Abstract
Biodiesel is increasingly used as a fuel in transportation. It is generally considered an environmentally friendly alternative for diesel from fossil oil, because of lower emissions of the greenhouse gas carbon dioxide (CO2). However, nitrous oxide (N2O) emissions during the growth of energy crops can be considerable. N2O is emitted as a result of fertiliser use, needed to cultivate the energy crops. Fertiliser use not only increases the direct agricultural soil emissions, but also the indirect N2O emissions from aquatic systems, after leaching and runoff of nitrogen from fertilised soils. The aim of this study is to quantify future N2O emissions associated with the cultivation of energy crops in European river basins. We analyse three future scenarios for biodiesel production in Europe, and the associated N2O emissions from fertilised fields. Our focus is on biodiesel produced from first generation energy crops. The scenarios assume that by the year 2050, 15–30% of the demand for fossil diesel is replaced by biodiesel. This would change the European fertiliser needs and, as a result, N2O emissions from fertilised soils. Our results indicate that increased biodiesel production may increase N2O emissions in Europe by about 25–45% relative to a scenario without a growth in biodiesel production, but not equally in all regions and all scenarios. The rate of change depends on where energy crops are grown, and whether or not they replace agricultural crops, or natural vegetation.
1. Introduction
Biofuels are considered sustainable energy sources because the cultivation of the energy crops potentially does not result in an increase of greenhouse gas (GHG) emissions. In particular biodiesel is considered a fuel with a low greenhouse potential (Fischer et al. Citation2010) and is therefore favoured in the European energy policies (EU Citation2009). The amount of carbon dioxide (CO2) that is released as a result of combustion of biofuels is compensated by the uptake of CO2 by the crop previously and therefore the net CO2 emissions are generally assumed to be considerable less than the CO2 emissions of fossil fuels (Fischer et al. Citation2010). However, for the cultivation of the energy crops and the production of the biofuel from these crops, additional inputs are necessary, that could adversely affect the GHG balance. For growing first generation energy crops a considerable amount of synthetic fertiliser and fossil energy is needed and also the biofuel production processes require additional fossil energy. These inputs give rise to GHG emissions additional to the emissions caused by the combustion of the biofuel itself, making the use of biofuels less favourable to combat GHG emissions, as demonstrated in studies on the life cycle of biofuels from energy crops (Erisman et al. Citation2010; de Wit et al. Citation2011). A major concern is the use of synthetic nitrogen containing fertilisers (N-fertilisers), because these could be partly converted into nitrous oxide (N2O), which has a large Global Warming Potential (GWP), about 296 times larger than CO2 (Crutzen et al. Citation2008).
Agriculture is the most important source of atmospheric N2O (Syakila & Kroeze Citation2011). In 2005 about two-thirds (4.1 Tg N2O–N/y) of the global anthropogenic N2O emissions were derived from agriculture, which highly exceeded the contribution of other sources like biomass burning (0.7 Tg N2O–N/y) and industry and fossil fuel combustion (0.9 Tg N2O–N/y) (UNEP Citation2013). The increased use of synthetic nitrogen containing fertilisers leads to an increased availability of reactive N in soils and sediments, that can be converted in N2O by microorganisms (nitrification and denitrification processes). For this conversion in N2O there are two major pathways: direct conversion, when N in soil is microbiologically converted to N2O and indirect conversion, when dissolved N is transported to the aquatic environment by leaching and runoff and there converted to N2O (IPCC Citation2006).
To estimate the N2O emissions as a result of fertiliser use at the national scale, the IPCC presents Emission Factors (EFs) for calculation of direct and indirect N2O emissions from managed soils (IPCC Citation2006). Although these EFs are derived from analysis of experimental data and have been evaluated several times, they are relatively uncertain, and also questioned in studies that focus on cultivation of energy crops (Smith et al. Citation2012).
Emissions of N2O are important in the discussion about biofuels. For some fuels, the N2O emissions as a result of increased N-fertiliser use and additional CO2 emissions during industrial fertiliser production may equal or exceed the avoided CO2 emissions from fossil fuels (Crutzen et al. Citation2008; de Wit et al. Citation2011). Especially first generation energy crops that demand a lot of N fertiliser, like rapeseed and corn, could therefore have a higher GWP than the fossil fuels they replace.
The aim of this study is to quantify future N2O emissions from European river basins that are associated with the cultivation of energy crops. We consider three future scenarios in which a considerable area is used for the production of biofuels. As a starting point for our scenario analysis we used one of the Millennium Ecosystem Assessment (MA) scenarios for the year 2050, Global Orchestration (GO) (Alcamo et al. Citation2005). The MA scenarios were developed as part of a United Nations initiative, starting in 2000, to assess the future consequences of changing ecosystems for society. In this context, four future scenarios (including the GO scenario) were developed, which estimated the developments up to the year 2050, differing from each other on assumed socio-economic development and ecosystem management (see Section 2.2 for more information). Here we take one of these scenarios as a baseline, and assume that annually 0.4 billion m3 biodiesel is needed to replace all current transport fuels in de EU-271 (Wijffels & Bardosa Citation2010). In our scenarios we assume that a hypothetical first generation energy crop will meet 15–30% of this European (bio)diesel demand. The scenarios differ in the assumptions about the area that is converted for growing energy crops: they assume reallocation of existing agricultural land for cultivation of energy crops and/or conversion of non-agricultural land.
2. Method
2.1. Scenario overview
We calculated N2O emissions from agricultural soils as induced by synthetic fertiliser use for a number of European river basins We used a collection of 42 European river basins, that were selected in an earlier study (van Wijnen et al. Citation2015) These river basins were selected because they have a nitrogen load at the river mouth of 10 Gg/y or more (Blaas & Kroeze Citation2014) and an agricultural area of at least 5% Among them are the largest basins in the EU-27 countries,Footnote1 in terms of total area of the river basin (Figure ).
To estimated amount of N fertilisers used in European river basins are taken from the Global NEWS models. (Mayorga et al. Citation2010). Global NEWS is a set of global models that has been used to predict future river export of nutrients in a spatially explicit way. We analysed three future scenarios assuming increased first generation energy crop cultivation. Starting point of our scenario building (S0) was a scenario that has been implemented in the Global NEWS models (Mayorga et al. Citation2010; Seitzinger et al. Citation2010), for the year 2050. This is one of the MA scenarios, GO. We built three scenarios using this GO2050 scenario. The scenarios differ from each other in terms of the amount of agricultural area or non-agricultural area that was attributed to the cultivation of first generation energy crops (Table , Figure ).
Table 1. Scenario descriptions: assumptions about growing energy crops in the study area for three scenarios, using the GO 2050 MA scenario as a baseline (S0).
Figure 2. Land use in the scenarios S0–S3 (total area: 2.9 106 km2, see Table for scenario descriptions; source: Global NEWS).
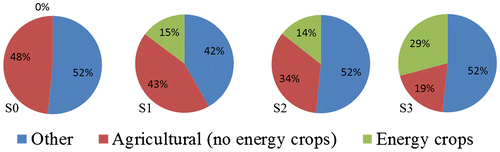
We developed hypothetical scenarios. The biodiesel production in the three scenarios differed: in the first two scenarios (S1 and S2) we assumed the biodiesel production to be about 15% of the current European (bio)diesel demand (Wijffels & Bardosa Citation2010) and in the third scenario (S3) about 30%. In our first alternative scenario (S1) we used non-agricultural land of the baseline scenario (S0) for growing energy crops to produce a reasonable amount of biodiesel without harming food and feedstock production too much. Another reason to use non-agricultural land for energy crops was the projected decrease, by about 10%, of total agricultural area in the GO2050 scenario (our baseline scenario) relative to the situation in the year 2000. This land is assumed to be converted to non-agricultural land (such as urban and recreational areas) (Mayorga et al. Citation2010). Therefore, in our first alternative scenario (S1) we assumed that in each watershed a part of the non-agricultural area as large as 10% of the total area of the watershed could be used as agricultural land for growing energy crops. For the total study area this meant that 19% of the non-agricultural area was used to grow energy crops, fully compensating for the 10% of agricultural land that was projected to be lost between 2000 and 2050 in the MA-GO2050 scenario. In addition 10% of agricultural land was used for energy crops in this scenario (S1). In scenario S2, 30% of the agricultural area of the baseline scenario (S0) was converted for growing energy crops instead of the traditional crops for food- and feedstock. This percentage was considered the highest that can be used for cultivation of energy crops without endangering food production (Fischer et al. Citation2010). In scenario S3 we assumed that 60% of the agricultural area of the baseline scenario (S0) could be used for growing energy crops, providing for 30% of the current diesel demand. This scenario (S3) is on average a rather extreme scenario, because in many European countries it will severely threaten food production.
In all three scenarios (S1, S2 and S3) we assumed cultivation of a hypothetical first generation energy crop. This crop was applied in all three scenarios and in all the river basins. The input of N fertiliser for a first generation energy crop like rapeseed in Europe is generally in the range of 100–200 kg N/ha/y. We used a N input of 121 kg N/ha/y for this hypothetical crop, based on a study on biofuel (rapeseed) cropping in Germany (de Vries et al. Citation2014).
2.2. Global NEWS and the MA Scenarios
We used information from the Global NEWS (Nutrient Export from WaterSheds) models to calculate N2O emissions in our future scenarios (Mayorga et al. Citation2010; Seitzinger et al. Citation2010) Global NEWS is a global, spatially explicit model that calculates river export of nutrients as a function of human activities on the land, basin characteristics and hydrology One of the important drivers of nutrient loads of rivers in these models is the N input to land, which includes fertiliser use in agriculture Other nutrient inputs are for example animal manure used in agriculture, biological fixation of N2 and atmospheric deposition of nitrogen containing compounds The different future MA scenarios were implemented in the Global NEWS models to analyse future nutrient export by rivers to coastal areas (Alcamo et al. Citation2005; Carpenter et al. Citation2005; Cork et al. Citation2005) To achieve this, after interpretation of the MA storylines, input data sets for diffuse sources, point sources and hydrology were developed for Global NEWS Most Global NEWS model input data are at a scale of 0.5 × 0.5 degree longitude by latitude Input databases for Global NEWS were generated using the IMAGE model (a global ecological-environmental model framework for simulating the consequences of human activities) and the Water Balance Plus model (a hydrological model) (Bouwman et al. Citation2009; Van Drecht et al. Citation2009; Fekete et al. Citation2010) In this study, we used the GO scenario for the year 2050 (GO2050) This scenario is characterised by a fast economic growth and a reactive approach towards environmental issues In this study we used the GO2050 scenario N-fertiliser input data in Global NEWS, for the selected 42 European river basins, as a starting point of our scenario building The Global NEWS models have been validated in different earlier studies, at the global scale (Mayorga et al. Citation2010; Seitzinger et al. Citation2010), for specific continents (Qu & Kroeze Citation2010; Yasin & Kroeze Citation2010) and at the scale of selected river basins (Strokal & Kroeze Citation2013; Suwarno et al. Citation2013; Blaas & Kroeze Citation2014; Sattar et al. Citation2014)
2.3. Input of synthetic N fertiliser
We calculated the total amount of synthetic N fertiliser applied to soils in the different scenarios using the input data of the GO2050 scenario in Global NEWS (Bouwman et al. Citation2009; Seitzinger et al. Citation2010) In the model, synthetic fertilisers, manure, natural fixation and atmospheric deposition contribute to the total anthropogenic input of N to watersheds (WSdifant,N) (Mayorga et al. Citation2010) We changed for our alternative scenarios the input of synthetic N fertilisers (WSdiffe,N), following our assumptions on the production of energy crops in the different scenarios and used these adjusted values of WSdiffe,N to calculate N2O emissions for all river basins considered.
2.4. N2O emissions
We calculated N2O emissions associated with synthetic fertiliser use following the 2006 IPCC Guidelines for National GHG Inventories (IPCC Citation2006) Chapter 11 of these Guidelines (N2O Emissions from Managed Soils and CO2 Emissions from Lime and Urea Applications) provides equations to calculate direct and indirect emissions of N2O Both direct and indirect N2O emissions depend on the input of N in synthetic fertiliser
For direct N2O emission as a result of fertiliser use we applied the equation:
For indirect N2O emissions as a result of fertiliser use we took into account N2O from leaching and runoff using the equation:
Where
WSdiffe,N = annual amount of synthetic fertiliser N applied to soils (kg N/ y)
FracLEACH = fraction of all N added to mineralised soils in regions where leaching/runoff occurs that is lost through leaching and runoff (kg N/kg of N applied)
EF1 = emission factor for N2O emissions from N inputs (kg N2O–N/kg N input)
EF5 = emission factor for N2O emissions from N leaching and runoff (kg N2O–N/kg leached and runoff)
In the IPCC Guidelines EFs and fractions are proposed for both direct and indirect N2O emissions. We use EF1 = 0.01, EF5 = 0.0075 and FracLEACH = 0.30, leading to:
Using WSdiffe,N in kg N/km2/y, the equation to calculate the N2O emission results in the amount of nitrogen (N) in N2O (N2O–N) in kg/km2/y; to obtain the amount of N2O (kg N2O/km2/y) we used a correction factor of 44/28. This equation for the total N2O emissions implies that about 18% of the total biogenic N2O emissions associated with the use of synthetic fertilisers is the result of indirect N2O emissions and 82% of direct N2O emissions.
3. Results
We calculated the amount of N2O emitted as a result of cultivation of energy crops in our future scenarios (S1–S3) and the increase of N2O emissions relative to the baseline scenario (S0). In all three scenarios the total N2O emission from synthetic fertiliser use in Europe increased by 43–86 Gg/y (24–45%) compared with the 178 Gg N2O/y in the baseline scenario (Table ).
Table 2. Total N2O emissions (Gg N2O/y) from the use of synthetic fertilisers in the baseline scenario and the biofuel scenarios (S0–S3, see for description Table ) and in 2000 and the increase of N2O emissions in the biofuel scenarios relative to S0Table Footnotea and 2000Table Footnoteb.
In the baseline scenario S0, the N2O emissions increased by 20 Gg N2O/y (13%) between 2000 and 2050 as a result of increased fertiliser use in the GO2050 scenario. This implies that in our alternative scenarios (S1–S3) the N2O emissions increase by 63–106 Gg/y (40–67%) relative to 2000. In the first two alternative scenarios (S1 and S2), which have a comparable biodiesel yield, we calculated an increase of the total N2O emissions as a result of growing energy crops. For scenario S1 the increase was about twice as high as that in scenario S2. This is not surprising because in scenario S1 non-agricultural land was converted to grow energy crops . For scenario S3, which was designed as S2, but with a two times higher biodiesel yield, also a larger increase of N2O emissions was calculated.
Analysis of our future biofuel scenarios (S1–S3) indicated a large spatial variation of N2O emissions among the river basins (Figure ).
Figure 3. Increase in biogenic N2O emissions (direct and indirect) associated with the use of synthetic fertilisers in the three future scenarios S1–S3 (as percentage of the baseline scenario S0, calculated (for scenario Sx) as N2O emission ((Sx–S0)/S0×100%) (see Table for scenario overview).
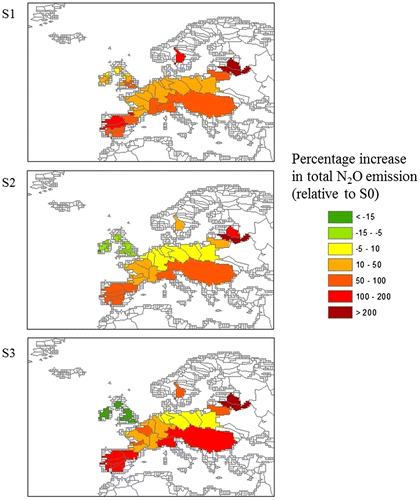
Although we calculated an overall increase of N2O emissions in all three scenarios, this is not necessarily true for each individual river basin (Figure ). Our calculations showed that cultivation of energy crops may lead to an increase of N2O emissions for many river basins, but that there are also some river basins where a decrease of N2O emissions could be expected. In scenario S1, in which agricultural as well as non-agricultural land was converted to grow energy crops, N2O emissions increase in all river basins (calculated increase ranges from 10 to 200%). In the scenarios in which only existing agricultural land was used (S2 and S3), N2O emissions are calculated to increase in some basins (by 10–200%), but to decrease in others (by up to 15%). Comparing scenario S1 with scenario S2 (the two scenarios with a comparable yield of energy crop) indicates that although scenario S1 showed a larger increase of total N2O emissions, on a regional scale both scenarios showed large increases of N2O emissions. For example for the Danube basin, the basins in France, Spain and the Baltic States we calculated increases of N2O emissions by 50–100% (Figure ).
For a better understanding of the large differences in the increase of N2O emissions as a result of cultivating energy crops for the different river basins we calculated the N2O emissions relative to the baseline scenario in a number of large river basins (Table ). We have chosen these river basins to illustrate the consequences of our different biofuel scenarios on basis of river basin dimensions and percentage of agricultural land.
Table 3. The differenceTable Footnotea in biogenic N2O emissions (kg N2O/ha/y) from selected river basins as a result of synthetic fertiliser use in energy crop cultivation in the year 2050. Differences are presented for our future scenarios (S1–S3), relative to the baseline scenario S0 (see for scenario description Table ). Unit: difference with S0 in kg/ha/y (in parentheses the % change relative to S0). The last two columns show the N2O emissions in S0 in the year 2050 in kg/ha/y.
In the baseline scenario S0, the N2O emissions associated with synthetic fertiliser use range from about 1 to almost 4 kg N2O/ha agricultural land/y by 2050 (Table ). In our biofuel scenario S1 these emissions were 15–66% higher as a result of increased fertiliser use. In the other scenarios the change in N2O emissions varied from –12% (a decrease) to 69% (S2) and from –24 to 139% (S3). We also calculated the differences between the biofuel scenarios and the baseline scenario per hectare of basin. In some basins, emissions may double as a result of increased fertiliser use.
In scenario S1 the agricultural area is larger compared to that in the baseline scenario (S0), and also the ratio of agricultural to non-agricultural land has changed. Therefore, calculated in percentages, the changes in N2O emissions per hectare of agricultural area are lower than those in N2O emissions per hectare of the entire river basin. In this scenario all European river basins show an increase in N2O emissions. In river basins that show relatively small N2O emissions in the baseline scenario, scenario S1 leads to a large increase of N2O emissions. This is illustrated with the Lithuanian Nemanus basin, that has a small agricultural area in the baseline scenario, leading to a 66% increase of N2O emissions in scenario S1 (relative to S0). Another example is the Spanish Guadiana basin, that seems to have a very small N-fertiliser application in S0, leading to a 70% increase of N2O emissions in scenario S1. For scenario S2, where 30% of the agricultural area of the baseline scenario is converted to energy crops, an increase in N2O emissions has been calculated for river basins like the Danube (50%), the Loire (22%) and the Seine (27%). Other basins show a decrease of N2O emissions (for example the Shannon and the Humber basin (12 and 9%)) or a N2O emission that is comparable to that of the baseline scenario (for example the Odra and the Wisla basin (only an increase of 1 and 3%)). For scenario S3, where twice as much land is used for cultivation of energy crops, the same trends are calculated as in S2, with larger differences.
3.1. Uncertainties
We kept our scenarios simple, which enables us to give a rather quick insight in the consequences of large scale cultivation of first generation energy crops in Europe As a consequence, we made a number of assumptions in our study First, we used in our calculations only one hypothetical first generation energy crop, that we assumed to grow in all European regions This seems to be rather unlikely: the two major first generation energy crops that are applied in Europe are rapeseed and sunflower, depending on the climate of a particular region The N-fertiliser demand of both crops differ, but is for both crops often higher than the 121 kg N/ha we used for our hypothetical crop (Pimentel & Patzek Citation2005; de Vries et al. Citation2013)
To have some understanding of the consequences of this choice, we carried out a sensitivity analysis to test the sensitivity of our calculated N2O emissions to changes in synthetic fertiliser use. To this end, we calculated N2O emissions assuming that the N-fertiliser demand for the energy crops is 25% smaller (90 kg N/ha) or 25% higher (150 kg N/ha) than in our default calculations (Table ). Table indicates that changing to a crop with an N-fertiliser demand of 25% more or less may lead to a change of the total N2O emission by about 10% (or even more).
Table 4. European N2O emissions (Gg N2O/y) from synthetic fertilisers in our scenarios for energy crops assuming an N-fertiliser demand that is 25% lower (90 kg N/ha) or 25% higher (150 kg N/ha) than in our (default) energy scenarios (for scenario description see Table ).
A second uncertainty that we should take into account is that of the EFs we used (EF1 and EF5) to estimate the direct and indirect N2O emissions from managed soils. The range of these EFs is rather large: for EF1 the default value is 0.01 with an uncertainty range of 0.003–0.03 and for EF5 the default value is 0.0075 with an uncertainty range of 0.0005–0.025 (IPCC Citation2006). Translation of applied N-fertiliser to N2O emissions in our scenarios with these uncertainty ranges leads to a low and a high estimate of the total N2O emissions as a result of our energy scenarios (Table ). Because the N2O emissions are proportional to the N-fertiliser use in a scenario, the total N2O emissions using the low EFs for each scenario were about one fourth (26%) of those using the default EFs. Using the high EFs the N2O emissions more than tripled (306%) compared to the N2O emissions using the default EFs.
Table 5. Total N2O emissions (Gg N2O/y) in our scenarios for energy crops if the EFs are low (EF1 = 0.003 and EF5 = 0.0005), default (EF1 = 0.01 and EF5 = 0.0075) or high (EF1 = 0.03 and EF5 = 0.025).
A third concern might be that we assumed that in all European river basins the conditions for growing energy crops are the same, which is definitely not the case. In our scenarios each river basin has the same potential to be used for cultivating energy crops and no effort has been done to specify the potentials of individual river basins, which could have resulted in a situation in which the best suitable river basins produce more biomass for biodiesel that others.
Finally the assumption that in all river basins 10% of the non-agricultural area can be used for agriculture (energy crops) is not realistic for all river basins. For example in river basins with a small percentage of agricultural land in the baseline scenario, it could be impossible to use 10% of the non-agricultural land for energy crops. In these basins the non-agricultural area might not be suitable for agriculture practices because the climate is too cold or the landscape too hilly. It should be realised that our scenarios are hypothetical cases.
4. Discussion
In this study we analysed the effect of large scale biodiesel production on N2O-emissions in Europe. We developed three future scenarios in which cultivation of first generation energy crops played an important role and we used the Global NEWS models to analyse these scenarios. Our scenarios could be able to yield an amount of biodiesel that can replace 15 to 30% of the current diesel demand for transport in Europe. All our scenarios indicate increased N2O emissions relative to our baseline scenario, GO2050, as a result of cultivation of energy crops. In the scenarios where only already existing agricultural land was used for cultivating energy crops (S2 and S3) the calculations showed a large spatial diversity in N2O emissions: especially for regions in Southern- or Eastern Europe we calculated relatively large increases in N2O emissions as a result of cultivation of first generation energy crops. Unfortunately, these regions, especially in Eastern Europe, might have the best potentials to contribute to the increases European biomass based biofuel demand (de Wit et al. Citation2011). Because an increase of N2O emissions might not be in line with regional policies towards GHG emission reduction, cultivating first generation energy crops in these regions could be discouraged by the government.
We focussed in our study on the N2O emissions as a consequence of large-scale biodiesel production in Europe. Our study helps to better understand the N2O budget. We did not intend to present a total GHG balance for energy crops or biodiesel as is typically done in life cycle assessments. We realise that the production and use of biodiesel from energy crops also results in fossil CO2 emissions, which we did not include in our analysis. Therefore, we cannot draw conclusions about the sustainability of biofuels. Our results give us a better understanding of the amount and the spatial variation of N2O emissions in Europe in future scenarios.
Our scenarios assume that energy crops are replacing other land use types, among which agriculture. In some basins, the implications may be that food production is replaced by energy crop production. It should be realised that this may have consequences for food production, and for import and export of food into and out of Europe. In some basins these consequences may be larger than in others, depending on the type of crops that is replaced.
A United Nations Environment Programme (UNEP Citation2013) report quantifies global anthropogenic N2O emission to be 5.3 Tg N2O–N/y for 2010, of which 66% is from agriculture. Europe is responsible for about 13% of the global N2O emissions (528 Gg N2O–N/y). In the UNEP report several future scenarios are suggested to mitigate N2O emissions (e.g. more efficient use of N in growing crops). Analysis of these mitigation scenarios for 2050 indicates that a reduction of global N2O emissions to 3.0 Tg N2O–N/y might be possible. For Europe this would mean a total N2O emission from agriculture of 390 Gg N2O–N/y (a 26% reduction compared to 2010).
In our energy crop scenarios the total N2O emission increase relative to the baseline scenario is 27–55 Gg N2O–N/y (43–86 Gg N2O/y), about 5–10% of the total European agricultural N2O emissions (relative to 2000 this increase is 8–13%). Although the mitigation scenarios for 2050 mentioned above are likely to affect our energy crop scenarios too, our results indicate that large scale cultivation of first generation crops most probably have a substantial negative effect on (European) mitigation programs for N2O emissions in the future.
The assumptions we made to keep our scenarios simple lead to uncertainties in the outcome of the calculation of the N2O emissions. The uncertainty about the amount of fertiliser needed for energy crops leads to an possible deviation of the N2O emissions of 10–19%. However, the uncertainty of the EFs determines the predictions of the N2O-emissions as a result of the cultivation of energy crops much more. Depending on the value of the EFs, the N2O emissions range from 26 to 306% of those calculated with the default EFs (IPCC Citation2006). Different authors discuss the uncertainties in these EFs (Crutzen et al. Citation2008; Smith et al. Citation2012). More than once an underestimation of EFs was reported, indicating that the N2O emissions we calculated as a result of energy cropping might be higher than those calculated with the default EFs.
5. Conclusions
In our future scenarios we calculated both direct and indirect N2O emissions as a result of fertiliser use in the cultivation of first generation energy crops. Our 2050 scenarios indicate that total N2O emissions from the European river basins as a result of energy crop cultivation may be as large as 220 to 260 Gg N2O/y. This is 24–45% higher than in our baseline scenario without increased energy crop production. European policies aim to reduce GHG emissions and therefore the UNEP has developed mitigation scenarios for the year 2050, with the aim to reduce N2O emissions (UNEP Citation2013). Our biofuel scenarios, however, indicate that cultivation of first generation energy crops may increase N2O emissions relative to our baseline scenario (S0) by about 5–10% of the total European agricultural N2O emissions in 2050, which is not in line with the 2050 mitigation scenarios of the UNEP.
Our scenarios indicate that the spatial variation in N2O emissions is large. We calculate the highest N2O emissions for the Southern and Eastern European region. Two of our scenarios, that have a comparable biodiesel yield (S1 and S2), show a quite different spatial pattern of N2O emissions: in scenario S1, where both agricultural area and non-agricultural area were converted to cultivation of energy crops, fertiliser use and therefore N2O emissions increase all over Europe (on average by 45% relative to the baseline scenario S0). In scenario S2, in which only existing agricultural land was used the increase of fertiliser use differs for the river basins depending on the former agricultural land use. In some river basins in Northern Europe the fertiliser demand does not change or decrease as a result of cultivating energy crops, and therefore the N2O emissions will not increase either.
European policy towards sustainable energy use is aimed at the increasing use of renewable energy sources, including biomass for biofuel production. Our study shows that although biofuels could contribute to lower GHG emissions, increased biomass production could increase N2O emissions considerably in regions where energy crops are cultivated. This is a result of increased fertiliser use, especially in the Southern and Eastern European countries. We argue that, to minimise the N2O emissions, energy crops with a low fertiliser demand deserve priority. Second generation energy crops, like miscanthus and willow may be more suitable to achieve this than first generation crops, because these crops demand less N-fertiliser (Rebelo de Mira & Kroeze Citation2006; Smith et al. Citation2012). However, these crops are currently not applied on a large scale in Europe (Fischer et al. Citation2010).
Disclosure statement
No potential conflict of interest was reported by the authors.
Notes
1. The EU-27 member states until July 1st, 2013 were: Austria, Belgium, Bulgaria, Cyprus, the Czech Republic, Denmark, Estonia, Finland, France, Germany, Greece, Hungary, Ireland, Italy, Latvia, Lithuania, Luxembourg, Malta, the Netherlands, Poland, Portugal, Romania, Slovakia, Slovenia, Spain, Sweden and the United Kingdom.
References
- Alcamo J, Van Vuuren D, Cramer W, Alder J, Bennett E, Carpenter S, Christensen V, Foley J, Maerker M, Masui T, et al. 2005. Changes in ecosystem services and their drivers across the scenarios. In: Carpenter S, Pingali P, Bennet E, Zurek M, editors. Millennium ecosystem assessment volume 2 scenarios. Washington (DC): Island Press; p. 297–373.
- Blaas H, Kroeze C. 2014. Possible future effects of large scale algae cultivation for biofuels on coastal eutrophication in Europe. Sci Total Environ. 496:45–53.10.1016/j.scitotenv.2014.06.131
- Bouwman AF, Beusen AHW, Billen G. 2009. Human alteration of the global nitrogen and phosphorus soil balances for the period 1970–2050. Global Biogeochem Cycles 23:GB0A04.
- Carpenter SR, Pingali PL, Bennet EM. 2005. Ecosystems and human well-being scenarios, findings of the scenarios working group. Millennium Ecosystem Assessment. Washington (DC): Island Press.
- Cork S, Peterson G, Petschel-Held G, Alcamo J, Alder J, Bennett E, et al. 2005. Four Scenarios. In: Carpenter S, Pingali, P., Bennet, E., Zurek, M., editors. Millennium Ecosystem Assessment. Volume 2: Scenarios. Washington (DC): Island Press; p. 223–294.
- Crutzen P, Mosier A, Smith K, Winiwarter W. 2008. N2O release from agro-biofuel production negates global warming reduction by replacing fossil fuels. Atmos Chem Phys. 8:389–395.10.5194/acp-8-389-2008
- de Vries SC, van de Ven GWJ, van, Ittersum MK. 2014. First or second generation biofuel crops in Brandenburg, Germany. A model-based comparison of their production-ecological sustainability. Eur J Agron. 52:161–179.
- de Wit M, Londo M, Faaij A. 2011. Productivity developments in European agriculture: relations to and opportunities for biomass production. Renewable Sustainable Energy Rev. 15:2397–2412.10.1016/j.rser.2011.02.022
- Erisman JW, Grinsven Hv, Leip A, Mosier A, Bleeker A. 2010. Nitrogen and biofuels; an overview of the current state of knowledge. Nutr Cycling Agroecosyst. 86:211–223.10.1007/s10705-009-9285-4
- EU. 2009. Directive 2009/28/EC of the European Parliament and of the Council of 23 April 2009 on the promotion of the use of energy from renewable sources and amending and subsequently repealing Directives 2001/77/EC and 2003/30/EC Legal notice of the Directive 2009/28/CE of 23 April 2009.
- Fekete BM, Wisser D, Kroeze C,Mayorga E, Bouwman L, Wollheim WM, Vörösmarty CJ. 2010. Millennium ecosystem assessment scenario drivers (1970–2050): climate and hydrological alterations. Global Biogeochem Cycles 24:GB0A12.
- Fischer G, Prieler S, van Velthuizen H, Berndes G, Faaij A, Londo M, de Wit M. 2010. Biofuel production potentials in Europe: sustainable use of cultivated land and pastures, Part II: land use scenarios. Biomass Bioenergy. 34:173–187.10.1016/j.biombioe.2009.07.009
- IPCC. 2006. IPCC Guidelines for National Greenhouse Gas Inventories, Prepared by the National Greenhouse Gas Inventories Programme. In: Eggleston HS, Buendia L, Miwa K, Ngara T, Tanabe K, editors. Hayama: IGES; Chapter 24; p. 1–54.
- Mayorga E, Seitzinger SP, Harrison JA, Dumont E, Beusen AHW, Bouwman AF, Fekete BM, Kroeze C, van Drecht G. 2010. Global nutrient export from watersheds 2 (NEWS 2): model development and implementation. Environ Model Soft. 25:837–853.
- Pimentel D, Patzek TW. 2005. Ethanol production using corn, switchgrass, and wood; biodiesel production using soybean and sunflower. Nat Resour Res. 14:65–76.10.1007/s11053-005-4679-8
- Qu H, Kroeze C. 2010. Past and future trends in nutrients export by rivers to the coastal waters of China. Sci Total Environ. 408:2075–2086.10.1016/j.scitotenv.2009.12.015
- Rebelo de Mira R, Kroeze C. 2006. Greenhouse gas emissions from willow-based electricity: a scenario analysis for Portugal and The Netherlands. Energy Policy. 34:1367–1377.10.1016/j.enpol.2004.10.021
- Sattar MA, Kroeze C, Strokal M. 2014. The increasing impact of food production on nutrient export by rivers to the Bay of Bengal 1970–2050. Mar Pollut Bull. 8:168–178.10.1016/j.marpolbul.2014.01.017
- Seitzinger SP, Mayorga E, Bouwman AF, Kroeze C, Beusen AHW, Billen G, et al. 2010. Global river nutrient export: a scenario analysis of past and future trends. Global Biogeochem Cycles 24:GB0A08.
- Smith KA, Mosier AR, Crutzen PJ, Winiwarter W. 2012. The role of N2O derived from crop based biofuels, and from agriculture in general, in Earth’s climate. Philos Trans R Soc B: Biol Sci. 367:1169–1174.10.1098/rstb.2011.0313
- Strokal M, Kroeze C. 2013. Nitrogen and phosphorus inputs to the Black Sea in 1970–2050. Regional Environ Change. 13:179–192.10.1007/s10113-012-0328-z
- Suwarno D, Löhr A, Kroeze C, Widianarko B. 2013. Past and future trends in nutrient export by 19 rivers to the coastal waters of Indonesia. J Integr Environ Sci. 10:55–71.10.1080/1943815X.2013.772902
- Syakila A, Kroeze C. 2011. The global nitrous oxide budget revisited. Greenhouse Gas Meas Manage. 1:17–26.10.3763/ghgmm.2010.0007
- UNEP. 2013. Drawing N2O to Protect Climate and the Ozone Layer. A UNEP Synthesis Report. United Nations Environment Programme (UNEP), Nairobi, Kenya.
- Van Drecht G, Bouwman AF, Harrison J,Knoop JM. 2009. Global nitrogen and phosphate in urban wastewater for the period 1970 to 2050. Global Biogeochem Cycles 23:GB0A03.
- van Wijnen J, Ivens WPMF, Kroeze C, Löhr A. 2015. Coastal eutrophication in Europe caused by production of energy crops. Sci Total Environ. 511:101–111.10.1016/j.scitotenv.2014.12.032
- Wijffels RH, Bardosa MJ. 2010. An outlook on microalgal biofuels. Science. 329:796–799.
- Yasin J, Kroeze C. 2010. Nutrients export by rivers to the coastal waters of Africa: past and future trends. Global Biogeochem Cycles. 4:1–14.