction
The idea of energy security is, in simplest form, a local concept. This is no longer true. Energy security nowadays is taken to include the provision of available, affordable, reliable, efficient, environmentally benign, properly governed and socially acceptable energy services. It embraces energy efficiency, diversification, innovation, trade, and public health (Barton Citation2004; Muller-Kraenner Citation2007; UN [United Nations] Citation2008; Luft and Korin Citation2009; Sovacool Citation2009, Citation2011a, Citation2011b; US [United States Congress] Citation2009; Löschel et al. Citation2010; Pascual and Elkind 2010; Sovacool and Brown Citation2010; Bambawale and Sovacool Citation2011a, Citation2011b, Citation2011c; Sovacool et al. Citation2011). Meeting each item on this broadened list of conditions requires establishing relevant energy policies and shifting our point of emphasis from technology to society (Pasqualetti Citation2011). This change has expanded the discussion about energy security from a single theme to multiple themes.
We see evidence of this change wherever we look. For example, while some people worry about the rising prospect of international “resource wars” (Klare Citation2007), others focus at the national scale about “the resource curse” (Watts Citation2010). Still others concentrate on fine-grained matters such as how windfall revenues from oil or gas production can change government behavior, imperil economic development, institutionalize corruption, and enable oppressive regimes to maintain their political power (Karl Citation1998; Sachs and Warner Citation1999; Karl Citation2000; Auty Citation2004; Robinson et al. Citation2006).
Energy security is part of the political and economic geography of energy (Bridge Citation2004, Citation2008; Bridge and Wood Citation2005; Hess and Yueng Citation2006; Coe et al. Citation2008). As such, it should be pertinent at every scale. In turn, it should be reflected in the formation and implementation of energy policy.Footnote1 This article has a three-fold purpose. First, to provide illustrations of energy security at several scales. Second, to suggest that related energy policy must be developed within a similar scalar context. Third, to stimulate empirical research that develops the theme of energy security at various scales. We begin with the individual household and proceed step-wise to the entire planet.
Household Scale
Energy security at the household scale are primarily matters of equity and public health. They circulate within the cauldron of energy poverty, environmental impacts, and growing inequities. This stew of worries is most common in the developing world. For these countries, improved access to energy services is essential to economic development. Worldwide, nearly 2.4 billion people use traditional biomass fuels for cooking and heating (). Another 1.6 billion have little or no access to electricity. Even accounting for significant increases in development assistance and rural electrification programs in emerging economies, by 2030 about 1.4 billion will still be at risk of having to live without modern energy services (Modi et al. Citation2005). When correlated with the amount of energy consumed per capita, a person living in North America consumes almost 20 times the amount of a person living in India.
Table 1. Number of people relying on traditional biomass.
Such stark energy disparity and energy poverty intersects with other pressing problems including gender equity, social justice, and environmental degradation. Without access to modern energy carriers such as transmission lines, women and children are typically expected to devote considerable parts of their days searching for firewood to heat their homes and prepare meals. Tragically, these activities present many occupational hazards and personal threats. For example, 10,000 fuel-wood carriers in Addis Ababa suffer frequent falls, bone fractures, eye problems, headaches, anemia, internal body disorders, and miscarriages from carrying heavy loads that are often equal to their body weights. Fuel collection also places women in locations of physical or psychological abuse. Hundreds of documented cases have revealed women facing rape while collecting necessary fuels for cooking or heating (UNDP [United Nations Development Programme] 1997).
In addition, energy insecurity often has a rippling effect on health, hunger and environmental impacts. For example:
• | Using traditional fuels is often unhealthy, since these fuels are typically used indoors in unvented household cooking stoves – such as a pit or a U-shaped construction made from mud. This means that not only does the fuel burn inefficiently, but that its combustion can lead to deadly concentrations of carbon monoxide (Holdren and Smith 2000). | ||||
• | The scarcity of cooking fuels is linked to hunger, as the poorest families must devote a higher share of their income to purchase fuel. This leaves less money for food (Modi et al. Citation2005; UNESCAP [United Nations Economic and Social Commission for Asia and the Pacific] 2008). | ||||
• | Gathering fuel wood by such impoverished people removes bark and dung, strips away live branches to supply smaller pieces needed as kindling, thereby depleting forestlands of needed nutrients. In turn, such fuel depletion instigates competition and conflict over land, further decreases food supply, diminishes sources of traditional medicine, and accelerates malnutrition. |
Sudden shifts in energy prices also tend to hurt poorest families the most, since they expend a larger share of their income on energy fuels and services (Barnes and Willem Citation1996; Masud et al. Citation2007; Legros et al. Citation2009). One study looking at the effects of increases in the price of fuels in four developing Asian economies from 2002 to 2005 found that poorer households paid 171% more of their income for cooking fuels and 120% more for transportation, 67% more for electricity and 33% more for fertilizers when compared to the expenditures on energy from middle and upper class households (UNESCAP 2008).
All considered, increased energy security at the household scale can have the most immediate and personal benefits. Yet, energy policies that can produce such increased energy security at this scale tend to attract the least attention, in part because issues of safety and health are more diffuse and hold less political weight and influence.
Workplace scale
While working at the household scale may involve traveling for fuel collection, the emphasis is still family and home, including its maintenance and its sense of personal space. The workplace is different; it is usually under the auspices of people with other priorities. Here, two interests in energy security dominate: (1) occupational hazards at the workplace; and (2) the energy that is embodied in produced products. Although energy security in the workplace is needed to maintain heating, cooling, and ventilation, it has other functions as well. For example, energy security is essential to maintain safe operation of machinery, especially in dangerous working environments such as coal mines (Healy Citation2003). It is critical in hospitals, which all require near-perfect energy security to carry out their mission without the life-threatening consequences that a supply interruption could cause (Newton Citation2001).
Life cycle assessments reveal that the embodied energy in various products can be quite high. This means that, for products such as steel and cement, energy security is particularly important (Worrell et al. Citation2008). For these and other products such as aluminum and paper that rank high on the list of embodied energy, however, there are others parts to the story; just as important as the amount of energy supply is its consistency and its purity. This is true because the general trend towards high product purity rises as well, and with it so too does the energy required to achieve it. All these considerations of security must be addressed in corporate energy policies.
Perhaps nowhere is energy security more important than in the manufacturing of semiconductors. The greater the required purity, the more energy is needed, and the higher is the cost paid to guarantee its secure supply. This means that the chips that are inside so many of the products we use have high energy intensities. This trend was illustrated by Krishman et al. (Citation2008) in their examination of the potential secondary materialization in semiconductor manufacturing for three cases: cleanrooms, producing ultrapure water (UPW), and purifying elemental gases. As they pointed out, purification of water and elemental gases requires dramatic increases in energy use, and electricity use per square foot tends to increase with increasing air purity.
The same can be said for everyday consumer products. For example, the total energy used in producing a desktop computer with 17-inch CRT monitor is estimated to be 6400 megajoules (MJ), equivalent to 260 kg of a fossil fuel like coal or oil. This high-energy intensity of manufacturing, combined with rapid turnover in computers, results in an annual life cycle energy burden that is about 2600 MJ per year, or 1.3 times that of a refrigerator (Williams et al. Citation2002).
The high growth in secondary – or embodied – energy use suggests that the characterization and management of energy deserves increased attention not just as matters of good business but as matters of energy security in the workplace. For many products, policies must ensure energy security, often over efficiency and even production cost. In such industries, energy security and supportive energy policies have the highest possible priority.
National scale
In developing and developed countries, as industry goes so goes the economy. For this reason, the national scale presents its own unique set of energy security issues. They fall into three major categories: national defense, national infrastructure, and the environmental costs of maintaining both.
National defense, especially for large countries, is an especially energy-intensive activity. The US Department of Defense, for example, is the largest single consumer of energy in the nation, accounting for about three-quarters of the energy used by the Federal Government, at a cost of over $34 billion in FY 09. It has long been recognized that a secure country depends upon a secure supply of energy. Recently, the challenges of such dependency have increased with the intensification of concern for the effects of terrorism. For example, to combat terrorism and ensure security for our energy and utility systems, “it's important to look at the anti-terrorism/force protection measures needed and equally important is how to reduce our weaknesses and vulnerability and how to provide for the needs of our military and at the same time ensure their ‘quality of life’” (Moy 2003, p. 509–510).
Supply lines from the Middle East are especially expensive to maintain and protect, particularly at natural ‘chokepoints’ such as the Strait of Hormuz. By one estimate, the US military spends between $39 billion and $98.5 billion annually to secure the production and transport of foreign oil here and elsewhere (CTA [International Center for Technology Assessment] 2005). Such costs come due when we pay our taxes, if not in direct energy charges. Whatever the total price now, costs are expected to rise and some people will find that meeting their full energy needs will recede out of reach. For them, as energy prices go up, energy security goes down.
Satisfactory national defense presumes that energy distribution systems operate efficiently and without interruption. Even in the absence of intentional interruptions, this is a daunting challenge, especially in large countries. In the US, for example, there are about 20,000 miles of natural gas gathering lines, 278,000 miles of natural gas transmission lines, and 1.9 million miles of distribution lines (ANL [Argonne National Laboratory] n.d.). Together with over 1400 compressor stations, this network presents innumerable security soft targets for intentional disruptions (See ). There are also thousands of miles of oil pipelines, and a vast network of transmission lines, much of which is isolated and unguarded.
To the extent that national defense in larger nations requires greater energy resources and a more extensive energy supply infrastructure to keep supplies available, it typifies what Lovins and Lovins (1983) 30 years ago termed our “brittle” infrastructure: an energy system characterized by relatively large sources of supply and distribution, heavily dependent on a few critical nodes and links. Recent studies have confirmed that such vulnerabilities persist (Kalicki and Goldwyn 2005; CNA 2009; Kleber 2009). Several well-publicized blackouts in the past ten years in the US underscore this problem.
Because of the threat that interruptions pose to national security and the national economy, an apparatus for quick repairs is essential, albeit not always feasible (Nye 2009). In the US, the policy reaction to this security problem has been to establish several “reliability districts” to limit the impacts of such breakdowns. These districts have helped increase security, as desired, but much vulnerability remains.
Two options are being proposed in response to these types of energy insecurities. There has been substantial discussion of establishing a so-called “smart grid” that will improve efficiency, uniformity, and reliability. It is an expensive proposal, but it is gaining traction in large part because it has the potential to address concerns about the security of energy supplies at the national level by making energy production and use more efficient (Garrity 2008). The second option is to encourage the establishment of more ‘distributed generation’ (US DoE [US Department of Energy] n.d.). The logic of this approach – such as in the form of roof-top solar – rests on the idea that having many sources of generation is more secure than having only a few. Encouraging this approach to energy security will rest on the successful implementation of several adjustments in technology and policy.
Regardless of how each nation pursues the goal of greater energy security, it will be costly, and not all of these costs will be economic in nature. As Andres (2009) points out, environmental degradation is one price of energy production and use, and it is occurring both within countries and among them. For example, as the United States continues to sustain a high demand for imported oil, the threats to environmental quality continue as well. The frequent oil spills in Nigeria, the Straits of Malacca, the Caspian Basin and Siberia illustrate this point. The 2009 Deepwater Horizon oil well blowout in the Gulf of Mexico is another case in point, and it is likely just the first of what may become common as development moves into less hospitable and politically unstable places (Miller 2010).
Many policies are available to increase energy security at the national scale. In the US, three are prominent. One has been to shift the dependency on oil imported from the Middle East in favor of oil imported from closer and more secure countries such as Canada, especially from the giant oil sands reserves in Alberta.2 Another policy decreased energy demand through the greater exploitation of energy efficiency in the residential, commercial, and industrial sectors, essentially considering it equivalent to a resource. A third policy has been to promote greater mileage efficiency in the transportation sector, particular of US automobile fleet. A fourth has been to subsize the use of biofuels, such as ethanol. Whatever policies turn out to be effective at increasing energy security at the national scale, they will all also insinuate energy security repercussions at the global scale as well.
Global scale
Energy security is more complex at the global scale than it is at any other. Roughly speaking, three areas dominate interest: geopolitics and war, global investment barriers, and trans-boundary externalities (Florini and Sovacool 2011).
Geopolitics
Modern economies and militaries depend heavily on fossil fuels and uranium. The most important of these fuels, oil, is highly concentrated among a few key countries and is under the control of only a few companies. Three of the biggest of these companies – Saudi Arabian Oil Company, National Iranian Oil Company, and Qatar Petroleum – own more crude oil than the next 40 largest oil companies, combined. The 12 largest oil companies control roughly 80% of petroleum reserves and are all state owned. When considered by country, 80% of the world’s oil can be found in nine countries (which hold only 5% of world population); 80% of the world’s natural gas is in 13 countries, particularly Russia; 85.1% of the world’s coal is found in just six – the United States, Russia, China, India, Australia, and South Africa (Kessels et al. 2008; Brown and Sovacool 2011). Six countries control more than 80% of global uranium resources, led by Australia and Kazakhstan.3 Looking at current reserve-to-production ratios for proven fossil fuel reserves and identified uranium resources (assuming a zero increase in growth of production) we find that the world has 137 years of coal left, 60 years of natural gas, 43 years of petroleum, and 85 years of uranium. The difficulty, assuming development costs, is that these resources are finite, while the demand for them is not. Taken together, this characteristic of fossil fuels poses such a threat to energy security that Jackson (1991, p. 7) once referred to them as “thermodynamic time-bombs”. Moreover, the issue of reserves is not just geographical and geological, it is also economic. That is, how much are they worth in the global energy marketplace?
Further complicating the situation is the spatial disequilibrium between reserves and demand; dozens of countries need the oil, but few countries can supply it. The resulting pattern of international trade – particularly of oil – triggers major security concerns whenever supplies are constrained. Consider the situation with Russian gas pipelines. In 1997, Russia restricted natural gas transmission to Turkmenistan to coerce higher prices after a dispute over contracts. Russia again used control of their natural gas pipelines in 2005 to manipulate the market to their advantage and gain concessions on gas prices from Ukraine, even at the risk of blackouts and international outrage further along the pipeline (Kleveman 2003; Pamir 2006; Bilgin 2009; Ericson 2009). Russia has used the same tactic at least five times since, in natural gas disputes with Belarus, Georgia, Moldova, and again in Ukraine in 2007 (Financial Times 2008). The lesson is simple: suppliers and energy carriers frequently manipulate energy dependency to their advantage.
These instances of resource control are recent and relatively minor when compared to far more serious historical examples. Geographer Vaclav Smil (2004) traces the role of energy and war by documenting numerous times where energy reserves prompted or exacerbated international conflict. Apart from identifying the interconnections between energy reserves and the World Wars, Smil argues that energy and energy resources were factors in the Korean War (North Korea is the coal-rich part of the peninsula), the Vietnam War (waged by France until 1954 and by the US after 1964 in part for Vietnamese oil and gas reserves), the Soviet occupation of Afghanistan (which had significant mineral resources), and the first Gulf War (explicitly about oil and the Iraqi occupation of Kuwait). Smil also suggests that “almost all” recent cross-border wars of the twentieth century were related to energy, including the conflict between India and Pakistan, Eritrea and Ethiopia, China and India, and civil wars such as those in Sri Lanka, Uganda, Angola, and Columbia. The more recent examples of Sudan can be added to this list.
Projections about trade and investment from the International Energy Agency, among other organizations, suggest that the world will need to invest upwards of $40 trillion to meet growing demand for energy by the middle of this century (IEA [International Energy Agency] 2009). This will require more intense international trade and produce a rise in accompanying risks to energy security as a result. New energy policies are needed to manage this risk, policies that will include incentives for domestic energy production, subsidies for renewable energy development, and changes in military postures to protect production fields and the complicated apparatus of transportation.
Global investment barriers
Not all energy security issues rest on the map of supply and demand. Energy security is also tied to the current global structure of intellectual property rights. The structure of such rights can create impediments to the diffusion of new energy technologies among countries. For example, intellectual property rights have been used to block entry into markets for wind turbines, solar panels, and hybrid electric vehicles in Japan and the United States and prevent the acquisition of clean coal technology by Chinese firms (Sovacool Citation2008). Many countries in the developing world, moreover, do not own the intellectual property rights for the newest or most efficient energy systems, meaning that they have to license Western technology to avoid reliance on fossil fuels, particularly in markets such as China and India. One assessment of the barriers facing energy systems to reduce greenhouse gases found that many firms were reluctant to distribute new energy technologies to developing markets for fear that their intellectual property rights would not be respected and enforced by the World Trade Organization and other relevant authorities (Brown et al. Citation2007).
Transboundary environmental externalities
The environmental externalities of current energy systems are threatening global energy security. One of the most compelling examples is the growing evidence of rapid climate change that threatens the entire planet with rising sea level, loss of species, and destruction of habitats. It is not, however, the only worldwide concern. Others include nuclear meltdowns, long-term storage of nuclear wastes, oil well blowouts, dam breaches, and the transport of toxic pollutants such as mercury and sulfuric acid across national borders. Each of these problems can cause chronic disease, morbidity, and mortality among humans, destroy crops, and damage ecosystems.
Another global challenge to energy security is how to maintain the quality and availability of water that is used in various energy processes. Although energy and water are addressed by almost entirely separate communities of policymakers, in fact the two are deeply intertwined. Energy fuel processing and generation cannot be accomplished without attention to water, as every single energy source and energy phase uses at least some water. In particular, thermoelectric power plants running on coal, natural gas, oil, and uranium are water-cooled, consuming billions of gallons of water from local aquifers, rivers, lakes, and oceans.
But just as water for energy may not be available, the opposite is also true. By 2025, demographers, geologists, and water managers anticipate that more than 60% of the global population will live in countries with significant imbalances between water supply and demand (Feeley et al. 2008). Nearly a billion people already lack adequate access to potable water, a figure that may rise to more than 3 billion by 2015. Either they cannot purify the water they can get, or they cannot get the water at all because of the increasing lifting costs that are resulting from the dropping level of aquifers. For example, water tables for major grain producing areas in northern China are dropping at a rate of 5 feet per year. For both of these reasons, per capita water availability in India is expected to drop 50–75% over the next decade (Pope Citation2005). There are many countries that are at the crisis level, including urban areas across Asia, parts of Mexico, the Ogallala aquifer in the Midwestern United States, and Saudi Arabia (Boberg Citation2005). Some of the driest and poorest countries completely reliant on water for agriculture also lack it. Ninety percent of water use in Egypt, Libya, and the Sudan support irrigation and agricultural systems, meaning droughts and shortages can cause widespread shortages of food (Gleick Citation2003). Energy security is entwined with the secure supply of other critical natural resources as well. Water is one of those resources.
Conclusions and policy recommendations
The purpose of this article has been to illustrate that energy security is an important consideration at every scale of consideration, to emphasize that energy policy must be adjusted to each scale to increase energy security, and to stimulate empirical studies into the scalar relationships between energy security and policy. The preceding discussion leads to the following summary observations and preliminary policy recommendations.
First, energy security is significant at every scale, and policy decisions have to adjust for this reality. For the most part, however, this has not been the case; energy policies tend to be considered within an individual level of security, rather than across them. For example, policies affecting the future of nuclear power in the US are taken at the national and global scales. Nonetheless, nuclear power policies have repercussions at every scale. This means that at the same time that a pro-nuclear policy might provide a sense of increased national independence and security, people at the community and household levels may constantly feel less secure, as we have recently noticed in response to the nuclear accident at Fukushima Daiichi, Japan. Energy policies must be considered in terms of their cross-scalar effects if they are to embrace both efficacy and equity.
Second, sensitivity to energy security varies nationally, from one country to another. Countries with multiple points of entry for critical energy supplies, for example, are usually less vulnerable than are countries that rely on only a few. Sometimes this variable vulnerability will be a function of climate, as where areas of colder climates are more sensitive to supply interruptions than those in warmer climates. Other times vulnerability will vary with the economic complexity of the country. For example, OECD countries tend to have critical energy needs, complex infrastructures, and numerous points of vulnerability as a result. However, such complexity also suggests that any supply interruptions will have impacts that will tend to be localized. The principal way to increase energy security at the national level is for countries to initiate policies that increase a diversity of energy supplies and an abundance of routes for energy delivery.
Third, energy security varies with the context not only at smaller scales but also on the national scale. For example, climate can quickly affect energy security at the household scale, as when the weather interrupts power supplies. In a typical office setting, energy security is usually a matter of producing a welcoming working environment. Outside the office, the significance of energy security rises as the critical function of the energy need goes up. For manufacturing processes, energy security can be high for some traditional products such as steel and aluminum, but critical for newer products such as semiconductors. In some jobs it is a matter of life and death, in terms of keeping the workplace safely lit or refrigerating vaccines. The national energy security needs of an Afghanistan or Nepal will differ greatly from a China or a United States. Establishing proper and effective policies for energy security must closely consider context if they are to be adequate and useful.
Fourth, energy security requires a nuanced approach to policy formation if threats and challenges are to be adequately conceptualized and addressed. As illustrates, worries about energy security abound at multiple scales, and that is how energy policies affecting energy security must be formulated. Energy security cannot be reduced to its constituent parts; rather, it is multi-scalar. Since energy security concerns and responses exist at many levels and different spatial scales, the relevant ideas and paradigms required to comprehend events or challenges at one level may not scale up or down to other levels. Scale matters; energy policies that consider only one scale ignore important dimensions at others and weaken energy security everywhere.
Table 2. Energy security scales, actors, and concerns.
While our study has mapped the relationship between scale and energy security, it has only hinted at the answers. Further research is needed to better investigate the dynamics of this complex affiliation. For example, is energy security a useful umbrella for all energy-related problems? Should the solutions to the energy security challenges identified here match the scales at which they occur, or should solutions transcend them? Are energy access policies best addressed by policy tools at the household level; energy intensity by policies at the industrial and consumer level; and global environmental change and nuclear weapons proliferation by appropriate global regimes at the international level? Or do we need predominantly national approaches and policies to these problems, or polycentric approaches that blend and combine separate scales together? Can existing policy goals in the field of energy security be achieved if the energy security policies continue to focus only at one scale? Put another way, are existing policies up to the task of the world's energy security challenges? Is there is a policy vacuum in certain energy-related areas and could this vacuum be most appropriately filled by expanding energy security policies? Answering these questions would enable researchers and policymakers to more fully comprehend the correlation between energy security, scale, and policy, and we encourage all readers of the journal to consider them.
Figure 1. US Natural Gas Supply Basins Relative to Major Natural Gas Pipeline Transportation Corridors, 2008. This complicated and extensive network is necessary for the energy security of large and robust countries such as the US. Its extensive nature also makes it vulnerable to accidental and intentional disruption.
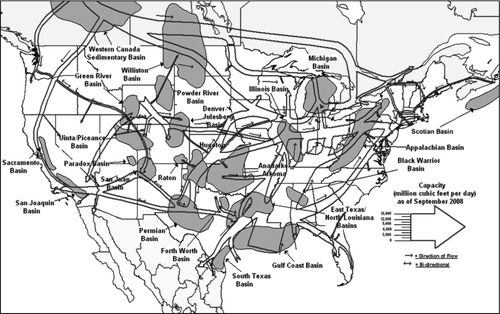
Notes
1. This was part of the discussion at a workshop held November 2009 at the Lee Kuan Yew School of Public Policy, National University of Singapore, with the sponsorship of the John D. and Katherine T. MacArthur Foundation.
2. While such a policy enhances energy security in the U.S., it is likely to result in a new scale of problems for Canada (Pasqualetti 2009).
3. Also contributing to the volatility of oil prices is the increasingly limited world oil surplus production capacity. In the 1990s, significant surplus capacity for world oil production existed in five or more countries: Saudi Arabia, United Arab Emirates, Iraq, Kuwait, Iran, and other OPEC countries. Since 2005, surplus oil production in the world has been limited essentially to one or two million barrels per day in Saudi Arabia. Energy Information Administration (EIA), Short-Term Energy Outlook, September 2006; [cited 2011 April 15]. Available from http://www.eia.doe.gov/pub/forecasting/steo/oldsteos/sep06.pdf.
References
- Andres , R B . 2009 . Energy and environmental insecurity . JFQ: Joint Force Q , 55 : 109 – 112 .
- ANL , [Argonne National Laboratory] . Energy transmission in the United States; [cited 2010 Jun 3] n.d, Available from: http://teeic anl gov/er/transmission/restech/dist/index cfm
- Auty , R M . 2004 . Natural resources and civil strife: a two-stage process . Geopolitics , 9 ( 1 ) : 29 – 48 .
- Barnes , D F and Willem , M F . 1996 . Rural energy in developing countries: a challenge for economic development . Annu Rev Energy Environ , 21 : 497 – 530 .
- Bambawale , M J and Sovacool , B K . 2011a . India's energy security: a sample of business, government, civil society, and university perspectives . Energy Policy , 39 ( 3 ) : 1254 – 1264 .
- Bambawale , M J and Sovacool , B K . 2011b . China's energy security: the perspective of energy users . Appl Energy , 88 ( 5 ) : 1949 – 1956 .
- Bambawale , M J and Sovacool , B K . 2011c . Sheikhs on barrels: what Saudi Arabians think about energy security . Contemp Arab Affairs , 4 ( 2 ) : 208 – 224 .
- Barton , B, . 2004 . Energy security: Managing risk in a dynamic legal and regulatory environment , Oxford : Oxford University Press .
- Bilgin , M . 2009 . Geopolitics of European natural gas demand: Supplies from Russia, Caspian and the Middle East . Energy Policy , 37 ( 11 ) : 4482 – 4492 .
- Bridge , G . 2004 . Mapping the bonanza: Geographies of mining investment in an era of neoliberal reform . Prof Geographer , 56 : 406 – 421 .
- Bridge , G . 2008 . Global production networks and the extractive sector: governing resource-based development . J Econ Geogr , 8 : 389 – 419 .
- Bridge , G and Wood , A . 2005 . Geographies of knowledge, practices of globalization: learning from the oil exploration and production industry . Area , 37 : 199 – 208 .
- Boberg , J . 2005 . Liquid assets: how demographic changes and water management policies affect freshwater resources , 20 Santa Monica , CA : RAND Corporation .
- Brown , M A and Sovacool , B K . 2011 . Climate change and global energy security: an overview of technology and policy options , Cambridge , MA : MIT Press .
- Brown , M A , Chandler , J , Lapsa , M V and Sovacool , B K . 2007 . Carbon lock-in: barriers to the deployment of climate change mitigation technologies , Oak Ridge : Oak Ridge National Laboratory. TN ORNL/TM-2007/124 .
- Coe , N M , Dicken , P and Hess , M . 2008 . Global production networks: realizing the potential . J Econ Geogr , 8 : 271 – 295 .
- CNA . 2009 . Powering America's defense: energy and the risks to national security. Alexandria , Maryland : CNA Corporation .
- CTA [International Center for Technology Assessment] . 2005 . Gasoline cost externalities: security and protection services [cited 2010 Jun 2] Available from: http://www icta org/doc/RPG%20security%20update pdf
- Ericson , R E . 2009 . Eurasian natural gas pipelines: the political economy of network interdependence . Eurasian Geogr Econ , 50 ( 1 ) : 28 – 57 .
- Feeley , T J , Skone , T J , Stiegel , GJ Jr , McNemar , A , Nemeth , M , Schimmoller , B , Murphy , J T and Manfredo , L . 2008 . Water: a critical resource in the thermoelectric power industry . Energy , 33 : 1 – 11 .
- Financial Times . 2008 . Lukoil stops German delivery . Financial Times , : 5 Feb 20
- Florini , A E and Sovacool , B K . 2011 . Bridging the gaps in global energy governance . Global Governance , 17 ( 1 ) : 57 – 74 .
- Garrity , T F . 2008 . Getting smart . Power Energy Mag , 6 ( 2 ) : 38 – 45 .
- Gleick , P H . 2003 . Water use. Annu Rev . Environ Resour , 28 : 275 – 314 .
- Hess , M and Yueng , H WC . 2006 . Whither global production networks in economic geography? Past, present, and future . Environ Plann A , 38, pp : 1193 – 1204 .
- Healy , H M . 2003 . “ Safety and security of indoor air quality (IAO) ” . In Energy & high performance facility sourcebook , Edited by: Buff , D L . 543 – 548 . Georgia : The Fairmont Press .
- Holdren , J P and Smith , K R . 2000 . “ Energy, the environment, and health ” . In World energy assessment: energy and the challenge of sustainability , Edited by: Kjellstrom , T , Streets , D and Wang , X . 61 – 110 . New York : United Nations Development Program .
- IEA (International Energy Agency) . 2009 . World energy outlook , Paris : OECD .
- Jackson , T . 1991 . Renewable energy: great hope or false promise? . Energy Policy , 19 ( 1 ) : 2 – 7 .
- Kalicki , J H and Goldwyn , D L . 2005 . “ Energy, security, and foreign policy ” . In Energy security – toward a new foreign policy strategy , Edited by: Kalicki , J H and Goldwyn , D L . 561–578 Baltimore : Johns Hopkins Press .
- Karl , T L . 1998 . “ State building and petro revenues ” . In The geopolitics of oil, gas, and ecology in the Caucasus and Caspian Sea Basin , Edited by: Garcelon , M , Walker , E W , Patten-Wood , A and Radovich , A . 3 – 14 . Berkeley , CA : Berkeley Institute of Slavic, East European, and Eurasian Studies .
- Karl , T L . 2000 . “ Crude calculations: OPEC lessons for the Caspian region ” . In Energy and Conflict in Central Asia and the Caucasus , Edited by: Ebel , R and Menon , R . 29 – 54 . New York : Rowman & Littlefield .
- Kessels , J , Bakker , S and Wetzelaer , B . 2008 . Energy security and the role of coal , 31 London : IEA Clean Coal Centre, CCC/131 .
- Klare , M T . 2007 . The futile pursuit of energy security by military force . Brown J World Aff , 13 ( 2 ) : 139 – 153 .
- Kleber , D . 2009 . The U.S. Department of Defense: valuing energy security . J Energy Security , : 12 – 22 . June
- Kleveman , L . 2003 . The new great game: blood and oil in Central Asia , New York : The Grove Press .
- Krishman , N , Williams , E D and Boyd , S B . 2008 . Case studies in energy use to realize ultra-high purities in semiconductor manufacturing; [cited 2011 Apr 15] Available from: http://ieeexplore ieee.org/stamp/stamp jsp?arnumber=04562913
- Legros , G , Ines , H , Nigel , B , Sophie , B , Kamal , R , Minoru , T and Carlos , D . 2009 . The energy access situation in developing countries: a review focusing on the least developed countries and sub-Saharan Africa , New York : World Health Organization and United Nations Development Program .
- Löschel , As , Ulf , M and Dirk , T GR . 2010 . Energy security – concepts and indicators . Energy Policy , 38 ( 4 ) : 1607 – 1608 .
- Lovins , A and Lovins , H . 1983 . Brittle power: energy strategy for national security , Andover (MA) : Brick House Publishing .
- Luft , G and Korin , A . 2009 . Turning oil into salt: energy independence through fuel choice , Washington (DC) : BookSurge Publishing .
- Masud , J , Diwesh , S and Bindu , N L . 2007 . Energy for all: addressing the energy, environment, and poverty nexus in Asia , Manila : Asian Development Bank .
- Miller , G D . 2010 . The security cost of energy independence . Washington Q , 33 ( 2 ) : 107 – 119 .
- Modi , V , McDade , S , Lallement , D and Saghir , J . 2005 . Energy services for the millennium development goals , Washington and New York : The International Bank for Reconstruction and Development/The World Bank and the United Nations Development Programme .
- Moy , G W . 2003 . “ Reducing the vulnerabilities of department of defense utilities and energy use ” . In Solutions for energy security & facility management challenges , Edited by: Joyce , W . Lilburn , Georgia : The Fairmont Press .
- Muller-Kraenner , S . 2007 . Energy security , London : Earthscan .
- Newton , J F . 2001 . Hospital power: critical care; [cited 2010 Jun 1] . Engineered Systems , Available from: http://www.esmagazine.com/Articles/Cover_Story/cdb6fde511ca8010VgnVCM100000f932a8c0
- Nye , D E . 2009 . When the lights went out: a history of blackouts in America , Cambridge , MA : MIT Press .
- Pamir , N . 2006 . Energy (In)Security and the most recent lesson: the Russia–Ukraine gas crisis , Ankara : Center for Eurasian Strategic Studies .
- Pascual , C , Elkind , J and editors . 2010 . Energy Security: economics politics, strategies, and implications , Washington , DC : Brookings Institution Press .
- Pasqualetti , M J . 2011 . “ The competing dimensions of energy security ” . In The Routledge energy security handbook. Chapter 14 , Edited by: Benjamin , S . 275 – 290 . London : Routledge .
- Pasqualetti , M J . 2009 . The Alberta oil sands from both sides of the border . Geographical Rev , 99 ( 2 ) : 248 – 267 .
- Pope , C . 2005 . The state of nature: our roof is caving . Foreign Policy. July/August. p , : 65 – 74 .
- Robinson , J A , Torvik , R and Verdier , T . 2006 . Political foundations of the resource curse . J Dev Econ , 79 : 447 – 468 .
- Sachs , J D and Warner , A M . 1999 . The big push, natural resource booms and growth . J Dev Econ , 59 : 43 – 76 .
- Smil , V . 2004 . “ War and energy ” . In Encyclopedia of energy , Edited by: Cleveland , C . 363 – 371 . New York : Elsevier .
- Sovacool , B K . 2008 . Placing a glove on the invisible hand: how intellectual property rights may impede innovation in energy research and development (R&D) . Albany Law J Sci Technol , 18 ( 2 ) : 381 – 440 .
- Sovacool , B K . 2009 . “Reassessing energy security and the trans-ASEAN natural gas pipeline network in Southeast Asia . Pacific Affairs , 82 ( 3 ) : 467 – 486 .
- Sovacool , B K and Brown , M A . 2010 . Competing dimensions of energy security: an international review . Annu Rev Environ Resour , 35 : 77 – 108 . November
- Sovacool , B K . 2011a . Evaluating energy security in the Asia Pacific: towards a more comprehensive approach . Energy Pol , 39 ( 11 ) : 7472 – 7479 .
- Sovacool , B K . 2011b . Seven suppositions about energy security in the United States . J Cleaner Prod , 19 ( 11 ) : 1147 – 1157 .
- Sovacool , BK , Mukherjee , I , Drupady , IM and D'Agostino , AL . 2011 . Evaluating energy security performance from 1990 to 2010 for eighteen countries . Energy , 36 ( 10 ) : 5846 – 5853 .
- US DoE [US Department of Energy] . The Smart Grid: An Introduction Prepared by Litos Strategic Communication under contract No DE-AC26-04NT41817 n.d, Subtask 560 01 04 (http://www.oe.energy.gov/DocumentsandMedia/DOE_SG_Book_Single_Pages. pdf) Accessed 6 Nov 2010
- UNDP [United Nations Development Programme] . 1997 . “ Energy and major global issues ” . In Energy after Rio: prospects and challenges , Edited by: Reddy , AKN , Williams , RH and Johansson , TB . 111–145 New York : UNDP .
- UNESCAP [United Nations Economic and Social Commission for Asia and the Pacific] . 2008 . Energy security and sustainable development in Asia and the Pacific , Oxford : UNESCAP . ST/ESCAP/2494 185
- UN [United Nations] [Internet] . 2008 . Energy security and sustainable development in Asia and the Pacific. United Nations Bangkok [cited 2012 May 23] Available from: http://www.unescap.org/esd/publications/energy/theme_study/energy-security-ap.pdf
- US [United States Congress] . 2009 . Energy security: historical perspectives and modern challenges , Washington , DC : GPO .
- Watts , M, editor . 2010 . Curse of the Black Gold: 50 years of oil in the Niger Delta , Brooklyn (NY) : PowerHouse Books .
- Williams , E D , Ayres , R U and Heller , M . 2002 . The 17 kilogram microchip: Energy and material use in the production of semiconductor devices . Environ S Technol , 36 ( 24 ) : 5504 – 5510 .
- Worrell , E , Galitsky , C and Price , L . 2008 . Energy efficiency improvement opportunities for the cement industry , Berkeley (CA) : Environmental Energy Technologies, Division Lawrence Berkeley National Laboratory .