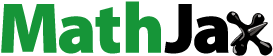
Abstract
Bimsoils are a loose rock and soil system, and the occurrence of geological hazards is closely related to water. To investigate the permeability and seepage characteristics of bimsoils, factors on permeability are discussed in detail considering cementation state, and variable mass seepage is studied tentatively with self-developed apparatus. Results shown that the order of factors on permeability is rock content > cementation degree > rock size > Talbot index (describing the mass percentage for different particle size of sand), and there are significant differences between factors. Besides, permeability generally increases with the increase of rock content and decreases with the increase of cementing agent content, while increases slightly due to agglomeration effect at the clay content of 8%. It is more obvious for reducing the permeability of higher rock content bimsoils by strong cementing agent, however, it tends to be same eventually with cement content increasing. The seepage of bimsoils is dominated by fine particle losses, and the secondary inrush occurs under the larger particle radius ratio. Moreover, particle losses and the time needed for secondary inrush both increase with the increase of particle radius ratio. The results would provide consults for the preparation of similar materials and the prevention of tunnels and underground engineering disasters.
1. Introduction
The geological body composed of gravel or block stone as aggregate and clay and sand as filler material is defined as bimsoils, which is widely distributed in nature (Xu et al. Citation2008; Belkhatir et al. Citation2013; Park and Santamarina Citation2017). Meanwhile, projects such as tunnels and underground engineering, slopes, hydropower dams and road foundations are inevitably built on engineering geological bodies of bimsoils, and these areas usually are extremely water-rich environment (Huang et al. Citation2016; Justo et al. Citation2019; Liu et al. Citation2020). Therefore, the research on the stability of bimsoils is an important part to analyze the geological disasters of bimsoils as well as the safe construction and operation of engineering (Khorasani et al. Citation2019).
Bimsoils are a special coupling porous medium between soil and rock mass, but its physical and mechanical properties are more complex than those of the traditional soil mechanics or rock mechanics (Wei et al. Citation2018a; Xu and Zhang Citation2021). As it is a heterogeneous loose soil-rock medium system composed of rocks with a certain engineering scale, high strength, fine-grained soil and pores, the bimsoils generally have the marked characteristics of looseness, unevenness and water-rich condition. Thus, the hydraulic characteristics of bimsoils are obviously different from those of homogeneous geo-materials. In addition, bimsoils of different regions and origins have different morphological and structural characteristics, which will ultimately result in different permeability and seepage characteristics (Kalender et al. Citation2014; Zhou et al. Citation2019; Qian et al. Citation2020; Wang et al. Citation2021b, Citation2021c). Hence, it is of great theoretical and practical significance to study the permeability and variable mass seepage characteristics of bimsoils under water-rich conditions.
Permeability and strength are the important parameters for studying the physical and mechanical properties and stability of bimsoils. Those two parameters are closely related to particle size, grain composition, particle shape, cementation state and as well as pore characteristics (Zhang et al. Citation2021). According to the strength characteristics of bimsoils, a large number of relative systematic studies have been carried out from the perspective of influencing factors, soil–rock interface effects and so on. Meanwhile, with the help of transparent soil technology, a series of beneficial results have been obtained (Ding et al. Citation2018; Wei et al. Citation2018b; Hu et al. Citation2021). However, limited by the difficulty of sampling, the development of test equipment, the errors of field tests and the size effect, there are few research report on the permeability and seepage characteristics of bimsoils, thus, laboratory test and numerical calculation are the main research methods at present (Napoli et al. Citation2018; Chen et al. Citation2019; Xue et al. Citation2020).
Due to the discontinuity of the composition structure of bimsoils, there are some potential cracks between the fine and coarse particles, which are more prone to failure and instability under the action of water (Wu et al. Citation2020). lists the research methods and research factors on the permeability of bimsoils in previous studies, among which the research factors are mainly focus on rock content, rock particle size and rock particle size distribution. Besides, in terms of seepage inflow erosion, Feng et al. (Citation2018) adopted custom-made seepage apparatus and nuclear magnetic resonance technology to study the seepage failure process of bimsoils under different hydraulic gradients. Ma et al. (Citation2017) developed a new rock testing system to analyze the migration process of fine particles with gravel filling in the fault during water inrush and its influence on the porosity of the sample. Wang et al. (Citation2020) conducted laboratory experiments with a self-developed apparatus and obtained the law of particle migration process and the characteristics of particle gradation on the migration of bimsoils during water inrush.
Table 1. Summary of research methods and factors of the permeability of bimsoils in latest literatures.
In practical engineering, there are many factors affecting the permeability of bimsoils, among which the cementation state is an important parameter (Lin et al. Citation2019; Ren and Zhao Citation2021). The previous research employed compaction number to ensure the relative density of specimens and study on the influence of compaction characteristics to maximum dry density and optimum water content (Wang et al. Citation2019a; Spagnoli and Shimobe Citation2020). However, the impact of cementation degree on the permeability of bimsoils is rarely taken into consideration, especially at the condition of water infiltration. In addition, the lack of targeted test equipment is also a pity to study the seepage erosion of bimsoils.
Therefore, this study mainly focuses on the permeability and variable mass seepage of bimsoils. With the consideration of the cementation sate of bimsoils, the influencing factors on the permeability of bimsoils including Talbot index, rock content, rock size and cementation degree are analyzed by orthogonal experiments. Moreover, the interaction between rock content and cementation degree which have greater influence on permeability is studied in detail with interactive tests. Last but not least, the self-developed apparatus is applied to explore the variable mass seepage characteristics of the bimsoils. The migration behavior of particles and water under the condition of seepage has obtained, and the impact of particle size on water inrush and mass loss during the process of particle migration is revealed.
2. Sample preparation and test scheme
2.1. Materials and sample preparation
The bimsoils in the engineering are mainly composed of rock, clay and sand, in which the rock content is 5–80% (Xu et al. Citation2007; Gao et al. Citation2019). Before designing the rock contents and the particle size of rock of bimsoils in laboratory tests, the boundary size of soil and rock that is also defined the soil and rock threshold should be determined. The threshold value definition method adopted in this paper is proposed by Medley, which is according to the scale-independence of bimsoils, and it should satisfy EquationEq. (1)(1)
(1) (Medley Citation1994). The establishment of this method was based on a large number of statistical data and self-similar theory, which is easy for samples preparation and weaken the influence of size effect.
(1)
(1)
Where dS/R is the soil and rock threshold, and A is the area of study zone of bimsoils, for laboratory experiments, Lc is the engineering characteristic scale of bimsoils, and it is also the diameter of samples in the laboratory tests.
The dimensions of samples in the experiments are 100 × 300 mm and 50 × 100 mm (d × h), as a reason, the smaller value (namely 2.5 mm) is determined as the soil and rock threshold. Meanwhile, according to the Test Methods of Soils for Highway Engineering (JTG E40-2007), the diameter of samples should be 5–6 times of the maximum particle size of samples, and the smaller value of 10 mm is taken as the maximum particle size of the bimsoils. To sum up, fine particles with particle size less than 2 mm are used as soil matrix, and coarse particles with particle size of 5–10 mm are treated as rock mass. Previous studies have shown that the permeability of the bimsoils depends on the joint action of the fine-grained soil and the coarse-grained soil only when the rock content ranges 30–70% (Xu et al. Citation2016). Therefore, the rock content of the bimsoils is designed as 30%, 45% and 60%, and the rock size distribution of 5–6 mm, 6–8 mm and 8–10 mm are employed.
In order to analyse the influence of fine-grained composition on the permeability of bimsoils quantitatively, the soil is divided into fine sand (0–0.5 mm) and coarse sand (0.5–2 mm). The common gradation methods include Fuller theory and Talbot theory (Zha et al. Citation2009). Among them, Talbot formula provides a method to describe the grain size distribution and it is simplified to one parameter, which is convenient for studying the influencing factors. Thus, the Talbot gradation theory is adopted to describe the grain size distribution of sand in this study. The fine particles with different particle sizes are proportioned according to Talbot formula as shown in EquationEq. (2)(2)
(2) (Lindquist Citation1994; Wang et al. Citation2020), and the mass percentage of sand with different particle size are listed in . It could be known from that the content of coarse sand in the bimsoil samples increases with the increase of Talbot index.
(2)
(2)
where n is Talbot index, Si is the current particle size of bimsoils, D is the largest particle size of bimsoils and Pi is the percentage of the particles that the particle size is smaller than or equal to Si.
Table 2. Mass percentage of sand particles in different particle sizes.
There are loose and weak cementation bimsoils in the natural conditions and the modified strong cemented bimsoils after artificial transformation also exist in the engineering. In present study, clay mixed with non-cohesive bimsoils is regarded as the natural weak cementation state, and cement mixed with non-cohesive bimsoils represents the artificially modified strong cementation state.
As shown in , the samples are prepared with quartz sand as the soil matrix, limestone as the rock and clay and cement as the cementing materials. It should be noted that the clay used in the experiment is collected from the fault zone fault gouge of Xuzhou metro project in the Jiangsu province of China, and the main minerals of clay are kaolinite, muscovite and quartz with an average density of 2.06 g/cm3. Besides, the composite Portland cement PC32.5 with the density of 2.98 g/cm3 is adopted.
TS-70 constant-head permeameter (see ) is adopted to test the permeability of non-cementation and clay cemented bimsoils, while HYS-4 permeameter for rock mass (see ) is used to measure the permeability of cement bonded bimsoils. Although the different apparatuses are employed for measuring the permeability of samples, the results that obtained from the permeameters both represent the permeability corresponding bimsoil materials. More specifically, the permeability coefficient measured by TS-70 constant-head permeameter is based on Darcy's Law and obeys EquationEq. (3)(3)
(3) and the permeability coefficient measured by HYS-4 permeameter for rock mass is calculated by EquationEq. (4)
(4)
(4) .
(3)
(3)
(4)
(4)
where KT,constant is the measured permeability coefficient at temperature T by TS-70 constant-head permeameter (cm/s), KT, rock is the measured permeability coefficient at temperature T by HYS-4 permeameter for rock mass (cm/s), Q is the amount of permeated water (cm3), t is the permeation time (s), h is the height of sample (cm), A is the sectional area of sample (cm2), H is the average water head difference (cm), ρ is the density of water (g/cm3), g is the gravitational acceleration (cm/s2), P is the hydraulic pressure difference at both ends of the sample (Pa).
Figure 2. Laboratory apparatus for testing the permeability coefficient of bimsoils. (a) TS-70 constant-head permeameter (b) Permeameter for rock mass.
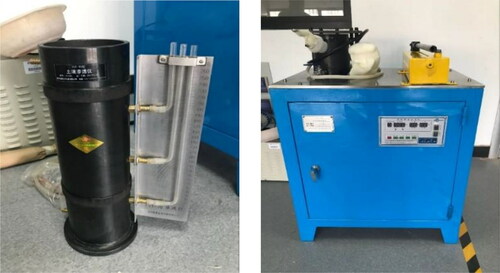
The bimsoil samples are prepared according to the mass mix proportion. According to Test methods of materials stabilized with inorganic binders for highway engineering (JTG E51-2009), the samples are prepared by layered loading and the depth of each layer is about 30 mm, meanwhile, each surface is scratched before the next layer added. The density of samples is controlled by filling amount, loading height and striking times. It is obtained that the striking times for each layer of bimsoils with the rock contents of 30%, 45% and 60% are approximately 4, 7 and 11 times, respectively, from the reference of research results and repeated compaction test in the laboratory (Wang et al. Citation2018). The sample dimension of constant-head permeameter is 100 × 300 mm with the filling capacity about 3600 g, and the sample of 50 × 100 mm with the loading capacity about 300 g for permeameter of rock mass. Thus, the average density of sample is about 1.52 g/cm3.
The sample of constant-head permeameter can be filled and prepared directly in the barrel of the equipment. The main steps are shown in including: mixing the raw materials uniformly in proportion, filling and compacting layer by layer, saturating the samples by the water from the bottom of the apparatus after filling each layer, and laying gravels as buffer layer after reaching the specified height. However, the sample of permeameter for rock mass needs to be prefabricated in the mold and the main steps are similar to above as shown in . Moreover, the sample of cement cementation is supposed to take into standard curing room with the temperature of 20 ± 2 °C and the humidity above 95% for 7d. It should be noted that the samples of cement cementation should be fully immersed in water for 24 h to ensure the samples could be saturated before permeability test.
In order to have an in-depth study on the variable mass seepage of bimsoils, a new apparatus has been designed and developed. As shown in , the test system is mainly composed of bearing tube, water tank, water pump with a water meter, sifter and collecting buckets with an electronic scale (Wang et al. Citation2019b). It should be noted that the device can accommodate a specimen with a 500 mm length and a 150 mm diameter.
Figure 4. Experimental apparatus for variable mass seepage of bimsoils. (a) Schematic layout of the experimental system (b) Seepage apparatus and lost materials collection system
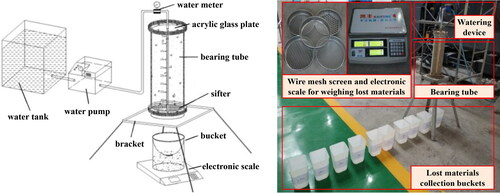
The raw materials of variable mass seepage experiments of bimsoils are shown in . According to the soil and rock threshold in EquationEq. (1)(1)
(1) , the particle sizes of 0–2 mm and 2–3 mm are selected as sand matrix for bimsoils with the Talbot index of 0.4. While the rock sizes of 8–12 mm, 12–16 mm, 16–20 mm and 20–24 mm are designed (representing the radius ratio of soil and rock of 4, 5.3, 6.6 and 8, respectively) and with the rock content of 60%.
2.2. Test schemes
The orthogonal design method is adopted to study the significant differences and the optimal combination of factors level among the factors of rock content, rock size, Talbot index and cementation degree. As the laboratory experiment contains four factors and at three different levels, the orthogonal table L9(34) is chosen to determine the test schemes. There are nine groups of orthogonal tests are carried out while without considering the interaction of various factors. In addition, the average value of repeat 3 times of parallel tests for each group is taken as the result to reduce the error of test. The influencing factors and levels of orthogonal test are shown in , and the detailed test scheme is shown in .
Table 3. Factors and levels of orthogonal test.
Table 4. Test schemes and results of orthogonal design.
Moreover, permeability tests of variable cementation degree under different rock contents are carried out for further study about the interrelations between cementation degree and rock content on the permeability of bimsoils. Two groups of clay cementation and cement bonded bimsoil specimens are designed, and the no cemented bimsoils tests are added for comparison. The rock contents of the samples of two groups are 30%, 45%, 60%, and the content of cementing agent are 6%, 8%, 10%. Amongst them, the Talbot index of clay cemented bimsoils is 1.0, and the rock size is 8–10 mm, while the Talbot index is 0.7 and the rock size is 6–8 mm for cement bond. The specific test scheme is shown in times of parallel tests is conducted for each group.
Table 5. Interactive test schemes and results of rock content and cementation degree.
In addition, the test scheme of variable mass seepage of bimsoils is designed and shown in . It should be noted that the mixture is filled into the acrylic glass bearing tube and in layers with depth of each layer is 50 mm. Each surface was scratched before the next layer was added. Moreover, the water velocity was characterized by the flow and the water flow of Q = 0.1 L/s is selected in this experiment. Therefore, according to the conversion relationship between water velocity and flow, the corresponding water velocity is 0.32 m/s (Wang et al. Citation2021a). During the experiment, the initial value of water meter was recorded, and the tap was turned on to the preset position to control the flow. Then, the mass loss process of the sample was observed and recorded. After the water inrush, the lost mixture was collected every 20 seconds by a series of collection buckets until the end of the experiment.
Table 6. Test schemes of variable mass seepage of bimsoils.
3. Experimental results and discussion
3.1. Analysis of main control factors
Based on the results of permeability test shown in , the important order of the factors on the permeability of bimsoils is determined by range analysis. Range (Rj) is an index of direct analysis for orthogonal test, the analysis process and results are shown in . Range (Rj) reflects the variation range of the permeability coefficient when the level of factor j changes. The greater the Range (Rj), the greater the impact of this factor on the permeability, namely it is more important. Moreover, the influence trend and range distribution of different factors are plotted as shown in .
Table 7. Range analysis of the results of orthogonal test.
It can be seen from that the rock content and cementation degree of the bimsoils have a great influence on the permeability, while it is less affected by Talbot index and rock size. More specifically, the permeability increases with the increase of Talbot index, and the larger of the Talbot index, the smaller for the content of fine particles in soil matrix, in other words, the permeability of bimsoils increases with the decrease of fine particle content to a certain extent. Moreover, the permeability increases with rock content and rock size increasing, and the effect of rock size on permeability is similar to Talbot index. Distinguishingly, the cementation degree has a significant effect on the decline of permeability, especially from the condition of no cementation to clay cementation. In addition, the permeability of cement bonded bimsoils is smaller than that of clay cementation. Therefore, A3B3C3D1 is the optimal combination of high permeability and A1B1C1D3 for the low permeability in this experiment.
According to the range analysis result of RB > RD > RC > RA in and , the order of influence of different factors on the permeability of bimsoils can be obtained that B → D → C → A. As a result, among the factors explored in this test, the rock content has the greatest influence on the permeability of bimsoils; the cementation degree comes second with the characteristic that the stronger the degree of cementation, the greater the influence on permeability; following by the rock size; and the Talbot index has the least influence.
Since the range analysis could not distinguish whether the difference of result is caused by test error or different levels of factors, variance analysis of orthogonal test is adopted to compensate for these shortcomings. Furthermore, the significance of each factor on the permeability coefficient of bimsoils could be evaluated by variance analysis.
The detailed calculation process of variance analysis is shown in . Since all columns in the orthogonal table of this experiment are arranged by factors and there is no empty column, the minimum value of SSj is regard as the sum of variation square of error SSe, namely SSe = SSA. The calculated F value of factors is compared with the corresponding critical value from the F-distribution Table. It is considered that the factor has a significant effect on the test results and with a credibility of (1 – α) ×100% when the F value is greater than the critical value Fα.
Table 8. Variance analysis of orthogonal test.
For the index of permeability coefficient, the statistic F of rock content is far greater than F0.025 when the test at level α = 0.025, which indicates that rock content has a highly significance on the permeability of bimsoils and with a credibility of 97.5%. Similarly, the influence of cementation degree on permeability is more significant and with a credibility of 90%, the influence of rock size on permeability is significant and with a credibility of 75%, and the influence of Talbot index on permeability is not significant. According to the definition of F value that the ratio of the average of sum of deviation squares caused by the change of factor level to the average of sum of deviation squares of error, the influence of factor level change on the experiment results is greater than that of error when F value is more than 1. Therefore, it is also indicated that the difference of test results is caused by different levels of factors, instead of test error in this study. Moreover, the permeability of bimsoils could be greatly affected by rock content and cementation degree based on the test result, and it might be of some significance for engineering or experiment materials preparation by changing rock content and cementation degree or adjusting Talbot index and rock size to reach the required permeability.
3.2. Analysis of interrelations between cementation degree and rock content
3.2.1. Analysis on permeability test of clay cemented bimsoils
According to the interactive test results of clay cemented bimsoils in and the analysis of , the permeability coefficient of clay cemented bimsoils decreases with the increase of clay content and increases with the increase of rock content. However, the permeability coefficient of the bimsoils does not continue to decrease, but has a certain extent increase when the clay content is from 6% to 8%, and the amplitude of the increase increasing with the increase of rock content. Furthermore, the growth rate of permeability coefficient with clay content under different rock content is given in . It is vividly that the permeability coefficient decreases about 20%–40% and 55% with clay content from 0% to 6% and 8% to 10%, respectively, while increases by about 30% with the clay content from 6% to 8%, and the greater the clay content, the smaller the difference of permeability coefficient growth rate in different rock contents.
With the analysis of the dried sample of bimsoils after permeability test shown in , the clay mainly exists in the form of powdery soil particles when the clay content of 6%, which mainly fills the spaces between particles, thus the cohering effect is not obvious. There are sand particles wrapped with clay in the dried sample of the clay content of 8%, which indicates the clay plays a role in the bond of sand and leads to the formation of the larger sizes of agglomerates. However, the content of clay is not enough to bond the rock particles and resulting in the loose and porous structure consisting by the particles such as agglomerates and rock. These agglomerates perform a similar role to rocks during the permeability test, and the agglomeration effect is equivalent to increasing the rock content of the bimsoils and brings more connected pore structure, thus, improving the permeability of the sample. Moreover, the relationship between the rock content and permeability growth is similar to the trend of exponential function according to the range analysis () in Subsection 3.1, as a reason, the existence of agglomerates enables the permeability of high rock content samples increases more rapidly. The permeability coefficient becomes decline when the clay content is from 8% to 10% and the slope of curve increases with the increase of rock content. The results show that there are still agglomerates of larger size in the dried samples at clay content of 10%, but there is also a lot of dense combination effect of agglomerates and rock. It is indicated that the clay has filled the space between the agglomerates and rock, which greatly reduces the permeability of the samples. Besides, the permeability of clay is smaller than that of sand and rock, therefore, the decrease of permeability coefficient of high rock content bimsoils is greater.
3.2.2. Analysis on permeability test of cement bonded bimsoils
From the interactive test results of cement bonded bimsoils in and the analysis of , the permeability coefficient of cement bonded bimsoils distinctly decreases with the increase of cement content and increases with the increase of rock content. In addition, the permeability coefficient of bimsoils with cement binder is far less than that of clay cementation. However, when the rock content is larger (more than 60% in the test) and the cement content is smaller (less than 6% in the test), the effect of clay and cement on the permeability coefficient of bimsoils is similar. It is indicated that the bimsoils are less affected by cement binder under the condition of higher rock content and smaller cement content, and it still reflects the hydraulic properties of the sand and rock matrix.
The permeability coefficient of bimsoils with higher rock content decreases more evidently under the action of cement cementation. For example, when the cement content is 0 → 6%, the permeability coefficient curve of the samples with different rock content changes basically the same and decline more rapidly. When the cement content is 6% → 10%, the permeability coefficient curve of the 60% rock content sample still maintains a faster decline rate, while the decline rate of the 45% and 30% rock content samples appear gradually slow down and tend to stability. According to previous research results, the influence of large voids on permeability is much greater than that of small voids, and cement is easy to fill the large voids preferentially (Garcia et al. Citation2020). From the analysis of , the cement content of 6% plays the role of filling large voids of bimsoils, and the filling effect is basically the same among different rock contents. The cement content of 8% has filled most of the larger voids of the 30% rock content samples. However, there is still large voids in bimsoils with high rock content, and result in the larger decline in permeability coefficient. As for the cement content of 10%, the large voids of the bimsoils with rock content of 45% and 30% have been basically filled, and the slightly smaller voids between the particles are gradually filled, while the sample with rock content of 60% is still in the stage of large voids filling. It can be seen from that the increase in cement content makes the slope of the permeability curve decrease gradually with rock content, that is, the permeability characteristics of bimsoils with different rock content will eventually come close to as the cement content increasing.
In a word, the permeability of the non-cohesive bimsoils can be adjusted by controlling the content of the weak cementing agent in practical applications. Attention should be paid to the existence of a certain content of weak cementing agent (clay content of 8% in this test) which produces agglomeration effect. With the increase of the content of strong cementing agent, the permeability of the strong cementation state bimsoils with different rock content tends to be the same. Therefore, in order to achieve the same permeability in engineering, the grouting volume can be designed in combination with rock content and other specific engineering parameters, and the higher the rock content, the corresponding grouting volume should be increased accordingly. The research results have a certain reference for the reinforcement of bimsoils and the preparation of fillers and similar materials.
3.3. Influence of rock size on time-varying characteristics of variable mass seepage of bimsoils
It is known from Subsection 3.2.1 that the permeability of bimsoils with different particle sizes are quite different, and the permeability characteristics are significantly reflected in the seepage process of loose materials. However, the permeability only reflects the static hydraulic characteristics of bimsoils, and the particles of bimsoils migrate and lose continuously under the action of seepage, and resulting in a dynamic process for the internal structure of samples. Moreover, variable mass seepage experiment could better reflect the engineering characteristics of bimsoils, especially in fault fracture zone or porous materials and it has more significant reference for engineering (Wang et al. Citation2019b, Citation2020, Citation2021a). Therefore, the hydraulic characteristics of bimsoils are analyzed from permeability characteristics and variable mass seepage characteristics in this study.
A self-developed apparatus of variable mass seepage was adopted to further study the influence of rock size on the variable mass loss characteristics of bimsoils under the seepage condition (detailed in Subsection 2.1). During the test, water entered at a speed of 0.32 m/s from the top of bimsoils and controlled by water flows of 0.1 L/s. The samples became wet and water-saturated gradually, and some of the small particles migrate to the bottom of the sample at the same time. After 20 to 30 seconds, water inrush and particle loss occured, and accompanied by settlement of sample surface under the action of seepage and gravity. Finally, a relatively stable structure was formed after particles migration, particles loss, and position adjustment. Note that the collecting and recording the mass of the lost material should be conducted every 20 seconds after water inrush occured (see ).
Figure 9. The collections of lost material during the whole of seepage experiments (radius ratio of 5.3).
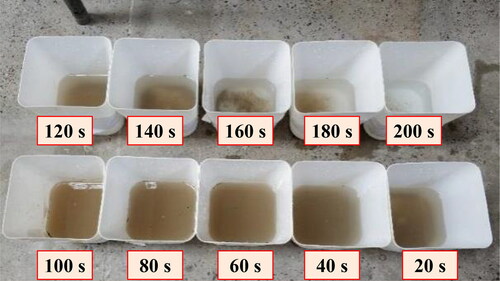
In order to further study the characteristics of lost materials, water and different particle sizes of each bucket are separated by wire mesh screen, and summarizes the curve of the water and the particle loss mass with time. It should be noted that the total loss mass in is the total mass of water and particles in each bucket, namely the total mass of lost materials every 20 seconds after water inrush. The results shown that the loss mass of different particles sizes in the four groups of variable mass seepage experiments is similar and taking an example of the radius ratio of 5.3 in , the loss of fine particles plays a dominant role in the process of variable mass seepage of bimsoils, and the lost mass gradually decreases with time. However, the secondary loss of a large amount of particles and secondary water and particles inrush will occur in the bimsoils with larger radius ratio. It is indicated that the process of particle migration is also a process of the internal structure adjustment of the bimsoils under the action of gravity and seepage conditions. The migration and loss of fine particles between the rocks are the main causes of the formation of rock-dominated skeleton structure. Besides, the adjustment of particle position increases the porosity of bimsoils and promotes the formation of advantageous seepage channels.
With the analysis of the particle loss mass of different radius ratios (see ), it could be known that the voids between the particles in bimsoils perform larger as the increase of particle radius ratio, which leads to the more particle loss. Furthermore, it is found that the secondary inrush is mainly caused by the loss of a large amount of fine particles and the time needed for the secondary inrush increases with the increase of particle radius ratio. The result indicates that the fine-grained sand has a certain self-stability and energy storage capacity, but it is prone to sudden instability and energy release. As shown in , the water loss mass increases with the loss of particles during water inrush, and the maximum water inflow occurs at the second water inrush. Finally, the amount of water inrush tends to be a constant value after the stable structure of the bimsoils is built and there is no particle loss. The water inflow and the total loss mass (see ) generally increase with the increase of particle radius ratio in the early stage of water inrush, but tend to a constant value in the final stable stage. As a reason, it is suggested that it is more accurate to judge the seepage stage and the stable state of bimsoils by the amount of water inflow.
4. Conclusions
Aiming at the permeability and seepage characteristics of bimsoils, laboratory tests have been conducted to investigate the permeability of bimsoils considering the cementation state, and the influence of Talbot index, rock content, rock size and cementation degree on the permeability of bimsoils are evaluated. Furthermore, the time-varying characteristics of the variable mass seepage of bimsoils with different rock particle sizes have been studied by the self-developed apparatus. The main conclusions are obtained as follows.
There are distinct differences in the influence of various factors on the permeability of bimsoils, and the order of the influencing factors is rock content > cementation degree > rock size > Talbot index. Moreover, the permeability decreases as the cementation degree increasing and increases with the increase of other three factors. According to the analysis of variance, the influence of rock content on the permeability of bimsoils is highly significant, the cementation degree is more significant, the rock size is significant, and the Talbot index of fine particle is not significant.
The permeability of clay cemented bimsoils generally increases with the increase of rock content and decreases with the increase of clay content. However, the permeability coefficient increases slightly at the clay content of 8%, and the main reason is that the lower content clay fills the particles space with the form of powder, and the cohere effect is not obvious; the medium content clay presents the agglomeration effect with sand particles, which causes more connected pores in bimsoils and increases permeability; the higher content clay fills a large number of interstices between rock, soil and agglomeration, which produces a well combination effect and greatly reduces the permeability of the bimsoils. In addition, the larger the clay content, the smaller the difference of permeability growth rates under different rock content.
The permeability of cement bonded bimsoils decreases obviously with the increase of cement content, and increases with the increase of rock content. Meanwhile, the permeability coefficient of cement-bonded bimsoils is much smaller than that of clay cementation, and the effect of strong cementing agent on the permeability of the bimsoils with high rock content is more obvious. However, as the content of cementing agent increasing, the permeability eventually tends to be the same.
The variable mass seepage of the bimsoils is dominated by the loss of fine particles. Furthermore, secondary water and particles inrush would occur under the condition of a relatively larger radius ratio, and the occurrence of secondary inrush is also caused by the loss of a large amount of fine particles. With the migration of fine particles, the internal structure of the bimsoils is adjusted constantly, and finally a skeleton structure dominated by rock and advantageous seepage channels is formed. The particle loss mass and the time needed for secondary inrush both increase with the increase of the particle radius ratio. It should be noted that the maximum water loss mass occurs in the process of secondary inrush, and the water inflow tends to a stable value after a stable structure is formed inside the bimsoils finally.
Disclosure statement
No potential conflicts of interests were reported by the authors.
Data availability statement
All data, models, and code generated or used during the study appear in the published article.
Additional information
Funding
References
- Belkhatir M, Schanz T, Arab A. 2013. Effect of fines content and void ratio on the saturated hydraulic conductivity and undrained shear strength of sand-silt mixtures. Environ Earth Sci. 70(6):2469–2479.
- Chen T, Yang YT, Zheng H, Wu ZJ. 2019. Numerical determination of the effective permeability coefficient of soil-rock mixtures using the numerical manifold method. Int J Numer Anal Methods Geomech. 43(1):381–414.
- Ding XH, Zhou W, Lu X, Gao Y. 2018. Physical simulation test of soil-rock mixture from synthetic transparent soil. J Cent South Univ. 25(12):3085–3097.
- Feng SX, Chai JR, Xu ZG, Qin Y. 2018. Evaluating the mesostructural changes of laboratory created soil-rock mixtures using a seepage test based on NMR technology. J Test Eval. 46(3):20160452–20160891.
- Gao QF, Dong H, Huang RQ, Li ZF. 2019. Structural characteristics and hydraulic conductivity of an eluvial-colluvial gravelly soil. Bull Eng Geol Environ. 78(7):5011–5028.
- Garcia ESH, Thives LP, Ghisi E, Antunes LN. 2020. Analysis of permeability reduction in drainage asphalt mixtures due to decrease in void volume. J Clean Prod. 248:119292.
- Hu F, Li ZQ, Tian YF, Hu RL. 2021. Failure patterns and morphological soil-rock interface characteristics of frozen soil-rock mixtures under compression and tension. Appl Sci. 11(1):461.
- Huang Z, Jiang ZQ, Zhu SY, Wu XS, Yang LN, Guan YZ. 2016. Influence of structure and water pressure on the hydraulic conductivity of the rock mass around underground excavations. Eng Geol. 202(4):74–84.
- Jin L, Cheng T, Zhang Y, Li JJ. 2021. Three-dimensional lattice Boltzmann simulation of the permeability of soil-rock mixtures and comparison with other prediction models. Int J Numer Anal Methods Geomech. 45(8):1067–1090. doi: https://doi.org/10.1002/nag.3193.
- Justo JL, Morales-Esteban A, Justo E, Jiménez-Cantizano FA, Durand P, Vázquez-Boza M. 2019. The dry closure of the Almagrera tailings dam: detailed modelling, monitoring results and environmental aspects. Bull Eng Geol Environ. 78(5):3175–3189.
- Kalender A, Sonmez H, Medley E, Tunusluoglu C, Kasapoglu KE. 2014. An approach to predicting the overall strengths of unwelded bimrocks and bimsoils. Eng Geol. 183:65–79.
- Khorasani E, Amini M, Hossaini MF, Medley E. 2019. Statistical analysis of bimslope stability using physical and numerical models. Eng Geol. 254:13–24.
- Lin YX, Peng LM, Lei MF, Yang WC, Liu JW. 2019. Mechanical properties of bimrocks with high rock block proportion. J Cent South Univ. 26(12):3397–3409.
- Lindquist ES. 1994. The strength and deformation properties of mélange [Ph.D. thesis]. Berkeley, CA: University of California.
- Liu W, Zhang ZX, Chen J, Jiang DY, Wu F, Fan JY, Li YP. 2020. Feasibility evaluation of large-scale underground hydrogen storage in bedded salt rocks of China: a case study in Jiangsu province. Energy. 198:117348.
- Luan BY, Zhou W, Meng X, Lu X, Liu ZC. 2020. Study on permeability of soil-rock mixture in water-blocking layer of open-pit coal mine dump site. Adv Civ Eng. 2020:1–10.
- Ma D, Bai HB, Miao XX, Pu H, Jiang BY, Chen ZQ. 2016. Compaction and seepage properties of crushed limestone particle mixture: an experimental investigation for Ordovician karst collapse pillar groundwater inrush. Environ Earth Sci. 75(1):11.
- Ma D, Rezania M, Yu HS, Bai HB. 2017. Variations of hydraulic properties of granular sandstones during water inrush: effect of small particle migration. Eng Geol. 217:61–70.
- Medley EW. 1994. The engineering characterization of melanges and similar block-in-matrix rocks (bimrocks) [Ph.D. thesis]. Berkeley, CA: University of California.
- Napoli ML, Barbero M, Ravera E, Scavia C. 2018. A stochastic approach to slope stability analysis in bimrocks. Int J Rock Mech Min. 101:41–49.
- Park J, Santamarina JC. 2017. Revised soil classification system for coarse-fine mixtures. J Geotech Geoenviron Eng. 143(8):04017039.
- Qian JF, Yao YS, Li J, Xiao HB, Luo SP. 2020. Resilient properties of soil-rock mixture materials: preliminary investigation of the effect of composition and structure. Materials. 13(7):1658.
- Ren MH, Zhao GS. 2021. Prediction of compressive strength of the welded matrix-rock mixture by meso-inclusion theory. Int J Rock Mech Min. 139:104612.
- Spagnoli G, Shimobe S. 2020. An overview on the compaction characteristics of soils by laboratory tests. Eng Geol. 278:105830.
- Wang T, Liu SH, Feng Y, Yu JD. 2018. Compaction characteristics and minimum void ratio prediction model for gap-graded soil-rock mixture. Appl Sci. 8(12):2584.
- Wang Y, Li CH, Hou ZQ. 2019a. Mechanical behaviors of bimsoils during triaxial deformation revealed using real-time ultrasonic detection and post-test CT image analysis. Arab J Geosci. 12(1):10.
- Wang Y, Li X, Zheng B, Mao TQ, Hu RL. 2016a. Investigation of the effect of soil matrix on flow characteristics for soil and rock mixture. Geotech Lett. 6(3):226–233.
- Wang Y, Li X, Zheng B, Zhang YX, Li GF, Wu YF. 2016b. Experimental study on the non-Darcy flow characteristics of soil–rock mixture. Environ Earth Sci. 75(9):756.
- Wang YC, Chen F, Li XZ, Yin X, Geng F, Zheng SH. 2021a. Experimental investigation on mass loss characteristics of broken rocks with discontinuous gradation. Int J Geomech. 21(9):4021168.
- Wang YC, Chen F, Li XZ, Yin X, Lei YT. 2020. The variable-mass seepage law of broken porous rock: an experimental study. Geomat Nat Haz Risk. 11(1):1991–2005.
- Wang YC, Geng F, Yang SQ, Jing HW, Meng B. 2019b. Numerical simulation of particle migration from crushed sandstones during groundwater inrush. J Hazard Mater. 362(2019):327–335.
- Wang YC, Li ZY, Jing HW, Li YB, Wang MT. 2021b. Study on the seepage characteristics of deep buried tunnels under variable high-pressure water heads. Bull Eng Geol Environ. 80(2):1477–1487.
- Wang YC, Zheng SH, Li YL, Wang YM, Huang YH. 2021c. The failure characteristics around shallow buried tunnels under rainfall conditions. Geomat Nat Haz Risk. 12(1):363–380.
- Wei H-Z, Xu W-J, Xu X-F, Meng Q-S, Wei C-F. 2018a. Mechanical properties of strongly weathered rock-soil mixtures with different rock block contents. Int J Geomech. 18(5):04018026.
- Wei HZ, Xu WJ, Wei CF, Meng QS. 2018b. Influence of water content and shear rate on the mechanical behavior of soil-rock mixtures. Sci China Technol Sci. 61(8):1127–1136.
- Wu WN, Yang YT, Zheng H. 2020. Hydro-mechanical simulation of the saturated and semi-saturated porous soil-rock mixtures using the numerical manifold method. Comput Method Appl Mech Eng. 370:113238.
- Xu JC, Shang YQ. 2006. Influence of permeability of gravel soil on debris landslide stability. Chin J Rock Mech Eng. 25(11):2264–2271.
- Xu WJ, Hu RL, Tan RJ. 2007. Some geomechanical properties of soil-rock mixtures in the Hutiao Gorge area, China. Géotechnique. 57(3):255–264.
- Xu WJ, Wang S, Zhang HY, Zhang ZL. 2016. Discrete element modelling of a soil-rock mixture used in an embankment dam. Int J Rock Mech Min. 86:141–156.
- Xu WJ, Yue ZQ, Hu RL. 2008. Study on the mesostructure and mesomechanical characteristics of the soil-rock mixture using digital image processing based finite element method. Int J Rock Mech Min. 45(5):749–762.
- Xu WJ, Zhang HY. 2021. Research on the effect of rock content and sample size on the strength behavior of soil-rock mixture. Bull Eng Geol Environ. 80(3):2715–2726.
- Xu Y, Gao Q, Li X, Li JH, Jia YX. 2009. In-situ experimental study of permeability of rock and soil aggregates. Rock Soil Mech. 30(3):855–858.
- Xue YG, Li GK, Wang XK, Yang WM, Qiu DH, Su MX. 2020. Analysis and optimization design of submarine tunnels crossing fault fracture zones based on numerical simulation. Mar Georesour Geotec. 38(9):1106–1117.
- Zha JF, Guo GL, Wang Q, Ma ZG. 2009. Study of in-situ sieving experiment and gradation optimization of gangue. Proc Earth Planet Sci. 1(1):754–759.
- Zhou Z, Li F, Yang H, Gao WY, Miao LW. 2019. Orthogonal experimental study of soil-rock mixtures under the freeze-thaw cycle environment. Int J Pavement Eng. 22(11):1376–1388.
- Zhou Z, Yang H, Wang XC, Liu BC. 2017. Model development and experimental verification for permeability coefficient of soil-rock mixture. Int J Geomech. 17(4):04016106.
- Zhang C, Ma CK, Chen QL, Liu HM, Wu SW, Pan ZK, Zhang L. 2021. Influence of rock percentage on strength and permeability of tailing-waste rock mixtures. Bull Eng Geol Environ. 80(1):399–411.