Abstract
Methane is produced in anaerobic environments by obligate anaerobic microorganisms through the decomposition of organic matters. To investigate the regression relationships between methane emissions and soil microbes in a double-rice paddy under various field practices, a two-year study was conducted to investigate the seasonal variation of methane emissions and the total activities of soil microbes (TASM) as well as the populations of methanogens (PMET) using the static closed-chamber-GC (gas chromatography) and the most probable number methods. Seven management practices were included in this study to look at the average effect of field treatments on methane emissions and TASM as well as PMET, viz. CWS (conventional tillage + without straw residues + urea), NWS (no tillage + without straw residues + urea), SCU (conventional tillage + without straw residues + controlled-release urea), HN (high stubbles + no tillage + urea), HC (high stubbles + conventional tillage + urea), SN (straw cover + no tillage + urea) and SNF (straw cover + no tillage + urea + continuous flooding). The daily average values of methane emissions and TASM as well as PMET from seven treatments were used for the analysis. Regression analysis was conducted using the R statistical software. Different field practices have significant effect on methane flux and TASM and PMET and similar seasonal variations of methane flux and TASM as well as PMET were found during the rice-growing seasons. Pronounced positive correlations between methane flux and TASM, and PMET were observed. Such relationships can be well described by the exponential or quadratic polynomial models, respectively. Regression analysis indicated that PMET could explain individually at least 97% of variance of methane flux (R2 = 0.97, P < 0.001), while the fitting precision of multiple nonlinear regression model for methane flux with two predictors of TASM and PMET was slightly higher than the univariate regression analysis (R2 = 0.98, P < 0.001). However, as we know, methane emissions from paddy soils are affected by many factors, of which TASM and PMET are the most direct influential variants. In order to reasonably reveal the interactions between methane emissions and environmental factors, the multivariate nonlinear regression analysis should be carried out based on data derived from the extensive field experiments rather than few laboratory trials.
Introduction
Methane (CH4) is the most important non-carbon dioxide (CO2) greenhouse gas (GHG) and the key contributor of greenhouse effect in double-rice fields (Akiyama et al. Citation2005; Minamikawa et al. Citation2006). The concentrations of the atmospheric methane has been increasing since the industrialization, and, to date, the annual increase rates of methane can still not be neglected, i.e. 1.1% (Guo and Zhou Citation2007). Besides, as the most important agricultural source of CH4, rice (Oryza sativa L.) contributes 60 Gt CH4 yr−1, accounting for 10%–30% of its global budget (Bouwman Citation1990).
The production of methane from soil is the result of the activity of various groups of microorganisms including zymogenic bacteria and methanogenic archaea (Woese et al. Citation1978), which are the dominative microbes for the formation of methane. The metabolism of these different microbial communities completes the degradation of organic carbon from large molecules to the most reduced status like methane (Chidthaisong and Watanabe Citation1997; Hou et al. Citation2000; Le Mer and Roger Citation2001). The other two procedures that are also included in the emissions of methane from soil to atmosphere (Schütz et al. Citation1989) are: reoxidation and transportation. Both production and oxidation of methane are closely related to the populations and activities of soil microbial communities. The methanogenic archaea has comparatively large quantities, by 2000, 26 types and 60 kinds of methanogens have been found (Garcia et al. Citation2000). As soils like flooded rice paddy field and other wetlands provide favorable environment for methanogens, the paddy soils are normally expressed as a major source of methane emissions. Therefore, a remarkable close relationship between methane emissions and soil microorganisms may exist in the rice fields (Le Mer and Roger Citation2001). Because there are great spatial variations for soil and climate characteristics in different study areas (Khalil et al. Citation1998), the interacted effects of soil microbes and other environmental factors on methane emissions should be necessarily and fully addressed to clarify the mechanism of CH4 emissions from paddy fields based on long-term in situ observations on typical croplands.
The researches on the correlation between methane flux and populations of methanogens (PMET) have been focused for a number of years, but most of them were carried out either in the laboratory (Peter Mayer and Conrad Citation1990; Gu et al. Citation2008; Zheng et al. Citation2008; Chen et al. Citation2009; Ma and Chen Citation2010) or in the pots of micro-plot (Chen et al. Citation2001). The direct field experiment investigations are very few (Hou et al. Citation2000; Yue et al. Citation2003). Furthermore, most of these studies were based on short-term observations, and few experiments lasted for more than two years (Hutsch et al. Citation1993). In addition, researches of the correlation between methane emissions and total activities of soil microbes (TASM) are also lacking. Most of the previous researches concerned only one factor that affects the emissions of methane from paddy fields (Hou et al. Citation2000; Yue et al. Citation2003), and also few efforts have been conducted to combine multiple field practices and multivariate analysis simultaneously. While, the complexity of methane evolution from soils to the atmosphere is to be fully investigated, a long-term comprehensive field experiment should be implemented. Hence, the aim of this study was to investigate the regression relationships between methane emissions and biological features of soil microorganisms in a double-rice field under various management practices, based on a field trial of five rice growing seasons.
Materials and methods
Site description and experiment establishment
The experiment was conducted at the agricultural station of Gansha town (113°11′E 28°8′N), Changsha county, Hunan province, China (). The site has a subtropical humid monsoon climate. The mean annual air temperature is 17.5°C; the cumulative temperatures of greater than 10°C is 5450°C day; and the mean annual sunshine and annual precipitation are 1700 h and 1300–1400 mm, respectively. The paddy soil studied is classified as the sandy clay developed from Quaternary red clay. The basic soil physicochemical characteristics are: total nitrogen (N): 2.14 g kg−1, soil organic matter: 32.7 k kg−1, hydrolysis N: 233.3 mg kg−1, effective phosphorus: 11.1 mg kg−1, soil pH: 5.3. The cropping rotation at the study site is early rice – late rice – fallow. Following the basal fertilizer incorporation into the field in the end of April, the early rice is transplanted and harvested in the early July. For the late rice, it is transplanted in the middle of July, following its basal fertilizer application and harvested in the middle of October. The geographic location of the study site is presented in . The climate data were obtained from the weather station of meteorological bureau of Changsha County near the site ().
Figure 2. Daily air temperature and precipitation of the experimental site in the rice-growing season of 2008–2009.
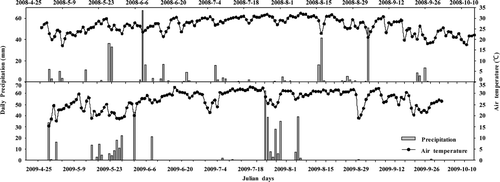
Seven treatments for various management practices were setup with three replicates, and they are: (1) CWS (conventional tillage + without straw residues + urea application), (2) NWS (no tillage + without straw residues + urea application), (3) SCU (conventional tillage + without straw residues + controlled-release urea application), (4) HN (high stubbles retained + no tillage + urea application), (5) HC (high stubbles retained + conventional tillage + urea application), (6) SN (straw mulching + no tillage + urea application), and (7) SNF (straw mulching + no tillage + urea application + continuous flooding), for water management, intermittent irrigation plus mid-season drainage was used for all the other six treatments. Totally 21 field plots were established by a randomized block design, and the dimension of each plot area is 15.6 m2. The detailed management practices are described in .
Table 1. Design of the field experiment (kg ha- 1).
Methane sampling and analysis
The observation of methane emissions from soils was carried out during the rice-growing seasons of 2008–2009 using the static chamber and gas chromatography (GC) method (Qin et al. Citation2006). Methane flux was measured in the frequency depending on rice-growing stage, e.g. seedling stage, tillering stage, before, during and after mid-season drainage, heading stage and ripe stage, generally seven times per season; consequently, during the two years, totally 22 effective methane fluxes were determined during four rice-growing seasons. The manually transparent closed chamber with open bottom was made of polycarbonate (0.5 mm in thickness) with a stainless steel frame; a schematic map of the chamber is described in . For each plot, one base frame was placed permanently in the field for observation during the whole season; one chamber was placed and took out on every sampling day; four hills of rice plants were included in the base frame. The gas samples were collected during 9:00 to 11:00 am by a 60 ml syringe at 0, 10, 20 and 30 min after the chamber was closed, some water was injected into the water tank for seal of whole system during the closure. About 30 ml air sample was then immediately transferred into a pre-evacuated vial (12 ml in volume) with rubber stoppers. This sampling time was based on the diurnal variation pattern of methane emissions (Hou et al. Citation2000). The air temperature inside the chamber was auto logged during gas collection using the Hobo data recorder (HOBO Pro-U23003, Onset Inc., USA, 2007), which was equipped into field before placement of the base frame until harvest of rice. The gas sample in the vial was stored for analysis by GC (Agilent 7980A, Agilent Inc., USA, 2007) in the lab within a few hours. The GC was equipped with a flame ionization detector (FID) for analyzing the concentration of methane. The column was packed with 80–100 mesh porapack Q. The detector and column were operated at 200°C and 70°C, respectively. A purified nitrogen gas (N2, > 99.999%) was used as carrier gas with flow rate of 30 ml min−1. Standard gas of methane was provided by China national research center of standard material. Flux of methane was determined from the slope of the mixing ratio change in four samples collected after the chamber was closed. Sample sets were rejected unless they yielded a linear regression value of R2 greater than 0.90. Daily average flux and standard error of methane were calculated from triplicate plots. Then, the mean methane flux of seven treatments was calculated with standard error, and was used for the synthetic analysis to see the average effect of these practices on methane emissions.
Soil sampling and microbiological analysis
Soil samples were collected at the same frequency of the methane sampling for analyzing TASM and the PMET. Extra soil samples were also collected during the fallow periods before the tillage in early 2008 in comparison with soil samples in the rice-growing seasons. By removing the topsoil of 1–2 cm, approximately 1 kg fresh soil at the depth of 5–15 cm was collected and stored in a desterilized plastic bag by a soil auger, which is also desterilized using ethanol in situ. Then, soil samples of each plot were transported to the laboratory immediately for soil biological analysis. The daily average values of TASM and PMET and their standard errors were calculated from three replicates, and subsequently the overall means and standard errors of TASM and PMET for seven treatments were used for the regression analysis.
The methods for microbiological analysis are described here. The alkali absorption method (Yim et al. Citation2002) for the total activities of soil microorganisms was used to determine the quantity of CO2 respiration of soil to represent the activity of soil microbes. The most-probable-number (MPN) method (Qian and Min Citation1986; Papen and von Berg Citation1998) for PMET was used to enumerate the methanogenic bacteria. First, 0.5 ml of the soil solution was incubated in three vials, which contained with 4.5 ml methanogenic culture medium. Secondly, the incubated soil solutions were then diluted into six continuous concentration magnitudes of 10−2, 10−3, 10−4, 10−5, 10−6 and 10−7, and subsequently the mixed solutions composed of 0.1% Na2S and 5% NaHCO3 with 0.1 ml penicillin (50 mg ml−1) were injected into the dilutions. Eventually, the vials were incubated at 30°C for 10 d, and then CH4 bacteria were accounted.
Data analysis
The non-linear regression of the mean values of methane flux (MF) with the mean values of soil TASM and PMET of seven treatments and the related correlation analysis were carried out by using R software (R Development Core Team Citation2010). Two-way analysis of variance with no repetition (two-way ANOVA) in Microsoft Excel (Version 2010) was used for the statistical analysis of the effect of different treatments on daily values of MF, TASM and PMET during the two years. All the data were demonstrated in the form of mean ± standard error. Sigmaplot (Version 10, Systat Software Inc., USA), R (Version 2.13.1) and Arcview GIS desktop (Version 3.2, ESRI Inc., USA) were used for graph plotting and geographic mapping.
Results
Effect of different field practices
The average value of methane flux, TASM and PMET of each treatment during the two-year observation are presented in . Among the seven treatments, SNF caused maximal methane emissions, 27.30 mg m−2 h−1, and NWS induced less methane flux than the others, 5.14 mg m−2 h−1. While the largest value of TASM and PMET was due to SCU and HN, it was 1.0 mg CO2 d−1 g dry soil−1 and 8.37 × 104 cell g dry soil−1, respectively. However, similar trend of TASM and PMET with the variation of MF could still easily been found in , which indicates that strong relationship may exist between methane emissions and TASM and PMET under the effect of varied practices. The results of the two-way ANOVA () confirmed this conclusion, i.e. both sampling date (P < 0.05 or P < 0.01) and field treatments have a strong effect on methane flux, TASM and PMET during the two-year observation, especially for the treatments, which have the extremely significant influence on the variation of MF, TASM and PMET (P < 0.001).
Figure 4. Effect of different field practices on methane flux (MF) and total activities of soil microbes (TASM) and the populations of methanogens (PMET) from double-rice paddy. The definition of each practice is demonstrated in . The data are the average daily observed value of MF, TASM, and PMET during the two years.
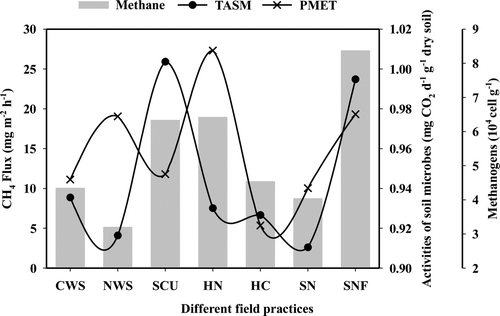
Table 2. Results of the two-way ANOVA.
Furthermore, the relationship between methane flux and TASM as well as PMET for each treatment was analyzed by correlation analysis (). Almost all the correlation coefficients of the paired data are greater than 0, except MF_NWS and PMET_NWS. From the correlation coefficient value in , we also can see that the correlation relationship of MF and PMET is stronger than that of MF and TASM, which means that the emitted methane depended more on the amount of soil methanogens than the total activities of all soil microbes.
Figure 5. Correlation coefficient matrix of methane flux (MF) and total activities of soil microbes (TASM) and the populations of methanogens (PMET) under the effect of different field practices. (A) MF with TASM and (B) MF with PMET. The definition of each practice is demonstrated in . The data for the correlation analysis of MF, TASM, and PMET are the observed daily values of each practice during the two years.
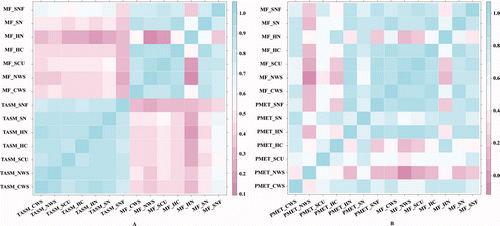
Seasonal variation
As analyzed above, there was strong positive relationship between methane emissions and the variation of soil TASM and PMET as influenced by different field managements. To investigate the general correlation between methane flux and TASM as well as PMET in a double-rice field, we use the average value of varied treatments to disclose this regularity.
Under the synthetic effects of seven field practices, pronounced average seasonal pattern was observed for the methane emissions and the performance of soil microbial communities during the two-year period. Methane flux observed in the two years ranged from 0.25 to 100.59 mg m−2 h−1, and a consistent temporal trend of methane emissions with the TASM and PMET was found. During the early rice-growing stage of each season, TASM and PMET were both low. When the rice grew and the fertilizers were applied, soil microorganisms were getting active, especially at the tillering stage, when the highest methane emissions occurred and accompanied with very strong activities of soil bacteria, which was stimulated by the rhizospheric exudation of rice plant. Different patterns of methane emissions occurred at the late growing stages (after the mid-season drainage): the methane emissions decreased sharply, and lower activities of soil anaerobes and PMET were observed until harvest. The average TASM of seven treatments during two years ranged from 0.38 to 1.42 mg CO2 d−1 g dry soil−1, with a mean value of 0.91 mg CO2 d−1 g dry soil−1. However, the TASM sampled in the fallow before the 2008 early rice was just 0.66 mg CO2 d−1 g dry soil−1, much lower than that of the rice-growing seasons. Additionally, the average values of PMET for rice-growing seasons and winter fallow periods were 5.46 × 104 cell g dry soil−1 and 0.89 × 104 cell g dry soil−1, respectively. This suggested that the growth of rice plants in the paddy fields stimulated the behavior of soil microorganisms. A similar seasonal pattern of methane emissions with the two microbial indexes was observed, this also proved the important role of rice plant growth for seasonal transportation of methane to the atmosphere. This seasonal emission pattern was similar to the findings in central China (Ahmad et al. Citation2009), Japan (Minamikawa and Sakai Citation2005), and the USA (Wassmann et al. Citation1994). This pattern is likely related to temperature change, soil moisture regime, soil redox condition, as well as rice growing stage (Hou et al. Citation2000).
Multivariate regression analysis
Various field managements could cause different methane emissions from rice fields, especially water managements and mid-season drainage have been widely suggested as a key factor in reducing methane flux. In this study, there were two water regimes that have been designed: mid-season drainage (the first six treatments) and continuous flooding (SNF), so we calculated separately by the water management among the seven practices. Consequently, the relationship of methane flux and TASM as well as PMET was analyzed by multivariate regression model with regard to the water using measurement: mid-season drainage (MSD) (the average value of all the treatments with MSD), continuous flooding (the treatment of SNF) and general situation (the average value of all the seven treatments).
For the general situation, a significant positive correlation was found between methane flux and TASM (r = 0.80, P < 0.0001, n = 22) and PMET (r = 0.70, P < 0.001, n = 22). Simple regression model was calculated for methane flux and TASM and also for methane and PMET by univariate regression analysis of R program (). Their relationships were markedly represented by the exponential or quadratic polynomial models, respectively. But obviously, methane emission was correlated much closer with PMET (R2 = 0.97) than TASM (R2 = 0.31). This indicated that PMET could explain almost all the variance (97%) of methane emissions from the paddy fields. But if the two factors were considered simultaneously, the mixed binary exponential and polynomial regression models could explain more variance of methane flux (R2 = 0.98), compared with the univariate regression analysis. In fact, a pronounced correlation existed between TASM and PMET (rho = 0.63, P < 0.01, n = 22), indicating that there was a very important effect of both TASM and PMET on methane production and transportation. Therefore, the multivariate regression model could be more useful to describe the mechanism of methane emissions from the double-rice paddy fields rather than the univariate regression analysis. As a comparison, significant regression model was also found in the MSD and SNF situation ().
Table 3. Results of monadic and multivariate regression analyses.
Furthermore, for the criterion of regression analysis, the R2 of the monadic model of MF and TASM in MSD situation is greater than that of the value in general situation, while the R2 of quadratic polynomial model of MF and PMET is lesser than that of the value in general situation. Similarly, the R2 of the monadic model of MF and TASM in SNF situation is greater than the value in general situation, and the regression coefficient of MF and TASM in SNF situation is also greater than the value of general situation, this means that the soil microbial activities favored more in the continuous flooding environment than the other two water management conditions. As for the significance of these regression models, all of them were at least significant at 0.01 levels ().
Discussion
Influence of field managements on methane emissions
The emission of methane from soil to atmosphere is generally affected by many factors, such as soil microbial community, available organic matter, soil moisture, temperature, redox potential and field managements. In this study, seven treatments were designed (), which include fertilization, irrigation, tillage and organic matter management, all of which may have different effects on methane emissions, variation of TASM and PMET. As presented in , when varied practices being considered, the NWS affected less methane emissions than the others, due to no additional organic matter input and with normal irrigation regime, while the largest methane average flux occurs in SNF, which may primarily be attributed to the continuous flooding water management. As a direct comparison, the methane emitted by SN was lesser than SNF; the only difference in these two treatments is the water application, which emphasizes again that MSD may be a good choice to mitigate methane emissions from a double-rice paddy as introduced by Smith et al. (2007, 2008) and Qiu (Citation2009). Additionally, there were four treatments about the organic matter usage, i.e. CWS, NWS, HN and HC. Besides NWS, we found that the methane emissions of the practices with high stubble retained after harvest (HN and HC) was greater than that of straw covering with or without tillage performed (CWS and NWS). This means that compared to rice residue recovering the ‘high stubble retained’ enhanced the pathway of methane transfer from soil to atmosphere by the aerenchyma of rice plant especially in the early stage of every rice-growing season. Among the seven treatments, the SCU used controlled-release urea (CRU) instead of normal urea, as a new fertilizer style, CRU could enhance rice growth and inhibit the nitrous oxide (N2O) emissions from rice paddy (Li et al. Citation2004), but as investigated by Li and Fan (Citation2005), CRU led to greater methane emissions than normal urea like that discovered in this study (); we found methane flux from SCU was greater than CWS, the only difference in these two treatments is the chemical fertilizer application, which explained that the long-term released nitrogen nutrient offered perdurable substances for methane emissions than normal urea. In another investigation by Delgado and Mosier (1996), no difference was found in methane emissions between normal urea and CRU, which indicated that varied soil and climate conditions may cause distinct results. To conclude, different field managements may lead to various methane emissions, both in patterns and quantities; the major challenge to quantify the methane emissions from soil is its great variance in both temporal and spatial horizon. Consequently, the research network of methane emissions include different climate, and soil types should be further considered.
Temporal patterns of methane emissions and TASM and PMET
Methane is initiated from the complicated decomposition procedure of organic substrates by various kinds of methanogenic archaea in an anaerobic environment, which occupies tremendous area of the earth, such as water logged wet land and irrigated double-rice fields as described in this study (Le Mer and Roger Citation2001). It is very difficult to understand how the activities and populations of soil methanogens around the rhizosphere of rice plants remarkably affect the production, oxidation and transportation of methane as demonstrated by some previous studies conducted in the laboratories (Peter Mayer and Conrad Citation1990; Gu et al. Citation2008; Zheng et al. Citation2008 Chen et al. Citation2009; Ma and Chen Citation2010). But the condition of the field was totally different from the environment of laboratory and only few conclusions were documented based on field experiments (Hou et al. Citation2000; Yue et al. Citation2003). It is well known that most of the methane emissions were generated during the flooding period before mid-season drainage in double-rice fields (Qin et al. Citation2006); several explanations may be given for the higher methane emissions in this stage (Qin et al. Citation2010). Firstly, rapid decomposition of organic matter and fertilizer during flooding offered sufficient source of methanogenic substrates, and then stimulated methane production. Furthermore, soil microbial communities and their activities may be changed by the evolution of rice rhizosphere and the nutrient supply, the microflora mentioned include methanogenic archaea, which can change depending on the rice growth and decomposition of organic matters. Relatively, in the late stage of rice-growing season (after mid-season drainage, even the continuous flooding treatment (SNF) only contained shallow water layer), the soil water content and soil air-filled porosity were much lower and greater than the early stage, respectively, which caused significantly oxidised environment which favored the methanotrophy instead of methanogens and then consumed most of the methane formatted in the rice rhizosphere zone. Consequently, seasonal variation of methane emissions obeyed similar regularity with TASM and PMET under the influence of rice growth stage. In addition, the different patterns of methane emissions before and after mid-season drainage suggested that MSD is regarded potentially as a promising option for the mitigation of methane emissions from double-rice paddy fields as documented in the study of Shang et al. (2011).
Regression relationships of methane emissions and TASM and PMET
We found a nonlinear relationship of methane flux and TASM and PMET and their multivariate regression model (), and the determining coefficient of the multivariate model was much greater than the univariate models; thus, the former analysis method should be considered for the similar mechanism research. We did not analyze the methanotrophy in this study, but we found that PMET could explain 97% of the variance of methane flux in the flooded rice fields, suggesting that the methanogenic archaea dominated the balance of “production-reoxidation” of methane emissions from soil, and we got greater coefficient of determination if TASM and PMET were used together to explain the variance of methane emissions (). Consequently, in the irrigated double-rice fields, the long-term methanogenesis favored the obligately anaerobic bacteria and determined the methane flux. Chen et al. (Citation2001) pointed out that there was almost no obvious methane emission if the PMET was lower than 104 cell g dry soil−1. Another research in a rice field of Shenyang of Liaoning province in northeast China only observed the significant correlation between methane flux and the logarithm numbers of zymogenic bacteria instead of methanogens (Hou et al. Citation2000). This was not coincident with our results (), but it is easy to understand that the temporal and spatial variation of soil and climatic characteristics caused the various outcomes from different studies, and this variation also offered a great challenge for the quantitative researches of methane flux from soils (Wachinger et al. Citation2000). Another similar study in the rice paddy fields in northeast China also offered a simple regression model of methane emissions and methanogens (Yue et al. Citation2003). All of these studies provided a strong dependency of methane emissions on TASM and PMET, even in different patterns, existed.
Multiple practices should be considered simultaneously when trying to find a way to abate methane emissions from rice paddy, such as water regime, fertilization method and tillage performance. In this study, water management plays a key role among those agricultural activities, because of the much more methane flux of SNF than the other treatments (). So, multiple regression analysis was carried out depending on the water application method to the field. In spite of different R2 discovered in different situations when univariate or multivariate regression was conducted, a strong significance of regression relationship still exists between methane flux and TASM as well as PMET in the form of exponential or polynomial ().
Conclusions
Various field activities have remarkable effects on methane flux and TASM as well as PMET. Under the synthetic influence of different field managements, significant similar seasonal variations of methane emissions and TASM and PMET were observed. The methane flux, TASM and PMET varied from 0.25 to 100.59 mg m −2 h−1, 0.38 to 1.42 mg CO2 d−1 g dry soil−1 and 0.13 × 104 to 18.50 × 104 cell g dry soil−1, respectively in two years. Pronounced nonlinear positive correlations existed between methane flux and TASM and PMET. These correlations could be represented as exponential or quadratic polynomial regression models. Multivariate nonlinear regression analysis should be conducted when treated with the mechanism research of methane emissions from double-rice field.
Acknowledgements
We thank the hard work of the reviewers. Thank the financial support by the project of “Non-Profit Research Foundation for Agriculture (201103039)”, the “National Basic Research Program of China (2012CB417106)”, and the “Basic research fund of non-profit institute (2012ZL027)”.
References
- Ahmad , S , Li , C , Dai , G , Zhan , M , Wang , J , Pan , S and Cao , C . 2009 . Greenhouse gas emission from direct seeding paddy field under different rice tillage systems in central China . Soil Tillage Res , 106 : 54 – 61 .
- Akiyama , H , Yagi , K and Yan , X . 2005 . Direct N2O emissions from rice paddy fields: summary of available data. Global Biogeochem Cycl , 19: GB1005 .
- Bouwman , A . 1990 . “ Exchange of greenhouse gases between terrestrial ecosystems and the atmosphere ” . In Soil and the greenhouse effect , Edited by: Bouwman , A F . 62 – 127 . New York , NY : John Wiley and Sons .
- Chen , Z Y , Min , H , Chen , M C and Zhao , Y H . 2001 . Studies on relationships among methane emission and methane-oxidizing and methanogenic bacteria in three types of rice-field soil . Acta Ecologica Sinica , 21 ( 9 ) : 1498 – 1505 . (in Chinese with English abstracts)
- Chen , Z , Yuan , H C , Wu , J S and Wei , W X . 2009 . Activity and composition of the denitrifying bacterial community respond differently to long-term fertilization . Acta Ecologica Sinica , 29 ( 11 ) : 5923 – 5929 . (in Chinese with English abstracts)
- Chidthaisong , A and Watanabe , I . 1997 . Methane formation and emission from flooded rice soil incorporated with 13C-labeled rice straw . Soil Biol Biochem , 29 : 1173 – 1181 .
- Garcia , J L , Patel , B KC and Ollivier , B . 2000 . Taxonomic, phylogenetic, and ecological diversity of methanogenic archaea . Anaerobe , 6 : 205 – 226 .
- Gu , Y F , Zhang , X P , Tu , S H , Sun , X F and Lindström , K . 2008 . Effect of long-term fertilization on nitrification and nitrobacteria community in a purple paddy soil under rice–wheat rotations . Acta Ecologica Sinica , 28 ( 5 ) : 2123 – 2130 . (in Chinese with English abstracts)
- Guo , J and Zhou , C . 2007 . Greenhouse gas emissions and mitigation measures in Chinese agroecosystems . Agric Forest Meteorol , 142 : 270 – 277 .
- Hou , A X , Chen , G X , Wang , Z P , Van , Cleemput O and Patrick , WH Jr . 2000 . Methane and nitrous oxide emissions from a rice field in relation to soil redox and microbiological processes . Soil Sci Soc Am J , 64 : 2180 – 2186 .
- Hutsch , B W , Webster , C P and Powlson , D S . 1993 . Long term effects of nitrogen fertilization on methane oxidation in soil of the broadbalk wheat experiment . Soil Biol Biochem , 25 ( 10 ) : 1307 – 1315 .
- Khalil , M AK , Rasmussen , R A , Shearer , M J , Chen , Z L , Yao , H and Yang , J . 1998 . Emissions of methane, nitrous oxide, and other trace gases from rice fields in China . J Geophys Res , 103 : 25241 – 25250 .
- Le , Mer J and Roger , P . 2001 . Production, oxidation, emission and consumption of methane by soils: a review . Eur J Soil Biol , 37 : 25 – 50 .
- Li , F M and Fan , X L . 2005 . Effect of controlled release fertilizers on methane emission from paddy field . China J Appl Environ Biol , 11 ( 4 ) : 408 – 411 .
- Li , F M , Fan , X L , Liu , F and Wang , Q . 2004 . Effects of controlled release fertilizers on N2O emission from paddy field . Chin J Appl Ecol , 15 ( 11 ) : 2170 – 2174 .
- Ma , Y and Chen , Z L . 2010 . Effects of enrofloxacin on molecular diversity of nitrous oxide reductase genes (nosZ) . Acta Ecol Sin , 30 ( 4 ) : 1011 – 1017 . (in Chinese with English abstracts)
- Minamikawa , K and Sakai , N . 2005 . The effect of water management based on soil redox potential on methane emission from two kinds of paddy soils in Japan . Agric Ecosyst Environ , 107 : 397 – 407 .
- Minamikawa , K , Sakai , N and Yagi , K . 2006 . Methane emission from paddy fields and its mitigation options on a field scale . Microb Environ , 21 : 135 – 147 .
- Papen , H and von Berg , R . 1998 . A most probable number method (MPN) for the estimation of cell numbers of heterotrophic nitrifying bacteria in soil . Plant Soil , 199 : 123 – 130 .
- Peter Mayer , H and Conrad , R . 1990 . Factors influencing the population of methanogenic bacteria and the initiation of methane production upon flooding of paddy soil . FEMS Microbiol Lett , 73 ( 2 ) : 103 – 111 .
- Qian , Z S and Min , H . 1986 . Microbiology of biogas fermentation , Hangzhou : Zhejiang Science and Technology Press . (in Chinese with English abstracts)
- Qin , X B , Li , Y E , Liu , K Y and Wan , Y F . 2006 . Methane and nitrous oxide emission from paddy field under different fertilization treatments . Trans Chin Soc Agric Eng , 22 ( 7 ) : 143 – 148 . (in Chinese with English abstracts)
- Qin , Y , Liu , S , Guo , Y , Liu , Q and Zou , J . 2010 . Methane and nitrous oxide emissions from organic and conventional rice cropping systems in Southeast China . Biol Fert Soils , 46 : 825 – 834 .
- Qiu , J . 2009 . China cuts methane emissions from rice fields . Published online 18 August 2009, Nature News , doi: 10.1038/news. 2009.833
- R Development Core Team . 2010 . R Development Core Team R: a language and environment for statistical computing , Vienna , , Austria : R Foundation for Statistical Computing .
- Schütz , H , Holzapfel-Pschorn , A , Conrad , R , Rennenberg , H and Seiler , W . 1989 . A 3-year continuous record on the influence of daytime, season, and fertilizer treatment on methane emission rates from an Italian rice paddy . J Geophys Res , 94 : 16405 – 16416 .
- Shang , Q , Yang , X , Gao , C , Wu , P , Liu , J , Xu , Y , Shen , Q , Zou , J and Guo , S . 2011 . Net annual global warming potential and greenhouse gas intensity in Chinese double rice-cropping systems: a 3-year field measurement in long-term fertilizer experiments. Global Change Biol . J Geophys Res , 17 ( 6 ) : 2196 – 2210 .
- Smith , P , Martino , D , Cai , Z , Gwary , D , Janzen , H , Kumar , P , McCarl , B , Ogle , S , O'Mara , F and Rice , C . 2007 . Policy and technological constraints to implementation of greenhouse gas mitigation options in agriculture . Agric Ecosyst Environ , 118 : 6 – 28 .
- Smith , P , Martino , D , Cai , Z , Gwary , D , Janzen , H , Kumar , P , McCarl , B , Ogle , S , O'Mara , F and Rice , C . 2008 . Greenhouse gas mitigation in agriculture . Phil Trans R Soc B: Biol Sci , 363 : 789 – 813 .
- Wachinger , G , Fiedler , S , Zepp , K , Gattinger , A , Sommer , M and Roth , K . 2000 . Variability of soil methane production on the micro-scale: spatial association with hot spots of organic material and archaeal populations . Soil Biol Biochem , 32 : 1121 – 1130 .
- Wassmann , R , Neue , H U , Lantin , R S , Aduna , J B , Alberto , M CR , Andales , M J , Tan , M J , van der Gon , HACD , Hoffmann , H Papen , H . 1994 . Temporal patterns of methane emissions from wetland rice fields treated by different modes of N application . J Geophys Res , 99 : 16457 – 16462 .
- Woese , C R , Magrum , L J and Fox , G E . 1978 . Archaebacteria . J Mol Evol , 11 : 245 – 252 .
- Yim , M H , Joo , S J and Nakane , K . 2002 . Comparison of field methods for measuring soil respiration: a static alkali absorption method and two dynamic closed chamber methods . Forest Ecol Manage , 170 : 189 – 197 .
- Yue , J , Huang , G H , Liang , W , Jiao , Z H , Liang , Z B , Wang , C R and Shi , Y . 2003 . Relationship between CH4 and N2O emissions and related microorganism populations in paddy soils under different water management regimes . Chin J Appl Ecol , 14 ( 12 ) : 2273 – 2277 . (in Chinese with English abstracts)
- Zheng , J F , Zhang , P J , Pan , G X , Li , L Q and Zhang , X H . 2008 . Effect of long term different fertilization on methane oxidation potential and diversity of methanotrophs of paddy soil . Acta Ecol Sinica , 28 ( 10 ) : 4864 – 4872 . (in Chinese with English abstracts)