ABSTRACT
In a recent study, we reported on two distantly related, epidemic lineages of Clostridium difficile that have acquired independent mechanisms to metabolize low concentrations of trehalose. Here we provide further comment and evidence that a third epidemic lineage common in Asia and Europe, ribotype 017, has evolved this ability.
Introduction
Clostridium difficile infection (CDI) is the leading cause of hospital associated infection in the developed world. Symptoms range from mild diarrhea to life threatening pseudomembranous colitis. The Clostridium difficile species is composed of hundreds of genetic lineages (also known as ribotypes), though only a small number are responsible for the majority of infections.Citation1 We have recently shown that two epidemic ribotypes, RT027 and RT078, have evolved novel methods to metabolise low levels of trehalose.Citation2 A disaccharide of glucose, trehalose was introduced to the food chain en masse during the early 2000s following a precipitous drop in manufacturing costs and “generally regarded as safe” (GRAS) status in the USA and Europe. In this addendum we expand on the ideas put forth in our prior publication and provide new data on a third epidemic ribotype, RT017, that is frequently found in Asia and Europe.
Colonization resistance, nutrient niches, and CDI infection
Treatment with antibiotics is the predominant risk factor associated with CDI; as antibiotics significantly alter the composition and diversity of the intestinal microbiota.Citation3,Citation4 It has been demonstrated that the microbial community present in native microbiota provide resistance against intestinal pathogens, including C. difficile, and antibiotic treatment lowers this resistance.Citation5,Citation6 Colonization resistance is mediated in part by microbial competition for available resources and nutrient limitation,Citation7 a hypothesis first put forth by Rolf Freter.Citation8 Freter’s ‘nutrient niche’ hypothesis states that for a species to be successful in the intestine, it must grow better than other competitors on one or on a small number of growth-limiting nutrients. This hypothesis has been extensively tested and is supported by a robust collection of evidence, including our recent study of C. difficile utilization of trehalose.Citation2,Citation9 The ability of RT027 and RT078 strains to metabolize very low concentrations of trehalose, not detected by other ribotypes, provides a competitive advantage to these strains, potentially helping drive their prevalence clinically.
Dietary trehalose consumption has significantly increased
Prior to 1995 in Japan and 2000/2001 in the United States and Europe, almost all trehalose consumed came via natural sources. Mushrooms, yeast, honey, and shellfish all contain trehalose but their combined dietary contribution is low in comparison to added sugars in processed foods. Data from the USDA lists the per capita consumption of fresh mushrooms and honey in the USA in 2000 as 1.18 kg (with ~ 60% being the common white mushroom, agaricus bisporus) and 0.5 kg respectively. One-hundred grams of fresh white mushrooms contains 0.16 g of trehalose with other common edible mushroom species containing between 0.22–8.01 g trehalose per 100 g fresh weight.Citation10 Honey primarily consists of glucose and sucrose with trehalose accounting for a tiny fraction of the sugar content at 4.6 mg g−1,Citation11 Taken together, a reasonable estimate of trehalose consumption from these products stands at ~ 21 g per person per year. Allowing a liberal 5 fold increase from other dietary sources, realistic trehalose consumption from natural sources could be estimated at < 0.3 g per person per day (USA).
The mid-1990s saw development of an innovative enzymatic process for industrial scale production of trehalose from starch.Citation12 This process led to a precipitous drop in price to less than a hundredth of its initial cost and paved the way for trehalose’ use in the food industry. Regulatory approval in the 2000s led to widespread adoption of trehalose in the USA and Europe. Foods where trehalose addition has been proposed to be beneficial include: baked goods, breakfast cereals, rice and pasta, processed vegetables, fruit (dried and juiced), milk products, meat and fish products, sweets, chewing gum, and ice cream. Owing to its many uses, the FDA GRAS report for trehalose considers a potential mean consumption level of 34.43 g day – many times that consumed via natural sources.Citation13
Trehalose metabolism
Trehalose is not transported as a disaccharide into the bloodstream upon ingestion. Instead, a brush border trehalase enzymatically hydrolyzes trehalose to two D-glucose molecules which are subsequently absorbed and metabolized. Trehalase activity is greatest in the proximal and mid-small intestine, decreasing towards the distal ileum.Citation14
A number of studies have compared blood glucose levels (and other responses) of fasted subjects following consumption of either glucose or trehalose. A consistent finding of these studies was that trehalose ingestion results in a significantly lower blood glucose peak when compared to ingestion of glucose. Crucially, not only were the initial spikes lower but the incremental area under the curve was also significantly reduced.Citation15,Citation16 Trehalose can inhibit glucose transport through solute carrier 2A (SLC2A) transporters with a half-maximal inhibitory concentration (IC50) of 17.3–73.1 mM (SLC2A1–4).Citation17 This could partially account for the observed reduction in blood glucose levels. Paradoxically, if trehalose were efficiently converted to glucose by the brush border trehalase, these glucose molecules would be available to uninhibited SLC2A transporters further along the small intestine. It is likely therefore that not all of the trehalose is hydrolyzed to glucose and absorbed but rather an undetermined amount remains in the lumen where it is available for microbial fermentation. Indeed, this hypothesis is supported by data demonstrating microbial detection of trehalose in the ceca of mice fed trehalose (but not those given water) and in the ileostomy effluent of patients consuming their normal diet.Citation2,Citation18
Able to act as both an energy source and stress protectant, trehalose and its associated metabolic pathways are widely distributed among prokaryotes.Citation19 Although Clostridium difficile is unable to generate its own trehalose, all strains sequenced to date possess a two gene operon (treRA) enabling its catabolism. In the absence of trehalose, a repressor (TreR) binds upstream of treA preventing its transcription (). When present, trehalose is transported into the cell and phosphorylated to trehalose-6-phosphate (T6P) via an as yet unknown transporter. Buildup of sufficient T6P within the cell enables binding of T6P to TreR, derepression of treA, and its subsequent transcription. Despite all ribotypes possessing the ability to metabolise trehalose, only a small number are able to utilize low concentrations, namely RT027/RT244/RT176 (closely related ribotypes in clade 2) and RT078/RT033/RT045/RT066/RT126, found in clade 5. To do this they have evolved independent mechanisms (see ). The RT027 group possess a conserved L172I substitution in the repressor (TreR, see ) that results in exquisite sensitivity to trehalose (). The RT078 group possess a canonical treRA operon as well as an extra 4 gene insert encoding a second trehalase (treA2), repressor (treR2), transporter (ptsT), and trehalose associated protein (treX) (). In this case it is the trehalose transporter that provides the ability to metabolize low trehalose levels by efficiently importing trehalose into the cell.
Figure 1. Trehalose metabolism in Clostridium difficile. (A) Canonical metabolism. Under low trehalose conditions the repressor (TreR) remains bound upstream of treA preventing transcription. Under high trehalose concentrations, trehalose-6-phosphate binds to TreR enabling transcription of treA, its subsequent translation and metabolism of trehalose-6-phosphate to glucose and glucose-6-phosphate. (B) In RT027 group strains a conserved mutation in treR, encoding an L172I amino acid substitution near the the effector site, results in increased sensitivity to trehalose-6-phosphate and derepression of treA under low trehalose conditions. (C) RT078 group strains possess an extra 4 gene cassette as well as the canonical treRA operon. The additional trehalose transporter, PtsT, enables efficient transport and metabolism under low trehalose conditions.
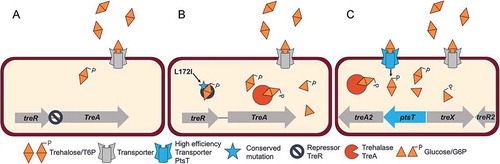
Figure 2. A ribotype 017 strain can utilize low concentrations of trehalose. (A) Biolog 96-well Phenotype MicroArray carbon source plate PM1 was used to examine the metabolic profiles of strains CD2048 (RT053), M68 (RT017) and CD2015 (RT027). Among the carbohydrates tested, strains M68 (RT017) and CD2015 (RT027) displayed a ~ 3 fold increase in growth in the presence of trehalose over no carbohydrate media. CD2048 (RT053) was unable to utilize trehalose. (n = 2) (B) M68 reaches a similar maximum optical density in defined minimal media supplemented with 10 mM trehalose as epidemic ribotype 027. No growth difference is observed in 20 mM glucose. (n = 3). (C) Both M68 (RT017) and CD2015 (RT027) strongly induce treA in the presence of 10 mM trehalose and at a significantly higher level than CD2048 (RT053) . No treA induction is observed in 20 mM glucose.(n = 3). treA expression was normalised to the 16S ribosomal subunit. (D) Multiple sequence alignment of the TreR protein. The consensus sequence for C. difficile near the predicted TreR operator is displayed in large letters while the individual sequences are aligned below. * RT053 sequence is the consensus sequence for all other ribotypes. Figs A-C: All replicates are biological, bars = mean, error = sd.
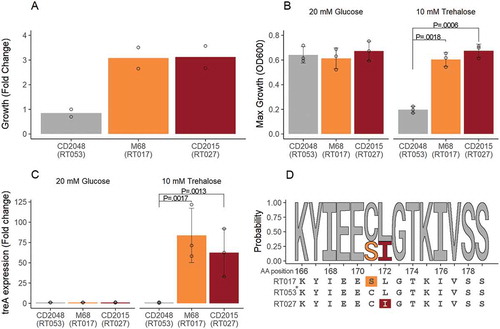
Global spread of C. difficile
First described in 1935 by Hall and O’Toole,Citation20 it wasn’t until 1978 that C. difficile was identified as the predominate bacterial cause of antibiotic-associated diarrhea and pseudomembranous colitis.Citation21 For the ensuing two decades, C. difficile was regarded as little more than an easily treatable, general nuisance.Citation22 Since the turn of the century, however, a small number of epidemic strains (including RT027 and RT078) have caused a resurgence in CDI with increased disease severity.
The spread of C. difficile ribotypes driving disease has not been uniform. While RT027 and RT078 strains are common throughout North America and Europe they are relatively rare in Asia.Citation23 Indeed, the overall prevalence of CDI in Asia has, historically, been low in comparison to North America.Citation24 This may be partially due to lack of surveillance and reporting with recent studies indicating increasing disease incidence. Despite this, the scarcity of these ribotypes in Asia and especially Japan, where consumption of trehalose is high, is both interesting and puzzling. Trehalose is one factor of many that have contributed to the rise of RT027 and RT078 groups. One possibility is another key factor, present in North America and Europe but lacking in Japan, contributes to RT027 and RT078 outbreaks. Another hypothesis is that ribotypes common in Asia have also acquired the ability to utilize low trehalose levels.
A ribotype 017 strain can metabolise low levels of trehalose
RT017 (sequence type 37) strains are characterized as toxin A-negative, toxin B-positive strains and have been attributed to a large number of outbreaks in Asia and Europe.Citation25 One of these strains, M68, was isolated in 2004 from an outbreak in Dublin, Ireland.Citation26 We examined this strain for carbon utilization compared to other C. difficile clinical isolates in Biolog 96-well Phenotype MicroArray carbon source plates. Surprisingly, M68 was able to utilize trehalose at a level comparable to RT027 strains (). To further explore the threshold of trehalose utilization in this strain we performed growth assays in chemically defined minimal medium supplemented with 10 mM trehalose (). In a complementary approach we measured expression of treA, encoding the trehalase enzyme, in minimal medium supplemented with 10 mM trehalose. Expression of treA was induced 83.7 ± 27.4 fold in M68 and 62.4 ± 24.2 fold in CD2015 over the no trehalose control (). In contrast CD2048 (RT053) showed no increase in treA expression. Taken together, these results demonstrate that like epidemic RT027 strains, RT017 strains, can grow on low trehalose concentrations and that metabolism is via the TreA enzyme. Comparative genomic analysis revealed a conserved sequence variation in treR resulting in a C171S amino acid substitution in all RT017 strains sequenced to date. This amino acid substitution is near the predicted effector binding pocket of TreR and immediately adjacent to the L172I amino acid substitution found in RT027 strains ().
Conclusion
The human gastrointestinal tract (GIT) is home to hundreds of coexisting species; each competing within a niche space limited by environmental conditions, nutrient availability, competitors, and predators. To survive bacteria have evolved to become experts at sensing and taking advantage of even small changes in nutrient availability.
High in fats and added sugars, the Western diet has undergone profound changes in recent years. Overall consumption of caloric sweeteners in the USA remained relatively stable between 1970 and 2015 (96.1 ± 7.48 g day). The composition of the sugars we consume, however, has changed significantly. Other than the addition of trehalose during the 2000s, high fructose corn syrup (HFCS) went from accounting for < 0.5% of all sweeteners consumed in 1970 to over 42% by 2003.Citation27 Dietary changes can have multiple effects on the microbiome. Acute shifts in microbial composition have been shown to take place rapidly following a change in diet.Citation27 Further, microbiota in the distal gut of mice fed a Western diet have been shown to be significantly enriched for KEGG pathways involved in the import and fermentation of simple sugars.Citation28 This suggests that the microbiota could not only detect these simple sugars in the distal gut but were able to compete with the host for their absorption and metabolism. Any sustained increase or novel nutrient availability (e.g.trehalose) in the environment provides selective pressures for evolution to work – not only on enteric pathogens but the gut microbiota as a whole. New or existing mutations that enhance detection, import or metabolism of these nutrients provide an advantage to their host and would likely be selected for. The adaptation of the microbiota to dietary sugars (and changes in diet as a whole) is therefore likely an ongoing process.
In the case of trehalose consumption, our data suggests that the increase in dietary trehalose during the 21st century has resulted in this sugar being available in the distal gut where it can be metabolised by microbes. We have now provided evidence that three epidemic strains of Clostridium difficile have evolved independent mechanisms to better metabolize this nutrient resource. Our work does not suggest that people should be broadly concerned about trehalose consumption. We do, however, expect that the impact of dietary trehalose in the severity of CDI will be further investigated in clinical settings.
Additional information
Funding
References
- Knetsch CW, Lawley TD, Hensgens MP, Corver J, Wilcox MW, Kuijper EJ. Current application and future perspectives of molecular typing methods to study Clostridium difficile infections. Eurosurveillance. 2013;18:20381.
- Collins J, Robinson C, Danhof H, Knetsch CW, Van Leeuwen HC, Lawley TD, Auchtung JM, Britton RA. Dietary trehalose enhances virulence of epidemic Clostridium difficile. Nature. 2018;553:291–294.
- Britton RA, Young VB. Role of the intestinal microbiota in resistance to colonization by Clostridium difficile. Gastroenterology. 2014;146:1547–1553.
- Leffler DA, Lamont JT, Longo DL. Clostridium difficile infection. N Engl J Med. 2015;372:1539–1548.
- Schubert AM, Sinani H, Schloss PD. Antibiotic-Induced Alterations of the Murine Gut Microbiota and Subsequent Effects on Colonization Resistance against Clostridium difficile. MBio. 2015;6:e00974–15.
- Pickering T, Hamm JM, Page AF Jr, Wuestner S, Hess O. Cavity-free plasmonic nanolasing enabled by dispersionless stopped light. Nat Commun. 2014;5:3114.
- Wilson KH, Perini F. Role of competition for nutrients in suppression of Clostridium difficile by the colonic microflora. Infect Immun. 1988;56:2610–2614.
- Freter R, Brickner H, Botney M, Cleven D, Aranki A. Mechanisms that control bacterial populations in continuous-flow culture models of mouse large intestinal flora. Infect Immun. 1983;39:676–685.
- Johnston A, Crombie AT, El Khawand M, Sims L, Whited GM, McGenity TJ, Colin Murrell J. Identification and characterisation of isoprene-degrading bacteria in an estuarine environment. Environ Microbiol. 2017;19:3526–3537.
- Reis FS, Barros L, Martins A, Ferreira ICFR. Chemical composition and nutritional value of the most widely appreciated cultivated mushrooms: an inter-species comparative study. Food Chem Toxicol. 2012;50:191–197.
- De La Fuente E, Ruiz-Matute AI, Valencia-Barrera RM, Sanz J, Martínez Castro I. Carbohydrate composition of Spanish unifloral honeys. Food Chemistry. 2011;129:1483–1489.
- Maruta K, Nakada T, Kubota M, Chaen H, Sugimoto T, Kurimoto M, Tsujisaka Y. Formation of trehalose from maltooligosaccharides by a novel enzymatic system. Biosci Biotechnol Biochem. 1995;59:1829–1834.
- GRAS Notice: 45. Trehalose [Internet]. FDA; 2018 May 18. Available from: https://www.accessdata.fda.gov/scripts/fdcc/?set=grasnotices&id=45.
- Asp NG, Gudmand-Höyer E, Andersen B, Berg NO, Dahlqvist A. Distribution of disaccharidases, alkaline phosphatase, and some intracellular enzymes along the human small intestine. Scand J Gastroenterol. 1975;10:647–651.
- Cooper AR, Page S, Fox KR, Misson J. Physical activity patterns in normal, overweight and obese individuals using minute-by-minute accelerometry. Eur J Clin Nutr. 2000;54:887–894.
- Yoshizane C, Mizote A, Yamada M, Arai N, Arai S, Maruta K, Mitsuzumi H, Ariyasu T, Ushio S, Fukuda S. Glycemic, insulinemic and incretin responses after oral trehalose ingestion in healthy subjects. Nutr J. 2017;16:9.
- DeBosch BJ, Heitmeier MR, Mayer AL, Higgins CB, Crowley JR, Kraft TE, Chi M, Newberry EP, Chen Z, Finck BN, et al. Trehalose inhibits solute carrier 2A (SLC2A) proteins to induce autophagy and prevent hepatic steatosis. Sci Signal. 2016;9:ra21.
- Schneider T, Keiblinger KM, Schmid E, Sterflinger-Gleixner K, Ellersdorfer CCGM, Roschitzki B, Richter A, Eberl L, Zechmeister-Boltenstern S, Riedel K. Who is who in litter decomposition? Metaproteomics reveals major microbial players and their biogeochemical functions.. ISME J. 2012;6:1749–1762.
- Ruhal R, Kataria R, Choudhury B. Trends in bacterial trehalose metabolism and significant nodes of metabolic pathway in the direction of trehalose accumulation. Microbial Biotechnology. 2013;6:493–502.
- Hall IC. Intestinal flora in new-born infants: with a description of a new pathogenic anaerobe, Bacillus difficilis. Am J Dis Child. 1935;49:390.
- Bartlett JG, Chang TW, Gurwith M, Gorbach SL, Onderdonk AB. Antibiotic-associated pseudomembranous colitis due to toxin-producing clostridia. N Engl J Med. 1978;298:531–534.
- Gerding DN. Clostridium difficile 30 years on: what has, or has not, changed and why?. Int J Antimicrob Agents. 2009;33(Suppl 1):SS2–8.
- Borren NZ, Ghadermarzi S, Hutfless S, Ananthakrishnan AN, Deshpande A. The emergence of Clostridium difficile infection in Asia: A systematic review and meta-analysis of incidence and impact. PLoS One. 2017;12:e0176797.
- Burke KE, Lamont JT. Clostridium difficile infection: a worldwide disease. Gut Liver. 2014;8:1–6.
- Kosack CS, Shanks L, Beelaert G, Benson T, Savane A, Ng’ang’a A, Bita A, Zahinda J-PBN, Fransen K, Page A-L, et al Designing HIV Testing Algorithms Based on 2015 WHO Guidelines Using Data from Six Sites in Sub-Saharan Africa.. J Clin Microbiol. 2017;55:3006–3015.
- Aboltins CA, Page MA, Buising KL, Jenney AWJ, Daffy JR, Choong PFM, Stanley PA. Treatment of staphylococcal prosthetic joint infections with debridement, prosthesis retention and oral rifampicin and fusidic acid. Clin Microbiol Infect. 2007;13:586–591.
- David LA, Maurice CF, Carmody RN, Gootenberg DB, Button JE, Wolfe BE, Ling AV, Devlin AS, Varma Y, Fischbach MA, et al. Diet rapidly and reproducibly alters the human gut microbiome. Nature. 2014;505:559–563.
- Turnbaugh PJ, Bäckhed F, Fulton L, Gordon JI. Diet-induced obesity is linked to marked but reversible alterations in the mouse distal gut microbiome. Cell Host & Microbe. 2008;3:213–223.