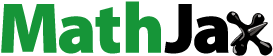
Abstract
Commercial animal feed in Texas was characterized by determining natural gamma emitters including 40K,137Cs, and Uranium (235U and 238U) and Thorium (232Th) series to obtain basic radioactivity values. The measured activity concentration of natural radionuclides in animal feed was low enough for safe consumption by animal and largely depended on the type of animal feed.40K was the predominant radionuclide showing the highest activity concentration in animal feed. The radioactivity concentration of 214 Bi and 214Pb in 238U decay series was 1.39 and 1.33 Bq/kg in corn, respectively, lower than in other animal feed types. On the other hand, the vitamin/mineral mix samples showed higher concentrations of 214 Bi (9.04 Bq/kg) and 214Pb (10.19 Bq/kg). Beef cattle feed, poultry feed, and vitamin/mineral mix exhibited higher activity concentration of 228Ac and 212Pb in 232Th decay series. Gamma radionuclides appeared to be highly and significantly correlated within each decay series. 235U was present at low levels in all feed samples while the anthropogenic radionuclide of 137Cs was not detected irrespective of the type of animal feed. This study highlights an importance of establishing a current baseline of radioactivity concentration in animal feed in Texas in which the largest animal feed consumption in the US exists.
Introduction
As a consequence of nuclear weapon testing, nuclear accidents, industrial use of radioactive compounds, and use of phosphate mineral fertilizers, a significant number of artificial radionuclides has been released into the environment which has led to serious environmental contamination (Mitrović et al. Citation2009; Haase et al. Citation2021). The primary anthropogenic radionuclides are 90Sr and 137CS. Such environmental contamination with artificial radioactive materials has served as a major route of radioactive contamination of agricultural products, animal feed, and human food (Mitrović et al. Citation2019). The degree of contamination derived from anthropogenic radionuclides is known to decrease as time goes on. Radioactivity also originates from natural sources, mainly cosmic rays and the radioactive content in soil and rocks. The most common and important natural sources are U (238U, 230Th, 226Ra, and 210Pb) and Th (232Th, 228Ra, and 228Th) series as well as naturally occurring 40K (Eisenbud Citation1995). The natural radioactivity can vary depending on geological and geographical factors. For example, the presence of minerals with high natural radioactivity is much greater in Brazil and India than in other parts of the world (Srinivasa et al. Citation2022; Lunardi and Bonotto Citation2023). As observed in anthropogenic radionuclides, natural radionuclides series have also been of great biological significance and affected all living beings (Linsalata Citation1994). Radioactive radiation from natural and artificial sources can be carcinogenic and cause certain types of health problems (e.g. leukaemia) in animals and human (Sahoo et al. Citation2011).
Radioactive elements and their decay products from soil, water, and air can be directly or indirectly transferred to plant-based feed and food products. In livestock production, the presence of radioactive substances may cause adverse effects and risks since radionuclides and their environmental contamination can persist for a very long period of time and move through the livestock production systems and markets to reach human food chains (Linsalata Citation1994; Mihaljev et al. Citation2019). The transfer of radionuclides from one phase to another in agriculture and feed production is the main reason why agricultural raw materials used for animal feed should be monitored for preventive radiation protection as they can be contaminated with natural and anthropogenic contamination by radionuclides (Mihaljev et al. Citation2019). The previous studies reported strong correlation between dietary intakes and concentration of radioactive elements in human tissues and bones (Cannas et al. Citation2020; Rizzoli et al. Citation2021; Salminen-Paatero and Paatero Citation2021; Shi et al. Citation2022). Consumption of radionuclide contaminated food and water is an important route for radionuclide to enter the human body (Desideri et al. Citation2014; Kim et al. Citation2018; Cannas et al. Citation2020; Boryło et al. Citation2022; Fan et al. Citation2023).
In most cases, radionuclides enter the food chain through the soil-plant-animal-human pathways. The radionuclides formed from nuclear weapon test series including 90Sr, 137Cs, Pu, and Am receive attention because they can still remain in the surface layer of soils and be transferred to crops and animal feed to enter the food chain (Frissel et al. Citation1986). Animals and their body tissues can be radioactively contaminated in several ways, perhaps most commonly through animal feed they consume or to a lesser extent drinking water and inhalation (Müller and Pröhl Citation1993; Carvalho et al. Citation2006; Casacuberta et al. Citation2009; Shanthi et al. Citation2010; Desideri et al. Citation2014; Angeleska et al. Citation2022). The previous studies documented that animal’s intake of natural series radionuclides and artificial radionuclides occurs through soils, forage, phosphate mineral feed additives, and feeds (Linsalata Citation1994; Voigt et al. Citation2007). Transfer of radioiodine, radiostrontium, and radiocesium present in animal feeds to milk, bone, eggs, and meat was also demonstrated in the previous works (Howard et al. Citation1997; Boradiya et al. Citation2018). Woodman and Nisbet (Citation1999) proposed practical working levels in animal feed and feeding water for some radionuclides based on information about animal diets, activity concentrations of feed, and feed-to-product transfer as well as the fact that only one or two feed types significantly contribute to intakes of the radionuclides. It has been known that radioactive pollutions from industrial development and human activities including mining and smelting activities, phosphate ore processing, and the application of fertilizers enhance concentrations of radionuclides in animal feeds (Mitrović et al. Citation2019). Therefore, it is necessary to monitor the radioactive contamination of animal feed to reduce the risk of human exposure to radioactive substances since they can easily deposit in humans through their typical pathways (Hernandez et al. Citation2004; Carvalho et al. Citation2006).
In the Federal Register, Food and Drug Administration (FDA) provides guidance to State and local agencies to assist planning emergency response and protective actions against accidentally contaminated human food and animal feeds and also presents information about radionuclides and guidance levels for radionuclide activity concentration in food through Compliance Policy Guide (GPC) (FDA Citation2004, Citation2020). In Europe, various regulations that are aimed to control radioactivity in food and animal feed and their ingredients from domestic production and imported products have been established to ensure the safety of foods from anthropogenic sources and also lower the overall human exposure to radiation from all relevant sources (EURATOM Citation1989; Mihaljev et al. Citation2019). The Joint FAO/WHO Codex Alimentarius Commission also developed guideline levels for radionuclides in foods that focused on following a nuclear or radiological emergency (Richards et al. Citation1996; Godefroy Citation2014) while the IAEA issued several international standards related to the use of radioactivity concentration in foods for radiological emergency preparedness (WHO Citation1996; Roberts Citation2016). Food Safety Modernization Act (FSMA) considers radiological hazards that need to be analysed at every step of food production when establishing food safety plans (FDA Citation2019; Tarnate Citation2019; Malik et al. Citation2021). Comprehensive monitoring and strict regulations of radioactivity from various dietary sources are essential to control and ensure the limits of the effective dose and human exposure in order to improve the consumer and food safety (Haase et al. Citation2021).
FDA and the European Commission have provided intervention limits for radionuclides in animal feeds following nuclear accidents and other radiological emergency situations (EURATOM Citation1989; FDA Citation2020). Besides, it has been known that the radiation levels in animal feed are very critical since animals may ingest contaminated animal feed and foods of animal origin constitute major human dietary components, consequently increasing the risk of animal and human health hazard (Angeleska et al. Citation2022). However, despite being of such importance of monitoring the radioactivity level in animal feed, there has been less paid attention to the control of animal feed and also a lack of information and studies about the natural radionuclide activity concentrations in commercial animal feeds in the US as well as in other parts of the world. More than 4000 firms located in Texas, the US and abroad distribute approximately 23 million tons of feed in Texas annually. The state also has a large animal population including approximately 900,000 dairy cows, 5 million cattle on feed, 12.6 million cows and calves, 21 million layers, 115 million broilers along with 12 million dogs and cats, 500,000 equids, and 1.5 million sheep and goats (TDA Citation2023).
A need exists to establish baseline levels of natural gamma emitters to facilitate monitoring of the Texas and US feed supply. Therefore, it would be worthwhile to investigate and assess the amount of natural radionuclides such as 238U, 235U, 232Th and their progenies, 40K, and the anthropogenic 137Cs, in commercial animal feeds produced in Texas and compare the values with those reported in the previous works performed in other countries and US. In this study, we determined natural gamma emitters in select types of animal feeds that were collected and analyzed for regulatory compliance. This study provides the baseline information on the activity concentration of some natural radionuclides and helps establish a roadmap for future research for commercial animal feeds.
Materials and methods
Samples
Animal feed samples were obtained from the Office of the Texas State Chemist (OTSC) regulatory samples collected according to the state’s annual plan of work (). A total of 69 samples of different animal feed types were selected, prepared, and analysed for the present study. The animal feed types studied were cattle feed, corn, pet food, poultry feed, swine feed, and vitamin/mineral mix. The mass of each feed sample was approximately in the range of 400-600 g.
Table 1. Feed usage and select chemical compositions (%) of animal feed samples collected for the state’s surveillance activities.Table Footnotea
Sample preparation
The collected samples were dried in a conventional dry oven at 105 °C for 24 hr. until the constant mass of the sample was achieved. The dried samples were divided into two test samples using a sample splitter (e.g. riffle) and the two of the sample was then taken from each of split sample. The four split samples were ground through a sample preparation equipment (e.g. grinder) with 0.75 mm diameter screen (Dai et al. Citation2016). The ground sample was then mixed for 30 min with a blender for homogenization and placed in 0.5 L Marinelli cup. The containers were sealed with electrical tape and stored for a period greater than 4 weeks to prevent radon escape and allow for particular radionuclides and their short-lived progenies to reach secular equilibrium before measurements (Alnour et al. Citation2012; Desideri et al. Citation2014; Ageda et al. Citation2017).
Gamma analysis
Gamma emitters 40K, 137Cs, 232Th decay products, 235U, and 238U decay products, were measured by gamma spectroscopy which determines simultaneously a variety of radionuclides with no or little requirement of sample pre-treatment. Particular radionuclides of interest such as 214 Bi, 214Pb, 228Ac, and 212Pb were quantified and compared to the results reported from the previous studies.
A gamma spectroscopy system consists of a high purity germanium (HPGe) detector (Canberra GC2518, Mirion Technologies, Inc., Meriden, CT) of type ‘p’ in coaxial geometry with Genie 2000 software. The detector used in measurement had a relative efficiency of 28% (determined by placing a source of 60Co at 25 cm from the detector) and connected to a 4096-channel computerized multi-channel analyser (MCA). The energy resolutions of FWHM and FWTM at 1.332 MeV of 60Co are 1.741 keV and 3.195 keV, respectively, which is sufficient to differentiate the gamma energies of interest in the present study. The detector was placed inside a lead shield with pre-amplifier to minimize background scattering and cooled by liquid nitrogen. A computer-based MCA that can transform pulses to gamma spectra was used for data acquisition and spectral analysis.
The energy calibration of the spectrometer was performed using certified reference materials including 8 gamma point sources: 241Am (59.5 keV), 109Cd (88.0 keV), 57Co (122.1 keV), 203Hg (279.2 keV), 113Sn (391.7 keV), 137Cs (661.7 keV), 88Y (898.0 and 1836.1 keV), and 60Co (1173 and 1333 keV). The activity of 40K was determined by measuring a single 1460.6 keV gamma rays emitted during its decay. The activity concentration of 214 Bi (from gamma ray peaks of 609.3, 1120.2 and 1764.5 keV) and 214Pb (from gamma ray peaks of 295.2 and 351.9 keV) was used to assess an estimate of 238U (226Ra) in animal feed. Likewise, the activity concentration of 232Th was determined from 911.2, 964.6, and 969.0 keV gamma-ray peaks of 228Ac and 238.6 keV gamma-ray peak of 212Pb. The activities of 235U and 137Cs were obtained from the 185.7 keV and 661.66 keV gamma ray peaks, respectively. The background spectrum of the gamma spectroscopy system was acquired on a weekly basis. The geometry configuration remained the same throughout the analysis.
Calculation of activity concentrations
The gamma background level was measured under the same conditions in which the activity of samples was determined using deionized-water filled Marinelli beaker. Specific activities for each gamma ray peak were estimated by subtracting counts due to Compton scattering and other background sources from the total peak area using the following equation:
where A is the activity concentration of radionuclide; S, the net peak area; M, the mass of the sample measured; ε, the attenuation corrected efficiency; Pγ, the gamma yield or the probability of decay for the specific gamma; T, the sample counting time; Kc, decay correction factor during counting, and Kw, the decay correction factor between the sample collection and measurement. The decay correction factors were calculated using
where T is a counting time for Kc or time interval between the sample collection and measurement for Kw and t1/2 is the half-life of radionuclide.
The Minimum Detectable Activity (MDA) were also determined by applying the same equation for the calculation of the activity concentration. However, the peak net account was obtained from a background spectrum. The uncertainty of the sample concentration was calculated by propagating the uncertainty values estimated for concentration and net counts of collected samples.
Statistical analyses
The measured values are grouped according to the type of animal feed and radionuclide. The arithmetical mean and standard deviation were calculated and analysed using Microsoft Excel® by animal feed type and radionuclide. Pearson’s simple correlation coefficients were used to determine statistical correlations between radionuclides using SAS 9.4 (SAS Inc, Cary, NC). The statistical comparisons between animal feeds within each radionuclide were also performed by analysis of variance based on SAS Proc GLM procedure. The mean difference among animal feed types was also examined using least significant differences (LSD) at p < 0.05.
Results and discussion
The radioactive content of the different types of animal feeds measured by a standard gamma spectroscopy system, with a high-resolution HPGe detector showed a variety of activity concentration of natural radionuclides (). In the present work, radionuclides that 226Ra and its progenies in the Uranium series and 228Ra and its progenies in the Thorium series were mainly observed along with 40K while detectable anthropogenic radionuclides were not present in any feed samples.
Table 2. Activity concentration (Bq/kg) of 40K, 214 Bi, and 214Pb in the different types of commercial animal feeds in Texas.
The mean activity concentration of select natural radionuclides in all feed samples measured for the study was 257.23 ± 103.98 Bq/kg for 40K, 3.01 ± 3.89 Bq/kg for 214 Bi, 3.11 ± 4.36 Bq/kg for 214Pb, 3.61 ± 1.52 Bq/kg for 228AC, 2.53 ± 3.62 Bq/kg for 212Pb, and 4.21 ± 9.21 Bq/kg for 235U ( and ). The range and mean of measured activities of natural radionuclides in feed samples appear largely dependent on the type of animal feed. The measured activity concentrations of natural gamma radionuclides are unlikely harmful and within the recommended values (UNSCEAR Citation2000) indicating no significant impact on animal health risks. Commercial animal feeds produced in Texas revealed equivalent activities of select radionuclides depending on the type of animal feed compared to those reported in other countries.
Table 3. Activity concentration (Bq/kg) of 228AC, 212Pb, and 235U in the different types of commercial animal feeds in Texas.
40K activity
The activity concentration of 40K was highest in all types of investigated feed samples including beef cattle feeds, corn, pet foods, poultry feeds, swine feed, and vitamin/mineral mixes collected in Texas while other radionuclides occurred at low activity concentration and appear to be within the nominal concentrations in animal feed samples. 40K decaying by emission of beta particles and gamma photons is a naturally occurring and dominant radionuclide with a global average activity of 400 Bq/kg in soil while the natural radioactivity in food commodities is mostly in a range of 40 to 600 Bq/kg (UNSCEAR Citation2000; IAEA Citation2002; Mitrović et al. Citation2019). In addition, potassium is an essential element for human and animal so the presence and radioactivity of 40K cannot be avoidable.
While 40K showed the highest activity concentration in each type of animal feed, some radioisotopes in vitamin/mineral mix had a larger contribution to the sample radioactivity. However, the high activity concentration of 40K does not imply that human and animals may be at a risk of health problems caused by the radioisotope. The obtained results showed that the mean activity concentration of the 40K was highest in beef cattle (334.15 ± 54.25 Bq/kg) while the lowest activity concentration of 40K was observed in corn products (131.65 ± 70.73 Bq/kg). The average activity of vitamin/mineral mix was lower than that of beef cattle, but some mineral mix samples displayed either much higher or much lower 40K activity than beef cattle samples, consequently resulting in high standard deviation (). 40K activity in mineral/vitamin mix appears to be in proportion to the level of potassium in samples. The mean 40K activity concentration of the other feed samples were slightly lower than vitamin/mineral mix and ranged from 255.75 to 261.28 Bq/kg. Potassium used for vitamin/mineral mix is in general obtained from the mining of underground ore deposits and salty lakes, mainly in US, Canada, Chile, and German (Ciceri et al. Citation2015) although the mined and manufactured potassium salts (potash) are essentially used to manufacture a variety of fertilizers for crop production (Manning Citation2010). Therefore, the high activity of 40K found in the test samples may be considered to be fundamentally associated with individual ingredients used in the manufacture of commercial animal feed, particularly those contaminated with fertilizer at harvest (Angeleska et al. Citation2022).
238U radioactive series
Uranium is likely present in animal feed as a result of its ubiquitous occurrence in soil and fertilizers. The complex radioactive decay processes of the element generate various minerals producing radioactive radiation. The radioactive decay process of 238U includes 14 steps to reach the final stable decay product 206Pb. Among its decay products, inert radon gas (222Rn) with a half-life of 3.8 days proceeds the formation of 214 Bi and 214Pb which represent the major source of gamma irradiation associated with 238U (Alnour et al. Citation2012). Since 238U is a naturally occurring radionuclide and its level is closely related to the type of rock, natural environmental radiation, and radioactivity in soil and plant are mainly determined by geological and geographical features (Tzortzis and Tsertos Citation2004; Mitchell et al. Citation2013). However, radioactive equilibrium between natural 226Ra and 238U in biological samples should not be expected due to their difference in mobility (Carvalho et al. Citation2006; Luz Filho et al. Citation2016).
The activity of 226Ra in the 238U series was estimated based on the measurements of 214 Bi and 214Pb in its decay chain although the secular equilibrium between 226Ra and 214 Bi and 214Pb cannot be assumed (Desideri et al. Citation2014). The range (mean) of 214 Bi activity concentration in samples was 1.39-3.21 Bq/kg (2.21 Bq/kg) for cattle feed, 1.22-1.66 Bq/kg (1.39 Bq/kg) for corn, 1.37-1.95 Bq/kg (1.64 Bq/kg) for pet food, 1.29-2.52 Bq/kg (1.70 Bq/kg) for poultry feed, 1.34-1.74 Bq/kg (1.53 Bq/kg) for swine feed, and 2.23-19.38 Bq/kg (9.04 Bq/kg) for vitamin/mineral mix. As a member of the same decay series in secular equilibrium condition, the mean activity concentration of 214 Bi was lowest in corn samples and significantly higher in vitamin/mineral mix. The range and mean values of 214Pb were almost equal to those of 214 Bi with the lowest concentration in corn and highest concentration in vitamin/mineral mix (). These results are intuitive since corn is grown above ground and would reflect naturally occurring contamination in the ground while minerals are mined from below ground.
Previous studies showed radium intake and human bone radium concentrations are strongly correlated with total dietary Ca intake (Linsalata Citation1994). A similar correlation between two elements would be expected in this study because calcium and phosphorus contents in animal feed samples appeared to be proportional to each other () and in general originate from the same nutritional supplement made of phosphorus ore minerals. In the present study, the activity concentration of 226Ra measured at 186.2 keV peak ranged from 13.26 to 21.31 Bq/kg (data not shown) which are substantially higher than those of 214 Bi and 214Pb. The activity concentrations of 226Ra at that peak appeared to be much higher than the concentration of natural activity of the element in foods and animal feed. Such a difference may be attributed to a significant interference of the 185.7 keV 235U emission on the 186.2 keV 226Ra emission if it’s assumed that the sample was completely sealed in air-proof container and 226Ra was in secular equilibrium with its subsequent decay products (Ebaid et al. Citation2005; Mitrović et al. Citation2019).
As expected, ANOVA test results for 214 Bi and 214Pb data showed statistical significant differences in the radioactivity concentration between vitamin/mineral mix and the other animal feeds (p < 0.05). The radioactivity concentration of 214 Bi and 214Pb was relatively higher in beef cattle and poultry feeds as well. The increased activities of 226Ra decay products in those animal feed types can be explained by the inclusion of end products of processing phosphorus ores that are commonly used as a nutritional supplement for animal feed to increase calcium and phosphate content. Thus, animal feed can be contaminated by uranium since the phosphate rocks may contain a high level of 238U and its decay products (Arruda-Neto et al. Citation1997; Casacuberta et al. Citation2007). Although the radioactive concentration of the 226Ra decay products were higher in poultry and beef cattle feeds, the estimates are lower or slightly higher than those reported in other countries (Desideri et al. Citation2014; Luz Filho et al. Citation2016; Ageda et al. Citation2017; Angeleska et al. Citation2022). The studies in which 186.2 keV energy peak was assigned for estimating the concentration of 226Ra reported particularly higher concentration of 226Ra than those of other studies presumably due to the interference of 235U on 226Ra concentration (Ageda et al. Citation2017; Angeleska et al. Citation2022). It would be also noteworthy that vitamin/mineral mix may be rich in 210Po and its precursor 210Pb that belong to the 238U series, but could not be determined by gamma spectrometer in the present study. The two radionuclides are of great interest because their presence in animal feed should be ubiquitous and their significant contribution of the effective dose to human and animal from injection would be much greater than 238U and its decay products (Linsalata Citation1994; Desideri et al. Citation2014).
232Th radioactive series
232Th is a metallic naturally occurring radionuclide and mainly found in the earth’s crust and also biosphere in very small amount due to its insolubility. Similar to 238U, 232Th undergoes complex radioactive decay processes before reaching to the final stable decay product of 208Pb. 232Th is approximately three times more abundant than 238U in rocks and soils. The gamma rays of 232Th typically originates from the decay products including 228Ac, 212Pb, and 208Tl and largely depend on geological and geographical conditions (Linsalata Citation1994; Tzortzis and Tsertos Citation2004).
232Th and 228Ra could be indirectly measured by the short-lived and predominant isotopes such as 228Ac and 212Pb. The activity concentration of 228Ac was in the ranges of 3.24-5.39 Bq/kg with a mean of 4.21 Bq/kg for beef cattle, 2.43-3.70 Bq/kg with a mean of 2.74 Bq/kg for corn, 2.53-3.92 Bq/kg with a mean of 3.30 Bq/kg for pet food, 2.37-3.90 Bq/kg with a mean of 3.19 Bq/kg for poultry feed, and 2.78-3.70 Bq/kg with a mean of 3.11 Bq/kg for swine feed, and 2.11-11.48 Bq/kg with a mean of 4.64 Bq/kg for vitamin/mineral mix. The activity values of 212Pb were on the whole slightly lower than those of 228Ac in all animal feed types except for vitamin/mineral mix showing equivalent or higher activity of 212Pb than 228Ac. The activities of 228Ac and 212Pb was relatively greater in beef cattle and poultry feeds than the other animal feeds (p > 0.05) while vitamin/mineral mix exhibited significantly higher activities than all other animal feeds (p < 0.05). The higher concentration of 228Ac and 212Pb activity for cattle feeds was also reported in some previous works (Casacuberta et al. Citation2009; Luz Filho et al. Citation2016). A nutritional supplement obtained from phosphate rocks is known as a main phosphate source commonly used for animal feeds to raise cattle (Arruda-Neto et al. Citation1997). A significantly higher activity of 232Th series was found in vitamin/mineral mix as observed in the Uranium series. Since the vitamin/mineral mix is a major source of calcium and phosphorus and obtained by processing phosphorus ore, this animal feed may contain large amounts of both Uranium and Thorium decay products. The activities of the Thorium series in animal feed were in general lower than those of the Uranium series, particularly in vitamin/mineral mix. However, the difference between the two series was not statistically significant among animal feed types (p > 0.05).
The range of the obtained concentrations of 232Th in the present study is much lower than the global average activity of 232Th cited in UNSCEAR (Citation2000). In fact, the concentrations of samples showed equivalent values to those reported in some previous works (Desideri et al. Citation2014; Luz Filho et al. Citation2016) while they are much lower than those determined in the other studies (Ageda et al. Citation2017; Angeleska et al. Citation2022) in the same type of animal feed. The difference in activity in similar animal feed produced in different locations may be mainly attributed to the level of phosphate mineral feed additives added to that type of animal feed (Angeleska et al. Citation2022; Luz Filho et al. Citation2016; Saleh Citation2012).
Simple correlation coefficient and their significance between select gamma radionuclides are shown in . As expected under secular equilibrium conditions, a higher correlation occurred within 238U decay products, between 214 Bi and 214Pb irrespective of animal feed type. Likewise, 228Ac was significantly correlated with another Thorium decay product of 212Pb with moderate or high correlation coefficients in select animal feed types. A significant linear correlation was also observed between Uranium and Thorium series depending on animal feed type and radionuclide. This can be attributed to the level and alteration of the natural composition of the radioactivity in animal feed. It is also known that fertilizer containing phosphate with high level of Uranium and Thorium series can concentrate and redistribute radionuclides in soil and agricultural products (Mortvedt Citation1994; Desideri et al. Citation2014; Mihaljev et al. Citation2019).
Table 4. Simple correlation coefficient between select gamma radionuclides detected in animal feed.Table Footnotea
235U and 137Cs activities
235U is a naturally occurring radionuclide that has always been present with 238U and 232Th on the Earth and known as fissile isotope yielding large amounts of energy. In this study, this radioactive element is present in all animal feed types and their measured concentrations were lower than those of radionuclides in 238U and 232Th series (). The mean activity concentration of 235U was less than 1 Bq/kg in corn and pet food samples while cattle feed, poultry feed, and swine feed samples showed a slightly higher activity, which was, however, low and concentrations below 1.5 Bq/kg. As a result, a relatively low concentration of 235U appeared to result in insignificant exposures to animals.
Compared to other animal feed types, significantly higher activities of 235U were found in vitamin/mineral mix whose concentrations ranged from 4.81 to 61.1 Bq/kg with a mean of 18.85 ± 15.53 Bq/kg. As discussed earlier, such high activity of the element can be explained by high contents of uranium included in this feed supplement, which contains a high portion of the phosphate-based products obtained from the phosphate ore processing. The measured activities of 235U in this study showed a slightly higher values than those reported in the previous works (Linsalata Citation1994; Saleh Citation2012; Mihaljev et al. Citation2019). This may be attributed to the fact that the primary 235U emission at 185.7 keV is somewhat interfered with 226Ra emission at 186.2 keV (Ebaid et al. Citation2005). Nevertheless, the results seem to imply that this naturally radioactive element occurring through consumption of phosphate-based mineral feed supplement needs to be monitored to assess the potential radiological risk to animals and humans.
Apart from naturally occurring radionuclides such as 235U, 238U, and 232Th, the contamination of the anthropogenic radionuclide 137C is observed in environment due to the deposition of 137C after nuclear disaster including the Chernobyl accident and the Fukushima nuclear disaster. The activity concentration of 137Cs decreases in soils and is rarely found in animal feed and human food as time passes. However, this long-lived radionuclide is still present in the environment as well as feed raw materials (Mitrović et al. Citation2019; Haase et al. Citation2021).
In all animal feed samples, the anthropogenic radionuclide of 137Cs was not detected in the present study. The measured activity concentration was below the detection limits approximately 0.25 Bq/kg in most of animal feed types while vitamin mineral mix showed a slightly higher background radiation level. A high background activity of 137Cs can be detected in animal feeds due to the contamination of clay minerals and organic matters that may affect retention and migration of the radionuclide in the environment (Staunton et al. Citation2002; El-Reefy et al. Citation2006). US and European Community provide maximum permitted levels of radiocesium in commercial animal feeds (EURATOM Citation1989; FDA Citation2004, Citation2020). However, these levels appeared to need to further take account of variations in animal feed type, animal metabolism, and season effects to apply for various feedstuffs (Woodman and Nisbet Citation1999).
Conclusions
The study results provided basic and useful information on the activity concentration of radioactive elements present in animal feed products commercialized in Texas where a significant amount of animal feed and animal origin products were produced and distributed. Although radioactive elements present in commercial animal feeds produced in Texas are low enough to be negligible and within the limits of permitted values, it is important to note that the radioactive contamination of animal tissues originates from the radioactive residues in animal feed and can last for an extended period. This study provides a baseline upon which potential radiochemical contamination can be monitored if an anthropogenic event occurs.
Comprehensive preventive measures that were developed and implemented by some countries and international organizations may serve as a basis for radioactivity monitoring and control program in producing animal feed products. The main purpose of the preventive measures should be to lower the radioactivity of the animal feed to reduce the transfer of radioactive isotopes to animal. Presently, the FDA Food Safety Modernization Act’s Preventive Controls for Animal Food regulatory guidance does not consider radiochemical contamination as a hazard that requires a preventive control. This study supports that conclusion.
Acknowledgements
The authors are thankful to staffs and administrations of the Office of the Texas State Chemist, Texas A&M AgriLife Research for their support and financial assistance offered for this research.
Disclosure statement
No potential conflict of interest was reported by the author(s).
References
- Ageda VI, Ike EE, Temaugee ST. 2017. Assessment of natural radionuclide levels in some Nigerian made poultry feedstuff. Int J Phys Sci. 12(19):243–246. doi:10.5897/IJPS2016.4594.
- Alnour IA, Ibrahim N, Hossain I. 2012. Concentrations of 214 Pb, 214 Bi in 238 U series and 208 Tl, 228 Ac in 232 Th series in granite rock in (Kadugli) Sudan.
- Angeleska A, Crceva Nikolovska R, Dimitrieska Stojkovik E, Blagoevska K, Dimzoska Stojanovska B, Uzunov R, Angelovski L. 2022. Activity concentration of natural radionuclides in chicken feeds in the Republic of North Macedonia. Krmiva (Online)). 63(1):19–23. doi:10.33128/k.63.1.3.
- Arruda-Neto JDT, Tavares MV, Filadelfo M. 1997. Concentrations of uranium in animal feed supplements: measurements and dose estimates. J Radioanal Nucl Chem. 221(1–2):97–104. doi:10.1007/BF02035249.
- Boradiya PC, Savsani HH, Odedra MD, Patil SS, Gohil BC, Trivedi SP, Patel JA. 2018. Animal feed safety: an overview. Int J Recent Res Interdiscip Sci. 5(2):1–4.
- Boryło A, Skwarzec B, Wieczorek J. 2022. Sources of Polonium 210Po and radio-lead 210Pb in human body in Poland. IJERPH. 19(4):1984. doi:10.3390/ijerph19041984.
- Cannas D, Loi E, Serra M, Firinu D, Valera P, Zavattari P. 2020. Relevance of essential trace elements in nutrition and drinking water for human health and autoimmune disease risk. Nutrients. 12(7):2074. doi:10.3390/nu12072074.
- Carvalho C, Anjos RM, Mosquera B, Macario K, Veiga R. 2006. Radiocesium contamination behavior and its effect on potassium absorption in tropical or subtropical plants. J Environ Radioact. 86(2):241–250. doi:10.1016/j.jenvrad.2005.09.003.
- Casacuberta N, Masqué P, Garcia-Orellana J, Bruach JM, Anguita M, Gasa J, Villa M, Hurtado S, Garcia-Tenorio R. 2009. Radioactivity contents in dicalcium phosphate and the potential radiological risk to human populations. J Hazard Mater. 170(2–3):814–823. doi:10.1016/j.jhazmat.2009.05.037.
- Casacuberta N, Masque P, Garcia-Orellana J, Bruach-Menché JM, Sanchez-Cabeza JA, Villa M, Hurtado S, Manjón G, Garcia-Tenorio R. 2007 Mar 19–22. Dicalcium phosphate: contents of uranium decay series. In: NORM V, Seville, Spain.
- Ciceri D, Manning DA, Allanore A. 2015. Historical and technical developments of potassium resources. Sci Total Environ. 502:590–601. doi:10.1016/j.scitotenv.2014.09.013.
- Dai SY, Jones B, Lee KM, Li W, Post L, Herrman TJ. 2016. Heavy metal contamination of animal feed in Texas. J Regul Sci. 4(1):21–32. doi:10.21423/JRS-V04N01P021.
- Desideri D, Roselli C, Forini N, Rongoni A, Meli MA, Feduzi L. 2014. Alpha and gamma spectrometry for the radiological characterization of animal feed. Microchem J. 116:41–46. doi:10.1016/j.microc.2014.03.009.
- Ebaid YY, El-Mongy SA, Allam KA. 2005 Feb. 235U–γ emission contribution to the 186 keV energy transition of 226Ra in environmental samples activity calculations. Int Congr Ser. 1276:409–411. doi:10.1016/j.ics.2004.12.020.
- Eisenbud M. 1995. Radioactivity from natural, industrial, and other sources. J Radioanal Nucl Chem Art. 197(1):15–27. doi:10.1007/BF02040217.
- El-Reefy HI, Sharshar T, Zaghloul R, Badran HM. 2006. Distribution of gamma-ray emitting radionuclides in the environment of Burullus Lake: I. Soils and vegetations. J Environ Radioact. 87(2):148–169. doi:10.1016/j.jenvrad.2005.11.006.
- EURATOM. 1989. Council Regulation (Euratom) No 2218/89 of 18 July 1989 amending Regulation (Euratom) No3954/87 laying down maximum permitted levels of radioactive contamination of food stuffs and of feeding stuffs following a nuclear accident or any other case of radiological emergency. Off J Eur Communties Law. 211.
- Fan Y, Pan D, Yang M, Wang X. 2023. Radiolabelling and in vivo radionuclide imaging tracking of emerging pollutants in environmental toxicology: a review. Sci Total Environ. 866:161412. doi:10.1016/j.scitotenv.2023.161412.
- [FDA] Food and Drug Administration. 2004. Supporting document for guidance levels for radionuclides in domestic and imported foods. Docket No. 2003D-0558. Silver Spring (MD).
- [FDA] Food and Drug Administration. 2019. Food Safety Modernization Act.
- [FDA] Food and Drug Administration. 2020. Guidance levels for radionuclides in domestic and imported foods. Compliance Policy Guide Sec. 555.880. Silver Spring (MD).
- Frissel MJ, van Ginkel JH, Stoutjesdijk JF, Koester HW. 1986. The transfer of radionuclides from soil to animal feed. (No. INIS-XA-C–069).
- Godefroy SB. 2014. Codex Alimentarius Commission at 50: major achievements and challenges ahead. Int Food Risk Anal J. 4:1. doi:10.5772/58862.
- Haase G, Vagt T, Fritsche J. 2021. Monitoring environmental radionuclide activity of the incident-relevant radionuclides 137 Cs und 90 Sr in animal feed and foodstuffs consumed in Germany. J Consum Prot Food Saf. 16(1):97–101. 2021) doi:10.1007/s00003-020-01309-7.
- Hernandez F, Madrid J, Garcia V, Orengo J, Megias MD. 2004. Influence of two plant extracts on broilers performance, digestibility, and digestive organ size. Poult Sci. 83(2):169–174. doi:10.1093/ps/83.2.169.
- Howard BJ, Beresford NA, Mayes RW, Hansen HS, Crout NMJ, Hove K. 1997. The use of dietary calcium intake of dairy ruminants to predict the transfer coefficient of radiostrontium to milk. Radiat Environ Biophys. 36(1):39–43. doi:10.1007/s004110050053.
- [IAEA] International Atomic Energy Agency. 2002. Nat Induc Radioact in Food. IAEA-TECDOC-1287.
- Kim S, Min BI, Park K, Yang BM, Kim J, Suh KS. 2018. Evaluation of radionuclide concentration in agricultural food produced in Fukushima prefecture following Fukushima accident using a terrestrial food chain model. J Radioanal Nucl Chem. 316(3):1091–1098. doi:10.1007/s10967-018-5779-3.
- Linsalata P. 1994. Uranium and thorium decay series radionuclides in human and animal foodchains—a review. J Environ Qual. 23(4):633–642. doi:10.2134/jeq1994.00472425002300040003x.
- Lunardi M, Bonotto DM. 2023. Natural radioactivity due to uranium and radon in thermal groundwaters of Central Brazil. J Radioanal Nucl Chem. 332(3):629–646. doi:10.1007/s10967-023-08784-w.
- gt;Luz Filho IVD, Scheibel V, Appoloni, CR. 2016. 40K, 226Ra and 228Ra series in bovine and poultry feed and in dicalcium phosphate (DCP) samples by gamma-ray spectrometry. Braz Arch Biol Technol. 59(2016):1–9.
- Malik S, Krishnaswamy K, Mustapha A. 2021. Hazard analysis and risk-based preventive controls (HARPC): current food safety and quality standards for complementary foods. Foods. 10(9):2199. doi:10.3390/foods10092199.
- Manning DA. 2010. Mineral sources of potassium for plant nutrition. A review. Agron Sustain Dev. 30(2):281–294. doi:10.1051/agro/2009023.
- Mihaljev Ž, Popov N, Kartalović B, Jakšić S, Živkov-Baloš M. 2019. Activity of natural radionuclides in animal feed. In: Ecological Movement of Novi Sad (Serbia).
- Mitchell N, Pérez-Sánchez D, Thorne MC. 2013. A review of the behaviour of U-238 series radionuclides in soils and plants. J Radiol Prot. 33(2):R17–R48. doi:10.1088/0952-4746/33/2/R17.
- Mitrović B, Vitorović G, Vitorović D, Pantelić G, Adamović I. 2009. Natural and anthropogenic radioactivity in the environment of mountain region of Serbia. J Environ Monit. 11(2):383–388. doi:10.1039/b813102c.
- Mitrović BM, Vranješ B, Kostić O, Perović V, Mitrović M, Pavlović P. 2019. Presence of radionuclides and toxic elements in feedstuffs and food of animal origin. Veterinarski Glasnik. 73(1):30–39.
- Mortvedt JJ. 1994. Plant and soil relationships of uranium and thorium decay series radionuclides—a review. J Environ Qual. 23(4):643–650. doi:10.2134/jeq1994.00472425002300040004x.
- Müller H, Pröhl G. 1993. ECOSYS-87: a dynamic model for assessing radiological consequences of nuclear accidents. Health Phys. 64(3):232–252.
- Richards JI, Hance RJ, Crick MJ. 1996. Standards and criteria established by international organisations for agricultural aspects of radiological emergency situations. In: Proceedings of the NEA workshop on The Agricultural Issues Associated with Nuclear Emergencies, June 1995, OECD/NEA, Paris.
- Rizzoli R, Biver E, Brennan-Speranza TC. 2021. Nutritional intake and bone health. Lancet Diabetes Endocrinol. 9(9):606–621. doi:10.1016/S2213-8587(21)00119-4.
- Roberts PB. 2016. Food irradiation: standards, regulations and world-wide trade. Radiat Phys Chem. 129:30–34. doi:10.1016/j.radphyschem.2016.06.005.
- Sahoo SK, Hosoda M, Kamagata S, Sorimachi A, Ishikawa T, Tokonami S, Uchida S. 2011. Thorium, uranium and rare earth elements concentration in weathered Japanese soil samples. Prog Nucl Energy Tech. 1(0):416–419. doi:10.15669/pnst.1.416.
- Saleh, IH. 2012. Radioactivity of 238U, 232Th, 40K, and 137Cs and assessment of depleted uranium in soil of the Musandam Peninsula, Sultanate of Oman. Turkish J Eng Environ Sci. 36(3):236–248.
- Salminen-Paatero S, Paatero J. 2021. Transfer of natural radionuclides in terrestrial food chains—a review of investigations in Finland. IJERPH. 18(20):10577. doi:10.3390/ijerph182010577.
- Shanthi G, Kumaran JTT, Raj GAG, Maniyan CG. 2010. Natural radionuclides in the South Indian foods and their annual dose. Nucl Instrum Methods Phys Res A. 619(1–3):436–440. doi:10.1016/j.nima.2009.10.068.
- Shi Z, Yong L, Liu Z, Wang Y, Sui H, Mao W, Zhang L, Li Y, Liu J, Wei S, et al. 2022. Risk assessment of rare earth elements in fruits and vegetables from mining areas in China. Environ Sci Pollut Res Int. 29(32):48694–48703. doi:10.1007/s11356-022-19080-7.
- Srinivasa E, Rangaswamy DR, Suresh S, Sannappa J. 2022. Natural radioactivity levels and associated radiation hazards in soil samples of Chikkamagaluru district, Karnataka, India. J Radioanal Nucl Chem. 331(4):1899–1906. doi:10.1007/s10967-021-08133-9.
- Staunton S, Dumat C, Zsolnay A. 2002. Possible role of organic matter in radiocaesium adsorption in soils. J Environ Radioact. 58(2–3):163–173. doi:10.1016/s0265-931x(01)00064-9.
- Tarnate JB. 2019. Food safety culture and technology enhance food safety modernization act.
- [TDA] Texas Department of Agriculture. 2023. Texas Livestock. Available from: https://www.texasagriculture.gov/Grants-Services/Marketing-and-International-Trade/International/Buyers-and-Consumers/Texas-Livestock.
- Tzortzis M, Tsertos H. 2004. Determination of thorium, uranium and potassium elemental concentrations in surface soils in Cyprus. J Environ Radioact. 77(3):325–338. doi:10.1016/j.jenvrad.2004.03.014.
- [UNSCEAR] United Nations Scientific Committee on the Effect of Atomic Radiation. 2000. Report to the General Assembly, with scientific annexes. Sources and effects of ionizing radiation. New York (NY): United Nations.
- Voigt G, Howard BJ, Beresford NA. 2007. Transfer of radionuclides in animal production systems. Radiotact Environ. 10:71–96.
- Woodman RFM, Nisbet AF. 1999. Derivation of working levels for radionuclides in animal feedstuffs for use following a nuclear accident. Health Phys. 77(4):383–391. doi:10.1097/00004032-199910000-00005.
- World Health Organization. 1996. International basic safety standards for protecting against ionizing radiation and for the safety of radiation sources, Safety Series No. 115. Vienna: IAEA.