ABSTRACT
To investigate the fate of pyrrolizidine alkaloids (PAs) during milk processing, milk of cows treated via rumen fistula with a mixture of 84% (w/w) ragwort (Jacobaea vulgaris, syn. Senecio jacobaea) and 16% narrow-leaved ragwort (Senecio inaequidens) was processed using laboratory scale heating systems with industrial settings. Pasteurised and sterilised (UHT) milk were produced, as well as set-type yoghurt and cheese. Samples were analysed for 29 PAs using LC-MS/MS, of which 11 PAs were detected above LOQ in the samples (0.1 µg l−1). Alterations in the PA concentration and composition between the standardised milk and the corresponding end-product(s) were evaluated. The heat treatments applied for pasteurisation and UHT sterilisation to prepare semi-skimmed consumption milk did not affect the PA levels in the end-products. In yoghurt, after fermentation of standardised milk (6 h, pH 4.4), 73% of total PAs were recovered. The PA concentration, specifically dehydrojacoline, was decreased, although not quantifiable, during cheese production. A further decrease of 38% during 6 weeks of ripening was observed. The results show that the PA concentration of natural contaminated cow’s milk is not affected by heat treatment applied for pasteurised and sterilised milk, but that microbial fermentation of the milk leads to a lowered PA concentration in yoghurt and cheese. This is probably due to microbiological degradation, since PAs are fairly stable under acidic conditions.
Introduction
Plants containing pyrrolizidine alkaloids (PAs), toxic secondary metabolites, present a potential risk for the health of humans and animals when ingested. Those PAs examined showed clear carcinogenic but also genotoxic properties, implying that a large margin of exposure (>104) is required to protect public health. EFSA evaluated the presence of these plant toxins in food and feed commodities and concluded that the current level of exposure of humans does not ensure this margin of exposure (EFSA (European Food Safety Authority) Citation2011). A recent study by EFSA (EFSA (European Food Safety Authority) Citation2016) showed that tea can currently be considered as the most important source of PAs exposure of humans. However, milk products cannot be excluded as a source of PAs: in a large survey for EFSA, 6–7% of randomly taken samples of fresh and UHT milk were contaminated with PAs with a highest level of 0.17 μg l−1 (Mulder et al. Citation2015).
It has been shown that PAs are transferred from animal feed to milk when dairy cows consume PAs containing weeds such as common ragwort (Jacobaea vulgaris syn. Senecio jacobaea) and narrow-leaved ragwort (Senecio inaequidens) as part of the roughage. A 0.1% transfer of total PAs to cow’s milk was observed by Hoogenboom et al. (Citation2011), which confirmed the results from an older study by Dickinson et al. (Dickinson et al. Citation1976; Hoogenboom et al. Citation2011). However, Hoogenboom et al. (Citation2011) also observed a selective excretion of certain PAs such as jacoline and some otonecine-type PAs in the milk. Therefore, the overall transfer strongly depends on the PA composition of the plants.
Cow’s milk is processed into various end-products for human consumption, including fresh and sterilised milk and fermented products, like yoghurt and cheese. It is unknown whether the applied heating and fermentation processes will lead to a reduction of the PA concentration and/or changes in the PA composition. A certain stability to processing can be assumed, given that Mulder et al. (Citation2015) detected PAs in heat-treated milk from retail in the EU and Kakar et al. (Citation2010) reported occurrence of PAs in qurut, a goat cheese from Afghanistan (Kakar et al. Citation2010).
To obtain a more detailed estimate of the exposure of consumers to PAs, it is important to take into account the potential fate of contaminants during processing. For this reason, milk from cows exposed to a mixture of 84% (w/w) common ragwort and 16% narrow-leaved ragwort was used to produce heat-treated consumption semi-skimmed milk (pasteurised and sterilised [UHT]), and the fermented products yoghurt and cheese. Alterations in the individual and total PA concentrations were assessed by comparing the PA concentrations in the standardised milk with those in the corresponding end-product.
Materials and methods
Animal study description
The animal study to obtain naturally PA-contaminated milk carried out in 2010 will be described in detail elsewhere. Shortly, the plant material used for this study was the same as that used in the study of Hoogenboom et al. (Citation2011). It consisted of a mixture of 84% (w/w) ragwort (Jacobaea vulgaris, syn. Senecio jacobaea) and 16% narrow-leaved ragwort (Senecio inaequidens) that was collected in June and July 2008 in the Netherlands, air dried, milled to 1 mm using a Peppink 200 AN grinding machine (Veerman, Olst, the Netherlands), and homogenised. Three multiparous Holstein-Friesian dairy cows (average live weight of 610, 720, and 790 kg; all third parity; producing 35, 24, and 41 kg milk day−1, respectively), equipped with rumen fistulas, were housed in a tie-stall barn. The test period consisted of a 4-day treatment period in which cows were administered 200 g of the dried plant material in the rumen through the fistula directly before morning feeding (once a day, around 10 am). On Day 4, 40 kg milk of the evening’s milking and 15 kg of the next morning’s milking were collected from the three animals and pooled to one milk sample of 55 L. The milk was stored at 8°C, immediately transported to NIZO, and processed within 2 h of arrival.
Milk processing
Milk processing equipment
The raw milk was processed in the NIZO Food Research pilot plant in Ede, the Netherlands. Heat treatments were carried out using the large or small laboratory scale heating systems developed by NIZO Food Research, with industrial settings. The Minicheeser was used for cheese making.
The principle of both heating systems is a continuous flow system consisting of a heat exchanger to heat the milk to the selected temperature, a holder section submerged in oil to keep the milk at the selected temperature, followed by a heat exchanger to cool the milk to the selected temperature. The dimensions of each system allow a flow of either 10 L h−1 in the large laboratory scale heating system and 5 L h−1 in the small laboratory scale heating system. The equipment does not allow homogenisation. A processing schedule and the processing setting conditions are given in .
The Minicheeser system allows cheese-making at a small scale using industrial scale manufacturing settings. It consists of a thermostable double-walled stainless steel vessel (10 L), round-shaped plastic moulds for cheeses of 250 g weight, and a cheese press. The vessel is equipped with curd-knives and stirring baffles, and contains inlet and outlet openings for water addition and whey drainage.
Preparation of skimmed milk and cream
shows the various treatments applied to the milk. Upon arrival at NIZO, the 55 kg raw milk was thermised, using the large laboratory scale heating system, to prevent deterioration of the milk before processing. Thermisation took place at 68°C for 13 s at a constant flow of 10 L h−1 with residence times in the heating and cooling section of 17 s using the large laboratory scale heating system. The thermised milk [1] was immediately cooled and centrifuged (Westfalia, GEA Westfalia Separator, Cuijk, the Netherlands) at 45°C into skimmed milk [2] (44 kg) and cream [3] (31% fat; 7.5 kg) and kept at 4°C for 18 h until further processing. Subsequently, the skimmed milk and cream were mixed by hand to the required fat percentage to prepare the three varieties of standardised milk for further processing to consumption milk, yoghurt, and cheese.
Pasteurised and sterilised (UHT) milk
Two aliquots of 2 kg standardised semi-skimmed milk [4] (1.5% fat) were pasteurised [5] (76°C; 15 s) or sterilised [6] (UHT; 140°C; 4 s) using the small laboratory scale heating system. The milk was immediately cooled to 4°C and two aliquots of 50 mL of each product were stored at −20°C until analysis.
Yoghurt
Five kilograms of standardised yoghurt milk (3.0% fat) was heated (93°C; 5 min), using the small laboratory scale heating system, and cooled to 42°C. The yoghurt milk was used for making set-type yoghurt, which is most often produced in countries other than the Netherlands. The standardised milk [7] was inoculated (0.02% v/v) with a concentrated commercial starter culture for the production of set-type yoghurt. This culture is a defined, commonly used starter culture and contains two strains of the species Streptococcus thermophilus and Lactobacillus delbrueckii subsp. bulgaricus. The yoghurt was kept at 42°C, a typical fermentation temperature for set-type yoghurt. The fermentation was stopped after 6 h when pH 4.4 was reached. The yoghurt [8] was gently stirred prior to sampling. Two 50 mL samples were stored at −20°C until analysis.
Cheese
Twelve kilograms of standardised cheese milk (3.5% fat) was pasteurised at 72°C for 15 s and cooled to 31°C using the large laboratory scale heating system. Ten kilograms of pasteurised cheese milk [9] was transferred to the Minicheeser cheese vessel and inoculated with a commercial mixed-strain mesophilic starter culture (0.04% v/v). This is an undefined but commonly used starter culture composed of various strains of the species Lactococcus lactis cremoris and Leuconostoc spp. Rennet of animal origin was added, with 0.43 g L−1 sodium nitrate and 0.7 g L−1 calcium chloride (both supplied by In2Food, Duiven, the Netherlands). After 56 min at 31°C, further processing in the Minicheeser system comprised of cutting, curd washing, scalding, draining, filling, and curd pressing.
A total of 4 kg first whey [10] was drained, leaving 6 kg of curd. The curd was washed with 2.1 L wash water, leaving a total volume of 8.1 kg. After draining about 8 kg (not measured) of second whey (including the 2.1 L wash water) [11], the remaining 1.1 kg cheese was formed into four cheeses of 250 g each [12–15]. Aliquots of 50 g of first and second whey were placed at −20°C until analysis.
The four cheeses of 250 g each were placed in a brine solution (17.5% sodium chloride) for 5 h. After brining, the cheeses were vacuum packed in moisture-impermeable foil and subsequently ripened in the warehouse for 6 weeks at 13°C and 88% relative humidity. A cheese was taken after 0 [12], 2 [13], 4 [14], and 6 [15] weeks of ripening and stored at −20°C until analysis.
Method of analysis of pyrrolizidine alkaloids in milk and dairy products
Chemicals and analytes
Analytical PA standards and the internal standard (IS) heliotrine were obtained from a number of commercial sources (Phytolab, Vestenbergsgreuth, Germany; Phytoplan, Heidelberg, Germany; Latoxan, Valence, France). Jacoline and florosenine were isolated from plant material by PRISNA (Leiden, the Netherlands) while riddelliine and riddelliine N-oxide were a gift of NCTR (Jefferson, AR). A total of 20 PA standards was available for the study ().
Table 1. MS/MS conditions used for the analysis of pyrrolizidine alkaloids in common ragwort (Jacobaea vulgaris) and narrow-leaved ragwort (Senecio inaequidens).
Sample preparation, extraction, and clean-up
The PA content in milk and milk products was assessed by means of LC-MS/MS as described by Mulder et al. (Citation2015), with minor adaptations (Mulder et al. Citation2015). Test portions of 3.0 mL thawed milk were transferred to 50 mL test tubes, 75 µL of the heliotrine IS solution (100 ng mL−1) was added, followed by 15 mL 0.2% formic acid solution and 5 mL hexane. The mixture was agitated for 30 min by means of a rotary tumbler and centrifuged for 15 min at 3500 g. The hexane layer, and most of the proteins that were present at the border between the aqueous and the hexane layer, were removed using a disposable pipette. The pH of the aqueous layer was adjusted to 10 by adding concentrated ammonia (25%) using a pH test strip. The sample was purified and concentrated by SPE over Strata-X 60 mg, 3 cc cartridges (Phenomenex, Torrance, CA). Cartridges were conditioned with 3 mL methanol and equilibrated with 3 mL water. After application of the sample extracts, the cartridges were washed with 3 mL 1% aqueous formic acid solution, followed by 3 mL 1% aqueous ammonia solution. The cartridges were dried under reduced pressure and eluted with 3 mL methanol. The eluates were evaporated to dryness under a gentle stream of nitrogen at 50°C in a heated water bath (TurboVap, Uppsalla, Sweden) and the dry residues were reconstituted in 500 µL water/methanol 9:1 (v/v) and transferred to mini UniPrep filtervials (Whatman, Maidstone, UK).
The PAs in the sample extracts were quantified by standard addition to each sample of a PA standard mixture (corresponding to 25 µg L−1) before sample clean-up. A 5-point calibration curve of PA standards (0–25 µg L−1) in blank milk was injected before the start and at the end of the study for check of linearity and quality control. The LOQ for the individual PAs and their N-oxides was 0.1 µg L−1 in milk, yoghurt, and cheese. LOQ was determined as lowest spiked concentration that fulfilled all validation requirements (Mulder et al. Citation2015). The results were corrected for the IS (heliotrine).
Instrumental analysis
Analysis of the milk and dairy products was performed on a Waters Acquity UPLC coupled to a Waters Xevo TQ-S tandem mass spectrometer (Waters, Milford, MA), operated in positive electrospray mode. A volume of 10 µL was injected on the LC-MS/MS system. Compounds were separated on a Waters UPLC BEH C18 150 × 2.1 mm, 1.7 µm analytical column, kept at 50°C and run at 0.4 mL min−1 with an acetonitrile/water linear gradient containing 6.5 mM ammonia. The gradient started at 100% water and the amount of acetonitrile was increased to 50% in 12 min. The gradient was changed back to the starting conditions in 0.2 min and the column was allowed to equilibrate for 2.8 min. MS/MS collision energy was optimised for each individual compound using reference standard solutions, and two precursors to product ion transitions were selected and incorporated in a MRM method. In total, 29 potentially relevant PAs (including some metabolites) from ragwort were included in the method (). All compounds could be characterised on the basis of retention time and fragmentation transitions. For those compounds for which no reference standard was available, a semi-quantitative (indicative) value was obtained by comparison with a closely related analogue (e.g. an isomer).
Results
The processing schedule for the milk from cows treated with ragwort is presented in . A total of 11 PAs, from 29 analysed, were detected in the dairy products by LC-MS/MS, as shown in . One sample was taken from each product and a single analysis for PAs was performed on each sample. The relative composition of the six major PAs detected in the milk, yoghurt, and cheese products are presented in .
Table 2. Pyrrolizidine alkaloids in samples of processed milk (µg L−1 and for cheese in µg kg−1)a.
Figure 2. Relative composition of the major PAs present in milk, yoghurt, and cheese products. Sample numbers are explained in .
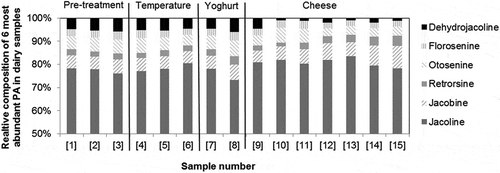
Fate of PAs in thermised milk, skimmed milk, and cream
The first step of commercial milk processing is separation of raw milk – or, as in this case, thermised milk [1] () – into skimmed milk (0.1% fat) [2] and cream (31% fat) [3]. Skimmed milk and cream are subsequently combined to standardised milk to obtain the required fat percentage for further use. Results for the concentrations of individual PAs in the samples are presented in . The results show that the total PA concentrations in thermised milk [1] and skimmed milk [2] are similar. The PA concentration was slightly reduced in the cream [3], which can be expected since PAs are highly water soluble and are not expected to readily distribute into the fat layer.
The PA profiles present in the thermised milk [1], skimmed milk [2], and cream [3] are comparable, as is shown in . The main contribution to the total PAs in all three products comes from jacoline (72–74%), followed by otosenine, jacobine, and dehydrojacoline (each around 4–6%).
Fate of PAs in heat-treated milk
Skimmed milk [2] and cream [3] were combined to standardised semi-skimmed milk [4] (1.5% fat) for preparation of pasteurised and sterilised consumption milk. Results for individual PAs are presented in . Comparing the total PA concentration in the starting material standardised semi-skimmed milk [4] with the two end-products, pasteurised semi-skimmed milk [5] and sterilised semi-skimmed milk [6], shows that neither heat treatment had any influence on the PA concentration in the end-products.
The PA profiles present in standardised semi-skimmed milk [4], pasteurised semi-skimmed milk [5], and sterilised semi-skimmed milk [6] are similar and do not differ from the starting material, thermised milk [1] ().
Fate of PAs in milk during yoghurt fermentation
Skimmed milk [2] and cream [3] were combined to standardised yoghurt milk [7] (3% fat) and given a heat treatment for the preparation of yoghurt (93°C; 5 min). This treatment is given in order to inactivate vegetative bacterial cells in the standardised milk and thus to allow an undisturbed fermentation. Fermentation of the standardised pasteurised yoghurt milk [7] by the bacteria typically used for yoghurt production, Streptococcus thermophilus and Lactobacillus delbrueckii subsp. bulgaricus, did result in a decrease of the PAs in set-type yoghurt [8], as presented in . About 73% of the total PAs were recovered in the yoghurt as compared to the standardised pasteurised yoghurt milk [7], after 6 h of fermentation at 42°C to pH 4.4 to produce set-type yoghurt. The decrease in the concentration of jacoline due to yoghurt fermentation was comparable to the total decrease in PA concentration. The reduction of PAs may result from lowered pH, bacterial activity, or a combination of the two. However, most likely it comes from microbiological action, since the experience obtained from working with PA analytical standard solutions and plant extracts is that the PAs in these solutions are stable for several months in the refrigerator at pH range of 4–8.
The PA profiles present in standardised pasteurised yoghurt milk [7] and yoghurt [8] are similar and not significantly different from the starting, thermised, milk [1]. The main contribution to the total PA content in both products is jacoline (68–73%), followed by otosenine, jacobine, and dehydrojacoline (each around 4–6%).
Fate of PAs in milk during cheese making
Standardised cheese milk [9] (3.5% fat) was prepared from skimmed milk [2] and cream [3] and pasteurised before processing into cheese. The heat treatment inactivates vegetative bacterial cells and allows safe and undisturbed curd formation and fermentation at 31°C. Results for PAs measured in the starting material, whey, and cheeses are presented in . presents the results based on the mass fractions of various products. The fate of PAs during cheese making was evaluated in two steps: first step from cheese milk [9] into whey [10 and 11] and cheese at t=0 [12]; and second step during cheese ripening over a period of 6 weeks [12 to 15].
Table 3. Pyrrolizidine alkaloids in cheese milk and subsequently produced whey and fresh cheese (µg L−1 and for cheese in µg kg−1).
The mass balance for the recovered volumes before and after processing could not be determined, as the results in show. The volume of second whey was not measured but is assumed to be 8 L. The total volume at start was 10 L of cheese milk. A volume of 2.1 L wash water was added during processing, which should have resulted in a theoretical total volume after processing of about 12.1 L or kg. The actual total volume recovered was 13.1 L (or kg), composed of 4 L first whey, 8 L second whey (in which the 2.1 L wash water is included), and 1.1 kg of cheese. Considering the assumed results presented in , about 86% of the PAs present in the pasteurised standardised cheese milk [9] was recovered in the first and second whey [10 and 11], and in the cheese at t=0 [12]. This may suggest that a reduction in PAs might be achieved during the first step of cheese making, 56 min, due to microbial activity. However, this should be confirmed in a repeated experiment where the exact volume of second whey is measured. The concentration of PAs in whey and cheese were comparable. The PA profiles of the six most abundant PAs, except for one, present in standardised pasteurised cheese milk [9], first whey [10], second whey [11], and cheese at t=0 [12] are similar to the profile found in whey and cheese (). Dehydrojacoline, however, was present at a much lower concentration in the whey and cheese samples. We have no explanation for this.
During the 6-week ripening of the cheese, the total PA concentration decreased by 38% (from 13.5 to 8.4 µg kg−1, ). The relative contribution of jacoline to the total PA concentration did not significantly change during the ripening process. The PA profiles present in the cheeses at t=0 weeks [12], t=2 weeks [13], t=4 weeks [14], and t=6 weeks [15], are all comparable. The main contribution to the total PAs in all four cheeses is jacoline (76–80%), followed by jacobine (6–10%), otosenine, and retrorsine (3–4%).
Discussion
The experiments show that microbial activity may lead to a certain degradation of PAs. The bacteria used in yoghurt production seem to degrade jacoline, while the bacteria used for cheese making tend to show a preference for degradation of dehydrojacoline. However, it should be taken into consideration that the experiments were not repeated and a single analysis for PA concentration was carried out.
In the framework of a European survey funded by EFSA, Mulder et al. (Citation2015) reported on the presence of PAs in, among other products, consumption milk (skimmed milk, semi-skimmed milk, whole fat milk, pasteurised, UHT sterilised, cow’s and goat’s milk) retrieved from retail stores in the EU. PAs were detected in 6% (11 samples) of the milk samples in the range of 0.05–0.17 µg PAs L−1. The PAs detected in milk were senkirkine and otosenine, each in three samples; jacoline, in two samples; lycopsamine and retrorsine, in one sample; and one sample contained both lycopsamine and echimidine. The type of PAs found in the milk samples in the EFSA study indicated the consumption of, notably, Senecio and to a lesser extent Boraginaceae spp. by the animals. The total PA concentration detected in the samples in the EFSA survey was 100–400 times lower than the total PA concentrations detected in the study described here. This is most probably the result of low intake of PA-containing plants by grazing animals. These results of the EFSA study, PAs present in several milk samples, confirm the conclusion that PAs are stable to thermal treatments. This means that control of PA-containing plants in feed – e.g. according to the Codex Alimentarius CAC/RCP 74–2014 code of practice for weed control to prevent and reduce pyrrolizidine alkaloid contamination in food and feed – is the most effective measure to lower the risk of presence of PAs in dairy (Codex Alimentarius Citation2014).
In the same EFSA-supported study, Mulder et al. (Citation2015) reported none of 25 yoghurt samples from retail with PA concentrations above the LOQ. This outcome is to be expected, given that the EFSA survey showed that the occurrence of PAs in milk is already low; a further reduction of PAs during yoghurt fermentation, as shown in the study presented here, will easily bring the PA concentrations to below the LOD.
This study shows that PAs can easily be transferred to whey during cheese making. Provided PAs are stable to the conditions applied during drying of whey, they might be present in whey powder. Whey powder is often used as ingredient in infant food formula. Therefore, Mulder et al. (Citation2015) analysed 25 samples of infant food formula in the EFSA-supported study, all taken from retail in the EU, but no PAs were detected above the LOD.
Conclusions
Results from a single experiment using milk obtained from cows treated via rumen fistula with a mixture of ragwort (J. vulgaris, syn. S. jacobaea) and narrow-leaved ragwort (S. inaequidens) show that industrial heating processes to prepare pasteurised milk (76°C; 15 s) and UHT sterilised milk (140°C; 4 s) had no influence on the PA concentration in the final products. The fermentation process for set-type yoghurt resulted in a decrease of the total PA concentration to 73% in the end-product. When using the naturally contaminated milk for commercial cheese making, PAs were transferred to the whey. A reduction, not quantifiable, of total PAs was seen in the first step of the process. The concentration of specifically dehydrojacoline was lowered much more quickly than the concentration of the other PAs. PA concentrations further decreased to about 62% during the subsequent 6 weeks of cheese ripening. It is advised to carry out follow-up experiments to determine the specific effects of bacterial cultures used for commercial dairy processing on PA concentrations in milk products. However, management practices to reduce the presence of feed with PA-containing weeds will be the most effective way to reduce exposure to PAs.
Disclosure statement
No potential conflict of interest was reported by the authors.
Additional information
Funding
References
- Codex Alimentarius. 2014. Code of practice for weed control to prevent and reduce pyrrolizidine alkaloid contamination in food and feed. CAC/RCP. 74-2014:1–7.
- Dickinson JO, Cooke MP, King RR, Mohamed PA. 1976. Milk transfer of pyrrolizidine alkaloids in cattle. J Am Vet Med Assoc. 169:1192–1196.
- EFSA (European Food Safety Authority). 2011. Scientific opinion on pyrrolizidine alkaloids in food and feed. EFSA J. 9(11):2406:133.
- EFSA (European Food Safety Authority). 2016. Dietary exposure assessment to pyrrolizidine alkaloids in the European population. EFSA J. 14(8):4572:50.
- Hoogenboom LAP, Mulder PPJ, Zeilmaker MJ, van den Top HJ, Remmelink GJ, Brandon EFA, Klijnstra M, Meijer GAL, Schothorst R, van Egmond HP. 2011. Carry-over of pyrrolizidine alkaloids from feed to milk in dairy cows. Food Addit Contam A. 28:359–372.
- Kakar F, Akbarian Z, Leslie T, Mustafa ML, Watson J, van Egmond HP, Omar MF, Mofleh J. 2010. An outbreak of hepatic veno-occlusive disease in Western afghanistan associated with exposure to wheat flour contaminated with pyrrolizidine alkaloids. J Toxicol. 2010:1–7. Epub 2010 Jul 24.
- Mulder PPJ, Lopez P, These A, Preiss-Weigert A, Castellari M. 2015. Occurrence of pyrrolizidine alkaloids in food. EFSA Supporting Publication. EN-859:114.