ABSTRACT
Obesity and type 2 diabetes (T2D) are growing burdens for individuals and the health-care system. Bariatric surgery is an efficient, but drastic treatment to reduce body weight, normalize glucose values, and reduce low-grade inflammation. The gut microbiome, which is in part controlled by intestinal antibodies, such as IgA, is involved in the development of both conditions. Knowledge of the effect of bariatric surgery on systemic and intestinal antibody response is limited. Here, we determined the fecal antibody and gut microbiome response in 40 T2D and non-diabetic (ND) obese individuals that underwent bariatric surgery (N = 40). Body weight, fasting glucose concentrations and inflammatory parameters decreased after bariatric surgery, whereas pro-inflammatory bacterial species such as lipopolysaccharide (LPS), and flagellin increased in the feces. Simultaneously, concentrations of LPS- and flagellin-specific intestinal IgA levels increased with the majority of pro-inflammatory bacteria coated with IgA after surgery. Finally, serum antibodies decreased in both groups, along with a lower inflammatory tone. We conclude that intestinal rearrangement by bariatric surgery leads to expansion of typical pro-inflammatory bacteria, which may be compensated by an improved antibody response. Although further evidence and mechanistic insights are needed, we postulate that this apparent compensatory antibody response might help to reduce systemic inflammation by neutralizing intestinal immunogenic components and thereby enhance intestinal barrier function after bariatric surgery.
Introduction
ObesityCitation1 and type 2 diabetes (T2D)Citation2 are pandemics that challenge health-care systems worldwide. The World Health Organization (WHO) defines obesity as excessive fat accumulation that can impair the individual’s health and may lead to comorbidities, such as glucose intolerance.Citation1 Treatment strategies aim to reduce body weight via lifestyle changes that include a balanced diet and an increase in physical activity.Citation1 However, if individuals are not able to reduce their body weight, bariatric surgery may be an efficient, but drastic intervention to overcome severe obesity and its long-term consequences.Citation3
Roux-en-Y gastric bypass (RYGB) reduces the volume of the stomach while bypassing the proximal part of the intestine and changing the route of the ingested food.Citation4 Long-term studies report a substantial reduction in body weight and indicate that RYGB is an efficient way to treat T2D3. Short- and long-term studies report a major improvement in glucose metabolism after RYBG.Citation3 However, bariatric surgery includes a drastic rearrangement of the intestinal route that comes with shifts in the gut microbiome composition.Citation5
The gut microbiome is a collection of microbial organisms, including bacteria, fungi, protozoa, and viruses in the gastrointestinal tract.Citation6 A plethora of studies have highlighted the involvement of the gut microbiome in numerous diseases like obesity and T2D6. An increase in pro-inflammatory bacterial species, including proteobacteria, and bacterial components, such as lipopolysaccharide (LPS) and flagellin may be partially responsible for the so-called low-grade inflammation observed in metabolic diseases.Citation6–8 A pro-inflammatory gut microbiome may also trigger an immune response via bacterial translocation or influx of LPS and flagellin into the blood circulation toward metabolically active tissues.Citation6,Citation7 Nevertheless, convincing evidence for this concept in humans is still lacking.
In line, an intact intestinal epithelium, a functional immune system, and a symbiotic gut community are the major defense systems to prevent leakage of intestinal bacterial strains and/or bacterial components into the blood circulation.Citation6 In obese mouse models, the intestinal epithelium is more permeable, and the intestinal immune response is insufficient to capture pro-inflammatory bacterial molecules.Citation7,Citation8 In particular, intestinal B cells produce less immunoglobulin A (IgA) in an obese setting, which is a sign of an inadequate immune response toward a pro-inflammatory gut microbiome.Citation8
In the current paper, we report on the intestinal and systemic immune response after bariatric surgery in paired samples of 40 severely obese non-diabetic (ND) and T2D individuals from our BARIA cohort.Citation9 RYGB surgery induced a shift toward a pro-inflammatory gut microbiome. Interestingly, systemic low-grade inflammation was reduced after surgery; albeit to a different extent in ND and T2D patients.Citation9 Intestinal IgA levels against pro-inflammatory bacteria and bacterial components increased after bariatric surgery. We postulate that increased intestinal IgA levels upon bariatric surgery neutralize immunogenic bacteria and bacterial components, which aids in lowering the inflammatory tone. However, some pro-inflammatory bacteria might evade the IgA response after surgery.
Results
To investigate the antibody and gut microbiome response after bariatric surgery, we included a total of 40 obese individuals with or without T2D from our BARIA cohort.Citation9 We matched individuals according to age, sex, and body mass index (Table S1) and measured antibody concentrations and gut microbiome composition before as well as one year after surgery. As expected, body weight and glucose response improved after surgery in both groups ().
Table 1. Comparison of characteristics before and after surgery
Bariatric surgery improves systemic inflammation
Systemic inflammation decreased after surgery in both groups (). For example, CRP () and blood leukocytes () decreased in all individuals. Translocation of bacterial components is associated with low-grade inflammation in obese humans.Citation7,Citation10 As a proxy for translocation of bacterial components, we measured bacterial LPS, flagellin, and LPS binding protein (LBP) in the blood circulation. Although serum LPS and flagellin did not significantly differ between the time points (Table S2), LBP decreased upon surgery in both groups (). Similarly, serum lipocalin-2, a player in inflammation and gut microbiota homeostasisCitation11 decreased after surgery ().
Figure 1. Bariatric surgery improves systemic inflammation in humans.
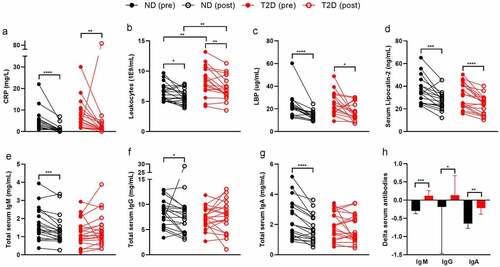
Low-grade inflammation and endotoxemia are associated with T2D.Citation7,Citation10 However, many studies compare lean controls to obese T2D individuals, where obesity is a major confounding factor.Citation12 Here, we matched severely obese ND to severely obese T2D individuals. Age, sex, and BMI did not differ at both time points (Table S1). As expected, fasting glucose and glucose response during a mixed meal test (Figure S1) and HbA1c, a marker for long-term glucose control, were increased in T2D (Table S1). Further, we noticed a numerical increase in C-reactive peptide (CRP, ) and a significantly higher leukocyte count in T2D (), suggesting a low-grade inflammation in this group (Table S1). These differences persisted after bariatric surgery.
Translocation of bacterial components has been associated with T2D,Citation13 yet, serum LPS, flagellin, and LBP did not differ between the two matched groups (Table S1). However, we observed a significant positive correlation between fasting glucose and serum LPS as well as serum flagellin (Figure S2), indicating a relation between disturbed glucose tolerance and translocation of bacterial components. This correlation did not persist after surgery, potentially due to the reduction of all glucose parameters, endotoxemia, and BMI. A reduction in body weight, particularly adipose tissue, has been associated with reduced inflammation and endotoxemia.Citation14,Citation15 Most of the participants had a BMI below 30 after surgery and therefore were categorized as overweight rather than obese.
Intestinal and circulating antibodies serve an important role in preventing translocation of bacterial components or neutralize immunogenic effects.Citation16 T2D individuals had slightly lower serum immunoglobulin (Ig)G, IgA, and IgM levels compared to ND at both time points (Table S1). Serum IgA, IgM, and IgG decreased in ND after surgery, highlighting that the inflammatory tone improved (Table S2, ). In individuals with T2D, serum IgM numerically increased and IgG levels did not change after surgery. Treatment-induced changes in serum antibody levels were significantly different in ND compared to T2D (), suggesting that bariatric surgery is less effective in reducing inflammation in diabetic individuals. Serum antibodies against intestinal-derived compounds, such as LPS and flagellin did not change significantly after RYGB surgery in both groups except for an increase in IgA against LPS (Table S2).
We next questioned whether antibody production from B cells was different between phenotypes and changed after surgery. Therefore, we isolated peripheral B cells from a subset of 12 individuals (at baseline and post-surgery) and measured B cell antibody production ex vivo. To do so, memory B cells with either of the immunoglobulin subsets (IgG, IgM, and IgA) were sorted and differentiated into antibody-produced plasma cells using IL-21 and CD40L. In line with serum antibody levels, B cells derived from individuals with T2D produced less antibodies at both time points than B cells derived from ND individuals (Table S3). The B cell subsets were not significantly different between both groups but changed after surgery. For example, there were less naïve B cells, potentially due to preferred differentiation into other effector B cells. Since we only had B cell subsets relative to CD19+ cells and not total blood B cells, we are unable to provide certainty on this suggestion. Furthermore, B cells derived from post-bariatric surgery individuals produced more antibodies ex vivo (Table S4).
RYGB Surgery induces bloom of typical pro-inflammatory bacteria
Despite the decrease in systemic inflammation after bariatric surgery, we observed an increase from 1.93% to 8.04% in pro-inflammatory Proteobacteria (–b, Table S5). This increase was mostly at the expense of Firmicutes (Figure S3A and S3B), which decreased after surgery in relative abundance (, Table S5). We did not observe major differences in microbial composition between ND and T2D (Table S6), potentially due to our small sample size and extensive group matching. Nevertheless, individuals with T2D had a lower abundance of Streptococcus salivarius than ND (Table S6). Interestingly, S. salivarius has been discussed as an anti-inflammatory (orally derived) bacterial strain.Citation17,Citation18 A decrease in that bacterium might contribute to the higher inflammatory tone of T2D.
Figure 2. Bariatric surgery induces a more pro-inflammatory gut microbiome one year after RYGB surgery.
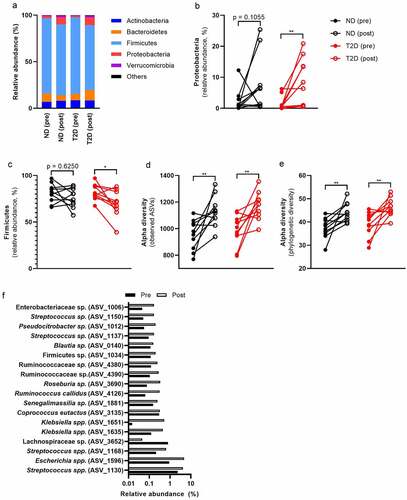
We found that alpha diversity increased after surgery in both groups (). Pre- and post-surgery microbiomes could clearly be separated in both groups (PERMANOVA, p = .005, R2 = 0.041, Figure S4). In this regard, 42 significant amplicon sequence variants (ASVs) changed after surgery (Table S9). Of those, 18 ASVs had an abundance of more than 0.1% () with ASV_1596 (E. coli, 2.8%) and ASV_1130 (S. salivarius, 3.2%) as the most abundant bacteria that changed after surgery. Mostly facultative anaerobic bacteria increased that are able to adapt to the changing environment after RYGB surgery. For example, eight of these ASVs belong to the family Enterobacteriaceae that, as a group, increased in ND and T2D (Table S5). Similarly, 11 ASVs belong to the facultative anaerobe genus Streptococcus that increased after surgery in both groups (Table S5). An increase in oxygenCitation19 or more easily fermented dietary components reach the colon due to the changed route of the intestine after RYGB surgery. That might result in altered relative abundance of facultative anaerobic bacteria.
Despite the improvement in systemic inflammation (CRP, blood leukocytes, serum IgA), we observed an increase in pro-inflammatory fecal LPS and flagellin abundance after RYGB surgery (–b, Table S7), without obvious differences between ND and T2D (Table S8). Increased LPS after RYGB surgery is likely derived from the increase in LPS-carrying Proteobacteria. The intestinal IgA response is important to neutralize bacterial ligands in the intestine.Citation16
Figure 3. Bariatric surgery increases fecal LPS, flagellin and IgA against both bacterial compounds.
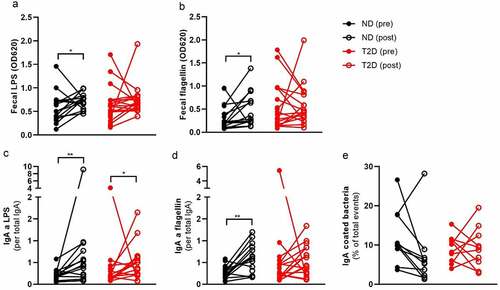
Typical pro-inflammatory bacteria evade IgA coating after RYGB Surgery
Previously, it was reported that RYGB surgery increased total fecal IgA one month after surgery.Citation20 However, there are no reports of IgA coated bacteria or compound-specific fecal IgA. We did not find any significant changes in total fecal IgA one year after surgery (Table S7). Similarly, we did not observe changes in the fecal lipocalin-2 (Table S7), which is a marker for intestinal inflammation.Citation21 However, we observed an increase in LPS and flagellin-specific IgA (–d), which is potentially a response toward the more pro-inflammatory gut microbiome. We postulate that this might also lead to a higher IgA coating of the gut microbiome. Therefore, we labeled fecal bacteria with an antibody against IgA and sorted the IgA-coated as well as IgA-uncoated fraction. Surprisingly, we found a non-significant decrease in IgA coating after surgery, suggesting that other parts of the intestinal barrier function buffer the increase in pro-inflammatory bacteria (Table S7, ). There was no change in total fecal IgM. Fecal IgM against LPS and flagellin were barely detectable.
EnterobacteriaceaeCitation22 and StreptococcusCitation23 include several pro-inflammatory bacteria that need to be contained in the intestine to prevent infections. We sorted IgA coated bacteria and sequenced their 16s rRNA. We did not observe any significant differences between IgA-coated bacteria in ND compared to T2D fecal samples (Figure S5). Therefore, we combined both groups and used linear mixed effect models to discern taxa that were enriched in either IgA negative or positive fractions and potential effects of surgery on IgA coating (Table S9). We then observed a clear separation between IgA positive and negative fractions at both time points (Figure S5). We found 77 ASVs that were significantly enriched in either of the IgA fractions and had diverse mean relative abundance (0.003% to 3.84%, Table S9). To simplify the results, we are going to elaborate on the relative abundance and IgA coatings of the most abundant ASVs as well as bacterial groups (mean relative abundance >0.1%, ).
Figure 4. Bariatric surgery changes the IgA coating of intestinal bacteria.
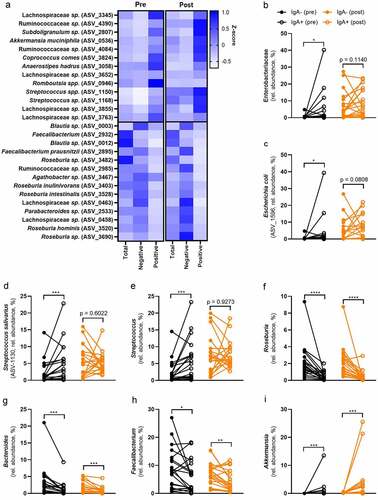
The phylum Proteobacteria was numerically enriched in the IgA-coated fraction (Figure S3C), which suggests that the majority of ASVs belonging to this phylum are IgA-coated. Of the eight significant ASVs in Proteobacteria, all were enriched in the IgA-coated fraction (p < .05). Similarly, the family Enterobacteriaceae was enriched in the IgA-positive fraction before surgery, which was surprisingly not significant after surgery (). Therefore, we can confirm results from previous studies that typical pro-inflammatory bacteria, belonging to the group of Proteobacteria as well as Enterobacteriaceae, are coated with IgA.Citation16,Citation24
As reported before, the IgA coating seems to be at least genus, species, or strain-specific.Citation24 Higher taxonomic ranks showed a diverse enrichment in either of the IgA fractions (Table S9). For example, out of the 41 ASVs belonging to the family Lachnospiraceae, 14 were enriched in the IgA coated fraction and 27 in the IgA negative fraction. In contrast, genera, such as Roseburia and Streptococcus, were significantly enriched in either of the fractions. This suggests that there are shared bacterial wall patterns or intestinal niches (e.g., close to the epithelial wall or in the mucus layer) that determine if a bacterium gets IgA coated.
ASV-1596 that includes Escherichia coli, was significantly enriched in the IgA-coated fraction before surgery, but not significant after surgery (). Similarly to the whole group of Proteobacteria, E. coli relative abundance increased after surgery (0.92% to 4.80%, Table S5) and had the highest abundance within that phylum. There was no difference between ND and T2D (Table S6). To simplify the vast number of significant findings, we will focus only on the most abundant ASVs in the total bacterial fraction (> 0.1%). The majority of significant ASVs that belong to the phylum Proteobacteria falls below that cutoff.
The genus Streptococcus includes several facultative anaerobes and opportunistic pathogens.Citation23 All five significant ASVs were enriched in the IgA-positive fraction (Table S9). ASV-1130, which includes S. salivarius, was the most abundant species of the genus in the present study. The relative abundance significantly increased from 2.35% to 4.18% after surgery (Table S5). Before surgery, S. salivarius was highly enriched in the IgA positive fraction (). After surgery, it was not significantly different, which might indicate either a more tolerant immune response toward bacteria with beneficial activities or the evasion of the immune response after surgery. Surprisingly this accounts for the whole genus Streptococcus, which was highly enriched in the IgA-positive fraction before surgery, but not differently abundant in either of the fractions after surgery ().
Roseburia is a highly abundant genus that includes several beneficial bacteria.Citation25 The mean relative abundance slightly decreased after surgery from 2.56% to 2.17% (Table S5) without any differences between ND and T2D (Table S6). Roseburia is strongly enriched in the IgA negative fraction at both time points (). All the 13 significant ASVs belonging to this genus were enriched in the IgA negative fraction. It included R. intestinalis (ASV-3528), which was highly abundant in our data set (7.81%) and has several beneficial effects on the host.Citation26
Similarly, Bacteroides includes several beneficial bacteria that are able to produce e.g., short-chain fatty acids (SCFAs). There was a non-significant reduction after surgery and no difference between the two groups (Table S5 and S6). The whole group of Bacteroides was enriched in the IgA negative fraction at both time points (). This suggests that the host displays a tolerance against beneficial bacteria.
Recently, we reported that ASVs of Faecalibacterium were enriched in IgA-positive as well as the IgA-negative fraction before antibiotic treatment.Citation24 Interestingly, in the present study all six significant ASVs of Fecalibacterium were in the IgA-negative fraction. Indeed, the whole genus was enriched in the IgA negative fraction (), supporting the notion that beneficial bacteria do not elicit an inflammatory response but an immune tolerance. The relative abundance did not change after surgery (Table S5), nor was there a difference between ND and T2D (Table S6).
Akkermansia muciniphila has several important functions for the host,Citation27 but may also induce an inflammatory response.Citation28 In line with our previous findings,Citation24 A. muciniphila was IgA-coated in the present study. The genus Akkermansia, which was mostly represented by ASV-0536 (A. muciniphila), was highly enriched in the IgA positive fraction (). This genus increased after surgery (Table S5) and did not show a difference between ND and T2D.
Discussion
In the present study, we addressed the intestinal and systemic antibody response in relation to the gut microbiome after bariatric surgery. We included severely obese individuals with or withoutT2D from the BARIA cohort.Citation9 We show that individuals with T2D have a higher inflammatory tone than non-diabetic controls, which improved after surgery. Furthermore, we found an increase in intestinal IgA and IgM against LPS as well as flagellin, which we hypothesize to be a response to the pro-inflammatory gut microbiome after surgery. Proteobacteria were particularly increased at this time point, whereas Firmicutes were decreased. Most of the typical pro-inflammatory bacteria were coated with IgA at both time points. Surprisingly, some of these bacteria seem to evade this IgA response after surgery.
It is well established that T2D individuals have a higher chronic low grade inflammatory tone than healthy controls.Citation6 Indeed, the T2D individuals in the present study had higher blood leukocytes and a numerical increase in CRP. Both decreased after surgery, but remained higher in T2D compared to ND individuals. Several factors seem to be involved in the inflammatory response in T2D. First, higher glucose levels, and other excessive nutritional factors in the blood circulation can induce an inflammatory cascade.Citation29 Glucose values improved in both groups after RYGB surgery, but were still elevated in T2D. That might explain the higher inflammatory tone before and after surgery. Second, gut-derived LPS can induce inflammation in T2D13. We did not find increased LPS levels in T2D, which might be due to the extensive matching of the present groups. LBP, as a proxy for LPS exposure, was increased numerically when comparing ND to T2D. In addition, we found a positive correlation between blood glucose concentration and serum LPS as well as flagellin. This suggests that there is a relationship between glucose values and endotoxemia. Indeed, Thaiss et al. (2018) proposed a mechanism how hyperglycemia reduces the intestinal barrier function.Citation30 Therefore, there is a strong association between inflammation, gut microbiome and hyperglycemia.Citation6
Many studies focus on macrophages and T cells in relation to inflammation in T2DCitation29, whereas the B-cell response is rather neglected. We found a numerical decrease in the B-cell response between T2D and ND. We can only speculate that reduced systemic antibody levels, which are still in a healthy range, may hint toward a less efficient immune response against pro-inflammatory bacterial components such as LPS. More research and more sensitive methods are necessary to further decipher the antibody response in T2D, particularly against gut-derived compounds.
Interestingly, the antibody response in T2D individuals after RYGB surgery differed from the response in ND. Whereas systemic IgA decreased in both groups, IgM and IgG slightly increased in T2D. If the hypothesis is true that T2D individuals have reduced antibody levels, then bariatric surgery might help to improve the antibody response to a healthier status. Indeed, there was a higher antibody production in B cell cultures ex vivo. Of note, these cultures were treated with cytokines to stimulate antibodies. In particular, IL-21, which we use in our B cell culture, dose dependently increases antibodies in vitro.Citation31 Therefore, we cannot compare ex vivo culture with total plasma antibody levels. Nevertheless, we believe that this increase further highlights a healthier immune response after surgery. However, we cannot exclude that technical artifacts may be responsible for this effect since B cells were cultured freshly and there is one year between both time points.
Next, we found that fecal LPS and flagellin increased after RYGB surgery. This increase may be attributed to an increase in Proteobacteria in the fecal microbiome as RYGB surgery induces a drastic change in the intestinal anatomy. Studies suggest that the shortened path from the stomach toward the large intestine, where a major portion of the gut microbiome resides, may introduce more oxygen.Citation19 Other factors include different delivery routes and concentrations of various bile acids,Citation32 different diet patterns,Citation32 proton pump inhibitor use after surgery, as well as a higher pH in the intestine.Citation33 One adaptation to these observed changes is a higher antibody production against the pro-inflammatory microbiome after RYGB surgery.
A previous study reported an increase in total fecal IgA one month after surgery.Citation20 In contrast, we did not find an increase in total fecal IgA, which might be due to the fact that we measured antibodies one year after surgery. Furthermore, fecal IgA is highly variable and standardized methods as well as concentrations are not clearly defined at this point in time. More research is needed to better map ‘healthy’ intestinal IgA levels and to create reference ranges as exist for systemic antibodies. Nevertheless, we found higher antibody levels against LPS and flagellin after surgery, which suggests that the intestinal barrier function is improving. Further, there was an increase in systemic IgA against LPS, similar to the intestinal IgA against LPS. Thereby, increased levels of fecal LPS and flagellin can potentially be neutralized, which aids in maintaining intestinal barrier function after RYGB surgery.Citation34–36
IgA coating of intestinal bacteria is an important tool of the host to prevent bacteria from interacting with the epithelium or even pass the epithelial wall.Citation16 Despite the increase in pro-inflammatory bacteria, we did not see an increase in IgA coated bacteria; surprisingly, there was a trend toward less IgA coating. Further, fecal lipocalin-2, as a marker for intestinal inflammation,Citation21 did not change after surgery. Other features of the intestinal barrier (e.g., mucus layer) could reduce the coating of IgA and the contact of bacteria with the epithelium. For example, a dense and thick mucus layer on top of the epithelium might prevent bacteria from being coated or inducing an immune response. It has been noted in animal studies that obese mice have a less intact mucus layer.Citation37 The mucus layer harbors major portions of IgA.Citation38 When intact, bacteria are less able to interact with the epithelium, and IgA coating is less likely. Although not tested in humans, there is a higher mucus production in animals after bariatric surgery.Citation39,Citation40 This might also explain why some bacteria, such as E. coli and S. salivarius, are enriched in the IgA coated fraction before surgery, but not significantly enriched in either of the fraction after RYGB surgery.
A. muciniphila is an important driver of a healthy intestinal mucus layer by promoting mucus production and thickness.Citation41 In the present study, we report an increase in A. muciniphila after surgery, which would support the notion of an improved mucus thickness after bariatric surgery. Interestingly, A. muciniphila is IgA coated in several studies.Citation24 This bacterium is a potent mucus degrader, which in turn enhances the mucus production. However, by degrading mucus, A. muciniphila comes close to the intestine and might induce a strong IgA response. Furthermore, its LPS structure is highly pro-inflammatory.Citation28 Therefore, this bacterium is beneficial for the host, but needs controlling to avoid inflammation. Other beneficial bacterial groups, such as Bacteroides, Roseburia, and Faecalibacterium are mostly uncoated. There might be numerous reasons: For example, the host might promote beneficial bacteria and those bacteria are not in close contact with the epithelial wall.Citation42,Citation43
We speculate that there are three drivers of IgA coating. First, proximity to the intestinal wall plays a major role in determining whether an inflammatory response is initiated (e.g. A. muciniphila). Second, bacteria in the small intestine are more coated with IgACitation44 (Figure S8), potentially due to a thinner mucus layer compared to the large intestineCitation45 (e.g., StreptococcusCitation46) and a major production of IgA in the small intestine.Citation47 Third, colonic bacteria are mainly uncoated due to the thick mucus layer and their niche in the lumen (e.g., Roseburia and FaecalibacteriumCitation46).
We need to acknowledge that our study shows several limitations. First, we show the relative abundance of bacteria; if one bacterial strain is expanding, then another one will decrease, which is not an indication for its absolute abundance. To further explore the concept of IgA coating, it would be interesting to measure the total abundance of these bacteria in either of the fractions instead of relative abundances. Second, we used TLR reporter cells that might not be very specific to small amounts of exogenous (bacterial) ligands, particularly in complex samples, such as blood or fecal samples. Thirdly, we cultured B cells at two different time points that were one year apart. Differences between time points in ex vivo cultures might therefore been subjected to technical artifacts (e.g., lot numbers, biological activity of cytokines, etc.).
In conclusion, in the present study we show that the anatomical change of intestine after RYGB surgery is associated with a shift toward a more pro-inflammatory gut microbiome. After RYGB surgery, we, however, observed a lower systemic inflammatory tone, with subtle differences between non-diabetic controls and T2D individuals. This can be explained by an improved intestinal barrier function, characterized by a compensatory IgA response, particularly against pro-inflammatory compounds such as LPS. Thereby, it prevents further systemic inflammation driven by the gut microbiome.
Methods
Data availability
The data that support the findings of this study are available in ENA at https://www.ebi.ac.uk/ena/browser/view/PRJEB48095, reference number PRJEB48095.
Study population
This work was carried out on a subsample from the BARIA cohort (Amsterdam UMC and Spaarne Gasthuis, The Netherlands).Citation9 The study protocols were approved by the Ethical Review Board of the Academic Medical Center, Amsterdam (approval code: NL55755.018.15), and all patients that have been included provided informed consent. In the present study, we included 20 obese individuals with Type 2 diabetes mellitus and 20 body-mass-index (BMI), sex and age matched controls. Individuals underwent RYGB (laparoscopic omega‐loop gastric bypass or laparoscopic sleeve gastrectomy). Samples were collected before RYGB surgery as well as a year after surgery. A 2-hour mixed meal test was performed after an overnight fast with two Nutridrinks compact 125 mL (Nutricia®) as described.Citation9
Biochemical analysis
Blood was collected in Vacutainer® tubes containing a polymer gel for serum separation (Beckton Dickinson, Franklin Lakes, NJ), centrifuged at 1550 × g (15 min, 4°C) and stored at −80°C until further analysis.
Antibody ELISAs
Total antibodies were measured in serum or fecal water according to the manufacturer’s instructions (Total IgG, IgA, and IgM Human Uncoated ELISA kit, ThermoFisher Scientific, US).
LPS and Flagellin specific antibody ELISA
Antibodies were measured according to Tran, Ley, Gewirtz, and Chassaing.Citation48 A 96-well half-area ELISA microplate (ThermoFisher Scientific, US) were coated with 50 uL flagellin (100 ng per well in 9.6 pH bicarbonate buffer; Invivogen, US) overnight at 4°C. Plates were washed 3x with 150 uL PBS with 0.05% Tween20 and were loaded with 50 uL of diluted serum samples (100x diluted in PBS) for 1 hours at 37°C. Plates were washed again and loaded with secondary antibodies for 1 h at room temperature (HRP-conjugated anti-human IgG, BD, clone G18-145, 2500x diluted in PBS with 0.05% Tween20; goat HRP-conjugated anti-human IgA, preadsorbed, abcam, polyclonal, 10.000× diluted; goat HRP-conjugated anti-human IgM, preadsorbed, polyclonal, abcam, 50.000× diluted). Plates were washed again and incubated with 50 uL TMB for 15 minutes and stopped with 50 uL 2 M H2SO4. Antibody activity was measured by reading OD450.
Flagellin and LPS detection
Flagellin and LPS were detected in serum and fecal water with the aid of HEK-blueTM TLR5 or HEK-blueTM TLR4 reporter cell line (InvivoGen, US), according to the manufacturer's instructions. For serum flagellin and LPS detection, 20 uL serum was mixed with 180 uL cell suspension.
Fecal IgA flow cytometry and sorting of IgA+ bacteria
Frozen fecal samples (100 mg) were diluted in 1:10 phosphate buffered saline (PBS) with cOmpleteTM Protease Inhibitory Cocktail (Roche, Basel, Switzerland). All steps were performed on ice. Samples were homogenized with the aid of a 5 mm stainless steel bead (Qiagen, US). Samples were centrifuged at 400 × g for 5 min to separate the bacteria from large debris. Next, 100 μL of the supernatant was taken and centrifuged for 5 min at 8000 × g to pellet the bacteria. Supernatant was saved for total antibody concentrations (fecal water). The pellet was washed with 1 mL of PBS and used for bacterial sorting as well as sequencing of total fecal bacteria.
Bacterial sorting was performed according to Palm et al (2014)Citation49 with some modifications. Fecal bacteria were prepared as described above. Bacteria were washed with 1 mL PBS containing 1% Bovine Serum Albumin (BSA, Sigma-Aldrich, US, staining buffer). Pellets were blocked in a 100 uL blocking buffer (20% normal mouse serum in staining buffer, Jackson Immuno Research, UK) for 20 min on ice and then stained with 100 uL PE-conjugated anti-human IgA in staining buffer (1:50; Miltenyi Biotec, clone IS11-8E10, Germany) for 30 min on ice. Samples were washed 3 times with 1 mL staining buffer before cell separation. Anti-IgA stained fecal bacteria were incubated in a 1 mL staining buffer containing 50 uL anti-PE magnetic activated cell sorting (MACS) beads (Miltenyi Biotec, Germany) for 15 min at 4°C. Next, the bacteria were washed twice with a 1 mL staining buffer (10.000 × g, 5 min, 4°C) and separated with an LS column on a manual separator (Miltenyi Biotec, Germany). Both positive and negative fractions were further purified with SH800 cell sorter (Sony, Japan). For each sample, 2 million bacteria were collected, pelleted (10,000 × g, 5 min, 4°C) and frozen for DNA isolation. Gating strategies can be found in supplementary Figure S6.
DNA Extraction and sequencing analysis of IgA-coated bacteria
DNA was extracted from 100 uL of homogenized fecal bacteria (see above) and the sorted fractions using a repeated bead beating protocolCitation50 (method 5). DNA was purified using Maxwell RSC Whole Blood DNA Kit. 16S rRNA gene amplicons were generated using a single-step PCR protocol targeting the V3-V4 region.Citation51 PCR products were purified using Ampure XP beads and purified products were equimolar pooled. The libraries were sequenced using a MiSeq platform using the V3 chemistry with 2 × 251 cycles.
Forward and reverse reads were truncated to 240 and 210 bases, respectively, and merged using USEARCH.Citation52 Merged reads that did not pass the Illumina chastity filter, had an expected error rate higher than 2, or were shorter than 380 bases were filtered. Amplified Sequence Variants (ASVs) were inferred for each sample individually with a minimum abundance of 4 reads.Citation53 Unfiltered reads were then mapped against the collective ASV set to determine the abundances. Taxonomy was assigned using the RDP classifierCitation54 and SILVACitation55 16S ribosomal database V132. Contaminants were identified using decontam software and subsequently, together with lab-specific known contaminants, removed from the data. Raw sequence reads were submitted to the ENA repository under study PRJEB47045.
B cell isolation and culture
Peripheral blood mononuclear cells (PBMCs) were isolated from 10 mL fresh blood with Lymphoprep density gradient (Stemcell Technologies, Canada), according to manufacturer’s instructions. B cells were enriched for CD19+ cells with magnetic beads and MS columns on a manual system (Miltenyi Biotec, Germany). CD19+ cells were stained with conjugated antibodies against CD20-BV510 (BD, US), CD27-PE (BD, US), IgG-FITC (BD, US), IgA-APC (Miltenyi Biotec, German), and sorted on a Sony SH800 sorter (Japan) in full B cell media (RPMI 1640, 5% FBS, 1x Pen/Strep, 1x L-Glutamine, 50 µM β mercaptoethanol). The gating strategy is depicted in Figure S7.
Four hours before co-culture, 24 well plates were prepared with irradiated 3t3-CD40L feeder cells (30 Gy, 40.000 wells per well, full B cell media). CD19+ CD20+ were plated separately in a 24 well plate according to their antibody isotype (IgA+, IgG+, or IgM+, 4000 cells per well). Cells were cultured for 6 days and harvested by separated cells from the supernatant (300× g, 5 minutes). Cells were lysed with 300 µL RIPA buffer (ThermoFisher, US). Samples were frozen until further use.
Statistical analysis
Data were checked for normality with the Shapiro-Wilk test. The effects of surgeryon fasting parameters were assessed using the paired t-test for normal continuous variables and the Wilcoxon signed rank test for other variables. A one-way ANOVA for repeated measures of normal continuous variables and the Friedman test for other variables, with Bonferroni post-hoc testing, were used to assess the effects of the high-fat meal on postprandial parameters.Statistical analysis was performed using GraphPad Prism version 8.0.2. Data are provided as mean with standard deviation. Microbiome data were analyzed and visualized in RCitation56 (V3.6.3). Permutation ANOVA from VeganCitation57 was used to test differences in composition. Multilevel PCA was performed, on clr transformed data, using the mixOmicsCitation58 package, statistical significance was tested using a permutation manova on the first 10 components. The effects of sorting on specific taxa were tested with linear mixed effect models using lme4.Citation59 P-values were corrected for multiple testing (FDR), were applicable, and P-values <0.05 were considered statistically significant. All authors had access to the study data and reviewed and approved the final manuscript.
Author contributions
TPMS performed the experiments, analysis, and writing of the manuscript. SM, MB, AL, and OA conducted the clinical study. MW, XV and SK assisted with experiments and analysis. MD analyzed the sequencing results. MN and DHR reviewed the manuscript. HH supervised and reviewed the manuscript.
Supplemental Material
Download MS Excel (136 KB)Acknowledgments
DHvR was supported by a fellowship of the Dutch Diabetes Research Foundation and the EU Marie Curie Program. The BARIA study is funded by the Novo Nordisk Foundation (NNF15OC0016798). The BARIA study is a Scandinavian‐Dutch collaboration. HH is supported by a Senior Fellowship of the Dutch Diabetes Research Foundation (2019.82.004). MN was supported by a ZONMW VIDI grant 2013 [016.146.327]. We thank Fredrik Bäckhed for his support.
Disclosure statement
No potential conflict of interest was reported by the author(s).
Supplementary material
Supplemental data for this article can be accessed on the publisher’s website.
Additional information
Funding
References
- Blüher M. Obesity: global epidemiology and pathogenesis. Nat Rev Endocrinol. 2019;15(5):288–15. doi:10.1038/s41574-019-0176-8.
- Saeedi P, Petersohn I, Salpea P, Malanda B, Karuranga S, Unwin N, Colagiuri S, Guariguata L, Motala AA, Ogurtsova K, et al. Global and regional diabetes prevalence estimates for 2019 and projections for 2030 and 2045: results from the international diabetes federation diabetes Atlas, 9(th) edition. Diabetes Res Clin Pract. 2019;157:107843. doi:10.1016/j.diabres.2019.107843.
- Puzziferri N, Roshek TB III, Mayo HG, Gallagher R, Belle SH, Livingston EH. Long-term follow-up after bariatric surgery: a systematic review. JAMA. 2014;312(9):934–942. doi:10.1001/jama.2014.10706.
- Nguyen NT, Varela JE. Bariatric surgery for obesity and metabolic disorders: state of the art. Nat Rev Gastroenterol Hepatol. 2017;14(3):160–169. doi:10.1038/nrgastro.2016.170.
- Aron-Wisnewsky J, Prifti E, Belda E, Ichou F, Kayser BD, Dao MC, Verger EO, Hedjazi L, Bouillot J-L, Chevallier J-M, et al. Major microbiota dysbiosis in severe obesity: fate after bariatric surgery. Gut. 2019;68(1):70–82. doi:10.1136/gutjnl-2018-316103.
- Scheithauer TPM, Rampanelli E, Nieuwdorp M, Vallance BA, Verchere CB, van Raalte DH, Herrema H. Gut microbiota as a trigger for metabolic inflammation in obesity and Type 2 diabetes. Front Immunol. 2020;11:571731. doi:10.3389/fimmu.2020.571731.
- Cani PD, Amar J, Iglesias MA, Poggi M, Knauf C, Bastelica D, Neyrinck AM, Fava F, Tuohy KM, Chabo C, et al. Metabolic endotoxemia initiates obesity and insulin resistance. Diabetes. 2007;56(7):1761–1772. doi:10.2337/db06-1491.
- Tran HQ, Ley RE, Gewirtz AT, Chassaing B. Flagellin-elicited adaptive immunity suppresses flagellated microbiota and vaccinates against chronic inflammatory diseases. Nat Commun. 2019;10(1):5650. doi:10.1038/s41467-019-13538-y.
- Van Olden CC, Van de Laar AW, Meijnikman AS, Aydin O, Van Olst N, Hoozemans JB, De Brauw LM, Bruin SC, Acherman YIZ, Verheij J, et al. A systems biology approach to understand gut microbiota and host metabolism in morbid obesity: design of the BARIA longitudinal cohort study. J Intern Med. 2021;289(3):340–354. doi:10.1111/joim.13157.
- Amar J, Chabo C, Waget A, Klopp P, Vachoux C, Bermúdez-Humarán LG, Smirnova N, Bergé M, Sulpice T, Lahtinen S, et al. Intestinal mucosal adherence and translocation of commensal bacteria at the early onset of type 2 diabetes: molecular mechanisms and probiotic treatment. EMBO Mol Med. 2011;3(9):559–572. doi:10.1002/emmm.201100159.
- Xiao X, Yeoh BS, Vijay-Kumar M. Lipocalin 2: an emerging player in iron homeostasis and inflammation. Annu Rev Nutr. 2017;37(1):103–130. doi:10.1146/annurev-nutr-071816-064559.
- Thingholm LB, Rühlemann MC, Koch M, Fuqua B, Laucke G, Boehm R, Bang C, Franzosa EA, Hübenthal M, Rahnavard A, et al. Obese individuals with and without Type 2 diabetes show different gut microbial functional capacity and composition. Cell Host Microbe. 2019;26(2):252–64.e10. doi:10.1016/j.chom.2019.07.004.
- Gomes JMG, Costa JDA, Alfenas Rd CG. Metabolic endotoxemia and diabetes mellitus: a systematic review. Metabolism. 2017;68:133–144. doi:10.1016/j.metabol.2016.12.009.
- Ellulu MS, Patimah I, Khaza’ai H, Rahmat A, Abed Y. Obesity and inflammation: the linking mechanism and the complications. Arch Med Sci. 2017;4:851–863. doi:10.5114/aoms.2016.58928.
- Boutagy NE, McMillan RP, Frisard MI, Hulver MW. Metabolic endotoxemia with obesity: is it real and is it relevant? Biochimie. 2016;124:11–20. doi:10.1016/j.biochi.2015.06.020.
- Bunker JJ, Bendelac A. IgA responses to microbiota. Immunity. 2018;49(2):211–224. doi:10.1016/j.immuni.2018.08.011.
- Kaci G, Goudercourt D, Dennin V, Pot B, Doré J, Ehrlich SD, Renault P, Blottière HM, Daniel C, Delorme C, et al. Anti-inflammatory properties of Streptococcus salivarius, a commensal bacterium of the oral cavity and digestive tract. Appl Environ Microbiol. 2014;80(3):928–934. doi:10.1128/AEM.03133-13.
- Vesty A, Gear K, Boutell S, Taylor MW, Douglas RG, Biswas K. Randomised, double-blind, placebo-controlled trial of oral probiotic Streptococcus salivarius M18 on head and neck cancer patients post-radiotherapy: a pilot study. Sci Rep. 2020;10(1):13201. doi:10.1038/s41598-020-70024-y.
- Celiker H. A new proposed mechanism of action for gastric bypass surgery: air hypothesis. Med Hypotheses. 2017;107:81–89. doi:10.1016/j.mehy.2017.08.012.
- Luck H, Khan S, Kim JH, Copeland JK, Revelo XS, Tsai S, Chakraborty M, Cheng K, Tao Chan Y, Nøhr MK, et al. Gut-associated IgA+ immune cells regulate obesity-related insulin resistance. Nat Commun. 2019;10(1):3650. doi:10.1038/s41467-019-11370-y.
- Chassaing B, Srinivasan G, Delgado MA, Young AN, Gewirtz AT, Vijay-Kumar M. Fecal lipocalin 2, a sensitive and broadly dynamic non-invasive biomarker for intestinal inflammation. PLoS One. 2012;7(9):e44328. doi:10.1371/journal.pone.0044328.
- Sanders WE, Sanders CC. Enterobacter spp.: pathogens poised to flourish at the turn of the century. Clin Microbiol Rev. 1997;10(2):220–241. doi:10.1128/CMR.10.2.220.
- Krzyściak W, Pluskwa KK, Jurczak A, Kościelniak D. The pathogenicity of the Streptococcus genus. Eur J Clin Microbiol Infect Dis. 2013;32(11):1361–1376. doi:10.1007/s10096-013-1914-9.
- Scheithauer TPM, Bakker GJ, Winkelmeijer M, Davids M, Nieuwdorp M, van Raalte DH, Herrema H. Compensatory intestinal immunoglobulin response after vancomycin treatment in humans. Gut Microbes. 2021;13(1):1–14. doi:10.1080/19490976.2021.1875109.
- Ryan KK, Tremaroli V, Clemmensen C, Kovatcheva-Datchary P, Myronovych A, Karns R, Wilson-Pérez HE, Sandoval DA, Kohli R, Bäckhed F, et al. FXR is a molecular target for the effects of vertical sleeve gastrectomy. Nature. 2014;509(7499):183–188. doi:10.1038/nature13135.
- La Rosa SL, Leth ML, Michalak L, Hansen ME, Pudlo NA, Glowacki R, Pereira G, Workman CT, Arntzen MØ, Pope PB, et al. The human gut Firmicute Roseburia intestinalis is a primary degrader of dietary β-mannans. Nat Commun. 2019;10(1):905. doi:10.1038/s41467-019-08812-y.
- Cani PD, de Vos WM, Chen S, Zeng Z. Next-Generation beneficial microbes: the case of Akkermansia muciniphila. Front Microbiol. 2017;8:8. doi:10.3389/fmicb.2017.00008.
- Vatanen T, Kostic AD, d’Hennezel E, Siljander H, Franzosa EA, Yassour M, Kolde R, Vlamakis H, Arthur T, Hämäläinen A-M, et al. Variation in microbiome LPS immunogenicity contributes to autoimmunity in humans. Cell. 2016;165(4):842–853. doi:10.1016/j.cell.2016.04.007.
- Donath MY, Shoelson SE. Type 2 diabetes as an inflammatory disease. Nat Rev Immunol. 2011;11(2):98–107. doi:10.1038/nri2925.
- Thaiss CA, Levy M, Grosheva I, Zheng D, Soffer E, Blacher E, Braverman S, Tengeler AC, Barak O, Elazar M, et al. Hyperglycemia drives intestinal barrier dysfunction and risk for enteric infection. Science. 2018;359(6382):1376. doi:10.1126/science.aar3318.
- Kuchen S, Robbins R, Sims GP, Sheng C, Phillips TM, Lipsky PE, Ettinger R. Essential role of IL-21 in B cell activation, expansion, and plasma cell generation during CD4+ T Cell-B cell collaboration. The Journal of Immunology. 2007;179(9):5886. doi:10.4049/jimmunol.179.9.5886.
- Ilhan ZE, DiBaise JK, Dautel SE, Isern NG, Kim Y-M, Hoyt DW, Schepmoes AA, Brewer HM, Weitz KK, Metz TO, et al. Temporospatial shifts in the human gut microbiome and metabolome after gastric bypass surgery. Npj Biofilms and Microbiomes. 2020;6(1):12. doi:10.1038/s41522-020-0122-5.
- Ulker İ, Yildiran H. The effects of bariatric surgery on gut microbiota in patients with obesity: a review of the literature. Biosci Microbiota Food Health. 2019;38(1):3–9. doi:10.12938/bmfh.18-018.
- Wilbrink J, Bernards N, Mujagic Z, van Avesaat M, Pijls K, Klaassen T, van Eijk H, Nienhuijs S, Stronkhorst A, Wilms E, et al. Intestinal barrier function in morbid obesity: results of a prospective study on the effect of sleeve gastrectomy. Int J Obes (Lond). 2020;44(2):368–376. doi:10.1038/s41366-019-0492-z.
- Yang PJ, Yang WS, Nien HC, Chen CN, Lee PH, Yu LC, Lin M-T. Duodenojejunal bypass leads to altered gut microbiota and strengthened epithelial barriers in rats. Obes Surg. 2016;26(7):1576–1583. doi:10.1007/s11695-015-1968-0.
- Casselbrant A, Elias E, Fändriks L, Wallenius V. Expression of tight-junction proteins in human proximal small intestinal mucosa before and after Roux-en-Y gastric bypass surgery. Surg Obes Relat Dis. 2015;11(1):45–53. doi:10.1016/j.soard.2014.05.009.
- Schroeder BO, Birchenough GMH, Pradhan M, Nyström EEL, Henricsson M, Hansson GC, Bäckhed F. Obesity-associated microbiota contributes to mucus layer defects in genetically obese mice. J Biol Chem. 2020;295(46):15712–15726. doi:10.1074/jbc.RA120.015771.
- Rogier EW, Frantz AL, Bruno MEC, Kaetzel CS. Secretory IgA is concentrated in the outer layer of colonic mucus along with gut bacteria. Pathogens. 2014;3(2):390–403. doi:10.3390/pathogens3020390.
- Olubuyide IO, Williamson RC, Bristol JB, Read AE. Goblet cell hyperplasia is a feature of the adaptive response to jejunoileal bypass in rats. Gut. 1984;25(1):62–68. doi:10.1136/gut.25.1.62.
- Akamatsu FE, Fontes LG, Itezerote AM, Saleh S, Teodoro WPR, Artifon E, Hojaij F, Andrade M, Pereira JA, Martinez CAR, et al. Is the colon mucosa affected by ten days of gastric restriction in an animal model? Acta Cir Bras. 2019;34(6):e201900610–e. doi:10.1590/s0102-865020190060000010.
- Paone P, Cani PD. Mucus barrier, mucins and gut microbiota: the expected slimy partners? Gut. 2020;69(12):2232–2243. doi:10.1136/gutjnl-2020-322260.
- Yasuda K, Oh K, Ren B, Tickle TL, Franzosa EA, Wachtman LM, Miller A, Westmoreland S, Mansfield K, Vallender E, et al. Biogeography of the intestinal mucosal and lumenal microbiome in the rhesus macaque. Cell Host Microbe. 2015;17(3):385–391. doi:10.1016/j.chom.2015.01.015.
- Donaldson GP, Lee SM, Mazmanian SK. Gut biogeography of the bacterial microbiota. Nat Rev Microbiol. 2016;14(1):20–32. doi:10.1038/nrmicro3552.
- Bunker JJ, Flynn TM, Koval JC, Shaw DG, Meisel M, McDonald BD, Ishizuka I, Dent A, Wilson P, Jabri B, et al. Innate and adaptive humoral responses coat distinct commensal bacteria with immunoglobulin A. Immunity. 2015;43(3):541–553. doi:10.1016/j.immuni.2015.08.007.
- Johansson MEV, Hansson GC. Immunological aspects of intestinal mucus and mucins. Nat Rev Immunol. 2016;16(10):639–649. doi:10.1038/nri.2016.88.
- Ruan W, Engevik MA, Spinler JK, Versalovic J. Healthy human gastrointestinal microbiome: composition and function after a decade of exploration. Dig Dis Sci. 2020;65(3):695–705. doi:10.1007/s10620-020-06118-4.
- Pabst O, Slack E. IgA and the intestinal microbiota: the importance of being specific. Mucosal Immunol. 2020;13(1):12–21. doi:10.1038/s41385-019-0227-4.
- Tran HQ, Ley RE, Gewirtz AT, Chassaing B. Flagellin-elicited adaptive immunity suppresses flagellated microbiota and vaccinates against chronic inflammatory diseases. Nat Commun. 2019;10:5650.
- Palm NW, de Zoete MR, Cullen TW, Barry NA, Stefanowski J, Hao L, Degnan P, Hu J, Peter I, Zhang W, et al. Immunoglobulin A coating identifies colitogenic bacteria in inflammatory bowel disease. Cell. 2014;158(5):1000–1010. doi:10.1016/j.cell.2014.08.006.
- Costea PI, Zeller G, Sunagawa S, Pelletier E, Alberti A, Levenez F, Tramontano M, Driessen M, Hercog R, Jung F-E, et al. Towards standards for human fecal sample processing in metagenomic studies. Nat Biotechnol. 2017;35(11):1069–1076. doi:10.1038/nbt.3960.
- Kozich JJ, Westcott SL, Baxter NT, Highlander SK, Schloss PD. Development of a dual-index sequencing strategy and curation pipeline for analyzing amplicon sequence data on the MiSeq Illumina sequencing platform. Appl Environ Microbiol. 2013;79(17):5112–5120. doi:10.1128/AEM.01043-13.
- Edgar RC. Search and clustering orders of magnitude faster than BLAST. Bioinformatics (Oxford, England). 2010;26(19):2460–2461. doi:10.1093/bioinformatics/btq461.
- Edgar RC. UNOISE2: improved error-correction for Illumina 16S and ITS amplicon sequencing. bioRxiv 2016:081257.
- Wang Q, Garrity GM, Tiedje JM, Cole JR. Naïve bayesian classifier for rapid assignment of rRNA sequences into the new bacterial taxonomy. Appl Environ Microbiol. 2007;73(16):5261. doi:10.1128/AEM.00062-07.
- Quast C, Pruesse E, Yilmaz P, Gerken J, Schweer T, Yarza P, Peplies J, Glöckner FO. The SILVA ribosomal RNA gene database project: improved data processing and web-based tools. Nucleic Acids Res. 2013;41(D1):D590–D6. doi:10.1093/nar/gks1219.
- Team R. A language and environment for statistical computing. Computing. 2013;3(1) : ;201.
- Oksanen J, Blanchet FG, Kindt R, Legendre P, Minchin P, O’Hara B, Simpson GL, Solymos P, Stevens MHH, Szoecs E, et al. Vegan: community ecology package. R Package Version 22-1. 2015;2:1–2.
- Rohart F, Gautier B, Singh A, Lê Cao K-A. mixOmics: an R package for ‘omics feature selection and multiple data integration. PLoS Comput Biol. 2017;13(11):e1005752. doi:10.1371/journal.pcbi.1005752.
- McMurdie PJ, Holmes S. phyloseq: an R package for reproducible interactive analysis and graphics of microbiome census data. PLOS ONE. 2013;8:e61217.