ABSTRACT
Quorum sensing is a molecular signaling-based communication mechanism in prokaryotes. In the basic mode, signaling molecules released by certain bacteria are sensed by intracellular receptors or membrane-bound receptors of other members in the community, leading to the collective isogenic signaling molecule synthesis and synchronized activities. This regulation is important for the symbiosis of the bacterium with the host, as well as virulence and biofilm formation. Notably, quorum sensing signaling molecules are not only able to control microbial community behavior but can likewise regulate the physiological status of host cells. Here, we provide a comprehensive review of the importance of quorum sensing signaling molecules in gram-negative bacteria in regulating host cell function and gut health, and suggest possible opportunities for application in combating human and animal diseases by blocking the pathways through which quorum sensing signaling molecules exert their functions.
Introduction
The international academic community calls a system in which multiple microorganisms live together a microbial community, also known as the microflora. However, many years ago, researchers discovered that bacteria communicate with each other, and through communication, they gain the ability to coordinate their operations.Citation1, Citation2 Bacterial communication mainly relies on microbial quorum sensing (QS). The concept of QS, first proposed by Fuqua et al., refers to the sensing phenomenon that can only occur when the number of bacteria reaches a certain density.Citation3 It was reported that QS is present in both gram-negative and gram-positive bacteria, and elevated local concentrations of QS signaling molecules (QSSMs) are often observed in bacterial infections.Citation4 QS has now become a major research area in the field of microbiology. In the 1960s, Tomasz et al. discovered the first QSSM in Streptococcus pneumoniae.Citation5 A subsequent study has shown that the QS system is widely present in various microbial populations.Citation6,Citation7 With new research developments, investigators have found that QSSM can not only regulate the group behavior of microorganisms, but also regulate the expression of virulence factors in human pathogenic bacteria, which has gradually attracted attention in the field of public health.
Bacterial QS, also known as self-induction, is a mechanism by which bacteria exchange intracellular or intercellular information, coordinate population behavior, and regulate gene expression, all of which depend on population density.Citation8 When a bacterial community reaches a certain density, it turns on the expression of genes related to bacterial community density by secreting diffusible signaling small molecules (also known as QSSMs). QSSMs diffuse into the environment, and when the signaling molecules in the environment reach a certain threshold concentration, they induce the expression of specific genes in bacteria that are dependent on cell density, thus causing bacteria to exhibit new behavioral characteristics on a community scale such as biological luminescence, regulation of virulence factor secretion, budding spore formation or biofilm formation, cell differentiation, motility, and extracellular polysaccharide formation.Citation9,Citation10 A variety of microbial-related QSSMs have been identified. For gram-negative bacteria, most QSSMs belong to the N-acyl-homoserine lactone (AHL) family. The main differences among different AHLs are the length of the N-side chain, the substituents at the 3-carbon position, and the presence or absence of one or more unsaturated bonds in the side chain.Citation11,Citation12
Previous studies on QS have mostly focused on the interactions between microorganisms. In recent years, the direct effects of QSSMs on host cell functions have also attracted widespread attention.Citation13–15 QSSMs are lipid-soluble small molecules that can easily penetrate into cells to affect cellular functions.Citation16 Here, we summarize the current knowledge on microbial QS from national and international studies. Based on recent advances in the understanding of QS, we also highlight the importance of the reciprocal effects between QSSM AHL and host cells, including the effects and potential mechanisms of AHL in mammalian host cells, the relationship between AHL and intestinal health, and the mechanism of intestinal barrier dysfunction by AHL. Multiple targets of microbial QSSM may provide new possibilities for the diagnosis and treatment of bacterial infectious diseases.
Characteristics of gram-negative bacterial QSSM
i) Small molecular weight: Gram-negative bacterial QSSM, such as AHL and its derivatives, oligopeptides, and γ-butyrolactones, are all small molecules that can freely enter and exit the cell or be secreted into the environment by oligopeptide permease and accumulate in the environment. ii) Species specificity: AHL in gram-negative bacteria has extraordinary specificity. Typically, gram-negative bacteria use LuxR-type receptors, a cytoplasmic transcription factor that detects AHLs produced by the chaperone LuxI-type synthases. LuxR proteins use amino acid variation and flexibility in the binding pocket to achieve AHL binding specificity.Citation7 iii) Dependence on growth period and bacterial density: Generally, gram-negative bacterial QSSM accumulation in the environment reaches a higher concentration during the logarithmic or stable phase of bacterial growth, when it regulates the expression of the most genes. Moreover, the supernatant from a stable phase of bacterial growth can induce changes in the physiological state of bacteria during the incubation phase (lower bacterial density). iv) Regulatory role in gram-negative bacterial infection: Many gram-negative bacterial QSSM-producing bacteria belong to the family of plant and animal pathogenic or symbiotic bacteria, which play important regulatory roles in the interactions between bacteria and hosts.
Gram-negative bacterial QS system
Gram-negative bacteria can release a class of small molecule signaling factors (AHL) into the surrounding environment that can move freely in and out of bacteria, and AHL plays a key role in the QS system.Citation7,Citation17 An informative table of different gram-negative bacterial QSSMs and function was shown in .
Table 1. Informative table of different gram-negative bacterial QSSMs and function
AHL was first discovered in the bioluminescence system of the marine bacterium Vibrio fischeri, and this phenomenon is positively correlated with the population density of the bacteria.Citation18 For gram-negative bacteria, the QS system consisting of LuxI/LuxR is the most extensively studied. Taking Vibrio fischeri as an example, the LuxI homologous gene of the bacterium is responsible for the synthesis of QSSM AHL, whereas the LuxR homologue is activated as the AHL receptor to regulate the transcription of diverse downstream genes.Citation30,Citation31 Inter-microbial signaling mediated by AHL can regulate many functions of gram-negative bacteria such as the production of virulence factors, biosynthesis of antibiotics and extracellular polysaccharides, cell clustering, and entry into the stable growth phase. During the exponential growth of bacteria, AHL is synthesized in the cytoplasm and diffuses through the cytomembrane, thereby reaching the outside of bacteria and accumulating in the environment. When AHL reaches a specific critical concentration, LuxR binds to AHL to form the LuxR-AHL complex, and this complex can activate the promoter of related genes and eventually initiate the transcription of target genes Citation32 (). LuxR stabilizes its structure by using AHL as a folding switch and degrades automatically when AHL is absent.Citation33 Many bacteria with QS can produce more than one type of AHL molecule. For example, Pseudomonas aeruginosa can produce both C4-HSL and 3-oxo-C12-HSL, and the functions and mechanisms of different types of AHL molecules can vary in their regulation of the population behavior of microorganisms.Citation34 Furthermore, several investigators have discovered that some bacteria lack genes that have functions similar to that of LuxI, rendering the bacteria unable to synthesize AHL by themselves. Interestingly, these bacteria encode LuxR<apos;>s homologous protein, SdiA,Citation35 which can sense various population signals produced by other bacteria and regulate the expression of its own QS-related genes through an “eavesdropping” mechanism.Citation36
Figure 1. Quorum sensing signaling in gram-negative bacteria. (A) N-acyl-homoserine lactones (AHLs) are QSSM that communicate with each other in gram-negative bacteria. LasI/RhlI directs LuxI to synthesize AHLs, which diffuse freely outside the bacterial membrane and bind to their receptor LuxR when AHLs accumulate to a threshold concentration. The LuxR-AHLs complex activates the expression of target genes, triggering the occurrence of toxins, biofilms, antibiotics, or fluorescence. (B) Chemical structure of a typical AHL: N-3-oxododecanoyl-homoserine lactone (3-oxo-C12-HSL).
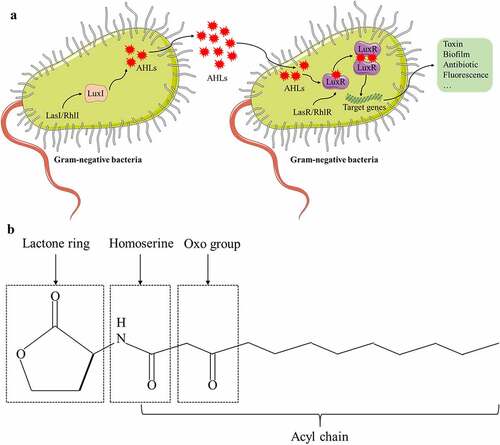
In the QS system of gram-negative bacteria with AHL as the QSSM, the signal transduction pathway is diverse. Presently, Pseudomonas aeruginosa is the most mature, and it mainly contains four sets of QS systems. The first set is the lasR/lasI system, consisting of the transcriptional activator lasR and the acetylhomoserine lactone synthase lasI protein.Citation37 LasI directs the synthesis of QSSM N-3-oxododecanoyl-homoserine lactone (3-oxo-C12-HSL) and secretes it into the extracellular compartment during active transport. It can bind lasR at a certain threshold concentration and activate gene transcription of virulence factors, including alkaline protease, exotoxin A, and elastase, which can increase the expression of virulence genes in Pseudomonas aeruginosa. The second set is the rhlR/rhlI system.Citation38 RhlR is a transcriptional regulator. RhlI encodes AHL synthetase. The system produces homoserine lactone QSSM with a structure of C4-HSL, which can freely pass through the cell membrane and regulate the expression of a large number of genes, such as chitinase, cyanide, pyocyanin, and other genes. The Pseusomonas quinolone signal (PQS) is the third QS system for Pseudomonas aeruginosa recently discovered.Citation39,Citation40 The signal molecule of the quinolone signal system has antibacterial activity and is insoluble in water. The mechanism by which it uses inter-bacterial signal transduction is still unclear. It may involve the transmission of PQS signals between bacteria through an “exocytosis”-like transport mechanism. PQS can connect the two systems of lasR/lasI and rhlR/rhlI. On the one hand, lasR/lasI and rhlR/rhlI control the generation of PQS. On the other hand, PQS affects the gene expression of lasR/lasI and rhlR/rhlI. There is a delicate balance between the two. Furthermore, PQS plays a role in regulating bacterial density and releasing virulence factors. In addition to the above three QS systems, another Pseudomonas aeruginosa QS helper system, the GacS/GacA system, has been recently discovered and reported to play important roles in improving the ability of bacteria to travel, releasing theobromine sodium acetate, and promoting biofilm formation.Citation41
Effect of QSSM AHL on host cells and potential mechanisms
Impact of AHL on cell apoptosis
QSSMs are a class of small molecules that are lipid soluble. They can reach mucosal epithelia or subcutaneous tissues by diffusion, even diffusing into the vasculature.Citation42 It has been shown that AHL, the QSSM of gram-negative bacteria, can affect the function of host eukaryotic cells. Due to their lipid solubility, AHL can rapidly enter mammalian cells and induce apoptosis. As such, AHL plays a direct role in inducing apoptosis in host cells. AHLs can quickly initiate apoptosis of mouse embryonic fibroblasts, activate caspases-3/7 and 8, depolarize the mitochondrial membrane potential, and release cytochrome c from mitochondria into the cytoplasm, leading to cell and nuclear shrinkage, and ultimately cell death.Citation43 Exposure of the respiratory epithelium to AHLs for only 1 hour results in the disintegration of tight junctions between epithelial cells. However, a membrane-penetrating pan-caspase inhibitor (Z-VAD-FMK) has been reported to block this damage, suggesting that the breakdown of tight junctions is an early event in apoptosis that is initiated by AHL.Citation44 Low concentrations of AHL were sufficient to reduce the viability of undifferentiated Caco-2 cells and induce apoptosis by inhibiting AKT phosphorylation, whereas AKT overexpression partially reversed apoptosis.Citation45 Furthermore, mucus protein mucin 3 (MUC3) protects against damage caused by AHL in epithelial cells.Citation46 The pro-apoptotic effect of AHL is related to intracellular Ca2+ signaling. The pretreatment of cells with thapsigargin, an inhibitor of Ca2+ uptake into the endoplasmic reticulum, for 10 min can protect the barrier from damage by AHL and reduce apoptosis.Citation44 AHL strongly induced apoptosis of macrophages and neutrophils.Citation47–50 Furthermore, AHL promoted sperm apoptosis in a dose-dependent manner, thus reducing their motility.Citation51 AHL also has a strong pro-apoptotic effect on cancer cells.Citation52
Impact of AHL on immunity
Depending on the acyl chain length, double bonds, and concentration, AHL can affect the host innate immune system to varying degrees. For macrophages, in general, AHL decreases their inflammatory response in a diverse manner, leading to chronic infection with Pseudomonas aeruginosa. It has been shown that Pseudomonas aeruginosa-produced AHL exerted an anti-inflammatory response in RAW264.7 mouse macrophages in a dose-dependent manner. At the same time, AHL induced a decrease in the concentration of TNF-α and an increase in the concentration of IL-10 secreted by RAW264.7 cells.Citation53 In addition, it has been reported that macrophages have stronger phagocytic activity in the presence of AHL.Citation54 For dendritic cells (DCs), the effects are different and depend on the cell type. In the presence of AHL, stimulation with lipopolysaccharides inhibited the secretion of pro-inflammatory cytokines IL-12 and interferon-γ in both human and mouse DCs. However, the same concentrations of AHL and lipopolysaccharides only enhanced the ability of human DCs, but not mouse DCs, to secrete IL-10.Citation55–57 AHL also has a certain degree of influence on host adaptive immunity. AHL can inhibit mitogen-stimulated Citation58 and antigen-stimulated Citation59 T lymphocyte proliferation and function, as well as regulate antibody production by B lymphocytes.Citation60 Furthermore, AHL can induce apoptosis in Jurkat cell lines via the mitochondrial pathway,Citation61 thus inhibiting the activation and proliferation of DCs and T cells and downregulating the expression of costimulatory molecules on DCs.Citation62,Citation63
Cell-type specific and dose-specific by which AHL affects host cell function
The induction of apoptosis in mammalian cells by AHL is distinctly cell specific. For example, numerous in vitro studies have shown that AHL can induce apoptosis in tracheal epithelial cells, breast cancer cells, macrophages, and neutrophils, but not in liver Hep2 and lung CCL185 epithelial cell lines.Citation64–68 The regulation of immunity in mammalian cells by AHL is also cell type specific, with some studies reporting that AHLs can upregulate inflammatory cytokines,Citation69–71 whereas other studies demonstrating that AHLs can reduce inflammation in cells.Citation60,Citation72–75 In addition, the effects of AHL on host cell function are similarly dose-specific. At higher concentrations (> 25 μM), intracellular events may predominate, but at lower concentrations (< 10 μM), more sensitive receptor-driven effects will predominate.Citation76 Relatively low concentrations (between 10 to 30 μM) of AHL can reduce cell viability by inhibiting AKT phosphorylation in undifferentiated Caco-2 cells accompanied by apoptosis.Citation77 The gastrointestinal tract is considered the most important reservoir of AHL-producing Pseudomonas aeruginosa in clinical settings, which is associated with higher mortality rates of patients. Human clinical study has demonstrated the ability of Pseudomonas aeruginosa to adhere to and penetrate intestinal epithelial cells as well as to form biofilms.Citation78 The AHL is present in Pseudomonas aeruginosa biofilms in concentrations as high as 300–600 µM.Citation79 Thus, the concentrations used in the studies of AHL affecting host cell function that we listed previously are of physiological significance, and these studies have contributed to guiding clinical practice.
Potential mechanisms by which AHL affects host cell function
Although the molecular mechanisms by which AHLs trigger host cell damage have not been identified and research on the targets for the identified receptors of AHL in mammalian cells is still at an early stage, several signaling pathways and mechanisms mediating the activity of AHL have been elucidated. Typically, the host activates the innate immune system through pattern recognition receptors (PPRs) when pathogenic microorganisms and their metabolites invade. As one type of PRR, Toll-like receptors (TLRs) are capable of directly activating an immune response.Citation80 AHL has been reported to modulate neutrophil phagocytosis Citation64,Citation81 and inhibit DC antigen presentation,Citation56 thus inducing pro-inflammatory cytokines and exacerbating airway inflammation.Citation53,Citation82 AHL has also been reported to counteract lipopolysaccharide-induced nuclear factor-κB (NF-κB) activation,Citation83 thus triggering lymphocyte death.Citation61 It has been shown that TLRs are not necessary for the host response to AHL.Citation84 In a previous study, we found that NF-κB phosphorylation did not change despite altered expression of TLRs after AHL treatment. Furthermore, inhibitors of NF-κB transcriptional activity did not alleviate AHL-induced oxidative stress and cell viability. These results indicate that the TLR–NF-κB signaling pathway is not involved in AHL-induced host cell damage.Citation85 Thus, the effector functions of AHL appear to occur through a unique signaling platform.
The lipid raft is a micro-domain on the plasma membrane that is rich in cholesterol and sphingomyelin. The lipid raft is like a platform for protein parking that is closely related to membrane signal transduction and protein sorting. Lipid rafts may initially form in the endoplasmic reticulum, and some lipid rafts can be cross-linked with submembrane cytoskeletal proteins to varying degrees after being transported to the cell membrane.Citation86–89 Presently, there is no consensus on whether lipid rafts mediate the harmful biological functions of AHLs on the host. Because AHL is a kind of lipid-soluble small molecule, it has been reported that cholesterol on the cell membrane is a potential receptor for AHL, and AHL can be transported into the cell through lipid rafts on the cell membrane to perform specific biological functions. The destroyer of lipid rafts, MβCD, disrupts the structure of lipid rafts by removing cholesterol from the cell membrane. It can effectively inhibit the increase in the permeability of Caco-2 cells induced by AHL.Citation90 However, another report indicates that AHL can enter the host cell through a passive transport pathway and has little interaction with the cell membrane.Citation42 The reason for this discrepancy in findings may be due to the different cell types that were used, but the relationship between lipid rafts and AHLs still needs further research ().
Figure 2. Proposed model of the potential mechanism of N-acyl-homoserine lactones (AHLs) mediating the biological effects in host cells. The lipid raft and tumor necrosis factor receptor (TNFR1) located on the cell membrane, as well as paraoxonase 2 (PON2) and peroxisome proliferator-activated receptor-γ (PPAR-γ) located in the cytoplasm, are potential receptors of AHLs, which may collectively induce apoptosis and the inflammatory response in host cells with AHLs.
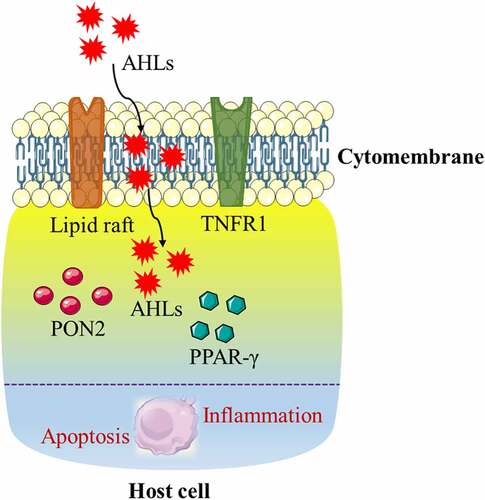
Currently, the mechanism by which AHLs regulate host cell function is unclear, and the receptors for host cell acceptance of AHLs have not been identified.Citation91 However, it has been proposed that peroxisome proliferator-activated receptor-γ (PPAR-γ) may serve as a potential receptor for mammalian AHL. PPAR-γ is a nuclear receptor that acts as a transcription factor to regulate lipid metabolism. Previous studies on PPAR-γ have focused on the regulation of adipocyte differentiation, whereas few studies have addressed its mediation of apoptosis. In vitro studies have also confirmed that PPAR-γ agonists can induce apoptosis in cultured T cells in vitro, suggesting that it is a potential anti-inflammatory molecule that plays a role in immune dysfunction during a severe inflammatory response.Citation92 An increase in PPAR-γ expression can also induce apoptosis in cancer cells.Citation93 However, the opposite is true for neuronal cells, where either PPAR-γ overexpression or its receptor agonist rosiglitazone can exert an anti-apoptotic effect by protecting mitochondria through the upregulation of anti-apoptotic proteins Citation94 ().
Paraoxonase 2 (PON2) is widely expressed in mammalian tissues, and its main function is to hydrolyze QSSM AHL. Therefore, PON2 may be a potential regulator of AHL to exert its biological functions. Presently, there is no agreement on the role of PON2 in AHL in regulating host cell function. Studies have shown that PON2 has antioxidative, anti-inflammatory, and anti-apoptotic properties in human aortic endothelial cells, depending on its hydrolytic capacity.Citation95–97 In support of this postulate, investigators have reported that PON2 can attenuate AHL-mediated biological effects that impair host cell function, based on its antioxidative and anti-apoptotic activities. By contrast, a recent study has shown that AHL induces apoptosis of mouse embryonic fibroblasts in a PON2-dependent manner.Citation98 Subsequent studies have shown that PON2 hydrolyzes AHL to AHL-acids and stores them in mitochondria, which subsequently results in a series of biological effects in mammalian cells Citation76,Citation99 ().
During Pseudomonas aeruginosa infection, the signal molecule AHL is secreted and sensed by neighboring bacteria through the quorum sensing system. AHL binds to LasR, leading to its own syngeneic synthesis. In the model of AHL treatment of host immune cells, a portion of AHL is incorporated into the host cell membrane, leading to the destruction of lipid domains, that is, the unique ordered lipid domains in eukaryotic cell membranes that are soluble in the presence of AHL. This in turn leads to the forced expulsion of tumor necrosis factor receptor 1 (TNFR1) to the disordered phase of the membrane, leading to a higher degree of spontaneous trimerization and TNFR1 signaling in the absence of external ligands. This distribution change alone drives the entire process of caspase 8 and caspase 3-axis activation and apoptosis, and it is used by Pseudomonas aeruginosa to suppress host immunity. Therefore, Pseudomonas aeruginosa gains a survival advantage when the host defense system is weakened. In this case, the toxicity of AHL is at least partially achieved by actively hijacking the host cell death signaling pathway. These findings indicate a previously unknown mechanism of how eukaryotic cells sense microbial metabolites Citation100 ().
Effects and potential mechanisms of QSSM AHL on host intestinal health
Impact of AHL on human and mouse intestinal health
The presence or absence of AHL in the mammalian gut is an unresolved issue in the scientific community. It has been suggested that the use of LuxR-based biosensors may not be able to detect AHL in the gut due to their detection limits being too high;Citation101,Citation102 thus, there is a need to develop new and more sensitive methods.Citation103 Kumari et al. developed a whole cell sensor system for detecting AHLs and showed that these signaling molecules detected in saliva and feces may be potential endogenous biomarkers for gastrointestinal diseases.Citation104 LC/MS-based methods were also used to detect AHLs in biological samples.Citation105–108 Furthermore, recent studies have developed a UPLC-MS/MS-based assay method to screen for 27 AHLs in gut microbiota and hosts. Various AHL molecules were identified in fecal content, serum, and the liver of conventionally-raised mice, but not in germ-free mice. Pathogen-produced C4-HSL was detected in fecal content and serum of mice infected with Citrobacter rodentium, but not in uninfected mice. In addition, Citrobacter rodentium infection significantly increased the serum levels of several AHL molecules. This study suggested that gut microbiota can produce a variety of AHLs and transport them into the host.Citation109 Furthermore, a team of investigators detected 14 different AHLs in the feces of IBD patients and healthy individuals by using mass spectrometry, and the distribution of AHLs correlated with disease status.Citation110
Impact of AHL on ruminant and pig intestinal health
In developed countries, such as Europe and the United States, cereal foods are widely used in the feeding of ruminants because they are economical. Due to the lack of quality forage, high percentage grain concentrate diets are also widely used in the feeding of ruminants in China to maximize the growth rate and economic efficiency of the animals. Administering high concentrate diets to dairy cows during specific physiological periods can effectively increase milk production.Citation111 However, long-term feeding can lower the pH of the gastrointestinal tract and cause excessive accumulation of volatile fatty acids (VFAs), leading to subacute rumen acidosis (SARA).Citation112–117 It has been reported that the prevalence of SARA in early and mid-lactation cows can reach 19% and 26%, respectively.Citation118,Citation119 SARA does not present serious clinical symptoms, but can cause physical damage to the mucosa of the digestive tract, and bacteria and bacterial products can easily invade the systemic circulation, which can then lead to diseases such as mastitis, laminitis, and hepatapostema, ultimately affecting ruminant health and performance and causing significant economic losses.Citation120,Citation121 Statistically, SARA costs the U.S. dairy industry $1 billion annually.Citation122 Erickson et al. Citation102 first studied the secretion of the AHL signaling molecule in the digestive tract of ruminants. The authors detected AHL in the rumen fluid of cattle fed at different concentrations in 80% of the animals. Moreover, AHL in the rumen fluid of ruminants fed a high concentrate diet had longer side chains than those fed a low concentrate diet. These results indicate that AHL does exist in the digestive tract of ruminants, and high concentrate diets can affect the secretion of AHL in the digestive tract to a certain extent. Furthermore, Edrington et al. Citation123 found that AHL was not detected in hindgut content samples of ruminants in all seasons by studying the effects of seasons on the secretion of AHL. The authors explained this phenomenon from two aspects: i) Under normal physiological conditions, the pH in the posterior segment of the intestine is alkaline, which leads to the opening of the AHL and its inactivation; ii) There may be a lack of microbes in the gut that produce AHL. However, after feeding the animals with a high concentrate diet, excessive fermentation in the posterior segment of the intestines of ruminants caused hindgut acidosis, which greatly reduced the pH in the intestinal lumen. As such, this may provide favorable conditions for the stabilization of the AHL structure. In our study, it was found that after ruminants were fed a high concentrate diet, the intestinal acidity decreased and the microflora drastically changed, which may increase the number of pathogenic microorganisms and certain conditional pathogens, eventually leading to the secretion of AHL.Citation124–128 So far, it is not clear whether AHL has any effect on the functional status of the host cells in the posterior segment of the gut of ruminants, let alone its potential mechanism.
The pig industry occupies a very important position in China<apos;>s economy. However, the pig<apos;>s birth weight and individual production performance variations in the breeding process have brought great inconvenience to the management of large-scale pig farms in terms of barn utilization, disease prevention, and feed preparation. Under modern breeding conditions, although pigs have the same genetic background and breeding environment, there are still obvious variations between their individual production performance.Citation129,Citation130 IUGR leads to the low birth weight of piglets, followed by high mortality during lactation and low growth performance throughout the life period. These are the outstanding problems in the pig industry.Citation131 According to statistics, 15% to 20% of IUGR piglets in China have a birth weight less than 1.1 kg.Citation132 IUGR piglets are usually accompanied by intestinal dysfunction after birth, which affects the animal<apos;>s production potential after birth.Citation133–135 Compared with normal birth weight piglets, the feed utilization efficiency of IUGR piglets in the later stage is reduced by 30%, and the average time to slaughter is extended by 30 days. The annual economic loss to China<apos;>s pig production is as high as 15 billion. Furthermore, the impact of IUGR on the intestinal health of pigs can continue until the growth period.Citation136–140 By systematically analyzing the function of the epithelial barrier in the hindgut (colon and cecum) of growing pigs, we found that IUGR leads to a persistent deterioration of the morphological structure, oxidative stress, and apoptosis. Furthermore, IUGR leads to impaired epithelial barrier function in the hindgut of growing pigs, and the proliferation of gram-negative bacteria in the intestine of pigs may play an important role.Citation141 It has been shown that gram-negative bacteria regulate the function of eukaryotic cells by secreting AHL, which disrupts the homeostasis of host intestinal epithelial cells and ultimately leads to intestinal epithelial barrier dysfunction.Citation142 It was shown that gram-negative bacteria, namely, Bacteroides and Clostridium, are the dominant microbiota in the intestine of IUGR pigs.Citation143 The proliferation of gram-negative bacteria in the intestinal lumen of IUGR piglets suggests that the concentration of AHL may be significantly increased in the intestine of IUGR pigs, and the deterioration of intestinal epithelial barrier function in IUGR pigs may be triggered by AHL. We further examined nine AHLs, including 3-oxo-C12-HSL and its analogs, and found that the concentrations of two AHL (3-oxo-C12-HSL and 3-oxo-C14-HSL) were significantly higher in the feces of IUGR pigs than those of normal birth weight pigs. Spearman correlation analysis showed a strong correlation between 3-oxo-C12-HSL and different microorganisms in the feces of IUGR pigs. In vitro cellular assay studies revealed that 3-oxo-C12-HSL impaired IPEC-J2 cell viability in a dose-dependent manner. By further exploring the molecular mechanism of 3-oxo-C12-HSL-induced damage in intestinal epithelial cells, we found that 3-oxo-C12-HSL mainly altered the “import across plasma membrane” and “arginine and proline metabolism” pathways in intestinal epithelial cells.Citation144
Potential mechanisms by which AHL impairs intestinal health
The gut<apos;>s first line of defense against invasion of pathogenic microorganisms and harmful metabolites is the mucus layer that covers the outer side of intestinal epithelial cells.Citation145,Citation146 The main component of the mucus layer is mucin, which is synthesized and secreted by intestinal goblet cells and acts as a barrier covering the entire intestinal surface.Citation147–149 Studies have shown that the absence or abnormal expression of mucins can lead to intestinal diseases, whereas pathogenic microorganisms and some of their metabolites can induce abnormal expression of mucins.Citation150–152 As for the influence of AHL on the intestinal mucus barrier, we first established a co-culture model of 3-oxo-C12-HSL and intestinal goblet cells, and demonstrated that 3-oxo-C12-HSL induced an imbalance of intestinal goblet cell homeostasis by causing mitochondrial swelling, mitochondrial membrane potential depolarization, mitochondrial dysfunction, and cell apoptosis. Furthermore, 3-oxo-C12-HSL inhibits mucin synthesis and sulfuration, ultimately destroying the intestinal mucus barrier. On this basis, the 3-oxo-C12-HSL/PON2 specific inhibitor/intestinal goblet cell co-culture model was established, and it was found that 3-oxo-C12-HSL induced a series of harmful biological effects in a PON2-dependent manner, eventually resulting in the disorder of intestinal goblet cell structure and function Citation85,Citation153,Citation154 ().
Figure 3. Proposed model of modulation of host gut damage by N-acyl-homoserine lactones (AHLs)-driven quorum sensing. Under the catalytic action of paraoxonase 2 (PON2), which activates the expression of caspase3, there is an induction of intestinal goblet cell apoptosis, an inhibition of mucin synthesis and secretion, and ultimately a destruction of the intestinal mucus barrier. AHLs induced calcium ion overload, mitochondrial damage, REDOX imbalance, and apoptosis of intestinal epithelial cells, but inhibited the expression of tight junctional and extracellular matrix proteins, thus destroying the intestinal epithelial cell barrier.
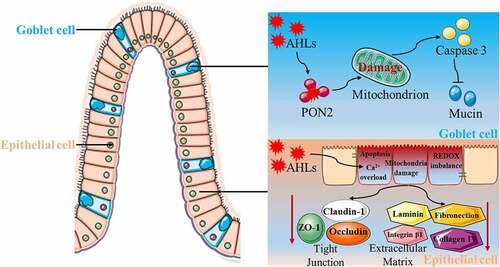
The physical barrier is an important feature of the intestinal barrier system. Its main function is to ensure the absorption of essential nutrients and prevent the translocation of pathogenic substances. Therefore, the physical barrier needs to have the characteristic of selective penetration.Citation155 Under normal circumstances, substances in the intestinal lumen cross the intestinal epithelial barrier through cell bypass and transcellular pathways. The completion of the cell bypass pathway requires the participation of junctional complexes located at the apical region of intestinal epithelial cells.Citation156,Citation157 The tight junctional complex between cells is mainly composed of tight junctions, adhesion junctions, and desmosomes. Tight junctions are a multi-protein complex composed of transmembrane proteins, peripheral membrane proteins, and regulatory molecules, which mainly include the claudin, occludin, and zona occludens (ZO) families.Citation158 The degradation, phosphorylation, or redistribution of proteins in the tight junctional complex can lead to changes in tight junction structure and function, disruption of the cytoskeleton or decreased transmembrane resistance of epithelial cells, increased epithelial permeability, disruption of epithelial barrier integrity, and dysfunction of the barrier. Therefore, maintaining the normal expression and distribution of tight junctional proteins between intestinal epithelial cells is essential for the function of the intestinal epithelial barrier. In recent years, many studies have shown that 3-oxo-C12-HSL can cause intestinal barrier dysfunction by destroying tight junctions between epithelial cells. A study on the human colon Caco-2 cell line showed that 3-oxo-C12-HSL can impair barrier function by reducing the expression and intracellular localization of tight junctional proteins, and induce matrix metalloprotease vitality loss, thus destroying barrier permeability and disrupting barrier function.Citation90 Vikstrom et al. found that 3-oxo-C12-HSL compromised the integrity of Caco-2 cells by decreasing transepithelial intercellular resistance, reducing the expression and distribution of ZO-1 and occluding, and redistributing F-actin proteins.Citation77,Citation159 Our research showed that 3-oxo-C12-HSL destroys the function of the intestinal epithelial cell barrier by inducing apoptosis, triggering mitochondrial dysfunction and redox imbalance, degrading the extracellular matrix, and disrupting tight junctions Citation160 ().
Conclusion
In summary, the knowledge of the microbial QS has been greatly improved in recent decades, and the interactive effects of the microbial QSSM with mammalian host cells have been studied in great depth. On this basis, we summarized the effects of AHL, a signaling molecule produced by gram-negative bacteria, on host cells and its potential mechanisms. Furthermore, we similarly reviewed the relationship between AHL and certain intestinal diseases and confirmed 3-oxo-C12-HSL as an AHL that plays a key role in the development of metabolic diseases such as SARA and IUGR, which may help to guide us in interventions of bacterial infections by intercepting QS signals.
Despite the rapid progress of studies related to AHL that affect the physiological status of mammalian cells, many questions remain to be addressed. i) Current studies on AHL-host interactions were largely limited to in vitro cell culture experiments, and there is an urgent need to understand whether AHL can exert deleterious biological effects by coordinating the behavior of microorganisms and modulating microbial networks or single bacteria through animal experiments. Recently, we used SPF and GF mice as animal models, which were combined with fecal microbial transplantation (FMT) technology, to discover that 3-oxo-C12-HSL causes intestinal barrier damage and systemic inflammation by perturbing the intestinal microbiome,Citation161 but the specific mechanisms need to be further investigated. ii) The explicit source of intestinal luminal AHL is unclear. The scientific community eagerly awaits well-designed experiments with a large number of individuals, as well as advanced technologies (metagenomics, metabolomics, and culturomics) to trace the origin of AHL. iii) A combination of qualitative, quantitative, and localization methods is needed to analyze the trajectory of AHL movement between the intestinal lumen and intestinal epithelial cells to better study how AHL contacts intestinal epithelial cells and exerts its biological effects. In conclusion, there is still a long way to understand the co-evolutionary pattern of microbial QS and QSSMs in humans and animals, and to scientifically develop strategies to prevent and treat QS-dependent bacterial infectious diseases from a multi-channel perspective.
Abbreviations
Authors’ contributions
ST and YX designed and wrote the review article. ZW, HZ, and JL revised the review article. TS, WL, and WW helped with designing figures and finding relevant literatures. TS approved the final manuscript. The authors read and approved the final manuscript.
Acknowledgments
Not applicable
Disclosure statement
No potential conflict of interest was reported by the author(s).
Additional information
Funding
References
- Dunny GM, Leonard BA. Cell-cell communication in gram-positive bacteria. Annu Rev Microbiol. 1997;51:527–19. doi:10.1146/annurev.micro.51.1.527.
- Surette MG, Bassler BL. Regulation of autoinducer production in salmonella typhimurium. Mol Microbiol. 1999;31:585–595. doi:10.1046/j.1365-2958.1999.01199.x.
- Fuqua WC, Winans SC, Greenberg EP. Quorum sensing in bacteria: the LuxR-LuxI family of cell density-responsive transcriptional regulators. J Bacteriol. 1994;176:269–275. doi:10.1128/jb.176.2.269-275.1994.
- Whiteley M, Diggle SP, Greenberg EP. Progress in and promise of bacterial quorum sensing research. Nature. 2017;551:313–320. doi:10.1038/nature24624.
- Tomasz A. Control of the competent state in Pneumococcus by a hormone-like cell product: an example for a new type of regulatory mechanism in bacteria. Nature. 1965;208:155–159. doi:10.1038/208155a0.
- Thompson JA, Oliveira RA, Djukovic A, Ubeda C, Xavier KB. Manipulation of the quorum sensing signal AI-2 affects the antibiotic-treated gut microbiota. Cell Rep. 2015;10:1861–1871. doi:10.1016/j.celrep.2015.02.049.
- Papenfort K, Bassler BL. Quorum sensing signal–response systems in Gram-negative bacteria. Nat Rev Microbiol. 2016;14(9):576–588. doi:10.1038/nrmicro.2016.89.
- Schuster M, Sexton DJ, Diggle SP, Greenberg EP. Acyl-homoserine lactone quorum sensing: from evolution to application. Annu Rev Microbiol. 2013;67(1):43–63. doi:10.1146/annurev-micro-092412-155635.
- Nealson KH. Autoinduction of bacterial luciferase. Occurrence, mechanism and significance. Arch Microbiol. 1977;112:73–79. doi:10.1007/BF00446657.
- Smith D, Wang JH, Swatton JE, Davenport P, Price B, Mikkelsen H, Stickland H, Nishikawa K, Gardiol N, Spring DR, et al. Variations on a theme: diverse N-acyl homoserine lactone-mediated quorum sensing mechanisms in gram-negative bacteria. Sci Prog. 2006;89:167–211. doi:10.3184/003685006783238335.
- Nievas F, Bogino P, Sorroche F, Giordano W. Detection, characterization, and biological effect of quorum-sensing signaling molecules in peanut-nodulating bradyrhizobia. Sensors. 2012;12:2851–2873. doi:10.3390/s120302851.
- Brelles-Marino G, Bedmar EJ. Detection, purification and characterisation of quorum-sensing signal molecules in plant-associated bacteria. J Biotechnol. 2001;91:197–209. doi:10.1016/S0168-1656(01)00330-3.
- Antonioli L, Blandizzi C, Pacher P, Guilliams M, Hasko G. Quorum sensing in the immune system. Nat Rev Immunol. 2018;18(9):563–568. doi:10.1038/s41577-018-0040-4.
- Bandyopadhaya A, Tzika AA, and Rahme LG. Pseudomonas aeruginosa quorum sensing molecule alters skeletal muscle protein homeostasis by perturbing the antioxidant defense system. mBio, 10 : 2019;e02211–19. doi:10.1128/mBio.02211-19.
- Kariminik A, Baseri-Salehi M, Kheirkhah B. Pseudomonas aeruginosa quorum sensing modulates immune responses: an updated review article. Immunol Lett. 2017;190:1–6. doi:10.1016/j.imlet.2017.07.002.
- Kendall MM, Sperandio V. What a dinner party! mechanisms and functions of interkingdom signaling in host-pathogen associations. mBio 2016. 7:e01748.10.1128/mBio.01748-15.
- Patankar AV, Gonzalez JE. Orphan LuxR regulators of quorum sensing. FEMS Microbiol Rev. 2009;33:739–756. doi:10.1111/j.1574-6976.2009.00163.x.
- Chong G, Kimyon O, Manefield M. Quorum sensing signal synthesis may represent a selective advantage independent of its role in regulation of bioluminescence in vibrio fischeri. PloS one. 2013;8:e67443. doi:10.1371/journal.pone.0067443.
- Bruger EL, Waters CM, Elliot MA. Bacterial quorum sensing stabilizes cooperation by optimizing growth strategies. Appl Environ Microbiol. 2016;82:6498–6506. doi:10.1128/AEM.01945-16.
- Venturi V, Bertani I, Kerenyi A, Netotea S, Pongor S, Herman C. Co-swarming and local collapse: quorum sensing conveys resilience to bacterial communities by localizing cheater mutants in Pseudomonas aeruginosa. PloS one. 2010;5:e9998. doi:10.1371/journal.pone.0009998.
- Popat R, Crusz SA, Messina M, Williams P, West SA, and Diggle SP. Quorum-sensing and cheating in bacterial biofilms. Proceedings Biological sciences . 2012; 279(1748) :4765–4771. doi:10.1098/rspb.2012.1976.
- Harrison F, Muruli A, Higgins S, Diggle SP, McCormick BA. Development of and ex vivo porcine lung model for studying growth, virulence, and signaling of pseudomonas aeruginosa. Infect Immun. 2014;82:3312–3323. doi:10.1128/IAI.01554-14.
- Valiente E, Bruhn JB, Nielsen KF, Larsen JL, Roig FJ, Gram L, Amaro C. Vibrio vulnificus produces quorum sensing signals of the AHL-class. FEMS Microbiol Ecol. 2009;69:16–26. doi:10.1111/j.1574-6941.2009.00691.x.
- Elmahdi S, DaSilva LV, Parveen S. Antibiotic resistance of vibrio parahaemolyticus and vibrio vulnificus in various countries: a review. Food Microbiol. 2016;57:128–134. doi:10.1016/j.fm.2016.02.008.
- Bruhn JB, Dalsgaard I, Nielsen KF, Buchholtz C, Larsen JL, Gram L. Quorum sensing signal molecules (acylated homoserine lactones) in gram-negative fish pathogenic bacteria. Dis Aquat Organ. 2005;65:43–52. doi:10.3354/dao065043.
- Vincent AT, Hosseini N, Charette SJ. The Aeromonas salmonicida plasmidome: a model of modular evolution and genetic diversity. Ann N Y Acad Sci. 2021;1488:16–32. doi:10.1111/nyas.14503.
- Wrobel A, Leo JC, and Linke D. Overcoming fish defences: the virulence factors of yersinia ruckeri. Genes. 2019;10: 700 doi: 10.3390/genes10090700.
- Lang J, Faure D. Functions and regulation of quorum-sensing in agrobacterium tumefaciens. Front Plant Sci. 2014;5:14. doi:10.3389/fpls.2014.00014.
- Milton DL, Hardman A, Camara M, Chhabra SR, Bycroft BW, Stewart GS, Williams, P . Quorum sensing in Vibrio anguillarum: characterization of the vanI/vanR locus and identification of the autoinducer N-(3-oxodecanoyl)-L-homoserine lactone. J Bacteriol. 1997;179:3004–3012. doi:10.1128/jb.179.9.3004-3012.1997.
- Engebrecht J, and Silverman M. Identification of genes and gene products necessary for bacterial bioluminescence. Proceedings of the National Academy of Sciences of the United States of America. 1984,P NATL ACAD SCI USA; 81 :4154–4158.
- Fuqua C, Winans SC, Greenberg EP. Census and consensus in bacterial ecosystems: the LuxR-LuxI family of quorum-sensing transcriptional regulators. Annu Rev Microbiol. 1996;50:727–751. doi:10.1146/annurev.micro.50.1.727.
- Hanzelka BL, Greenberg EP. Evidence that the N-terminal region of the vibrio fischeri luxr protein constitutes an autoinducer-binding domain. J Bacteriol. 1995;177:815–817. doi:10.1128/jb.177.3.815-817.1995.
- Zhu J, and Winans SC. The quorum-sensing transcriptional regulator TraR requires its cognate signaling ligand for protein folding, protease resistance, and dimerization. Proceedings of the National Academy of Sciences of the United States of America. P NATL ACAD SCI USA ,2001; 98:1507–1512.
- Winson MK, Swift S, Fish L, Throup JP, Jorgensen F, Chhabra SR, Bycroft BW, Williams P, Stewart GS . Construction and analysis of luxCDABE-based plasmid sensors for investigating N-acyl homoserine lactone-mediated quorum sensing. FEMS Microbiol Lett. 1998;163:185–192. doi:10.1111/j.1574-6968.1998.tb13044.x.
- Ahmer BM. Cell-to-cell signalling in escherichia coli and salmonella enterica. Mol Microbiol. 2004;52:933–945. doi:10.1111/j.1365-2958.2004.04054.x.
- Lee J, Maeda T, Hong SH, Wood TK. Reconfiguring the quorum-sensing regulator SdiA of escherichia coli to control biofilm formation via indole and N-acylhomoserine lactones. Appl Environ Microbiol. 2009;75:1703–1716. doi:10.1128/AEM.02081-08.
- Lee J, Wu J, Deng Y, Wang J, Wang C, Wang J, Chang C, Dong Y, Williams P, Zhang L-H, et al. A cell-cell communication signal integrates quorum sensing and stress response. Nat Chem Biol. 2013;9(5):339–343. doi:10.1038/nchembio.1225.
- Jimenez PN, Koch G, Thompson JA, Xavier KB, Cool RH, Quax WJ. The multiple signaling systems regulating virulence in pseudomonas aeruginosa. Microbiology and Molecular Biology Reviews: MMBR. 2012;76(1):46–65. doi:10.1128/MMBR.05007-11.
- Farrow JM 3rd, Pesci EC. Two distinct pathways supply anthranilate as a precursor of the pseudomonas quinolone signal. J Bacteriol. 2007;189(9):3312–3333. doi:10.1128/JB.00209-07.
- Bredenbruch F, Nimtz M, Wray V, Morr M, Muller R, Haussler S. Biosynthetic pathway of Pseudomonas aeruginosa 4-hydroxy-2-alkylquinolines. J Bacteriol. 2005;187:3630–3635. doi:10.1128/JB.187.11.3630-3635.2005.
- Ventre I, Goodman AL, Vallet-Gely I, Vasseur P, Soscia C, Molin S, Bleves S, Lazdunski A, Lory S, Filloux A . Multiple sensors control reciprocal expression of Pseudomonas aeruginosa regulatory RNA and virulence genes. Proceedings of the National Academy of Sciences of the United States of America. P NATL ACAD SCI USA, 2006; 103:171–176.
- Ritchie AJ, Whittall C, Lazenby JJ, Chhabra SR, Pritchard DI, Cooley MA. The immunomodulatory Pseudomonas aeruginosa signalling molecule N-(3-oxododecanoyl)-L-homoserine lactone enters mammalian cells in an unregulated fashion. Immunol Cell Biol. 2007;85:596–602. doi:10.1038/sj.icb.7100090.
- Schwarzer C, Fu Z, Shuai S, Babbar S, Zhao G, Li C, Machen TE . Pseudomonas aeruginosa homoserine lactone triggers apoptosis and Bak/Bax-independent release of mitochondrial cytochrome C in fibroblasts. Cell Microbiol. 2014;16:1094–1104. doi:10.1111/cmi.12263.
- Schwarzer C, Ravishankar B, Patanwala M, Shuai S, Fu Z, Illek B, Fischer H, Machen TE . Thapsigargin blocks Pseudomonas aeruginosa homoserine lactone-induced apoptosis in airway epithelia. Am J Physiol Cell Physiol. 2014;306:C844–55. doi:10.1152/ajpcell.00002.2014.
- Taguchi R, Tanaka S, Joe GH, Maseda H, Nomura N, Ohnishi J, Ishizuka S, Shimizu H, Miyazaki H. Mucin 3 is involved in intestinal epithelial cell apoptosis via N -(3-oxododecanoyl)- -homoserine lactone-induced suppression of Akt phosphorylation. Am J Physiol Cell Physiol. 2014;307:C162–8. doi:10.1152/ajpcell.00271.2013.
- Shimizu H, Baba N, Nose T, Taguchi R, Tanaka S, Joe GH, Maseda H, Nomura N, Hagio M, Lee JY . Activity of ERK regulates mucin 3 expression and is involved in undifferentiated Caco-2 cell death induced by 3-oxo-C12-homoserine lactone. Bioscience, Biotechnology, and Biochemistry. 2015;79:937–942. doi:10.1080/09168451.2015.1006570.
- Holm A, Magnusson KE, Vikstrom E. Pseudomonas aeruginosa N-3-oxo-dodecanoyl-homoserine lactone elicits changes in cell volume, morphology, and AQP9 characteristics in macrophages. Front Cell Infect Microbiol. 2016;6:32. doi:10.3389/fcimb.2016.00032.
- Vikstrom E, Magnusson KE, Pivoriunas A. The Pseudomonas aeruginosa quorum-sensing molecule N-(3-oxododecanoyl)-L-homoserine lactone stimulates phagocytic activity in human macrophages through the p38 MAPK pathway. Microbes and Infection. 2005;7:1512–1518. doi:10.1016/j.micinf.2005.05.012.
- Kaufmann GF, Park J, Mee JM, Ulevitch RJ, Janda KD. The quorum quenching antibody RS2-1G9 protects macrophages from the cytotoxic effects of the Pseudomonas aeruginosa quorum sensing signalling molecule N-3-oxo-dodecanoyl-homoserine lactone. Mol Immunol. 2008;45:2710–2714. doi:10.1016/j.molimm.2008.01.010.
- Horikawa M, Tateda K, Tuzuki E, Ishii Y, Ueda C, Takabatake T, Miyairi S, Yamaguchi K, Ishiguro M. Synthesis of Pseudomonas quorum-sensing autoinducer analogs and structural entities required for induction of apoptosis in macrophages. Bioorg Med Chem Lett. 2006;16:2130–2133. doi:10.1016/j.bmcl.2006.01.054.
- Rennemeier C, Frambach T, Hennicke F, Dietl J, Staib P. Microbial quorum-sensing molecules induce acrosome loss and cell death in human spermatozoa. Infect Immun. 2009;77:4990–4997. doi:10.1128/IAI.00586-09.
- Scheper MA, Shirtliff ME, Meiller TF, Peters BM, Jabra-Rizk MA. Farnesol, a fungal quorum-sensing molecule triggers apoptosis in human oral squamous carcinoma cells. Neoplasia. 2008;10:954–963. doi:10.1593/neo.08444.
- Glucksam-Galnoy Y, Sananes R, Silberstein N, Krief P, Kravchenko M VV, Meijler, MM MM, Zor T. The bacterial quorum-sensing signal molecule -3-oxo-dodecanoyl-l-homoserine lactone reciprocally modulates pro- and anti-inflammatory cytokines in activated macrophages. Journal of Immunology. 2013;191:337–344. doi:10.4049/jimmunol.1300368.
- Holm A, Karlsson T, Vikstrom E. Pseudomonas aeruginosa lasI/rhlI quorum sensing genes promote phagocytosis and aquaporin 9 redistribution to the leading and trailing regions in macrophages. Front Microbiol. 2015;6:915. doi:10.3389/fmicb.2015.00915.
- Guo J, Yoshida K, Ikegame M, Okamura H. Quorum sensing molecule N-(3-oxododecanoyl)-l-homoserine lactone: an all-rounder in mammalian cell modification. Journal of Oral Biosciences. 2020;62(1):16–29. doi:10.1016/j.job.2020.01.001.
- Skindersoe ME, Zeuthen LH, Brix S, Fink LN, Lazenby J, Whittall C, Williams P, Diggle SP, Froekiaer H, Cooley M . Pseudomonas aeruginosa quorum-sensing signal molecules interfere with dendritic cell-induced T-cell proliferation. FEMS Immunol Med Microbiol. 2009;55:335–345. doi:10.1111/j.1574-695X.2008.00533.x.
- Li Y, Zhou H, Zhang Y, Chen C, Huang B, Qu P, Zeng J, Shunmei E, Zhang X, Liu J . N-3-(oxododecanoyl)-L-homoserine lactone promotes the induction of regulatory T-cells by preventing human dendritic cell maturation. Exp Biol Med. 2015;240:896–903. doi:10.1177/1535370214564742.
- Ritchie AJ, Yam AO, Tanabe KM, Rice SA, Cooley MA. Modification of in vivo and in vitro T- and B-cell-mediated immune responses by the Pseudomonas aeruginosa quorum-sensing molecule N-(3-oxododecanoyl)-L-homoserine lactone. Infect Immun. 2003;71:4421–4431. doi:10.1128/IAI.71.8.4421-4431.2003.
- Ritchie AJ, Jansson A, Stallberg J, Nilsson P, Lysaght P, Cooley MA. The Pseudomonas aeruginosa quorum-sensing molecule N-3-(oxododecanoyl)-L-homoserine lactone inhibits T-cell differentiation and cytokine production by a mechanism involving an early step in T-cell activation. Infect Immun. 2005;73:1648–1655. doi:10.1128/IAI.73.3.1648-1655.2005.
- Telford G, Wheeler D, Williams P, Tomkins PT, Appleby P, Sewell H, Stewart GS, Bycroft BW, Pritchard DI . The Pseudomonas aeruginosa quorum-sensing signal molecule N-(3-oxododecanoyl)-L-homoserine lactone has immunomodulatory activity. Infect Immun. 1998;66:36–42. doi:10.1128/IAI.66.1.36-42.1998.
- Jacobi CA, Schiffner F, Henkel M, Waibel M, Stork B, Daubrawa M, Eberl L, Gregor M, Wesselborg S . Effects of bacterial N-acyl homoserine lactones on human Jurkat T lymphocytes-OdDHL induces apoptosis via the mitochondrial pathway. International Journal of Medical Microbiology: IJMM. 2009;299:509–519. doi:10.1016/j.ijmm.2009.03.005.
- Hooi DS, Bycroft BW, Chhabra SR, Williams P, Pritchard DI. Differential immune modulatory activity of Pseudomonas aeruginosa quorum-sensing signal molecules. Infect Immun. 2004;72:6463–6470. doi:10.1128/IAI.72.11.6463-6470.2004.
- Gupta RK, Chhibber S, Harjai K, DeLeo FR. Acyl homoserine lactones from culture supernatants of Pseudomonas aeruginosa accelerate host immunomodulation. PloS one. 2011;6:e20860. doi:10.1371/journal.pone.0020860.
- Tateda K, Ishii Y, Horikawa M, Matsumoto T, Miyairi S, Pechere JC, Standiford TJ, Ishiguro M, Yamaguchi K. The Pseudomonas aeruginosa autoinducer N −3-oxododecanoyl homoserine lactone accelerates apoptosis in macrophages and neutrophils. Infect Immun. 2003;71:5785–5793. doi:10.1128/IAI.71.10.5785-5793.2003.
- Schwarzer C, Fu Z, Patanwala M, Hum L, Lopez-Guzman M, Illek B, Kong W, Lynch SV, Machen TE . Pseudomonas aeruginosa biofilm-associated homoserine lactone C12 rapidly activates apoptosis in airway epithelia. Cell Microbiol. 2012;14:698–709. doi:10.1111/j.1462-5822.2012.01753.x.
- Li L, Hooi D, Chhabra SR, Pritchard D, Shaw PE. Bacterial N-acylhomoserine lactone-induced apoptosis in breast carcinoma cells correlated with down-modulation of STAT3. Oncogene. 2004;23:4894–4902. doi:10.1038/sj.onc.1207612.
- Shiner EK, Terentyev D, Bryan A, Sennoune S, Martinez-Zaguilan R, Li G, Gyorke S, Williams SC, Rumbaugh KP . Pseudomonas aeruginosa autoinducer modulates host cell responses through calcium signalling. Cell Microbiol. 2006;8:1601–1610. doi:10.1111/j.1462-5822.2006.00734.x.
- Valentine CD, Anderson MO, Papa FR, Haggie PM, Parsek MR. X-box binding protein 1 (XBP1s) is a critical determinant of Pseudomonas aeruginosa homoserine lactone-mediated apoptosis. PLoS Pathog. 2013;9:e1003576. doi:10.1371/journal.ppat.1003576.
- Smith RS, Fedyk ER, Springer TA, Mukaida N, Iglewski BH, Phipps RP. IL-8 production in human lung fibroblasts and epithelial cells activated by the Pseudomonas autoinducer N-3-oxododecanoyl homoserine lactone is transcriptionally regulated by NF-kappa B and activator protein-2. Journal of Immunology. 2001;167:366–374. doi:10.4049/jimmunol.167.1.366.
- DiMango E, Zar HJ, Bryan R, Prince A. Diverse Pseudomonas aeruginosa gene products stimulate respiratory epithelial cells to produce interleukin-8. J Clin Invest. 1995;96:2204–2210. doi:10.1172/JCI118275.
- Mayer ML, Sheridan JA, Blohmke CJ, Turvey SE, Hancock RE. The Pseudomonas aeruginosa autoinducer 3O-C12 homoserine lactone provokes hyperinflammatory responses from cystic fibrosis airway epithelial cells. PloS one. 2011;6:e16246. doi:10.1371/journal.pone.0016246.
- Valentine CD, Zhang H, Phuan PW, Nguyen J, Verkman AS, Haggie PM. Small molecule screen yields inhibitors of Pseudomonas homoserine lactone-induced host responses. Cell Microbiol. 2014;16:1–14.
- Grabiner MA, Fu Z, Wu T, Barry KC, Schwarzer C, Machen TE. Pseudomonas aeruginosa quorum-sensing molecule homoserine lactone modulates inflammatory signaling through PERK and eI-F2alpha. Journal of Immunology. 2014;193:1459–1467. doi:10.4049/jimmunol.1303437.
- Zhang J, Gong F, Li L, Zhao M, Song J. Pseudomonas aeruginosa quorum-sensing molecule N-(3-oxododecanoyl) homoserine lactone attenuates lipopolysaccharide-induced inflammation by activating the unfolded protein response. Biomedical Reports. 2014;2:233–238. doi:10.3892/br.2014.225.
- Miyairi S, Tateda K, Fuse ET, Ueda C, Saito H, Takabatake T, Ishii Y, Horikawa M, Ishiguro M, Standiford TJ . Immunization with 3-oxododecanoyl-L-homoserine lactone-protein conjugate protects mice from lethal Pseudomonas aeruginosa lung infection. J Med Microbiol. 2006;55:1381–1387. doi:10.1099/jmm.0.46658-0.
- Horke S, Xiao J, Schutz EM, Kramer GL, Wilgenbus P, Witte I, Selbach M, Teiber JF . Novel paraoxonase 2-dependent mechanism mediating the biological effects of the pseudomonas aeruginosa quorum-sensing molecule N-(3-oxo-dodecanoyl)-L-homoserine lactone. Infect Immun. 2015;83:3369–3380. doi:10.1128/IAI.00141-15.
- Vikstrom E, Bui L, Konradsson P, Magnusson KE. The junctional integrity of epithelial cells is modulated by Pseudomonas aeruginosa quorum sensing molecule through phosphorylation-dependent mechanisms. Exp Cell Res. 2009;315:313–326. doi:10.1016/j.yexcr.2008.10.044.
- Zaborina O, Kohler JE, Wang Y, Bethel C, Shevchenko O, Wu L, Turner JR, Alverdy JC . Identification of multi-drug resistant Pseudomonas aeruginosa clinical isolates that are highly disruptive to the intestinal epithelial barrier. Ann Clin Microbiol Antimicrob. 2006;5:14. doi:10.1186/1476-0711-5-14.
- Charlton TS, de Nys R, Netting A, Kumar N, Hentzer M, Givskov M, Kjelleberg S. A novel and sensitive method for the quantification of N −3-oxoacyl homoserine lactones using gas chromatography-mass spectrometry: application to a model bacterial biofilm. Environ Microbiol. 2000;2:530–541. doi:10.1046/j.1462-2920.2000.00136.x.
- Akira S, Uematsu S, Takeuchi O. Pathogen recognition and innate immunity. Cell. 2006;124:783–801.
- Wagner C, Zimmermann S, Brenner-Weiss G, Hug F, Prior B, Obst U, Hänsch GM . The quorum-sensing molecule N-3-oxododecanoyl homoserine lactone (3OC12-HSL) enhances the host defence by activating human polymorphonuclear neutrophils (PMN). Anal Bioanal Chem. 2007;387:481–487. doi:10.1007/s00216-006-0698-5.
- Smith RS, Harris SG, Phipps R, Iglewski B. The Pseudomonas aeruginosa quorum-sensing molecule N-(3-oxododecanoyl)homoserine lactone contributes to virulence and induces inflammation in vivo. J Bacteriol. 2002;184:1132–1139. doi:10.1128/jb.184.4.1132-1139.2002.
- Kravchenko K VV, Mathison GF, Scott JC, Katz DA, Grauer AZ, Grauer, DC DC, Lehmann M, Meijler MM, Janda KD, et al. Modulation of gene expression via disruption of NF-kappaB signaling by a bacterial small molecule. Science. 2008;321:259–263. doi:10.1126/science.1156499.
- Kravchenko K VV, Mathison GF, Scott JC, Katz DA, Wood AZ, Wood, MR MR, Brogan AP, Lehmann M, Mee JM, et al. N-(3-oxo-acyl)homoserine lactones signal cell activation through a mechanism distinct from the canonical pathogen-associated molecular pattern recognition receptor pathways. J Biol Chem. 2006;281:28822–28830. doi:10.1074/jbc.M606613200.
- Tao S, Sun Q, Cai L, Geng Y, Hua C, Ni Y, Zhao R. Caspase-1-dependent mechanism mediating the harmful impacts of the quorum-sensing molecule N-(3-oxo-dodecanoyl)- -homoserine lactone on the intestinal cells. J Cell Physiol. 2019;234:3621–3633. doi:10.1002/jcp.27132.
- Sonnino S, Prinetti A. Membrane domains and the ”lipid raft” concept. Curr Med Chem. 2013;20:4–21.
- Lingwood D, Simons K. Lipid rafts as a membrane-organizing principle. Science. 2010;327:46–50. doi:10.1126/science.1174621.
- Sezgin E, Levental I, Mayor S, Eggeling C. The mystery of membrane organization: composition, regulation and roles of lipid rafts. Nat Rev Mol Cell Biol. 2017;18(6):361–374. doi:10.1038/nrm.2017.16.
- Lee S-J, Jung YH, Oh SY, Jang KK, Lee HS, Choi SH, Han HJ. Vibrio vulnificus VvpE inhibits mucin 2 expression by hypermethylation via lipid raft-mediated ROS signaling in intestinal epithelial cells. Cell Death Dis. 2015;6(6):e1787. doi:10.1038/cddis.2015.152.
- Eum SY, Jaraki D, Bertrand L, Andras IE, Toborek M. Disruption of epithelial barrier by quorum-sensing N −3-(oxododecanoyl)-homoserine lactone is mediated by matrix metalloproteinases. American Journal of Physiology-Gastrointestinal and Liver Physiology. 2014;306(11):G992–G1001. doi:10.1152/ajpgi.00016.2014.
- Jahoor A, Patel R, Bryan A, Do C, Krier J, Watters C, Wahli W, Li G, Williams SC, Rumbaugh KP, et al. Peroxisome proliferator-activated receptors mediate host cell proinflammatory responses to Pseudomonas aeruginosa autoinducer. J Bacteriol. 2008;190:4408–4415. doi:10.1128/JB.01444-07.
- Harris SG, Phipps RP. The nuclear receptor PPAR gamma is expressed by mouse T lymphocytes and PPAR gamma agonists induce apoptosis. Eur J Immunol. 2001;31:1098–1105. doi:10.1002/1521-4141(200104)31:4<1098::AID-IMMU1098>3.0.CO;2-I.
- Ramer R, Heinemann K, Merkord J, Rohde H, Salamon A, Linnebacher M, Hinz B. COX-2 and PPAR-gamma confer cannabidiol-induced apoptosis of human lung cancer cells. Mol Cancer Ther. 2013;12:69–82. doi:10.1158/1535-7163.MCT-12-0335.
- Wu JS, Lin TN, Wu KK. Rosiglitazone and PPAR-gamma overexpression protect mitochondrial membrane potential and prevent apoptosis by upregulating anti-apoptotic Bcl-2 family proteins. J Cell Physiol. 2009;220:58–71. doi:10.1002/jcp.21730.
- Horke S, Witte I, Wilgenbus P, Kruger M, Strand D, Forstermann U. Paraoxonase-2 reduces oxidative stress in vascular cells and decreases endoplasmic reticulum stress-induced caspase activation. Circulation. 2007;115:2055–2064. doi:10.1161/CIRCULATIONAHA.106.681700.
- Kim JB, Xia YR, Romanoski CE, Lee S, Meng Y, Shi YS, Bourquard N, Gong KW, Port Z, Grijalva V . Paraoxonase-2 modulates stress response of endothelial cells to oxidized phospholipids and a bacterial quorum-sensing molecule. Arterioscler Thromb Vasc Biol. 2011;31:2624–2633. doi:10.1161/ATVBAHA.111.232827.
- Devarajan A, Bourquard N, Grijalva VR, Gao F, Ganapathy E, Verma J, Reddy ST. Role of PON2 in innate immune response in an acute infection model. Mol Genet Metab. 2013;110:362–370. doi:10.1016/j.ymgme.2013.07.003.
- Schwarzer C, Fu Z, Morita T, Whitt AG, Neely AM, Li C, Machen TE . Paraoxonase 2 serves a proapopotic function in mouse and human cells in response to the Pseudomonas aeruginosa quorum-sensing molecule N-(3-Oxododecanoyl)-homoserine lactone. J Biol Chem. 2015;290(11):7247–7258. doi:10.1074/jbc.M114.620039.
- Gupta SC, Singh R, Pochampally R, Watabe K, Mo YY. Acidosis promotes invasiveness of breast cancer cells through ROS-AKT-NF-kappaB pathway. Oncotarget. 2014;5:12070–12082. doi:10.18632/oncotarget.2514.
- Song D, Meng J, Cheng J, Fan Z, Chen P, Ruan H, Tu Z, Kang N, Li N, Xu Y, et al. Pseudomonas aeruginosa quorum-sensing metabolite induces host immune cell death through cell surface lipid domain dissolution. Nature Microbiology. 2019;4:97–111. doi:10.1038/s41564-018-0290-8.
- Jacobi CA, Bach A, Eberl L, Steidle A, Heesemann J. Detection of N-(3-oxohexanoyl)-L-homoserine lactone in mice infected with Yersinia enterocolitica serotype O8. Infect Immun. 2003;71:6624–6626. doi:10.1128/IAI.71.11.6624-6626.2003.
- Erickson DL, Nsereko VL, Morgavi DP, Selinger LB, Rode LM, Beauchemin KA. Evidence of quorum sensing in the rumen ecosystem: detection of N-acyl homoserine lactone autoinducers in ruminal contents. Can J Microbiol. 2002;48:374–378. doi:10.1139/w02-022.
- Swearingen MC, Sabag-Daigle A, Ahmer BM. Are there acyl-homoserine lactones within mammalian intestines? J Bacteriol. 2013;195:173–179. doi:10.1128/JB.01341-12.
- Kumari A, Pasini P, Deo SK, Flomenhoft D, Shashidhar H, Daunert S. Biosensing systems for the detection of bacterial quorum signaling molecules. Anal Chem. 2006;78:7603–7609.
- Lepine F, Milot S, Groleau MC, Deziel E. Liquid chromatography/mass spectrometry (LC/MS) for the detection and quantification of N-Acyl-L-homoserine lactones (AHLs) and 4-hydroxy-2-alkylquinolines (HAQs). Methods in Molecular Biology. 2018;1673:49–59.
- Lepine F, Deziel E. Liquid chromatography/mass spectrometry for the detection and quantification of N-acyl-L-homoserine lactones and 4-hydroxy-2-alkylquinolines. Methods in Molecular Biology. 2011;692:61–69.
- Patel NM, Moore JD, Blackwell HE, Amador-Noguez D, Omri A. Identification of unanticipated and novel n-acyl l-homoserine lactones (ahls) using a sensitive non-targeted LC-MS/MS method. PloS one. 2016;11:e0163469. doi:10.1371/journal.pone.0163469.
- Wang J, Ding L, Li K, Schmieder W, Geng J, Xu K, Zhang Y, Ren H. Development of an extraction method and LC-MS analysis for N-acylated-l-homoserine lactones (AHLs) in wastewater treatment biofilms. J Chromatogr B Analyt Technol Biomed Life Sci. 2017;1041-1042:37–44. doi:10.1016/j.jchromb.2016.11.029.
- Xue J, Chi L, Tu P, Lai Y, Liu CW, Ru H, Lu K . Detection of gut microbiota and pathogen produced N-acyl homoserine in host circulation and tissues. NPJ Biofilms and Microbiomes. 2021;7:53. doi:10.1038/s41522-021-00224-5.
- Landman C, Grill J-P, Mallet J-M, Marteau P, Humbert L, Le Balc’h E, Maubert MA, Perez K, Chaara W, Brot L . Inter-kingdom effect on epithelial cells of the N-Acyl homoserine lactone 3-oxo-C12:2, a major quorum-sensing molecule from gut microbiota. PloS one. 2018;13(8):e0202587. doi:10.1371/journal.pone.0202587.
- Morgante M, Stelletta C, Berzaghi P, Gianesella M, Andrighetto I. Subacute rumen acidosis in lactating cows: an investigation in intensive Italian dairy herds. J Anim Physiol Anim Nutr (Berl). 2007;91(5–6):527–564. doi:10.1111/j.1439-0396.2007.00696.x.
- Plaizier JC, Krause DO, Gozho GN, McBride BW. Subacute ruminal acidosis in dairy cows: the physiological causes, incidence and consequences. Veterinary Journal. 2008;176:21–31. doi:10.1016/j.tvjl.2007.12.016.
- Gozho GN, Krause DO, Plaizier JC. Ruminal lipopolysaccharide concentration and inflammatory response during grain-induced subacute ruminal acidosis in dairy cows. J Dairy Sci. 2007;90:856–866. doi:10.3168/jds.S0022-0302(07)71569-2.
- Penner GB, Beauchemin KA, Mutsvangwa T. Severity of ruminal acidosis in primiparous Holstein cows during the periparturient period. J Dairy Sci. 2007;90:365–375. doi:10.3168/jds.S0022-0302(07)72638-3.
- Penner GB, Taniguchi M, Guan LL, Beauchemin KA, Oba M. Effect of dietary forage to concentrate ratio on volatile fatty acid absorption and the expression of genes related to volatile fatty acid absorption and metabolism in ruminal tissue. J Dairy Sci. 2009;92:2767–2781. doi:10.3168/jds.2008-1716.
- Bevans DW, Beauchemin KA, Schwartzkopf-Genswein KS, McKinnon JJ, McAllister TA. Effect of rapid or gradual grain adaptation on subacute acidosis and feed intake by feedlot cattle. J Anim Sci. 2005;83:1116–1132. doi:10.2527/2005.8351116x.
- Steele MA, AlZahal O, Hook SE, Croom J, McBride BW. Ruminal acidosis and the rapid onset of ruminal parakeratosis in a mature dairy cow: a case report. Acta Vet Scand. 2009;51:39. doi:10.1186/1751-0147-51-39.
- Kleen JL, Hooijer GA, Rehage J, Noordhuizen JP. Subacute ruminal acidosis (SARA): a review. J Vet Med A Physiol Pathol Clin Med. 2003;50:406–414. doi:10.1046/j.1439-0442.2003.00569.x.
- Kleen JL, Hooijer GA, Rehage J, Noordhuizen JP. Subacute ruminal acidosis in Dutch dairy herds. Vet Rec. 2009;164:681–683. doi:10.1136/vr.164.22.681.
- Nagaraja TG, Titgemeyer EC. Ruminal acidosis in beef cattle: the current microbiological and nutritional outlook. J Dairy Sci. 2007;90(1):E17–38. doi:10.3168/jds.2006-478.
- Nocek JE. Bovine acidosis: implications on laminitis. J Dairy Sci. 1997;80:1005–1028. doi:10.3168/jds.S0022-0302(97)76026-0.
- Enemark JM. The monitoring, prevention and treatment of sub-acute ruminal acidosis (SARA): a review. Veterinary Journal. 2008;176:32–43. doi:10.1016/j.tvjl.2007.12.021.
- Edrington TS, Farrow RL, Sperandio V, Hughes DT, Lawrence TE, Callaway TR, Anderson RC, Nisbet DJ . Acyl-homoserine-lactone autoinducer in the gastrointestinal [corrected] tract of feedlot cattle and correlation to season, E. coli O157:H7 prevalence, and diet. Curr Microbiol. 2009;58:227–232.
- Tao S, Tian P, Luo Y, Tian J, Hua C, Geng Y, Cong R, Ni Y, Zhao R . Microbiome-Metabolome responses to a high-grain diet associated with the hind-gut health of goats. Front Microbiol. 2017;8:1764. doi:10.3389/fmicb.2017.01764.
- Tao S, Han Z, Tian J, Cong R, Duanmu Y, Dong H, Ni Y, Zhao R . Downregulation of prostaglandin E2 is involved in hindgut mucosal damage in lactating goats fed a high-concentrate diet. Exp Physiol. 2016;101:272–281. doi:10.1113/EP085256.
- Tao S, Duanmu Y, Dong H, Ni Y, Chen J, Shen X, Zhao R. High concentrate diet induced mucosal injuries by enhancing epithelial apoptosis and inflammatory response in the hindgut of goats. PloS one. 2014;9:e111596. doi:10.1371/journal.pone.0111596.
- Tao S, Duanmu Y, Dong H, Tian J, Ni Y, Zhao R. A high-concentrate diet induced colonic epithelial barrier disruption is associated with the activating of cell apoptosis in lactating goats. BMC Vet Res. 2014;10:235. doi:10.1186/s12917-014-0235-2.
- Tao S, Tian J, Cong R, Sun L, Duanmu Y, Dong H, Ni Y, Zhao R. Activation of cellular apoptosis in the caecal epithelium is associated with increased oxidative reactions in lactating goats after feeding a high-concentrate diet. Exp Physiol. 2015;100:278–287. doi:10.1113/expphysiol.2014.083352.
- Quesnel H, Brossard L, Valancogne A, Quiniou N. Influence of some sow characteristics on within-litter variation of piglet birth weight. Animal: An International Journal of Animal Bioscience. 2008;2:1842–1849. doi:10.1017/S175173110800308X.
- Yuan T-L, Zhu Y-H, Shi M, Li -T-T, Li N, Wu G-Y, Bazer FW, Zang -J-J, Wang F-L, Wang -J-J, et al. 母猪营养状态对仔猪初生重窝内变异的影响. Journal of Zhejiang University-SCIENCE B. 2015;16(6):417–435. doi:10.1631/jzus.B1500010.
- Wang J, Feng C, Liu T, Shi M, Wu G, Bazer FW. Physiological alterations associated with intrauterine growth restriction in fetal pigs: causes and insights for nutritional optimization. Mol Reprod Dev. 2017;84:897–904. doi:10.1002/mrd.22842.
- Wu G, Bazer FW, Wallace JM, Spencer TE. Board-invited review: intrauterine growth retardation: implications for the animal sciences. J Anim Sci. 2006;84:2316–2337. doi:10.2527/jas.2006-156.
- Hu Y, Hu L, Gong D, Lu H, Xuan Y, Wang R, Wu D, Chen D, Zhang K, Gao F, et al. Genome-wide DNA methylation analysis in jejunum of Sus scrofa with intrauterine growth restriction. Molecular Genetics and Genomics: MGG. 2018;293:807–818. doi:10.1007/s00438-018-1422-9.
- Su W, Zhang H, Ying Z, Li Y, Zhou L, Wang F, Zhang L, Wang T. Effects of dietary L-methionine supplementation on intestinal integrity and oxidative status in intrauterine growth-retarded weanling piglets. Eur J Nutr. 2018;57:2735–2745. doi:10.1007/s00394-017-1539-3.
- Wang W, Degroote J, Van Ginneken C, Van Poucke M, Vergauwen H, Dam TM, Vanrompay D, Peelman LJ, Smet SD, Michiels J . Intrauterine growth restriction in neonatal piglets affects small intestinal mucosal permeability and mRNA expression of redox-sensitive genes. FASEB Journal: Official Publication of the Federation of American Societies for Experimental Biology. 2016;30:863–873. doi:10.1096/fj.15-274779.
- Shen L, Gan M, Zhang S, Ma J, Tang G, Jiang Y, Li M, Wang J, Li X, Che L . Transcriptome analyses reveal adult metabolic syndrome with intrauterine growth restriction in pig models. Front Genet. 2018;9:291. doi:10.3389/fgene.2018.00291.
- Shen L, Zhang S, Li Q, Fu Y, Tang G, Jiang Y, Li, M, Wang, J, Li, X, Che, L . The landscape of non-coding rna in an adult pig model of intrauterine growth restriction. Cellular Physiology and Biochemistry: International Journal of Experimental Cellular Physiology, Biochemistry, and Pharmacology. 2018;50:1764–1778. doi:10.1159/000494794.
- Bjuland KJ, Rimol LM, Lohaugen GC, Skranes J. Brain volumes and cognitive function in very-low-birth-weight (VLBW) young adults. European Journal of Paediatric Neurology: EJPN: Official Journal of the European Paediatric Neurology Society. 2014;18:578–590. doi:10.1016/j.ejpn.2014.04.004.
- Jensen CB, Storgaard H, Madsbad S, Richter EA, Vaag AA, Bjuland KJ, Rimol LM, Løhaugen GCC, Skranes J. Altered skeletal muscle fiber composition and size precede whole-body insulin resistance in young men with low birth weight. J Clin Endocrinol Metab. 2007;92:1530–1534. doi:10.1210/jc.2006-2360.
- Longhi S, Mercolini F, Carloni L, Nguyen L, Fanolla A, Radetti G. Prematurity and low birth weight lead to altered bone geometry, strength, and quality in children. J Endocrinol Invest. 2015;38:563–568. doi:10.1007/s40618-014-0230-2.
- Tao S, Bai Y, Li T, Li N, Wang J. Original low birth weight deteriorates the hindgut epithelial barrier function in pigs at the growing stage. FASEB Journal: Official Publication of the Federation of American Societies for Experimental Biology. 2019;33:9897–9912. doi:10.1096/fj.201900204RR.
- Ashida H, Ogawa M, Kim M, Mimuro H, Sasakawa C. Bacteria and host interactions in the gut epithelial barrier. Nat Chem Biol. 2011;8:36–45. doi:10.1038/nchembio.741.
- Li N, Huang S, Jiang L, Wang W, Li T, Zuo B, Li Z, Wang J. Differences in the gut microbiota establishment and metabolome characteristics between low- and normal-birth-weight piglets during early-life. Front Microbiol. 2018;9:1798. doi:10.3389/fmicb.2018.01798.
- Tao S, Xiong Y, Wang Z, Wu Y, Li N, Pi Y, Han D, Zhao J, Wang J. N -Acyl-homoserine lactones may affect the gut health of low-birth-weight piglets by altering intestinal epithelial cell barrier function and amino acid metabolism. J Nutr. 2021. doi:10.1093/jn/nxab104.
- Kim YS, Ho SB. Intestinal goblet cells and mucins in health and disease: recent insights and progress. Curr Gastroenterol Rep. 2010;12:319–330. doi:10.1007/s11894-010-0131-2.
- Johansson ME, Sjovall H, Hansson GC. The gastrointestinal mucus system in health and disease. Nat Rev Gastroenterol Hepatol. 2013;10:352–361. doi:10.1038/nrgastro.2013.35.
- Ermund A, Schutte A, Johansson ME, Gustafsson JK, Hansson GC. Studies of mucus in mouse stomach, small intestine, and colon. I. Gastrointestinal mucus layers have different properties depending on location as well as over the Peyer’s patches. Am J Physiol Gastrointest Liver Physiol. 2013;305:G341–7. doi:10.1152/ajpgi.00046.2013.
- Holmen Larsson JM, Thomsson KA, Rodriguez-Pineiro AM, Karlsson H, Hansson GC. Studies of mucus in mouse stomach, small intestine, and colon. III. gastrointestinal Muc5ac and Muc2 mucin O-glycan patterns reveal a regiospecific distribution. Am J Physiol Gastrointest Liver Physiol. 2013;305:G357–63. doi:10.1152/ajpgi.00048.2013.
- Rodriguez-Pineiro AM, Bergstrom JH, Ermund A, Gustafsson JK, Schutte A, Johansson ME, Hansson, GC . Studies of mucus in mouse stomach, small intestine, and colon. II. Gastrointestinal mucus proteome reveals Muc2 and Muc5ac accompanied by a set of core proteins. Am J Physiol Gastrointest Liver Physiol. 2013;305:G348–56. doi:10.1152/ajpgi.00047.2013.
- Johansson ME, Gustafsson JK, Holmen-Larsson J, Jabbar KS, Xia L, Xu H, Ghishan FK, Carvalho FA, Gewirtz AT, Sjövall H, et al. Bacteria penetrate the normally impenetrable inner colon mucus layer in both murine colitis models and patients with ulcerative colitis. Gut. 2014;63:281–291. doi:10.1136/gutjnl-2012-303207.
- McElroy SJ, Prince LS, Weitkamp JH, Reese J, Slaughter JC, Polk DB. Tumor necrosis factor receptor 1-dependent depletion of mucus in immature small intestine: a potential role in neonatal necrotizing enterocolitis. Am J Physiol Gastrointest Liver Physiol. 2011;301:G656–66. doi:10.1152/ajpgi.00550.2010.
- der Sluis M V, De Koning BA, De Bruijn AC, Velcich A, Meijerink JP, Van Goudoever JB, Büller HA, Dekker J, Van Seuningen I, Renes IB, et al. Muc2-deficient mice spontaneously develop colitis, indicating that MUC2 is critical for colonic protection. Gastroenterology. 2006;131:117–129. doi:10.1053/j.gastro.2006.04.020.
- Tao S, Niu L, Cai L, Geng Y, Hua C, Ni Y, Zhao R . N-(3-oxododecanoyl)-l-homoserine lactone modulates mitochondrial function and suppresses proliferation in intestinal goblet cells. Life Sci. 2018;201:81–88.
- Tao S, Luo Y, Bin H, Liu J, Qian X, Ni Y, Zhao R. Paraoxonase 2 modulates a proapoptotic function in LS174T cells in response to quorum sensing molecule N-(3-oxododecanoyl)-L-homoserine lactone. Sci Rep. 2016;6:28778. doi:10.1038/srep28778.
- John LJ, Fromm M, Schulzke JD. Epithelial barriers in intestinal inflammation. Antioxid Redox Signal. 2011;15:1255–1270. doi:10.1089/ars.2011.3892.
- Gareau MG, Silva MA, Perdue MH. Pathophysiological mechanisms of stress-induced intestinal damage. Curr Mol Med. 2008;8:274–281. doi:10.2174/156652408784533760.
- Ballard ST, Hunter JH, Taylor AE. Regulation of tight-junction permeability during nutrient absorption across the intestinal epithelium. Annu Rev Nutr. 1995;15:35–55. doi:10.1146/annurev.nu.15.070195.000343.
- Schneeberger EE, Lynch RD. The tight junction: a multifunctional complex. Am J Physiol Cell Physiol. 2004;286:C1213–28. doi:10.1152/ajpcell.00558.2003.
- Vikstrom E, Tafazoli F, Magnusson KE. Pseudomonas aeruginosa quorum sensing molecule N-(3 oxododecanoyl)-l-homoserine lactone disrupts epithelial barrier integrity of Caco-2 cells. FEBS Lett. 2006;580:6921–6928. doi:10.1016/j.febslet.2006.11.057.
- Tao S, Xiong Y, Han D, Pi Y, Zhang H, Wang J. N -(3-oxododecanoyl)- -homoserine lactone disrupts intestinal epithelial barrier through triggering apoptosis and collapsing extracellular matrix and tight junction. J Cell Physiol. 2021;236(8):5771–5784. doi:10.1002/jcp.30261.
- Cheng W, Wang Z, Xiong Y, Wu Z, Tan X, Yang Y, Zhang H, Zhu X, Wei H, Tao S, et al. N-(3-oxododecanoyl)-homoserine lactone disrupts intestinal barrier and induces systemic inflammation through perturbing gut microbiome in mice. Sci Total Environ. 2021;778:146347. doi:10.1016/j.scitotenv.2021.146347.