ABSTRACT
Polyethylene terephthalate (PET) bottles are widely used as packaging material for natural mineral water. However, trace levels of acetaldehyde can migrate into natural mineral water during the shelf life and might influence the taste of the PET bottled water. 2-Aminobenzamide is widely used during PET bottle production as a scavenging agent for acetaldehyde. The aim of this study was the determination of the migration kinetics of 2-aminobenzamide into natural mineral water as well as into 20% ethanol. From the migration kinetics, the diffusion coefficients of 2-aminobenzamide in PET at 23 and 40°C were determined to be 4.2 × 10−16 and 4.2 × 10−15 cm2 s–1, respectively. The diffusion coefficient for 20% ethanol at 40°C was determined to be 7.7 × 10−15 cm2 s–1, which indicates that 20% ethanol is causing swelling of the PET polymer. From a comparison of migration values between 23 and 40°C, acceleration factors of 9.7 when using water as contact medium and 18.1 for 20% ethanol as simulant can be derived for definition of appropriate accelerated test conditions at 40°C. The European Union regulatory acceleration test based on 80 kJ mol–1 as conservative activation energy overestimates the experimentally determined acceleration rates by a factor of 1.6 and 3.1, respectively.
Graphical Abstract
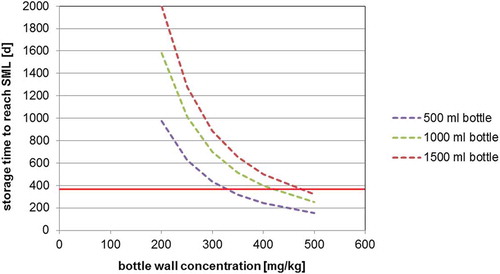
Introduction
Polyethylene terephthalate (PET) bottles are widely used as packaging material for natural mineral water, soft drinks and juices. PET is a transparent packaging polymer that is virtually unbreakable. This unbreakable nature of PET is an important feature for a beverage packaging that permits the safe handling of bottled water (e.g., at home or during sports) compared with competitive glass bottles. The global usage volume of PET packaging was almost 16 million metric tons in 2013. A total of 36% of this PET material was used for the manufacturing of mineral water bottles (Welle Citation2014a). In comparison with glass bottles, which release only inorganic compounds (Reimann et al. Citation2010, Citation2012), the migration of organic compounds from the PET bottle wall into bottled mineral water plays an important role. For example, during PET bottle manufacture, acetaldehyde can be generated and might migrate into PET bottled natural mineral water. According to European Regulation No. 10/2011, the specific migration of acetaldehyde into PET packed food is restricted to a specific migration limit (SML) of 6 mg kg–1 food (EU Citation2011). This SML cannot be reached under typical storage conditions of PET bottles (Welle Citation2014b). However, the organoleptic properties of the PET packed mineral water might be influenced due to acetaldehyde migration. The taste threshold of acetaldehyde in water is between 10 µg l–1 (retronasal) and 25 µg l–1 (orthonasal) (Buettner & Schieberle Citation2001). Such low concentrations of acetaldehyde in natural mineral water lead to an off-taste. In consequence, the packaging material is not in compliance with Article 3 of European Framework Regulation No. 1935/2004 (EU Citation2004) or the US Federal Food, Drug, and Cosmetic Act (USFDA Citation2015). Both regulations demand in general that the organoleptic properties of packed food should not be influenced by the packaging material. Therefore, migration of acetaldehyde into food is relevant for natural mineral water due to possible organoleptic complaints. Modern PET manufacturing practices have been used to minimise the formation of aldehydes during pre-form and bottle manufacturing. However, trace levels of acetaldehyde are found in PET bottles, which can still migrate into natural mineral water during the shelf life of 6–12 months. The migration of acetaldehyde into natural mineral water follows Fickian laws of diffusion (Ewender et al. Citation2003). A correlation between bottle wall concentration and migration after storage for 10 days at 40°C has been established (Welle Citation2014b).
According to migration theory, the migration of an organic compound in the packaging polymer correlates directly with the bottle wall concentration (Crank Citation1975). Therefore, the main strategy to reduce the migration of acetaldehyde into natural mineral water is to minimise the concentration of acetaldehyde in the PET bottle wall. Lower bottle wall concentrations of acetaldehyde lead to lower migration into mineral water. 2-Aminobenzamide (CAS No. 88-68-6; anthranilamide) is widely used as a scavenging agent for acetaldehyde during PET pre-form production in order to reduce the concentration of acetaldehyde in the PET bottle wall. shows the proposed mechanism of the reaction between 2-aminobenzamide and acetaldehyde during pre-form manufacturing (Mrozinski et al. Citation2012, Citation2013).
Figure 1. Mechanism of the reaction of 2-aminobenzamide with acetaldehyde during PET pre-form manufacturing.
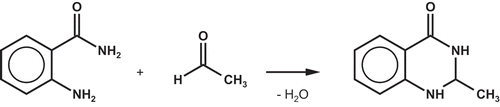
According to European Plastics Regulation No. 10/2011 (EU Citation2011), 2-aminobenzamide is approved as an additive for use in PET bottles for water and beverages with an SML of 50 μg l–1. In the United States, 2-aminobenzamide is also approved as a scavenger for acetaldehyde in PET beverage bottles by Food Contact Notification No. 137 (FCN Citation2001). According to this notification, 2-aminobenzamide may be present in the finished container at levels not exceeding 500 mg kg–1 in bottles used to hold water and at levels not exceeding 250 mg kg–1 in bottles used for non-water, aqueous, acidic and low-alcohol food applications.
European Plastics Regulation No. 10/2011 (EU Citation2011) came into force in 2011. One of the changes in comparison with the previous legislation was that the testing time and temperature conditions used for specific migration tests for long-term applications above 30 days at RT and below can now be calculated using the Arrhenius equation. This allows for different conditions of time and temperature, e.g., longer times at lower temperatures or shorter times at higher temperatures. For example, one possible test condition is 10 days at 60°C as an accelerated test at elevated temperature. In contrast, the most severe test conditions for long-term contact applications were previously 10 days at 40°C. According to new rules of compliance testing as laid down in Regulation No. 10/2011, the testing conditions ‘10 days at 60°C’ should cover long-term storage above 6 months at RT and below including heating up to 70°C for up to 2 h, or heating up to 100°C for up to 15 min. According to the new testing conditions of Regulation No. 10/2011 (EU Citation2011), PET bottles for natural mineral water, soft drinks and juices are often tested with 20% ethanol at the storage conditions of 10 days at 60°C. Sometimes also 95% ethanol is applied. The use of high ethanolic solutions like 50% or 95% ethanol has the advantage that migration testing results are applicable for compliance evaluation of all packed foods, including fatty or oily foodstuffs, even if the main application of PET bottles is natural mineral water, soft drinks, juices and beer. The major disadvantage of food simulants like 50% or 95% ethanolic solutions is that these simulants are swelling the PET matrix and lead to a significant overestimation of the specific migration (Franz & Welle Citation2008). In general, swelling of polymer increases the diffusivity of the packaging materials. This effect also increases the specific migration of polymer additives or polymer impurities. Due to the above-mentioned swelling effects, the specific migration of 2-aminobenzamide might exceed the migration limit of 50 µg l–1 if storage conditions of 10 days at 60°C in high ethanolic simulants are applied in the migration test. On the other hand, typical application conditions for 2-aminobenzamide containing PET bottles (e.g., bottles for natural mineral water) do not swell the PET matrix and, in consequence, the specific migration of 2-aminobenzamide is significantly lower (Franz & Welle Citation2008). Testing of mineral water bottles with high ethanolic solutions are therefore too rigorous testing conditions. In general, migration models provide a useful tool for the prediction of the migration from the concentration of 2-aminobenzamide in the PET bottles. However, the diffusion coefficient needs to be available for such predictions. Till now, the diffusion coefficients of 2-aminobenzamide in PET are not known.
The aim of this work was the determination of the migration kinetics of 2-aminobenzamide into 20% ethanol (simulant C according to Regulation No. 10/2011) as well as into carbonated natural mineral water at RT and 40°C. From the migration kinetics, the diffusion coefficients of 2-aminobenzamide in PET should be derived. From the experimental data, appropriate migration testing conditions should be established for simulation of the long-term storage of mineral water, juices and soft drinks an alternative to high concentrated ethanolic solutions at 60°C.
Materials and methods
Sample material
About 100 empty 0.5 L PET wide-neck bottles spiked with 2-aminobenzamide as acetaldehyde scavenging additive (trade name Triple A1+) were provided by Colormatrix (Knowsley, UK). The thickness of the bottle wall was determined to approximately 300 µm. The nominal concentration of 2-aminobenzamide was 500 mg kg–1 PET. Due to the fact that the concentration was reduced by the reaction with acetaldehyde, the concentration of 2-aminobenazmide in the bottle wall was determined experimentally. These bottles were used to determine the migration kinetics of 2-aminobenzamide into natural mineral water and 20% ethanol.
The concentration of 2-aminobenzamide was in addition determined in natural mineral water bottles at the end of the shelf life. For this purpose, five retain samples of different size (0.5 and 1.5 L) were provided by a German mineral water filling company. All bottles had reached the end of their shelf life and had been stored at the mineral water bottling company at ambient temperatures. The concentrations of 2-aminobenzamide in the PET bottle walls were also determined experimentally.
In order to determine the concentrations of 2-aminobenzamide according to alternative testing conditions of 60 days at 40°C, an additional seven freshly filled natural mineral water bottle were provided by a German mineral water filling company. These samples were stored for 60 days at 40°C. The 2-aminobenzamide concentrations of these samples were compared with the results of the samples stored at RT storage as well as to the results of the migration kinetics.
Determination of 2-aminobenzamide in PET bottles
The concentration of 2-aminobenzamide was determined in the empty PET bottles used for the migration kinetics (three different bottles out of about 100) as well as in the filled mineral water bottles stored at the end of the shelf life as well as for 60 days at 40°C. The filled bottles were emptied after the determination of the concentration of 2-aminobenzamid in mineral water.
The concentration of 2-aminobenzamide was determined according to the following procedure: the wall of the bottles was cut to small pieces. Two samples were taken from each bottle and extracted. A total of 1.0 g of PET was extracted with 10 ml dichloromethane p.A. (by Chemsolute) for 3 days at 40°C in closed glass vials. The extracts were decanted and the samples were extracted again with 10 ml dichloromethane for 3 days at 40°C. Subsequently the extracts were analysed directly by GC: an Agilent 6890 with liquid autosampler and flame ionisation detector (FID). Conditions: capillary column: ZB 1 (Phenomenex) – 30 m – 0.25 mm i.d. – 0.25 µm film thickness, temperature programme: 50°C (2 min), rate 10°C min–1 to 340°C (15 min), pressure: 50 kPa, carrier gas: hydrogen, column flow 1 ml min–1, split ratio 1:20, injection volume 5 µl, injector temperature 300°C, FID detector temperature 325°C. The chromatography software Chromeleon (Dionex, Idstein, Germany) was used to control the hardware and to integrate and analyse the data. Quantification was achieved with calibration standards of 2-aminobenzamide (purchased from Sigma-Aldrich, Schnelldorf, Germany; order no. A8,980-4, Lot no 34164-110, purity 98%) in dichloromethane. The standard concentrations were 0.1, 0.5, 1, 5, 10, 20, 50 and 100 µg ml–1. All standards and sample extracts were injected twice. The calculated concentrations of the first and second extracts were added.
Specific migration into carbonated mineral water
For the migration kinetic into mineral water, 36 empty PET bottles were filled with carbonated mineral water. The mineral water was purchased in glass bottles with an aluminium closure from a local mineral water bottling company. Subsequently the bottles were stored at 23 and 40°C. At 23°C three bottles of each kinetic point were drawn after 10, 20, 31, 40, 51 and 60 days. At 40°C three bottles of each kinetic point were drawn after 3, 6, 10, 20, 31 and 40 days. After storage the bottles were shaken, then an aliquot of 1 ml was filled into an 1 ml vial and analysed by HPLC-MS.
Migration kinetics into 20% ethanol
For the migration kinetic into the food simulant C, 36 empty PET bottles were filled with 20% ethanol. Subsequently the bottles were stored at 23 and 40°C. At 23°C three bottles of each kinetic point were drawn after 10, 20, 31, 40, 51 and 60 days. At 40°C three bottles of each kinetic point were drawn after 3, 6, 10, 20, 31 and 40 days. After storage the bottles were shaken, then an aliquot of 1 ml was filled into an 1 ml vial and analysed by HPLC-MS.
Quantification of 2-aminobenzamide in migration solutions
The migration solutions (carbonated mineral water and 20% ethanol) were analysed by HPLC/MS. HPLC equipment: Thermo Finnigan Surveyor; mass spectrometer: Thermo Finnigan TSQ Quantum Ultra AM (Dreieich, Germany). Column: Phenomenex Synergi polar RP 150×3 mm, particle size 4 µm. Analyses were performed with a gradient mobile phase using 0.5% formic acid (aqueous) and methanol at a flow of 0.6 ml min−1. The gradient method started at 20% methanol and linearly increased to 100% methanol in 3 min and was hold for 4 min. Injection volume: 5 µl, column oven temperature: 45°C. Ion source: heated electrospray ionisation (HESI), positive mode (spray voltage: 3000; vaporiser temperature: 350°C; capillary temperature: 250°C; sheath gas pressure: 40; aux gas pressure: 5). Analyte was detected by single reaction-monitoring mode (SRM positive, parent ion 137 Dalton, daughter ion 120 Dalton.
Diffusion modelling
The calculation of the migration was performed using the AKTS SML software version 4.54 (AKTS AG Siders, Siders, Switzerland). The programme uses finite element analysis. The mathematical procedure and the main equations are published by Roduit et al. (Citation2005). For the migration modelling, the diffusion coefficients of anthranilamde determined within this study were used. The inner surface of the bottles was derived from construction drawings of the blow moulding tools. The wall thickness was determined to about < 300 µm. A wall thickness of 300 µm was used for the migration calculations as a worst-case value.
Results
The concentration of 2-aminobenzamide in the PET bottle used for migration kinetics (sample 1) was determined to 197.6 ± 2.7 mg kg–1 as a mean value from three bottles. The concentration of about 200 mg kg–1 was significantly lower than the nominal concentration of 500 mg kg–1 which was the added concentration of 2-aminobenzamide in the formulation of the PET bottle. The difference is most probably due to the chemical reaction of 2-aminobenzamide and acetaldehyde during pre-form and PET bottle manufacturing. Similar concentrations of 2-aminobenzamide were found for the other PET bottles, which were supplied by the German mineral water company (). The results of the migration kinetics into carbonated natural mineral water and 20% ethanol are given in . The migration kinetic data are visualised in . The diffusion coefficients were calculated according to:
Table 1. Concentration of 2-aminobenzamide in natural mineral water after storage for 60 days at 40°C as well as at the end of the shelf life at RT storage.
Table 2. Result of the quantification of 2-aminobenzamide in kinetic test up to storage times of 60 days at 23°C and 40 days at 40°C (bottle wall concentration: 197.6 ± 2.7 mg kg–1).
Figure 2. Results of the migration kinetic of 2-aminobenzamide in mineral water and 20% ethanol at 23 and 40°C.
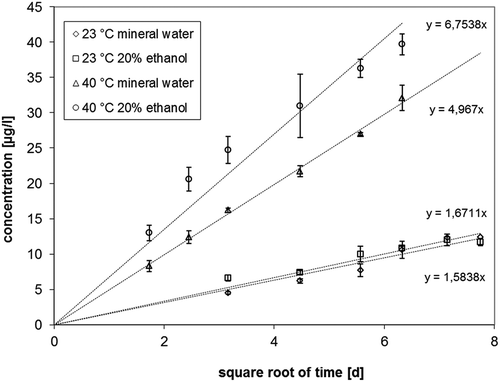
where m/A is the area related mass transfer into natural mineral water or 20% ethanol (in µg dm–2); cP,0 is the concentration of 2-aminobenzamide in the PET bottle wall (in µg g–1); and ρP is the density of the polymer (mg cm–3). The storage time is t (s); and the diffusion coefficient is DP (cm2 s–1). The diffusion coefficients derived from the migration kinetic concentrations () according to equation (1) are summarised in . The experimental data () show a linear correlation between the migration and the square root of time which is expected from migration theory (Crank Citation1975).
Table 3. Experimentally determined diffusion coefficient for 2-aminobenzamide in PET calculated from equation (1) with data from .
The concentrations of 2-aminobenzamide in real samples at the end of the shelf life are given in . In addition the concentrations of 2-aminobenzamide at forced storage conditions (60 days at 40°C) are also given in .
Discussion
In this study, the diffusion coefficients of 2-aminobenzamide were determined in contact with the food simulant 20% ethanol and with carbonated natural mineral water both at 23 and at 40°C (). The underlying experimental kinetic migration data show a linear correlation between the migration and the square root of time, which is expected from diffusion theory (Crank Citation1975). Therefore, it can be concluded that the migration of 2-aminobenzamide follows Fickian laws of diffusion in the performed experiments. At 23°C the slopes of the correlations between the square root of time and the migration is statistically the same and therefore the diffusion coefficients are very similar for both water and 20% ethanol. At 40°C, however, the diffusion coefficient of 2-aminobenzamide into 20% ethanol is significantly higher than for mineral water. This indicates that 20% ethanol is causing some swelling of the PET polymer at 40°C which increases the diffusion of 2-aminobenzamide correspondingly. On the other hand, the swelling effect of 20% ethanol appears to be negligible at RT. From these findings it is expected that swelling effects caused by higher ethanol contents in water already at RT will be further intensified by increased migration test temperatures up to 60°C.
The Arrhenius equation provided under section 2.1.4. of EU Regulation No. 10/2011 (EU Citation2011) can be used for the determination of the specific testing conditions for long-term storage at ambient temperature and can be used to adapt the migration testing to more realistic temperature conditions. As a worst-case activation energy of diffusion 80 kJ mol–1 for 2-aminobenzamide is assumed within this approach. Based on the Arrhenius equation the equivalent to 10 days at 60°C would be 60 days at 40°C. Therefore, the migration of 2-aminobenzamide into mineral water was also determined after storage for 60 days at 40°C. As a result it was found that the concentrations of 2-aminobenzamide in the mineral water after storage for 60 days at 40°C were borderline in relation to the legal SML of 50 µg l–1. Some of the mineral water samples showed concentrations of 2-aminobenzamide which are even slightly above this migration limit. In addition retain samples from a German mineral water filling company stored at RT in the dark were also analysed for the concentration of 2-aminobenzamide in the mineral water. Under these realistic storage conditions the specific migration of 2-aminobenzamide was in all cases in compliance with the legal migration limit ().
The determined diffusion coefficients () can be used for the prediction of the migration of 2-aminobenzamide in water and EU food simulant C as well as for foods which do not cause swelling effects in the PET polymer. The predicted migration into mineral water at 23°C (diffusion coefficient DP = 4.2 × 10−16 cm2 s–1) into 500, 1000 and 1500 ml bottles is shown in . From such correlations the maximum bottle wall concentration for 2-aminobenzamide for a given storage time (e.g., the shelf life) can be derived. For example, the migration limit of 2-aminobenzamide in a 500 ml PET bottle stored at 23°C with a bottle wall concentration of 200 mg kg–1 is reached after 975 days of storage (the green line in )). Under the same storage conditions, the migration limit is reached after 244 days when the bottle wall concentration is 400 mg kg–1 (the blue line in )). This makes it very obvious that the bottle wall concentration plays the crucial role for the level of migration of 2-aminobenzamide into mineral water. This suggests that, vice versa, the bottle wall concentration of 2-aminobenzamide can serve as an important parameter for production control. For example, the SML of 2-aminobenzamide for a 500 ml bottle after storage for 1 year (365 days) is not exceeded if the bottle wall concentration is below 327 mg kg–1. Similar correlations are given in and for the migration of 2-aminobenzamide at 40°C into mineral water and 20% ethanol, respectively. As expected, the migration at a storage temperature of 40°C is significantly higher in comparison with storage at 23°C. The predicted migration into mineral water at 40°C (diffusion coefficient DP = 4.2 × 10−15 cm2 s–1) into 500, 1000 and 1500 ml bottles is given in . From this graph, it is obvious that the SML is reached for a 500 ml bottle with a concentration of 2-aminobenzamide of 200 mg kg–1 after 98 days and that a bottle wall concentration of 400 mg kg–1 would reduce the time to reach the SML to only 24 days at 40°C. The predicted migration into 20% ethanol 40°C (diffusion coefficient DP = 7.7 × 10−15 cm2 s–1) into 500, 1000 and 1500 ml bottles is shown in .
Figure 3. (colour online) Predicted migration of 2-aminobenzamide into mineral water at 23°C as a function of the bottle wall concentration (calculated with DP = 4.2 × 10–16 cm2 s–1, partition coefficient K = 1, bottle wall thickness l = 300 µm, density of PET = 1.4 g cm–3): (a) 500 ml bottle (surface area = 420 cm2), (b) 1000 ml bottle (surface area = 660 cm2) and (c) 1500 ml bottle (surface area = 840 cm2).
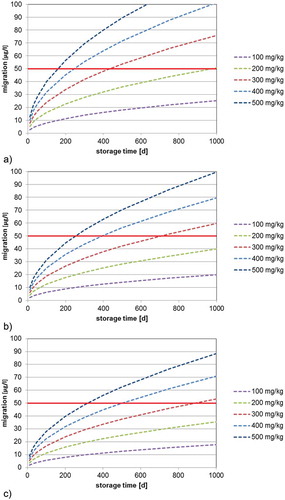
Figure 4. (colour online) Predicted migration of 2-aminobenzamide into mineral water at 40°C as a function of the bottle wall concentration (calculated with DP = 4.2 × 10–15 cm2 s–1, partition coefficient K = 1, bottle wall thickness l = 300 µm, density of PET = 1.4 g cm–3): (a) 500 ml bottle (surface area = 420 cm2), (b) 1000 ml bottle (surface area = 660 cm2) and (c) 1500 ml bottle (surface area = 840 cm2).
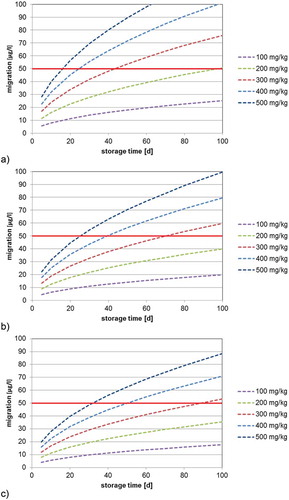
Figure 5. (colour online) Predicted migration of 2-aminobenzamide into 20% ethanol at 40°C as a function of the bottle wall concentration (calculated with DP = 7.7 × 10–15 cm2 s–1, partition coefficient K = 1, bottle wall thickness l = 300 µm, density of PET = 1.4 g cm–3): (a) 500 ml bottle (surface area = 420 cm2), (b) 1000 ml bottle (surface area = 660 cm2) and (c) 1500 ml bottle (surface area = 840 cm2).
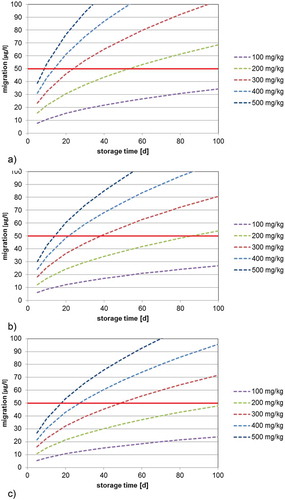
The findings of this study have consequences for migration testing. EU Regulation No. 10/2011 (EU Citation2011) prescribes short-term or accelerated test conditions at elevated temperatures for long-term packaging applications because the ideal migration test, i.e., under realistic contact conditions is not manageable for applications like mineral water bottles due to the potentially long storage times. Therefore, an accelerated test, at best at not too high temperatures, is required to allow realistic migration testing within reasonable time frames. In addition, 2-aminobenzamide is typically used as an acetaldehyde scavenger only in PET bottles for contact with natural mineral water. Therefore, water itself can be considered to be the best food simulant for compliance testing. The EU test scheme foresees here temperatures of 40 and 60°C. A temperature of 60°C represents a strong test temperature, which may already lead to swelling effects even in contact with water and which may cause physical changes like deformation of thin PET bottle wall structures. A temperature of 40°C is much closer to realistic storage temperature and was therefore selected for the objectives of this study. The comparison of the migration results between testing times at 23 and 40°C result directly into acceleration factors. For example, a concentration of 10 µg l–1 in mineral water is reached after 39.9 days at 23°C. At 40°C the same concentration is reached after 4.1 days (mineral water) and 2.2 days (20% ethanol), respectively. From these data migration testing acceleration factors of 9.7 and 18.1 can be derived for contact with mineral water and 20% ethanol, respectively. These factors can be applied to any long-term storage conditions of real market applications to define the corresponding accelerated test condition depending on the type of simulant. For comparison, EU Regulation No. 10/2011 foresees accelerated testing for long-term storage applications on the basis of a worst-case activation energy of diffusion of 80 kJ mol–1, which means an acceleration factor of 5.9 from realistic storage condition at RT (23°C) to a test temperature of 40°C. In consequence, the EU regulatory acceleration factor is by a factor of 1.6 (= 9.7/5.9) more conservative than measured in this study using mineral water, i.e., without swelling effects. In case, the EU factor would be applied to a test using 20% ethanol (simulant C) then the EU test would be more conservative by a factor of 3.1 because the EU calculation ignores swelling effects as observed and measured in this study when using 20% ethanol.
Conclusions
Diffusion coefficients of 2-aminobenzamide in PET bottle wall material were determined in contact with the food simulants 20% ethanol and with natural mineral water both at 23 and at 40°C. At 23°C both media do not have swelling effects on PET which is getting evident from the fact that diffusion coefficients are very similar. However, 20% ethanol at 40°C causes some swelling of the PET polymer which gets evident from a significantly higher diffusion coefficient compared to the water derived value. The experimentally derived diffusion coefficients obtained with water can be used to calculate migration of 2-aminobenzamide into PET bottled water under any realistic long-term storage conditions. From comparison of migration values between 23 and 40°C acceleration factors of 9.7 when using water as contact medium and 18.1 for 20% ethanol as simulant can be derived for definition of appropriate accelerated test conditions at 40°C. The EU regulatory acceleration test based on 80 kJ mol–1 as conservative activation energy overestimates the experimentally determined acceleration rates by a factor of 1.6 and 3.1, without swelling and with a slight swelling effect, respectively.
The most economical way of compliance evaluation appears to be (1) measurement of the free 2-aminobenzamide concentration in the bottle wall and (2) calculation of the migration for RT (or similar) for long-term storage. In this way, the bottle wall concentration of 2-aminobenzamide can be taken as an easily measurable compliance check parameter against the EU legal SML value of 50 µg l–1 (). Finally, it should be noted that the parameters and factors experimentally determined in this study refer to the evaluation of 2-aminobenzamide migration from PET bottle wall material into mineral water only. However, as general remark for potentially relevant for migration evaluation of other substances from PET, it can be stated that ethanol in higher concentrations than 20% in water may cause swelling effects which are further increased by the contact temperature with the effect that obtained migration values tend to overestimate the migration into the beverage or foodstuff to be simulated unless the product itself contains higher alcohol levels.
Disclosure statement
No potential conflict of interest was reported by the authors.
Additional information
Funding
References
- Buettner A, Schieberle P. 2001. Evaluation of aroma differences between hand-squeezed juices from Valencia Late and navel oranges by quantitation of key odorants and flavor reconstitution experiments. J Agric Food Chem. 49:2387–2394.
- Crank J. 1975. The mathematics of diffusion. Oxford: Oxford University Press.
- European Union (EU). 2004. Commission regulation (EU) no 1935/2004. Off J Eur Communities. L338/4.
- European Union (EU). 2011. Commission regulation (EU) no 10/2011. Off J Eur Communities. L12/1.
- Ewender J, Franz R, Mauer A, Welle F. 2003. Determination of the migration of acetaldehyde from PET bottles into non-carbonated and carbonated mineral water. Deut Lebensm-Rund. 99:215–221.
- Food contact notification (FCN) 2001 Jun 6. Food contact notification (FCN) no. 137, US Food and Drug Administration.
- Franz R, Welle F. 2008. Migration measurement and modelling from poly(ethylene terephthalate) (PET) into softdrinks and fruit juices in comparison with food simulants. Food Add Cont: Part A. 25:1033–1046.
- Mrozinski BA, Kim Y-W, Lofgren EA, Jabarin SA. 2013. Chemistry of the interactions of acetaldehyde scavengers for poly(ethylene terephthalate). J Appl Polym Sci. 130:4191–4200.
- Mrozinski BA, Lofgren EA, Jabarin SA. 2012. Acetaldehyde scavengers and their effects on thermal stability and physical properties of poly(ethylene terephthalate). J Appl Polym Sci. 125:2010–2021.
- Reimann C, Birke M, Filzmoser P. 2010. Bottled drinking water: water contamination from bottle materials (glass, hard PET, soft PET), the influence of colour and acidification. Appl Geochem. 25:1030–1046.
- Reimann C, Birke M, Filzmoser P. 2012. Temperature-dependent leaching of chemical elements from mineral water bottle materials. Appl Geochem. 27:1492–1498.
- Roduit B, Borgeat CH, Cavin S, Fragniere C, Dudler V. 2005. Application of finite element analysis (FEA) for the simulation of release of additives from multilayer polymeric packaging structures. Food Add Cont: Part A. 22:945–955.
- US Federal Food, Drug, and Cosmetic Act (USFDA) 2015 Apr 1. US federal food, drug, and cosmetic act, 21 CFR 174.5.
- Welle F. 2014a. Polyethylene terephthalate (PET). Kunststoffe Int. 104:45–47.
- Welle F. 2014b. Food law compliance of poly(ethylene terephthalate) (PET) food packaging materials. In: Kompolprasert V, Turowski P, editors. Food additives and packaging. Chapter 16, ACS Series 1162. Oxford: Oxford University Press; p. 167–195.