ABSTRACT
An HPLC method with fluorescence detection has been developed and validated for the quantification of six fluorescent whitening agents (FWA) in plastic beverage cups after extraction and in food simulants after migration at 70°C for 2 h. The sensitivity of the method was high with LODs ranging from 0.053 to 0.251 μg kg−1 and LOQs from 0.107 to 0.504 μg kg−1. Accuracy and precision were highly acceptable, with recoveries greater than 82% and RSDs (%) below 16%. The expanded combined uncertainty was found to be less than 23% for the measurements of all studied FWAs. In extracting the analytes from food contact materials (FCM), accelerated solvent extraction (ASE) and Soxhlet extraction were applied using ethanol as the extraction solvent. The results obtained for FWA in 10 different food plastic cups, made from different polymers, were compared. The ASE technique proved to be faster, more effective and efficient than Soxhlet extraction. Migration tests with official food simulants from Regulation (EU) No 10/2011 showed that the substances studied could potentially migrate using the selected migration conditions. The most pronounced effect was observed in case of simulant D1 (50% w/v ethanol in water). The analytical method proved to be a simple, fast, sensitive and reliable tool for the simultaneous quantification of six of the most used FWAs in both FCM extracts and food simulants after migration experiments.
Introduction
Fluorescent whitening agents (FWAs) are chemical compounds that can improve the whiteness and brightness characteristics of a material. These substances are commonly used in the production of textiles, paper, board and plastics, although they could also be used in other types of products, such as laundry detergents (Wu et al. Citation2016a). FWAs are often used in the production of plastic food packaging, mainly where transparency and clarity are the desired properties for a number of products. The applications involving the use of FWAs are also increasing due to the affordable price of polypropylene (PP) (Amine Fourati et al. Citation2011).
Many hundreds of FWAs are described to be available worldwide. However, only about 400 are currently being used, particularly in the manufacturing of paper and board packaging (Wu et al. Citation2016b). Among the different chemical families of FWAs, the most common are coumarins, imidazolines and stilbene derivatives, especially the biphenyl-stilbene ones (De Los Santos et al. Citation2005; Wu et al. Citation2016b). Regarding the toxicity of these substances, the few available studies are not conclusive. The potential dermal toxicity of some of these additives (Mathur and Khanna Citation2002) has been reported, and some studies state only the existence of low-to-zero toxicity effects associated with FWAs (De Los Santos et al. Citation2004; Yang et al. Citation2017). Additionally, in 2001, the German Institute for Consumer Health Protection and Veterinary Medicine (BgVV) published a review of toxicity studies for this class of additives and concluded that these substances did not pose any risks to consumers (BgVV Citation2011). However, in other publications, FWAs are presented as being readily absorbable by the organism but difficult to digest, such that it could lead to bioaccumulation and higher final levels in the human body (De los Santos et al. Citation2005; Guo et al. Citation2013; Wu et al. Citation2016b; Yang et al. Citation2017). As these substances exist in food contact materials (FCMs), they may potentially migrate into food with unknown consequences to human health. In some Asian countries, the use of FWAs in food contact paper has been banned (Wu et al. Citation2016b; Yang et al. Citation2017).
In the European Union, Regulation (EU) No 10/2011 establishes specific migration limits (SMLs) for some stilbene-based FWAs on plastic materials and articles that may come in contact with food (EC Citation2011). These compounds are 4,4ʹ-bis(2-benzoxazolyl)stilbene (FCM 422), 2,5-bis(5-tert-butyl-2-benzoxazolyl) thiophene (FCM 500), 4-(2-benzoxazolyl)-4ʹ-(5-methyl-2-benzoxazolyl)stilbene (FCM 919) and 4,4ʹ-bis(5-methyl-2-benzoxazolyl)stilbene (FCM 920). The latter two are not included individually in the positive list of the EU regulation, but are part of the mixture, FCM 65, comprising 4-(2-benzoxazolyl)-4′-(5-methyl-2-benzoxazolyl) stilbene (FCM 919), 4,4′-bis (2-benzoxazolyl) stilbene (FCM 422) and 4,4′-bis(5-methyl-2-benzoxazolyl)stilbene (FCM 920). Mixture FCM 65 cannot be used at greater than 0.05% (w/w) in the plastic and its formulation from the manufacturing process should have a typical ratio of (58–62%):(23–27%):(13–17%), respectively (EU Citation2011; European Commission Citation2017). While for the individual use of FCM 422 an SML of 0.05 mg kg−1 has been set in the Regulation; no SML has been established for the mixture FCM 65 (EU Citation2011; European Commission Citation2017).
The availability of a simple, rapid and sensitive analytical method for the determination of FWAs in simulants after migration experiments from food contact plastics is essential for monitoring, evaluating and enforcing Regulation (EU) No 10/2011 (EC Citation2011; Guo et al. Citation2013). Few analytical methods for the determination of stilbene-based FWAs have been reported in the literature, comprising mainly UPLC-tandem MS methods (Guo et al. Citation2013; Wu et al. Citation2016a, Citation2016b), HPLC with fluorescence detection (FLD) (De Los Santos et al. Citation2005), ion-pair HPLC with FLD (Shu and Ding Citation2005) and HPLC with MS detection (Chen et al. Citation2006). Of the studies mentioned, only Wu et al. has conducted an investigation to determine FWAs in four food simulants and food containers, namely FCM 422 (FWA 393), FCM 500 (FWA 184) and FCM 919 (FWA 368) (Wu et al. Citation2016a). The others reported the determination of FWAs in extracts from paper samples (De los Santos et al. Citation2005; Wu et al. Citation2016b) and from polyvinylchloride (PVC) plastics (Guo et al. Citation2013).
Some authors have reported liquid-solid extraction (LSE) (De Los Santos et al. Citation2005; Wu et al. Citation2016b), Soxhlet extraction (De los Santos et al. Citation2005), solid-phase extraction (Shu and Ding Citation2005; Chen et al. Citation2006) and liquid-liquid extraction after the dissolution of the FCM (Guo et al. Citation2013) as effective extraction techniques for FWAs from food and non-FCMs. Accelerated solvent extraction (ASE) is considered as quite efficient when compared to Soxhlet extraction, but has not previously been reported in the extraction of these substances from FCM.
This study aimed to develop and validate a simple and rapid HPLC method with fluorescence detection that could be used for the simultaneous determination of four EU regulated FWAs and two others commonly used as additives in the paper and board FCMs. The method was validated in terms of sensitivity, linearity, accuracy and precision. It has been applied to extracts and migration solutions of different types of commercially available beverage cups comprising three different types of plastics, namely polypropylene (PP), polyethylene terephthalate (PET) and polystyrene (PS).
Materials and methods
Chemicals and reagents
Methanol, acetonitrile, ethanol and chloroform were of HPLC gradient grade purity and were obtained from Sigma Aldrich (Steinheim, Germany). Ultrapure water (18.2 MΩ) was obtained from a Milli-Q system (Millipore, Bedford, USA). 4,4ʹ-bis (2-benzoxazolyl)stilbene (97%) and 2,5-bis(5-tert-butyl-2-benzoxazolyl)thiophene (99%) were purchased from Sigma Aldrich (Buchs, Switzerland). The compounds 1,4-bis-(2-benzoxazolyl)-naphthalene (>98%), 1,2-bis (5-methyl-2-benzoxazole) ethylene (>98%), 4-(2-benzoxazolyl)-4ʹ-(5-methyl-2-benzoxazolyl) stilbene (>95%) and 4,4ʹ-bis (5-methyl-2-benzoxazolyl) stilbene (>96%) were purchased from TCI Chemicals (Tokyo, Japan). The chemical formula of the compounds studied, along with their SML, CAS number and chemical structures, are presented in .
Table 1. Chemical structures of the FWAs studied.
Preparation of standard solutions
Stock solutions containing 100 μg mL−1 of each FWA were prepared using chloroform as the solvent. Appropriate working solutions were then prepared with methanol. Stock solutions were stored at −20°C, while the working standards were kept at 4°C. Fresh working standards were produced every week. Both stock and working standard solutions were prepared in amber vials to prevent light-induced degradation or isomeric conversion.
Plastic cup samples
Ten different plastic cups were bought from selected stores. All samples were coloured, and some of them were marketed as ‘fluorescent cups’. Three of the cups were made of PP, one cup was produced from recycled PET and the six others were made of PS.
Instrumentation
An ASE extraction system Dionex ASE350 (Dionex Corporation, Sunnyvale, CA, USA) was used. The chromatographic system was an Agilent Technologies 1200 series HPLC system (Waldbronn, Germany) equipped with a thermostatic column compartment, an autosampler, a Diode Array Detector (DAD) and a Fluorescence Detector (FLD). The chromatographic column was a Thermo Scientific HyPURITY C18 150 × 3.0 mm, 5 μm particle size (Thermo Fisher Scientific Inc., USA) which was thermostatted at 40°C. Separation of the target analytes was performed with a linear gradient elution programme using a mobile phase of a mixture of acetonitrile (A) and ultrapure water (B). The gradient elution programme was optimised as follows: an isocratic step with 30% B, from t = 0 min to t = 23 min, followed by a gradient decrease of B to 5% (t = 27 min) and an equilibration post-time of 6 min to reach the initial conditions prior to the next injection. The injection volume was set at 25 μL, and target analytes were measured using emission and excitation wavelengths that were studied and established for the best sensitivity for all the analytes (λexc. = 365 nm, λem. = 480 nm).
Sample preparation
Ethanol was selected as the extraction solvent for the establishment of the initial concentrations of the six FWAs in all three types of plastic beverage cups.
Two extraction methods were applied. First, a typical Soxhlet extraction where a portion of 0.5 g of the plastic cups was cut into small pieces, ground, homogenised and inserted in a Soxhlet extraction cartridge. The volume of ethanol was 100 mL and the extractions were conducted for 96 h. The extraction solutions were then made up to volume (100 mL) and filtered through PTFE 0.22 μm filters. Injection samples were prepared by concentrating 1:10 of the initial volume, re-filtering, and directly injected into the chromatographic system.
The protocol for the sample preparation by ASE extraction involved cutting the FCM test sample (app. 2 g) to small pieces (1.0 × 1.0 mm), and then grinding, homogenising and finally mixing the sample thoroughly with 2 g of inert glass balls. The mixture was placed in a 10 mL stainless steel ASE cell and extracted with ethanol, at an oven temperature of 100°C and a 5 min heat-up time under a pressure of 1500 psi. Then five static cycles were used with a static time of 3 min. The cell was finally rinsed with ethanol (40% of cell volume) and purged with nitrogen for 60 s (125–150 psi). The sample preparation procedure for the ASE extracts was the same as for the Soxhlet extracts.
Migration into food simulants
For the migration conditions, all the selected plastic beverage cups were expected to come in contact with beverages for up to 2 h with a contact temperature as high as 70°C. In fact, some of the cup manufacturers indicated on the label the possibility of using the product at up to 70°C for 2 h, and others advertised the suitability of the cups to be used for alcoholic content beverages. According to Regulation (EU) No 10/2011 (EU Citation2011), the conditions of contact for the ‘worst foreseeable use’ are set at 70°C for 2 h. Therefore, these conditions were selected for the migration test, according to EN 13130:2004 (EN 13130. Citation2004) and Regulation (EU) No 10/2011(EU Citation2011). All the cups were tested with food simulants A (10% v/v ethanol), C (20% v/v ethanol) and D1 (50% v/v ethanol) (EU Citation2011).
According to the Regulation (EU) No 10/2011, food simulant D1 is mimicking food that has a lipophilic character (as it can extract lipophilic substances), as well as for food with an alcohol content of above 20% and for oil-water emulsions. Food simulants A and C are assigned for foods that have a hydrophilic character and can extract hydrophilic substance (EU Citation2011).
Method validation
Prior to method validation, an examination of experimental conditions in standard solutions (0.5 ng μL−1) was performed in terms of retention time (Rt), repeatability of peak area, number of theoretical plate (N), retention factor (k), separation factor (a), resolution factor (Rs), and tailing factor (T), obtained graphically from the chromatograms.
Calibration curves with six points were constructed using mixtures of standard solutions, covering a concentration range from 0.1 to 10,000 μg kg−1. Equations were obtained by linear regression analysis of the peak area versus the spiked concentration. Calibration curves were constructed with food simulants (A, C and D1), spiked with standard solutions, vortexed, allowed to stand for 2 h at room temperature and analysed with the developed method.
In validation of the method, the present study followed the guidelines for performance and validation procedures of analytical methods used in controls of FCMs, regarding sensitivity, linearity, trueness and precision (Bratinova et al. Citation2009). In all the existing cases, the SMLs were taken into account regarding sensitivity, trueness and precision evaluation. The limit of detections (LOD) must be 10 times lower than the Legislative Limit (LL), if possible, or at least 5 times lower than the LL. The LOD and the limit of quantification (LOQ) have been calculated from the signal-to-noise (S/N) ratio with corresponding ratios of 3 and 6, respectively (Bratinova et al. Citation2009). Regarding the evaluation of trueness, a recovery study has been performed, while the precision has been evaluated in terms of repeatability and reproducibility, expressed as within-day precision (short-term repeatability) and between-day reproducibility (intermediate precision) by the relative standard deviations (RSD, %). The concentration levels have been selected based on the LLs (0.2 LL; 1 × LL; 2 × LL), where applicable. For the compounds without an LL, the selection of the tested concentrations was based on the calibration’s linear range by selecting a low, medium and high concentration level. The expanded combined uncertainty (U) was calculated by combining bias and the standard uncertainties (ui) of repeatability and intermediate precision results (ANOVA), taking a coverage factor (k) of 2 into account (Bratinova et al. Citation2009; Eurachem/Citac Citation2012).
Data processing and statistical analysis
Data were processed with the Agilent Chemstation application (Agilent, Germany), whereas regression analysis and validation data evaluation have been performed using Microsoft Excel. All statistical analysis, including normality tests, homogeneity of variances and ANOVA were conducted using Minitab statistical software (Minitab Inc.).
Results and discussion
Optimisation of the HPLC-FLD method
Acetonitrile was used in the mobile phase instead of methanol because the separation and the resulting peaks shapes were better in terms of sharpness and symmetry. A typical HPLC-FLD chromatogram is presented in .
Figure 1. HPLC-FLD chromatogram of Simulant D1 fortified with FWAs standard mixture.
Peak order and mass fractions: 1 = 1,2-bis (5-methyl-2-benzoxazole) ethylene, 3.6 min, 3.0 µg kg−1; 2 = 1,4-bis-(2-benzoxazolyl)-naphthalene, 12.4 min, 3.0 µg kg−1; 3 = FCM 422, 14.4 min, 5.0 µg kg−1; 4 = FCM 919, 20.2 min, 5.0 µg kg−1; 5 = FCM 920, 21.2 min, 5.0 µg kg−1; 6 = FCM 500, 21.7 min, 5.0 µg kg−1.
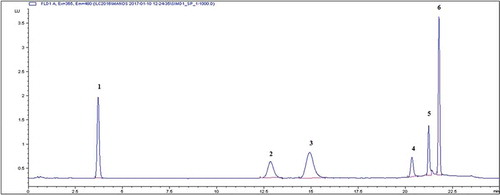
For the analysis of the six FWAs, the analytical method presented has the main advantages of being a cost-effective and simple method, but at the same time being highly sensitive to the target substances and comparable to UPLC-MS/MS systems. A literature survey revealed that few methods have been reported for this type of analysis, mostly involving the use of UPLC-MS/MS for food packaging coated papers analysis (Wu et al. Citation2016b), after migration with food simulants in food contact plastic-packaging containers (Wu et al. Citation2016a) and as residual concentration in PS and PVC plastics (Guo et al. Citation2013). None of the previously reported methods could simultaneously analyse the number of FWAs that the present method is capable of, an HPLC-FLD system, and only one of them analysed these substances in official food simulants.
In all the reported methodologies, the total analysis time was less than 8 min, but using an UPLC system, which is capable of total run times of only a few minutes. In the present method, the total run time is rather higher, reaching almost 23 min. However, the simplicity of the methodology, as well as the simplicity of the analytical system, should be taken into account. The method developed uses a standard HPLC-RP system, with a common C18 column (150 × 3.0 mm, 5 μm) rather than a more complex and expensive UPLC system. For the UPLC methods, it should also be noted that entirely different columns were used, such as a 10 cm C18 column with an internal diameter of 2.6 μm (Wu et al. Citation2016a), a UPLC BEH C18 5 cm of 1.7 μm (Guo et al. Citation2013) and a Luna C18 50 mm of 3 μm (Wu et al. Citation2016b). In all the cases, the internal diameter of the stationary phase was significantly lower than the one used in this study, which would result in not only a higher theoretical plate number and faster separation of the six FWAs, but also significant additional costs.
Method validation
Retention times (Rt) and peak areas of the six FWAs have been evaluated by performing 20 consecutive injections at a concentration of 300 μg kg−1. The results showed very good reproducibility, with RSDs being below 1.8%. The theoretical plates (N) were above 5000 for all the six FWAs, indicating that the chromatographic column was quite efficient. Resolution factors (Rs) were above 2 for all the target analytes, thus demonstrating good separations. In the case of peak symmetry, a good Gaussian peak shape could be observed in the chromatograms. Additionally, the tailing factor (T) was <1 for all the retained peaks (ranging from 0.84 up to 0.94) indicating good peak symmetry. All the respective results are summarised in .
Table 2. Combined method performance characteristics in fortified food simulants A, C and D1 with FWAs standard mixtures (300 μg kg−1).
The present method satisfied all the established criteria for the performance and validation of analytical methods used in the controls of FCMs regarding sensitivity, linearity, trueness and precision (Bratinova et al. Citation2009). The LODs reported were 10 times lower than the LL, where these exist. All the results on sensitivity and linearity are presented in .
Table 3. Linearity, linear range and sensitivity results of the method developed for food simulants A, C and D1.
The LODs and LOQs of the present method are lower compared with the other reported methods for the quantification of the six FWAs in food packaging paper samples, plastic materials after extraction, as well as PS and PVC plastic samples (Guo et al. Citation2013; Wu et al. Citation2016a, Citation2016b). The LOQs of the method presented here ranged from 0.107 μg kg−1 (FCM 500) to 0.504 μg kg−1 (FCM 422), hence lower compared to reported LOQs of 6 μg kg−1 for food packaging paper samples and 2.5 μg kg−1 and 5.0 μg kg−1 for PS and PVC plastic samples, respectively (Guo et al. Citation2013; Wu et al. Citation2016a).
The LOQs of the analytical method described here are much lower than the established SMLs in the Regulation (EU) No 10/2011 for FCM 422 (0.05 mg kg−1) and FCM 500 (0.6 mg kg−1) (EU Citation2011). Also FCM 65 could be quantified with this method, as it is a mixture comprising FCM 919, FCM No. 422 and FCM 920 (EU Citation2011; European Commission Citation2017). Finally, two other additional FWAs, 1,2-bis (5-methyl-2-benzoxazole)-ethylene and 1,4-bis-(2-benzoxazolyl)-naphthalene, could also be quantified at low μg kg−1 levels in food packaging. These substances have already been described in other research papers as occurring in FCMs, but are not yet regulated at the EU level (EU Citation2011; Guo et al. Citation2013; Wu et al. Citation2016b).
The present method represents a novel simple HPLC-FLD for the quantification of FWAs in FCM plastics and provides performance characteristics comparable to the only available UPLC-MS/MS study in the literature for the quantification of FCM 422, FCM 500 and FCM 919. The LODs and LOQs were similar, despite the fact that the UPLC-MS/MS method used substantial injection volumes (20 μL) for such a system (typically 5–10 μL), compared with a simple reversed phase HPLC-FLD system (Wu et al. Citation2016a). Both analytical techniques are capable of measuring at low μg kg−1 levels, hence sufficiently sensitive for the compliance checks of the SMLs, stated in Regulation (EU) No 10/2011(EU Citation2011).
Regarding precision and accuracy, the latter expressed as recoveries (R, %), two food simulants were tested, namely simulant A (10% v/v aqueous ethanol) and food simulant D1 (50% v/v aqueous ethanol). The recoveries were in all cases higher than 82%, and the RSDs for the within-day precision or between-day reproducibility did not exceed 16%. The expanded combined uncertainty was less than 23 % for the measurements of all studied FWAs. The corresponding results are presented in the supplementary information (Table S1).
Extraction on plastic beverage cups
Extraction of the plastic cups was tested with two different procedures, ASE and Soxhlet extraction. Ethanol was selected as the extraction solvent, as chlorinated solvents (e.g. dichloromethane and chloroform) could completely disperse and dissolve PS and PET samples using the selected ASE conditions. An extended 96 h Soxhlet extraction was employed. A fast and effective extraction protocol for the ASE extraction was developed and used with all the test specimens. In comparing the performance of the extractions ethanol was also selected as the extraction solvent. The results of both ASE and Soxhlet extractions are presented in .
Table 4. Mass fractions (μg per kg of plastic) of the tested FWAs in the tested plastic cup specimens as obtained after ASE and Soxhlet extraction with ethanol.
The results were statistically evaluated through a simple one-way ANOVA for the two applied extraction methods. They were significantly different statistically, with the ASE protocol appearing more efficient for all the target analytes and all the polymeric materials studied. There were considerable differences between the two methods, evidenced in the case of FCM 422, with the extraction efficiency of ASE being almost 8000 times higher than for the respective Soxhlet extraction. Other examples are FCM 919 and FCM 920 in PS3 plastic cups, where the FWAs were simply not extracted with Soxhlet, while their mass fraction levels appeared to be high (3.83 and 7.46 μg kg−1, respectively) when using ASE extraction. Overall, it can be concluded that the ASE extraction procedure was much more effective and efficient compared to the Soxhlet extraction, even if an extended time protocol was used for the latter (96 h). These differences may be explained by the aggressive extraction conditions used with ASE, as the high pressures used (1500 psi) allow the extraction solvents to be in their liquid state even at temperatures above their typical boiling point, thus decreasing viscosity and increasing the solubility of analytes in the selected solvents. These conditions are advantageous, especially with traditionally designed matrices such as polymeric materials. ASE with ethanol as an extraction solvent, proved to be a more suitable technique when extracting from polymers that cannot be treated with more aggressive solvents.
Besides the extraction protocol, it is worth mentioning that most of the tested cups, regardless of the material (PP, PS, PET), contain amounts of FCM 422, FCM 919 and FCM 920. According to Regulation (EU) No 10/2011, the latter two cannot be used as standalone additives, but only as a mixture with FCM 422 (named FCM 65), with a specified proportion, with a typical ratio of FCM 919 (58–62%), FCM 422 (23–27%) and FCM 920 (13–17%) (EU Citation2011). Sample PS3 may have been produced with FCM 65, although the mass fraction ratio of the substances is different from the Regulation. The other samples were made by using FCM 422 and 500. None of the EU regulated additives was individually found in the tested beverage contact cups. The FWAs 1,2-bis (5- methyl-2-benzoxazole)ethylene and 1,4 bis-(2-benzoxazolyl)-naphthalene were not detected in the analysed cups, either with Soxhlet or ASE extraction.
Migration with official food simulants
Different food simulants were tested to establish the migration potential of the target analytes, according to Regulation (EU) 10/2011 (EU Citation2011). The cups were intended to be used with alcoholic and non-alcoholic beverages; therefore simulants A, C and D1 containing 10% w/v, 20% w/v and 50% w/v ethanol in water were selected. The migration temperature for all the experiments was set at 70°C (± 2°C) for 2 h, in accord with the information on the cup labels and according to EN 13130:2004 (EN 13130. Citation2004).
FCM 500 was the only FWA quantified in simulant D1 in 2 PP cup samples, with mass fractions of 1.23 and 4.87 μg kg−1, substantially lower than the corresponding SML (0.6 mg kg−1) (EU Citation2011). For the PP and PS cups, no FWAs were found to migrate to any of the used food simulants. The aforementioned results are in accordance with the previous findings of Wu et al. for stilbene-based FWAs, who has shown that these substances could not easily migrate to food simulants with lower ethanol content (simulants A and C) (Wu et al. Citation2016a). These results reveal the high sensitivity of the method developed since it could quantify FCM 500 at very low levels found after migration.
Conclusions
There is seemingly an increasing use of FWAs with food contact plastics. Although 400 FWAs are described as being used in other FCMs, only four are EU regulated when used as food plastic additives. Several unregulated FWAs may be currently used in plastic FCMs. In assessing the level of application of the use of this class of substances, appropriate analytical methods are needed, with high selectivity and simplicity to be applicable for routine analysis by control laboratories.
The analytical method described here provided sufficient precision and accuracy for the determination of all six target FWAs in the selected matrixes at low mass fractions (μg kg−1). ASE extraction was used for the first time with these additives, and the comparison with results from traditional Soxhlet extraction demonstrated that the former is faster, more effective and efficient for all the target analytes throughout the different samples. The results obtained after migration with food simulants revealed that only FCM 500 could potentially migrate from PP into food simulant D1 (50% w/v ethanol in water).
The short period of analysis, good selectivity, high accuracy and precision, and simple sample preparation makes this method a useful tool for routine analysis to be used for the surveillance of the SMLs for the FWAs studied and regulated by Regulation (EU) No 10/2011.
Supplemental File
Download MS Word (18.5 KB)Disclosure statement
No potential conflict of interest was reported by the authors.
Supplementary material
Supplemental data for this article can be accessed on the publisher’s website.
References
- Amine Fourati M, Maris T, Skene WG, Bazuin G, Prud’homme RE. 2011. Photophysical, electrochemical and crystallographic investigations of the fluorophore 2,5-bis(5-tert-butyl-benzoxazol-2-yl)thiophene. J Phys Chem. 115:12362–12369.
- [BgVV] Bundesinstitut für gesundheitlichen Verbraucherschutz und Veterinärmedizin. 2011. Health Assessment of Certain Stilbene Derivatives. [accessed 2017 Jan 25]. https://www.bfr.bund.de/cm/349/stilbene_derivatives.pdf.
- Bratinova S, Raffael B, Simoneau C. 2009. Guidelines for performance criteria and validation procedures of analytical methods used in controls of food contact materials. Luxembourg: European Commission.
- Chen H-S, Wang S-P, Ding W-H. 2006. Determination of fluorescent whitening agents in environmental waters by solid-phase extraction and ion-pair liquid chromatography-tandem mass spectrometry. J Chromatogr A. 1102:135–142.
- De los Santos M, Nerin C, Domeno C, Batlle R. 2004. The analysis of fluorescent whitening agents using reversed-phase HPLC and mass spectrometry. LCGC North Am. 22:550–560.
- De los Santos M, Salafranca J, Nerin C. 2005. Subcritical water and dynamic sonication-assisted solvent extraction of fluorescent whitening agents and azo dyes in paper samples. J Chromatogr A. 1064:135–141.
- EN 13130. 2004. European Standard. Materials and articles in contact with foodstuffs.
- [EC] European Commission. 2011. Commission Regulation (EU) No. 10/2011 of 14 January 2011 on plastic materials and articles intended to come into contact with food. Off J Eur Comm. L 12:1.
- Eurachem/Citac. 2012. EURACHEM/CITAC Guide CG 4, Quantifying Uncertainty in Analytical Measurements, 2012. [accessed 2017 Jan 21]. https://www.eurachem.org/index.php/publications/guides/quam.
- European Commission. 2017. DG SANTE 2017, Food Contact Materials, List of Substances. [accessed 2017 Aug 01]. https://webgate.ec.europa.eu/foods_system/main/index.cfm
- Guo X, Xian Y, Luo H, Wu Y, Luo D, Chen Y, Lu Y, Xu D. 2013. Quantitative determinations of seven fluorescent whitening agents in polystyrene and polyvinyl chloride plastics by ultrahigh performance liquid chromatography-tandem mass spectrometry. Anal Meth. 5:6086–6093.
- Mathur AK, Khanna SK. 2002. Dermal toxicity due to industrial chemicals, skin pharmacol. Appl Skin Physiol. 15:147–153.
- Shu W-C, Ding W-H. 2005. Determination of fluorescent whitening agents in laundry detergents and surface waters by solid-phase extraction and ion-pair high-performance liquid chromatography, J. Chromatogr A. 1088:218–223.
- Wu Z, Xu Y, Li M, Guo X, Xian Y, Dong H. 2016a. Determination of three fluorescent whitening agents (FWAs) and their migration research in food contact plastic packaging containers and food simulants by UPLC-MS/MS method. RSC Advances. 6:17941–17946.
- Wu Z, Xu Y, Li M, Guo X, Xian Y, Dong H. 2016b. Simultaneous determination of fluorescent whitening agents (FWAs) and photoinitiators (PIs) in food packaging coated paper products by the UPLC-MS/MS method using ESI positive and negative switching modes. Anal Meth. 8:1052–1059.
- Yang Y, Xinghua W, Qiang F, Yong Y, Sizhu T, Kun W, Jia J, Daqian S, Aimin Y, Ziwei Z. 2017. On-site determination of the migration amount of fluorescent whitening agents from paper to finger by fluorescence spectrophotometry. Anal Meth. 9:465–472.