ABSTARCT
Enterohemorrhagic Escherichia coli (EHEC) O157:H7 is a human pathogen that causes a variety of diseases, such as hemorrhagic colitis and lethal hemolytic uremic syndrome. Flagellum-dependent motility plays diverse roles in the pathogenesis of EHEC O157:H7, including its migration to an optimal host site, adherence and colonization, survival at the infection site, and post-infection dispersal. However, it is very expensive for cellular economy in terms of the number of genes and the energy required for flagellar biosynthesis and functioning. Furthermore, the flagellar filament bears strong antigenic properties that induce a strong host immune response. Consequently, the flagellar gene expression and biosynthesis are highly regulated to occur at the appropriate time and place by different regulatory influences. The present review focuses on the regulatory mechanisms of EHEC O157:H7 motility and flagellar biosynthesis, especially in terms of flagellar gene regulation by environmental factors, regulatory proteins, and small regulatory RNAs.
Introduction
Enterohemorrhagic Escherichia coli (EHEC) O157:H7 is a foodborne pathogen that causes severe diseases, including diarrhea, hemorrhagic colitis (HC), and hemolytic uremic syndrome (HUC).Citation1,Citation2,Citation3 It has three major virulence factors, including Shiga toxins (Stxs), products of the pathogenicity island called the locus of enterocyte effacement (LEE), and products of the large virulence plasmid pO157.Citation4 Stxs are bacteriophage-encoded AB5 family toxins that cause damage to a variety of cell types and have often been associated with HC and the lethal hemolytic uremic syndrome (HUS) in humans.Citation5 LEE consists of five polycistronic operons (LEE1 to LEE5) encoding a type III secretion system and associated effectors and enables the bacteria to intimately adhere to host epithelial cells.Citation4 pO157, a highly conserved non-conjugated plasmid with a size ranging from 92 to 104 kb,Citation6 is known to harbor several genes involved in the pathogenesis of EHEC O157:H7 infections, including etpC-etpO, ehxA, toxB, katP, espP, and stcE .Citation7
The bacterial flagellum is a macromolecular machine that consists of a basal body (rotary motor), a hook (universal joint), and a filament (propeller).Citation8 Flagellar-mediated motility confers an important advantage for bacteria in moving toward favorable conditions or in avoiding detrimental environments and allows bacteria to pursue nutrients and to reach and maintain their preferred niches for survival.Citation9 In addition to having locomotive properties, flagellum-mediated motility plays diverse roles in the pathogenesis and progression of EHEC O157:H7 infection. Upon entering the host intestine, EHEC O157:H7 relies on flagellum-mediated motility to reach and adhere to optimal colonization sites in the host.Citation10 Subsequently, EHEC O157:H7 inhibits flagellar biosynthesis to save energy and minimize host immunity ().Citation10
Figure 1. Flagellar regulation during enterohemorrhagic Escherichia coli (EHEC) O157:H7 interaction with the host cells. Upon entering the host intestine, flagellum-mediated motility enables EHEC O157:H7 to reach and adhere to optimal colonization sites in the host. After successful infection, EHEC O157:H7 inhibits flagellar biosynthesis to minimize unnecessary energy expenditure and host immune response.
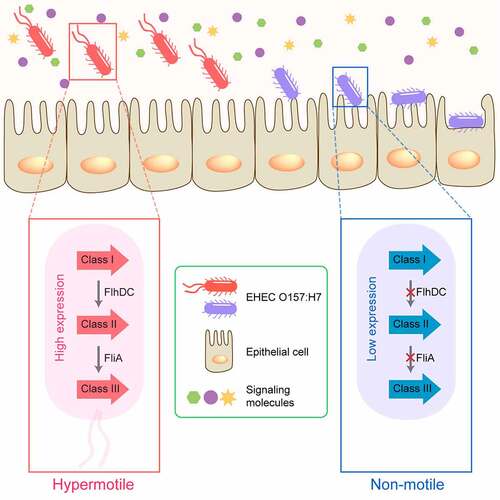
Flagellar biosynthesis is an energy-intensive process requiring 2% of the cell’s biosynthetic resources and flagellar rotation consumes 0.1% of the cell’s energy.Citation11 More than 50 genes constituting at least 14 operons are involved in the biosynthesis and assembly of a flagellum.Citation12,Citation13 These flagellar genes are divided into three hierarchical classes (Class I, II, and III) based on their timing and mode of expression ().Citation14 The Class I gene flhDC encodes the master flagellar regulator, with FlhD and FlhC forming a heterotetramer (FlhD4C2).Citation14 Class II genes, including fliFGHIJK, fliLMNOPQR, fliE, flhBAE, flgBCDEFGHIJ, fliAZY, and flgAMN, encode structural and assembly proteins required for the biosynthesis of the hook-basal body and a pair of flagellar regulatory proteins, FliA and FlgM ().Citation13,Citation15 Class III genes, including flgKL, fliDST, flgMN, fliC, tar-tap-cheRBYZ, and motAB-cheAW, encode cell-distal structural components of the flagellum and flagellar function (rotation and chemotaxis) ().Citation14,Citation16 The expression of flagellar genes is tightly regulated, with FlhDC, FliA, and FlgM playing major regulatory roles in the flagellar transcription network. Flagellar biosynthesis is also regulated by various environmental signals, regulatory proteins, and small RNAs (sRNA) by modulating flhDC and/or fliA transcription or translation. In the present review, we provide a broad overview of the regulatory mechanisms of flagellar biosynthesis and motility in EHEC O157:H7, especially focusing on the regulatory function of environmental factors, transcriptional regulatory proteins, post-transcriptional and post-translation regulatory proteins, and small regulatory RNAs on flagellar gene expression at both transcriptional and translational levels.
Figure 2. Flagellar genes and structure of EHEC O157:H7. (a) The genetically defined hierarchy of flagellar genes. More than 50 flagellar genes are arranged in a regulatory cascade of three classes (Class I, II, and III). The Class I gene flhDC encodes the master regulator of Class II genes. Class II genes are responsible for the synthesis of hook-basal body and two competing regulatory proteins (FlgM and FliA) of Class III genes, and Class III genes are involved in the synthesis of complete flagellum and chemotaxis system. (b) Schematic showing the major components of the flagella (basal body, hook, and filament) and the location of the flagellar gene products.
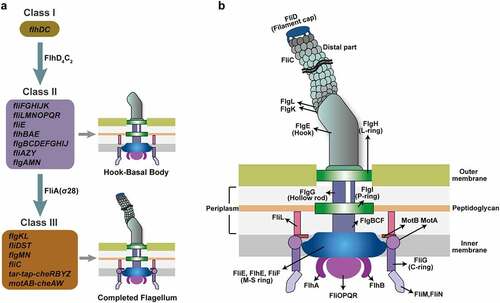
Three-tiered flagellar regulatory cascade
In E. coli, including EHEC O157:H7, the expression of flagellar genes is a tightly regulated and highly energetic three-tier process ().Citation12 The Class I gene flhDC encodes proteins FlhD and FlhC, that assemble into the heterohexamer (FlhD4C2).Citation14 The FlhD4C2 proteins complex binds to the DNA upstream of Class II genes, recruits RNA polymerase, and promotes σ70-dependent transcription ().Citation17 FliA is an alternate sigma factor (σ28), encoded by a Class II gene, specifically required for transcription initiation of Class III genes.Citation18 FlgM acts as an anti-sigma factor that binds to FliA directly, preventing interaction with RNA polymerase and repressing FliA-dependent transcription until after hook-basal body are formed.Citation19 Upon assembly of the basal body and secretion apparatus, FlgM is exported out of the cell, freeing FliA and allowing initiation of FliA-dependent transcription from Class III promoters.Citation20 This three-tiered flagellar regulatory cascade helps bacteria, including EHEC O157:H7, to conserve biosynthetic resources and ensure the efficiency of flagellar assembly.
Figure 3. Three-tiered flagellar regulatory cascade in EHEC O157:H7. In the three-tiered flagellar regulatory cascade, FlhDC promotes σ70-dependent transcription of Class II genes, FliA acts as an alternative sigma factor (σ28) specifically required for the transcription of Class III genes, and FlgM acts as an anti-sigma factor that binds to FliA and inhibits its activity.
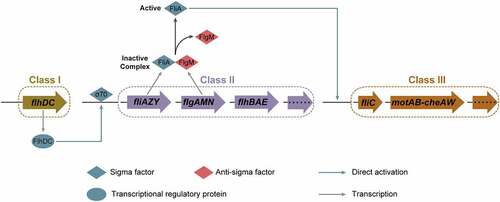
Environmental factors affecting EHEC O157:H7 motility and flagellar biosynthesis
EHEC O157:H7 can survive and persist in varied environments, such as soil, water, and food, as well as in host gastrointestinal tract,Citation21 for which it requires the ability to adapt to variations or extreme changes in conditions commonly encountered in nature. Flagellum-mediated motility allows EHEC O157:H7 to move away from stressful environments and to reach environments with optimal nutrients for survival.Citation9 The motility and flagellar biosynthesis of EHEC O157:H7 are affected by diverse environmental factors that include short-chain fatty acids (SCFAs), mucin, bile salts, epinephrine/norepinephrine, indole, and mechanical cues ( and ).
Figure 4. Regulation of flagellar gene expression by environmental factors in EHEC O157:H7. Environmental signals, including butyrate, mucin, bile salts, epinephrine/norepinephrine (EPI/NE), mechanical cue, temperature, pH, osmolarity, and DNA and ammonium concentrations, affect EHEC O157:H7 flagellar motility and biosynthesis.
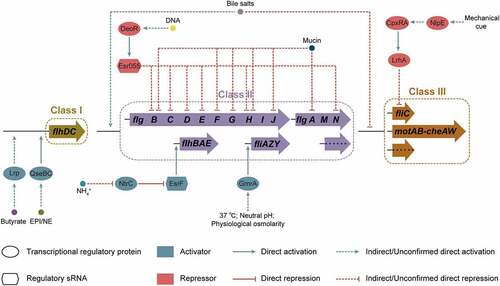
Table 1. Regulation of EHEC O157:H7 flagellar motility and biosynthesis by environmental factors, regulatory proteins and regulatory sRNAs.
Short-chain fatty acids
Short-chain fatty acids (SCFAs) are fermentation end-products of dietary fiber metabolism by gastrointestinal microorganisms.Citation58 They are mainly composed of acetic, propionic, and butyric acids, with acetic acid being the most abundant.Citation59 The concentration and composition of SCFAs in the gastrointestinal tract are uneven, being lower in the small intestine (20–40 mM) and higher in the large intestine (70–200 mM).Citation22 The expression of flagellar genes, including flhC and flhD (the master regulatory genes), motility genes (motAB) and chemotaxis gene (cheA), and bacterial motility in EHEC O157:H7 were significantly upregulated when exposed to SCFA mixture representative of the small intestine but were downregulated upon exposure to SCFA mixtures representative of the large intestine.Citation22 In addition, exposure to 20 mM butyrate, a concentration similar to that of the large intestine SCFA, was reported to enhance the expression of flagellar genes in EHEC O157:H7.Citation23 Butyrate, but not acetate and propionate, activated the flhDC regulatory genes through the leucine-responsive regulatory protein Lrp.Citation23 However, butyrate, acetate, and propionate also activated downstream flagellar genes independently of flhDC activation. Therefore, precise regulation of motility and flagellar biosynthesis in response to SCFAs provides EHEC O157:H7 with a profound advantage in transiting through the host gastrointestinal tract, permitting the pathogen to avoid detrimental locations and to find more favorable niches.Citation23
Mucin
The gastrointestinal tract is lined by a continuously secreted mucus layer formed mainly by high-molecular mass (200–2000 kDa) oligomeric mucin glycoproteins.Citation24 Mucins act as receptors for bacterial adhesin proteins and provide protection against the adherence of infectious pathogens by steric hindrance or through specific bacterial binding domains.Citation60,Citation61 A whole genome-scale transcriptome analysis revealed that 732 candidate genes were differentially expressed in EHEC O157:H7 in the presence of 0.5% porcine stomach mucin.Citation24 Of particular interest, eight genes associated with flagellar biosynthesis (including flgA, flgB, flgF, flgG, flgH, flgJ, fliP, and yaiU) were all downregulated in the presence of mucin. Furthermore, qRT-PCR analyses showed that the expression of six genes in the flg operon (i.e., flgABFGH and flgJ) was downregulated two- to five-folds in the presence of mucin, which further verified the transcriptome analysis results. Consistently, the bacterial motility was also significantly reduced when EHEC O157:H7 was grown on 0.3% tryptone agar plates containing mucin. Therefore, mucin may act as an intestinal environmental signal that negatively regulates EHEC O157:H7 motility through the transcriptional repression of the flg gene that encodes the components of the flagellar basal body.Citation24
Bile salts
Bile salts are the main component of human liver bile, with their concentration ranging from 0.2% to 2% in the small intestine.Citation62 EHEC O157:H7 encounters acute acid stress and broken bile during its passage through the gastrointestinal tract.Citation63 A transcriptome analysis revealed that bile salts induce an unusual effect on the transcript levels of flagellar genes.Citation25 The transcript levels of the regulatory genes/operons (flhDC, flgMN, and fliAZY) controlling flagellar biosynthesis remained relatively unchanged under bile salt treatment compared to that in untreated controls. However, flagellar genes encoding the components of the basal body-hook structure, including flgA, fliLMNOPQR, fliE, fliFGHIJK, flgBCDEFGHIJ, and flhBAE, had two- to four-folds increased transcription levels in the bile-salt treated cells. In contrast to the basal body-related genes, other flagellar genes, including fliC, fliDST, flgKL, tar-tap-cheZYBR, and motAB-cheAW, which encode the elements of the flagellar filament, motor stator, and chemotaxis system had approximately twofold reduction in their transcription levels. A significant increase in EHEC O157:H7 motility was observed when 0.8% or 0.1% bile salts were incorporated into the soft agar medium.Citation25 However, incorporating bile salts into the soft agar destabilized it, making it less robust. Therefore, the change in motility may be attributed to the differences in the viscosity of the soft agar with and without the addition of bile salts.Citation25 Nevertheless, the mechanism by which EHEC O157:H7 senses bile salts to regulate flagellar gene expression remains unclear, and the effect of bile salts on EHEC O157:H7 motility needs further experimental verification.
Epinephrine/norepinephrine
Quorum sensing is a cell-to-cell signaling system based on the production of hormone-like compounds known as autoinducers (AI),Citation64 which allow bacteria to detect their own population as well as the population of other bacterial species present in the same environment.Citation64 The host-derived stress hormones epinephrine (EPI) and norepinephrine (NE) are autoinducer analogs that are recognized by the quorum sensing system and are involved in the regulation of flagellar-mediated motility in EHEC O157:H7.Citation26,Citation27 The two-fluorophore chemotaxis assay showed a concentration-dependent migration of EHEC O157:H7 toward EPI and NE.Citation27 Furthermore, addition of 50 µM EPI or NE to motility agar plates significantly increased EHEC O157:H7 motility, compared to that in the untreated control.Citation27 The regulation of EHEC O157:H7 motility by EPI/NE is mediated by the two-component regulatory system QseBC,Citation26 which will be discussed later in the review.
Indole
Indole, produced by both commensal and pathogenic strains of E. coli, is an important signaling molecule in host‒microbe interactions in the gastrointestinal tract.Citation65 Because commensal E. coli produce as much as 600 μM indole in suspension cultures,Citation66 and indole has been detected in human feces at comparable concentrations (~250–1100 μM),Citation67 it is likely that EHEC O157:H7 are continually exposed to high concentrations of indole in the human intestinal tract. An agarose plug chemotaxis assay showed that EHEC O157:H7 is repelled by indole.Citation27 In addition, a high concentration of indole (500 μM) decreased EHEC O157:H7 motility by 2.8-fold.Citation27 However, the mechanisms by which EHEC O157:H7 senses indole as an environmental signal to regulate bacterial motility are still largely unknown.
Mechanical cue
Motility and biofilm development are mutually exclusive events, and the phase of transition from motility to sessile is determined by a sensory transduction mechanism termed surface sensing, which typically involves bacterial flagella.Citation68 As a mechanosensor, flagella play a critical role in surface sensing and the initial stages of surface adhesion.Citation69 Once attached to a surface, bacteria respond by producing cyclic diguanylate monophosphate (c-diGMP), a second messenger signaling molecule that drives the transition between the free-living and biofilm lifestyle.Citation70 EHEC O157:H7 perceives attachment to hydrophobic glass beads and host cells as a mechanical cue that leads to downregulated expression of the flagellin gene (fliC).Citation28 This mechanical cue-mediated flagellar gene regulation is mediated by NlpE, CpxRA, and LrhA, which will be discussed later in the review.
Transcriptional regulatory proteins affecting EHEC O157:H7 motility and flagellar biosynthesis
EHEC O157:H7 encounters various conditions when colonizing the host intestinal tract or surviving in the external environment.Citation71 To ensure its successful survival and colonization, flagellar genes are subjected to strict regulation that allows their expression only under optimal environmental conditions while also avoiding intense metabolic cost or alerting the host immune system.Citation10 The coordinated expression of flagellar genes is mainly controlled by transcriptional regulatory proteins, which precisely activate or repress particular genes depending on environmental factors and the stage of infection.Citation72 These transcriptional regulatory proteins include GmrA, PchE, Z5898, QseBC, Hha, Tol-Pal system, GrlA, FmrA, IHF, SdiA, CadA, AdhE, RcsB, NlpE-CpxRA-LrhA, and MraW ( and ).
Figure 5. Regulation of flagellar gene expression by transcriptional regulatory proteins in EHEC O157:H7. Transcriptional regulatory proteins, including GmrA, PchE, Z5898, Hha, QseBC, Tol‒Pal system, GrlA, FmrA, IHF, SdiA, CadA, AdhE, RcsB, NlpE‒CpxRA‒LrhA and MraW, affect EHEC O157:H7 flagellar motility and biosynthesis.
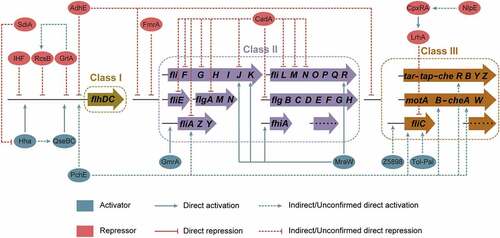
GmrA
Genomic island OI-29 in EHEC O157:H7 is a 2643-bp island that contains the z0638, z0639 (named gmrA), and z0640 genes, which encode hypothetical proteins of unknown function.Citation73 The gmrA gene encodes a transcriptional activator required for motility and flagellar biosynthesis in EHEC O157:H7.Citation29 A ΔgmrA mutant was found to have significantly reduced flagellar biosynthesis and motility on semi-solid LB agar as well as suppressed transcriptional and translational expression of flagellar genes in comparison to the wild-type and complemented strains of EHEC O157:H7. Conversely, the motility and transcriptional expression of flagellar genes were not affected by the deletion of z0638 and z0640. Electrophoretic mobility shift assays (EMSAs), competition experiments, and chromatin immunoprecipitation quantitative PCR (ChIP-qPCR) analysis revealed that GmrA directly binds to the promoter of fliA, an RNA polymerase sigma factor, and thereby regulates flagellar genes controlled by FliA. The expression of gmrA is maximal in conditions similar to those in the intestinal tract (37°C, neutral pH, and physiological osmolarity) and in the presence of host epithelial cells, indicative of the motility-promoting role of this gene in infection.Citation29 A bioinformatics analysis of 231 available E. coli genome sequences showed that gmrA is highly conserved and widely distributed in various E. coli lineages.Citation29 In addition, almost all strains with gmrA are important pathogens in human or animals, indicating that these hosts might have driven the acquisition of gmrA during the evolution of those pathogenic strains. Finally, GmrA was found to be a widespread regulator of bacterial motility and flagellar synthesis in different pathotypes of E. coli (including EHEC, enteropathogenic E. coli, neonatal meningitis-associated E. coli, and avian pathogenic E. coli).
PchE
Five EHEC O157:H7 homologs of the EPEC perC gene (pchA to pchE) have been studied extensively, and a clear role of pchA, pchB, and pchC in the control of LEE through regulation of ler has been demonstrated.Citation74 However, pchE had little effect on the LEE operons,Citation74 and its role in regulating bacterial motility and flagellar gene expression has been recently discovered.Citation30 The transcription of flhC and flhD, which directly or indirectly control the expression of the flagellar operons, were increased >2-fold by pchE overexpression in EHEC O157:H7.Citation30 In addition, genes in different flagellar operons, including chemotaxis, motor, structural, and regulatory genes, were upregulated by pchE overexpression. The expression levels of the fliA and fliC genes, encoding the RNA polymerase σ28 and the flagellar subunit flagellin, respectively, increased by 12- to 24-folds. The expression levels of the motor and chemotaxis genes tested (motB, cheA, and cheR) were also markedly enhanced by approximately five- to nine-folds. In accordance, the plasmid overexpression of pchE increased the diameter of the EHEC O157:H7 motility zone by nearly 50%. However, although pchE stimulates flagellar motility, it reduces the adhesion of EHEC O157:H7 to HEP-2 cells, which likely facilitates the movement of EHEC O157:H7 to the intestinal surface in an early stage of infection.Citation30
Z5898
OI-172 is a 44 434-bp genomic island that contains 27 open reading frames (ORFs) encoding several presumed RNA helicases, including a DEAH box RNA helicase (Z5898).Citation31,Citation73 A comparative proteomic analysis of wild-type EHEC O157:H7 and Δz5898 mutant showed that flagellin was significantly downregulated in the Δz5898 mutant.Citation31 A motility assay showed that Δz5898 mutant migrated slower than the wild-type EHEC O157:H7 and transmission electron microscopy found that approximately 90% Δz5898 mutant have no surface flagella. Z5898 promotes fliC expression at the transcription level by increasing the fliC promoter activity, as indicated by a qRT-PCR analysis and a promoter activity assay that measures green fluorescent protein production. In contrast, the fliA transcripts and its promoter activity were not affected by the deletion of z5898. Therefore, Z5898 regulates the flagella-based motility by exerting its effect on fliC but not its upstream regulator gene, fliA. However, the precise mechanisms by which Z5898 activates fliC expression are still unclear. Considering Z5898 is an RNA helicase, it is possible that Z5898 is involved in processing fliC transcript to increase its expression, which needs further investigation.Citation31
QseBC
The two-component regulatory system sensor protein QseC senses EPI/NE and causes autophosphorylation.Citation42 This phosphate is subsequently transferred to the cellular response regulator QseB, and the signals are transmitted to the genome for the differential regulation of signal-sensitive genes. An isogenic qseC mutation in EHEC O157:H7 resulted in reduced flagellin expression and motility compared with the wild-type and complemented strains.Citation43 The qseC mutant showed decreased transcription of flhD, fliA, motA, and fliC, suggesting that qseBC is involved in the transcriptional regulation of flagellar genes. QseBC activates the transcription of flhDC, which is the master regulator of the flagella and motility genes, and, in the absence of flhD, QseBC is unable to activate the transcription of fliA. Further, EMSAs, competition experiments, and DNaseI footprinting analyses showed that the phosphorylated QseB positively regulates EHEC O157:H7 flagellar gene expression by directly interacting with the flhDC regulatory region at the distal high-affinity site and the proximal low-affinity binding sites.Citation44
Hha
Hha is a nucleus-associated protein that controls gene expression through DNA structure and by regulating its topology according to osmotic pressure and temperature.Citation75 The swimming motility and expression of the flagellar gene fliC were significantly reduced in an isogenic Δhha mutant than in the wild-type EHEC O157:H7.Citation32 Hha regulates flagellar gene expression by inducing FlhDC, as demonstrated by reduced flhD transcription in the Δhha mutant. EMSA results showed that the purified Hha directly binds to the promoter of flhDC. The contribution and hierarchy of Hha and QseBC in controlling EHEC O157:H7 motility were also compared. The motility of the Δhha ΔqseC double mutant was highly and significantly compromised when compared to the motility phenotypes expressed by the Δhha or ΔqseC single mutants.Citation33 Complementation with qseBC-carrying plasmid increased the motility of the Δhha ΔqseC double mutant by a smaller magnitude than complementation of this mutant with the hha-carrying plasmid.Citation33 These results indicated that Hha exerts greater control than QseBC in regulating the motility of EHEC O157:H7. In addition, Hha is hierarchically superior in the transcriptional regulation of motility than QseBC because the transcription of qseC is significantly reduced in the Δhha mutant than that in the wild-type and complemented strains of EHEC O157:H7. Therefore, Hha can regulate EHEC O157:H7 motility and flagellar biosynthesis directly as well as indirectly by controlling the transcription of qseBC.Citation33
Tol‒Pal system
The Tol‒Pal system is a protein complex, which was originally characterized by E. coli K-12,Citation76 and is involved in outer membrane maintenance and uptake of colicin and filamentous phage DNA.Citation77 The Tol-Pal system consists of TolQ, TolR, TolA, TolB, and Pal proteins.Citation78 In EHEC O157:H7, the inactivation of tolA, which encodes the periplasm spanning protein, resulted in complete loss of motility and concomitant transcriptional repression of the major flagellar subunit encoding gene, fliC.Citation45 Complementation of tolA on a low-copy plasmid restored motility and fliC transcription to that of wild-type. Similar to the ΔtolA mutant, the ΔtolB mutant in EHEC O157:H7 also exhibited reduced motility on semi-solid agar compared with the wild-type parent, and the introduction of a heterologous tolB expression plasmid increased its motility up to the level of the wild-type parent.Citation46 Furthermore, EHEC O157:H7 ΔtolB mutant produced defective flagella as determined by microscopy using a 100× objective lens. Consistent with this phenomenon, the western blotting results showed that the flagellin protein, encoded by fliC, was undetectable in ΔtolB mutant, indicating that the deletion of tolB in EHEC O157:H7 decreases FliC levels, which in turn leads to the reduction of flagellar production and motility.Citation46 Similarly, the Δpal mutant strain was also less motile and produced fewer flagella than the wild-type EHEC O157:H7 strain.Citation46 Thus, these results suggest that the activity of the Tol‒Pal system contributes to EHEC O157:H7 motility and flagellar biosynthesis, although the underlying mechanism is still unclear.
GrlA
GrlA and GrlR, which are encoded within the LEE, are positive and negative regulators, respectively, of LEE gene.Citation4,Citation79 Notably, GrlR is an anti-GrlA factor that inhibits its function by interacting with GrlA.Citation80 The GrlR‒GrlA regulatory system not only regulates the expression of LEE genes but also EHEC O157:H7 motility and flagellar biosynthesis.Citation34 Both FliC protein levels and bacterial motility were significantly reduced in the ΔgrlR mutant compared with that in EHEC O157:H7 wild-type strain.Citation34 In accordance, the overexpression of grlA from a multicopy plasmid strongly represses FliC expression and EHEC O157:H7 motility, indicating that GrlA acts as a negative regulator of flagellar gene expression. Mechanically, GrlA negatively regulates flagellar gene expression by reducing the transcription of the flhDC operon in EHEC O157:H7. The constitutively expressed FlhD/FlhC complex inhibits efficient adhesion of EHEC O157:H7 to HeLa cells; therefore, the GrlA-dependent repression of the flagellar regulon may be important for efficient cell adhesion of EHEC O157:H7 to host cells.Citation34
FmrA
Genomic island OI-1 is a putative fimbria-encoding genomic island that contains six ORFs from z0020 to z0025.Citation35,Citation73 The deletion of z0021, also known as fmrA (Fimbrial-Operon-encoded motility regulator A), leads to higher swimming motility and flagellar biosynthesis in EHEC O157:H7 than in the wild-type strain, while the overexpression of fmrA inhibits motility.Citation35 In contrast, the deletion of other ORFs within OI-1 had no effect on EHEC O157:H7 motility, as the migration distances of the Δz0020 and Δz0022‒z0025 mutants in motility agar plates were comparable to that of wild-type EHEC O157:H7. Transcriptional reporter assays showed that FmrA exerted its regulatory effects prior to Class II and Class III flagellar gene transcription but downstream of the transcription and translation of flhDC. Furthermore, the motility defects caused by FmrA can be suppressed by increasing flhDC expression, thus implying a genetic link between FmrA and the FlhDC regulatory complexes. It is, therefore, conceivable that FmrA could regulate flagellar biosynthesis by interacting with the FlhDC complex and preventing it from binding to the promoters of Class II flagellar genes.Citation35
Integration host factor (IHF)
IHF is a DNA-binding protein that binds to specific consensus sites and bends the DNA to form nucleoprotein complexes.Citation81 IHF is a heterodimer composed of IHF-α and IHF-β subunits that are encoded by the ihfA and ihfB genes, respectively.Citation81 IHF is involved in various cellular processes, including site-specific recombination, DNA replication, transposition, packaging, and gene regulation.Citation81 In EHEC O157:H7 and EPEC O127:H6, the ihfA::kan mutant was highly motile, and the motility of the strains expressing IHF, either genomic or in trans from a plasmid, was attenuated.Citation36 Electron micrographs of the wild-type cells and the ihfA::kan mutant grown in Dulbecco’s modified Eagle medium (DMEM) showed that the wild-type cells either completely lack flagella or rarely possess (approximately 5% of bacteria) a single flagellum. In contrast, the ihfA::kan mutant produced numerous peritrichous flagella. The IHF-mediated repression of flagellar expression involves the silencing of flhDC, which encodes a positive regulator of the flagellar regulon. IHF indirectly represses flhDC via a putative regulator that is not encoded by LEE.Citation36 In addition, IHF-mediated repression of flhDC is unique to EPEC and EHEC and is not found in all E. coli strains, such as E. coli K-12.Citation36
SdiA
In most quorum-sensing systems, LuxR is required as a transcriptional factor that recognizes and binds to specific QS signaling molecules called autoinducers.Citation82 EHEC O157:H7 contains a LuxR homologue called SdiA, which has a helical‒angular‒helical DNA-binding motif.Citation37 The overexpression of SdiA from a high-copy-number plasmid significantly reduces the motility of EHEC O157:H7.Citation37 A qRT-PCR analysis revealed that the fliC expression in the ΔsdiA mutant was significantly increased relative to the wild-type EHEC O157:H7.Citation38 In contrast, fliC expression in the Δhha mutant was reduced compared with that in the wild-type strain, verifying Hha as a positive regulator of bacterial motility. In addition, the ability of the ΔsdiA Δhha double mutant to express fliC gene showed no significant difference from that of the Δhha single mutant. In other words, sdiA deletion had no effect on EHEC O157 fliC gene expression in a Δhha mutant background. Therefore, the regulatory role of SdiA for fliC gene expression in EHEC O157:H7 is mediated by Hha.Citation38
CadA
The cadA gene encodes a lysine decarboxylase that plays a role in EHEC O157:H7 motility and flagellar biosynthesis.Citation39 A gene array analysis revealed that 1464 genes (132 upregulated and 1332 downregulated) were differentially regulated by more than twofolds (with 99% confidence) in the ΔcadA mutant versus the wild-type strain.Citation39 Interestingly, among these most upregulated genes, 16 genes, including fliA, flgABCDEFGH, and fliEFGJLMN, are involved in flagellar biosynthesis and motility. The qRT-PCR results confirmed the elevated expression of flagellar basal body and hook-encoding genes flgB and flgE, in concordance with the microarray results. In accordance, an analysis of multiple electron microscopic fields revealed that the ΔcadA mutant strain possesses more flagellar filaments than the wild-type strain grown under the same conditions. Interestingly, the motilities of both wild-type EHEC O157:H7 and ΔcadA mutant strains were similar on motility agar plates.Citation39 The regulatory mechanisms of CadA on EHEC O157:H7 flagellar biosynthesis remain unclear. Considering that most upregulated flagellar genes in ΔcadA mutant are under the control of FliA, and the expression of fliA was also significantly increased in the ΔcadA mutant, the regulation of flagellar genes by CadA may be dependent on FliA.
AdhE
AdhE is a metabolic enzyme that has the dual enzymatic functions of converting acetyl-CoA to acetaldehyde and acetaldehyde to ethanol.Citation83 An RNA-sequence analysis revealed that the deletion of adhE in EHEC O157:H7 significantly upregulates all three flagellar gene classes, including the regulatory genes (flhC, flhD, and fliA), structural genes (such as fliF, flgE, and fliC), and genes encoding chemotaxis machinery (such as cheA and cheY) and energy transduction systems (motA and motB).Citation40 In accordance with these results, the SDS-PAGE, tandem mass spectrometry (MS), and immunoblotting analyses showed that the ΔadhE mutant exhibited increased expression of FliC, the major structural subunit of the flagellar filament, and FliA, the flagellar-specific sigma factor required for fliC transcription. The immunofluorescence microscopy of FliC revealed that the ΔadhE mutant with increased levels of FliC exhibited multiple intact flagellar filaments on its surface. Instead, the ΔadhE mutant was unable to migrate through semi-solid agar, indicating that the assembled flagella did not rotate. The authors speculated that the assembled flagella are nonfunctional either because a key flagellar component is misassembled and/or some flagellar component is inappropriately modified.Citation40
RcsB
The Rcs phosphorelay system consists of RcsC, RcsB, and RcsA, which encode a sensor kinase, a response regulator, and an auxiliary regulatory protein, respectively.Citation84 The Rcs phosphorelay system first identified by its role in the transcriptional regulation of the capsular polysaccharide synthesis genes in E. coli.Citation85 In addition to capsule synthesis, the Rcs system plays an important role in the regulation of motility and flagellar biosynthesis in EHEC O157:H7.Citation41 Compared with that in the wild-type strain, the deletion of rcsB enhanced the lateral growth of EHEC O157:H7 on motility plates by 30–40%.Citation41 Negative regulation of motility by RcsB has been shown to result from direct transcriptional repression of the global regulator of flagellar biosynthesis and motility genes flhDC in E. coli K-12.Citation86 Consistent with this, the expression of the flhD promoter, as measured by β-galactosidase activity, was significantly increased in EHEC O157:H7 ΔrcsB mutant.Citation41 Furthermore, the RcsB-dependent repression of flhDC and motility is positively regulated by GrlA at the post-transcriptional level.Citation41
NlpE‒CpxRA‒LrhA
EHEC O157:H7 can adhere to a large variety of surfaces, including glass, metals, many different polymers, as well as to other bacteria and eukaryotic cells.Citation87 When EHEC O157:H7 binds to surfaces, the outer-membrane lipoprotein NlpE senses a mechanical cue generated by surface attachment and signals to the sensor kinase CpxA at the inner membrane.Citation28 CpxA undergoes autophosphorylation, after which the phosphate group is transferred to the cytoplasmic response regulator CpxR. The phosphorylated CpxR binds directly to the lrhA promoter and consequently activates the expression of lrhA. LrhA, in turn, indirectly represses the expression of the fliC gene. Considering that the LrhA protein bound specifically to the flhD promoter in E. coli K-12,Citation88 and flhC expression was increased by the overexpression of LrhA in EHEC O157:H7,Citation28 the downregulation of flagellin expression in EHEC O157:H7 in response to adherence to hydrophobic glass beads and host cells might be attributed to the LrhA-induced increase in the levels of FlhDC.Citation28
MraW
MraW is a 16S rRNA methyltransferase that plays an important role in gene regulation through DNA methylation.Citation89 It was recently found to contribute to the motility and flagellar production of EHEC O157:H7.Citation47 The motility of ΔmraW mutant was significantly decreased in correlation with a decrease in the production or secretion of FliC, as determined by western blotting using anti-H7 antisera.Citation47 Consistent with decreased motility and flagellin secretion in the ΔmraW mutant, the majority (95%) of the ΔmraW mutant have no flagella, with the remaining 5% of the cells having one to two flagella. In contrast, most of EHEC O157:H7 wild-type strains possess one to three surface flagella. Whole-genome bisulfite sequencing showed that two flagellar genes (fliJ and fliR) and two flagellar gene promoters (fliK and fhiA) had lower levels of methylation in the ΔmraW mutant compared to the wild-type strain. Furthermore, MraW has been demonstrated to directly bind to these four flagellar gene sequences and increase methylation levels, which in turn promote gene expression.Citation47
Post-transcriptional and post-translational regulatory proteins affecting EHEC O157:H7 motility and flagellar biosynthesis
In addition to transcriptional regulation, post-transcriptional and post-translational regulation play a key role in coordinating flagellar gene expression networks.Citation72 Post-transcriptional regulation is highly versatile and adaptable; it controls RNA availability in cellular time and space.Citation90 Messenger RNA stability, transport, storage, and translation are largely determined by the interaction of mRNA with post-transcriptional regulatory proteins and sRNAs.Citation91,Citation92 Post-translational regulation controls the biochemical alteration of proteins involving generally reversible covalent modification or irreversible processing to regulate their activity, location, or stability.Citation93,Citation94 Post-transcriptional and post-translational regulatory proteins known to regulate EHEC O157:H7 motility and flagellar biosynthesis include CsrA, ClpXP, and Hfq ( and ).
Figure 6. Regulation of flagellar gene expression by post-transcriptional and post-translational regulatory proteins and regulatory small regulatory RNAs (sRNAs) in EHEC O157:H7. Post-transcriptional and post-translational regulatory proteins affecting EHEC O157:H7 motility and flagellar biosynthesis include CsrA, ClpXP, and Hfq. Regulatory sRNAs affecting EHEC O157:H7 flagellar motility and biosynthesis include EsrF, Esr055, Esr41, and MavR.
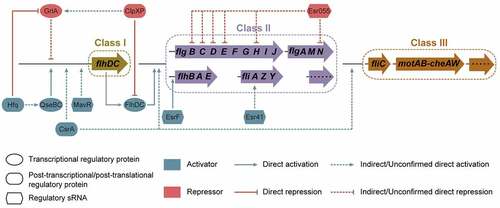
CsrA
CsrA is a well-documented RNA-binding protein that post-transcriptionally controls numerous genes and processes.Citation95,Citation96 The homodimeric protein CsrA regulates gene expression post-transcriptionally by binding to sites containing the AGGA/ANGGA motif in the leader segment of transcripts to alter their stability and/or translation.Citation97 Although the mechanism through which CsrA regulates target gene expression is well documented, the full regulatory impact of CsrA on cellular activities in EHEC O157:H7 is not fully understood. We recently investigated the global effects of CsrA in EHEC O157:H7 using RNA-seq technology, and found that the transcript levels of 53 flagella-related genes were significantly decreased in the csrA::kan mutant compared to those in the EHEC O157:H7 wild-type strain.Citation48 These downregulated genes comprise both genes required for the synthesis and assembly of flagella as well as transcriptional regulators of flagellar gene expression, such as flhD, flhC, flgM, and fliA. Subsequent motility assays confirmed that the csrA::kan mutant was non-motile on semi-solid agar plates, and the motility defect was rescued by complementation with a low copy number plasmid harboring csrA. As expected, the overexpression of csrA in EHEC O157:H7 resulted in enhanced bacterial motility. Previous study demonstrated that the CsrA protein of E. coli K-12 binds directly to the 5′ segment of flhDC mRNA to increase its steady-state level and half-life.Citation98 As in E. coli K-12, csrA disruption in EHEC O157:H7 also results in significant downregulation of flhDC gene expression, suggesting that these two strains might share similar motility regulatory mechanisms.Citation48
ClpXP
ClpXP protease is an ATP-dependent bipartite protease responsible for the degradation of some key regulatory proteins (such as RpoS) and aberrant translation products.Citation99,Citation100 ClpXP consists of a ClpP protein degradation component and a ClpX component that binds the substrate protein.Citation101 In EHEC O157:H7, both FlhD and FlhC proteins accumulated markedly following ClpXP depletion, and their half-lives were significantly longer in the mutant cells, suggesting that ClpXP causes the degradation of FlhD and FlhC proteins, leading to the downregulation of flagellar gene expression.Citation49 In addition, ClpXP negatively regulates the transcription of the flhD promoter through the GrlR‒GrlA system under conditions in which LEE gene expression is induced. Therefore, the ClpXP protease regulates EHEC O157:H7 motility and flagellar gene expression through two pathways, namely post-translational degradation of the FlhD/FlhC master regulator and transcriptional control of the flhDC operon through the LEE-encoded GrlR‒GrlA regulatory system.Citation49
Hfq
The RNA-binding protein Hfq is a global post-transcriptional regulator that is essential for the fitness and virulence of an increasing number of bacterial pathogens.Citation102 Hfq promotes interactions between an sRNA and its target mRNA to regulate gene expression; however, Hfq can also function independently by influencing polyadenylation or translation of mRNAs.Citation103 Transcriptome and qRT-PCR analyses showed that the deletion of hfq in EHEC O157:H7 led to decreased expression of the two-component system qseBC,Citation50 which is involved in the transcriptional activation of flagellar genes.Citation43,Citation44 In contrast, Hfq post-transcriptionally represses the expression of grlA that encodes a negative regulator of flagellar gene transcription.Citation51 In vitro analyses revealed that the Hfq distal face directly binds near the translational initiation site of the grlA mRNA and inhibits its translation in an sRNA-independent manner in EHEC O157:H7.Citation52 It is therefore conceivable that Hfq can indirectly activate EHEC O157:H7 motility and flagellar gene expression through post-transcriptional regulation of qseBC and grlA.
sRNA affecting EHEC O157:H7 motility and flagellar biosynthesis
Bacterial small regulatory RNAs (sRNAs) are generally untranslated RNA sequences ranging from 50 to 300 nucleotides in length.Citation104 The majority of sRNAs exert their regulatory effects by binding to target mRNAs, which in turn affect the transcriptional elongation, stability, or translation of mRNAs.Citation104 sRNAs have been demonstrated to co-regulate numerous biological processes, including motility, quorum sensing, biofilm formation, stress response, and virulence.Citation105 To date, four sRNAs, including EsrF, Esr055, Esr41, and MavR, have been identified to be involved in the regulation of EHEC O157:H7 motility and flagellar biosynthesis ( and ).
EsrF
In our recent work, the sRNA EsrF was identified in a screen for differentially expressed genes/sRNAs from EHEC O157:H7 attached to HeLa cells compared with EHEC O157:H7 grown in DMEM.Citation106 EsrF is 85 nucleotides in length and located in an intergenic region between ntrB and glnA.Citation53 esrF promoted the expression of several flagellar genes including flhB, flhD, flhC, fliA, and fliC, which resulted in enhanced EHEC O157:H7 motility.Citation53 Among these EsrF-regulated flagellar genes, flhB was identified as the direct target of EsrF, which directly binds to the 5′-untranslated region (UTR) of the flhB mRNA, which may release the Rho-dependent termination of the flhB 5′-UTR, resulting in increased flhB expression. A 9-nucleotide crucial motif (5′-GGGAUUUAG-3′) is vital to the ability of EsrF to bind to flhB mRNA. Further EMSAs and DNase I footprinting analyses revealed that the nitrogen regulatory protein NtrC directly bound to an 18-base pair motif (5′-CACGGATGAAGCGATCGA-3′; located at −106 to −89 from the esrF translational start site) within the esrF promoter region to repress the expression of esrF.Citation53 Furthermore, qRT-PCR analysis revealed that the transcription level of flhB was significantly increased in ∆ntrC mutant compared to that in the wild-type and complemented strains. Collectively, NtrC senses high ammonium concentrations in the colon to release the inhibitory effect on esrF, thereby enhancing its expression and subsequently promoting EHEC O157:H7 motility and colonization.Citation53
Esr055
Similar to the process of discovering esrF, we identified the sRNA Esr055 in genomic island OI-93 by re-analyzing our previous transcriptomic data for EHEC O157:H7 during the infection of HeLa cells.Citation106 Esr055 is located in an intergenic region between z3342 and stx1B, which encode a 9-O-acetyl-N-acetylneuraminic acid deacetylase and the B subunit of Stx 1, respectively.Citation54 The expression of Esr055 is directly activated by the regulator, DeoR, and its expression is positively affected by DNA, which is significantly more abundant in the ileum than in the colon.Citation54 Transcriptome analysis revealed that the expression of six flagellar genes, including flgB, flgC, flgD, flgE, flgH, and flgN, were significantly upregulated in the Δesr055 mutant compared with that in the wild-type EHEC O157:H7.Citation54 The regulation of these flagellar genes by Esr055 indicated by the transcriptome data was further confirmed by a qRT-PCR analysis. However, the mechanism of how these flagellar genes are regulated by Esr055 remains unclear.
Esr41
Esr41 (EHEC O157 small RNA #41) is a 70-nt-long sRNA, with a 3′ GC-rich palindrome sequence followed by a long poly(U),Citation55 which is essential for functional Hfq-binding.Citation107 EHEC O157:H7 harboring a high-copy-number plasmid carrying the esr41 gene showed increased bacterial motility on agar plates and fliC expression at both transcriptional and translational levels.Citation55 The enhancement of Esr41-induced bacterial motility was also observed in E. coli K-12, indicating that target genes controlled by Esr41 are conserved and present in both EHEC O157:H7 and E. coli K-12. Further studies found that Esr41 activated the expression of Class III flagellar genes by indirectly inducing the transcription of fliA and thereby enhanced EHEC O157:H7 motility.Citation56 Although direct targets of Esr41 remain unknown, Esr41 seems to upregulate fliA transcription via a transcriptional regulator whose encoding gene is targeted by Esr41.Citation56 FlhDC is the major transcriptional activator responsible for fliA expression, and a possible base-pairing region is located between Esr41 and the 5′-UTR of the flhDC mRNA.Citation55 However, Esr41 does not target flhD because the deletion of the possible base-pairing region had no effect on flhD expression.Citation55 The direct targets of Esr41 involved in regulating EHEC O157:H7 motility and flagellar biosynthesis remain unidentified and need further investigation.
MavR
MavR (Metabolic and virulence regulatory factor) is a ∼370-bp Hfq-dependent sRNA located within the genomic island OI-48 in the reverse orientation of genes encoding tellurite resistance (ter).Citation57,Citation73 Bioinformatics analyses revealed that MavR is conserved in diarrheagenic E. coli as well as in other pathogenic Enterobacteriaceae but is absent in nonpathogenic bacteria.Citation57 RNA-seq analyses revealed that the expression of nearly every gene that encodes flagellar biosynthesis or chemotaxis was decreased in the ΔmavR mutant compared to wild-type EHEC O157:H7 during aerobic growth.Citation57 The RNA-seq results were further confirmed by a qRT-PCR analysis of four differentially expressed flagellar genes, including flhD, fliK, fliC, and cheA. Further analyses found that MavR promotes flagellar/chemotaxis gene expression by influencing FlhD expression post-transcriptionally. Consistent with the gene expression results, the motility of the ΔmavR mutant on semi-solid LB agar was slightly decreased compared to that of wild-type EHEC O157:H7, and this difference could be rescued upon complementation.Citation57
Conclusions and future perspectives
Flagellum-dependent motility plays an essential role in EHEC O157:H7 colonization and pathogenesis in the host intestinal tract.Citation108,Citation109 However, flagellar protein synthesis comes at a cost to the bacterium in terms of growth rate and fitness within the host.Citation110 Furthermore, flagellin is a potent antigen that induces an immune response by eliciting the secretion of proinflammatory chemokines in human intestinal epithelial cells.Citation111 Therefore, flagellar gene expression must be tightly regulated to occur at the appropriate time and place during different stages of infection.Citation72 EHEC O157:H7 bacteria have evolved diverse regulatory mechanisms to control the expression of flagellar genes in response to specific intestinal environmental cues, including transcriptional regulatory proteins, post-transcriptional and post-translational regulatory proteins, and regulatory sRNAs. During early infection, EHEC O157:H7 upregulates flagellar genes in response to intestinal environmental signals, ultimately increasing bacterial flagellar biosynthesis and motility.Citation10 Subsequently, the increased motility enables the pathogen to reach and adhere to colonization sites in the host intestine. After successful infection, flagellum-dependent motility becomes less critical, and EHEC O157:H7 then downregulates flagellar gene expression to inhibit flagellar biosynthesis, not only to save energy but also to minimize host immunity.Citation10 This finely tuned strategy allows EHEC O157:H7 to precisely control the timing of activation of flagellar genes at the intended site of action, maximizing its virulence and pathogenicity. Nevertheless, only a few intestinal environmental signals affecting flagellar gene expression have been identified thus far, and the precise regulatory mechanisms remain largely unknown. Thus, whether other intestinal environmental cues in the human intestine elicit flagellar biosynthesis remains to be established in future studies.
A comparative genomic analysis showed that EHEC O157:H7 genome contained 177 genomic islands, termed O islands (OIs) that are not present in the genome of nonpathogenic E. coli K-12.Citation73 These islands comprise the main known virulence elements of EHEC O157:H7, including LEE pathogenicity island (OI-148) and Shiga toxin-converting phages (OI-45 and OI-93).Citation2,Citation112 The functions of several other islands have also been established in recent years, revealing more regulatory proteins and sRNAs associated with EHEC O157:H7 motility and flagellar biosynthesis. The gmrA gene on OI-29 and z5898 on OI-172 were found to encode the transcriptional activators of flagellar biosynthesis and bacterial motility, while fmrA on OI-1 was found to encode a repressor.Citation29,Citation31,Citation35 In addition, of the four known sRNAs that regulate EHEC O157:H7 motility and flagellar biosynthesis, two are located on OIs, with Esr055 on OI-93 and MavR on OI-48.Citation54,Citation57 Nevertheless, the majority of OI genes have not been assigned a function, and their effect on EHEC O157 motility and flagellar biosynthesis is still largely unknown. Moreover, the majority of current studies have mainly focused on investigating the relationship between core genome-encoded regulatory factors and the flagellar biosynthesis of EHEC O157:H7. In contrast, the studies on the functions of OI-encoded regulatory factors associated with flagellar biosynthesis have been relatively limited. Therefore, future studies should focus on the effect of OI-associated genes on bacterial motility and flagellar biosynthesis, which might facilitate an improved understanding of the mechanisms of EHEC O157:H7 pathogenicity and adaptation to different environmental conditions.
Diarrheal diseases caused by EHEC O157:H7 are an important public health problem worldwide. Outbreak surveillance data from the Center for Disease Control and Prevention (CDC) reported that EHEC O157:H7 causes more than 73 000 illnesses, 2100 hospitalizations, and 60 deaths annually in the United States.Citation113 The annual cost of illness due to E. coli O157:H7 infections, including lost productivity, medical care, and premature deaths, was 405 million dollars.Citation113 Intestinal tract infections caused by EHEC O157:H7 cannot be treated effectively by current antibiotic therapies because the antibiotics are either ineffective, cause severe dysbiosis of the intestinal microbiota, or trigger serious complications, such as HUS from antibiotic-induced Stxs release.Citation114,Citation115 Thus, new strategies must be employed to develop safe and effective antimicrobial therapies for the treatment of EHEC O157:H7 infections. Considering that flagellum-mediated motility plays diverse roles in EHEC O157:H7 pathogenesis and the deletion of many genes encoding regulatory proteins and sRNAs would significantly decrease EHEC O157:H7 motility and flagellar biosynthesis, these genes may hence be used as potential targets for the development of novel therapeutics for the treatment of EHEC O157:H7 infections.
Disclosure statement
No potential conflict of interest was reported by the author(s).
Additional information
Funding
References
- Munns KD, Selinger LB, Stanford K, Guan L, Callaway TR, McAllister TA. Perspectives on super-shedding of Escherichia coli O157:H7 by cattle. Foodborne Pathog Dis. 2015;12(2):89‒103. doi:10.1089/fpd.2014.1829.
- Jiang L, Yang W, Jiang X, Yao T, Wang L, Yang B. Virulence-related O islands in enterohemorrhagic Escherichia coli O157:H7. Gut Microbes. 2021;13(1):1992237. doi:10.1080/19490976.2021.1992237.
- Liu Y, Han R, Wang J, Yang P, Wang F, Yang B, Boyle JP. Magnesium sensing regulates intestinal colonization of enterohemorrhagic Escherichia coli O157:H7. mBio. 2020;11(6):e02470–21. doi:10.1128/mBio.02470-20.
- Connolly JP, Finlay BB, Roe AJ. From ingestion to colonization: the influence of the host environment on regulation of the LEE encoded type III secretion system in enterohaemorrhagic Escherichia coli. Front Microbiol. 2015;6:568. doi:10.3389/fmicb.2015.00568.
- Bryan A, Youngster I, McAdam AJ. Shiga toxin producing Escherichia coli. Clin Lab Med. 2015;35(2):247‒272. doi:10.1016/j.cll.2015.02.004.
- Lim JY, La HJ, Sheng H, Forney LJ, Hovde CJ. Influence of plasmid pO157 on Escherichia coli O157:H7 Sakai biofilm formation. Appl Environ Microbiol. 2010;76(3):963‒966. doi:10.1128/AEM.01068-09.
- Yoon JW, Hovde CJ. All blood, no stool: enterohemorrhagic Escherichia coli O157:H7 infection. J Vet Sci. 2008;9(3):219‒231. doi:10.4142/jvs.2008.9.3.219.
- Duan Q, Zhou M, Zhu L, Zhu G. Flagella and bacterial pathogenicity. J Basic Microbiol. 2013;53(1):1‒8. doi:10.1002/jobm.201100335.
- Colin R, Ni B, Laganenka L, Sourjik V. Multiple functions of flagellar motility and chemotaxis in bacterial physiology. FEMS Microbiol Rev. 2021;45(6):fuab038. doi:10.1093/femsre/fuab038.
- Mahajan A, Currie CG, Mackie S, Tree J, McAteer S, McKendrick I, McNeilly TN, Roe A, La Ragione RM, Woodward MJ, et al. An investigation of the expression and adhesin function of H7 flagella in the interaction of Escherichia coli O157:H7 with bovine intestinal epithelium. Cell Microbiol. 2009;11(1):121‒137. doi:10.1111/j.1462-5822.2008.01244.x.
- Fontaine F, Stewart EJ, Lindner AB, Taddei F. Mutations in two global regulators lower individual mortality in Escherichia coli. Mol Microbiol. 2008;67(1):2‒14. doi:10.1111/j.1365-2958.2007.05988.x.
- Chilcott GS, Hughes KT. Coupling of flagellar gene expression to flagellar assembly in Salmonella enterica serovar typhimurium and Escherichia coli. Microbiol Mol Biol Rev. 2000;64(4):694‒708. doi:10.1128/MMBR.64.4.694-708.2000.
- Kalir S, McClure J, Pabbaraju K, Southward C, Ronen M, Leibler S, Surette MG, Alon U. Ordering genes in a flagella pathway by analysis of expression kinetics from living bacteria. Science. 2001;292(5524):2080‒2083. doi:10.1126/science.1058758.
- Khan F, Tabassum N, Pham DTN, Oloketuyi SF, Kim YM. Molecules involved in motility regulation in Escherichia coli cells: a review. Biofouling. 2020;36(8):889‒908. doi:10.1080/08927014.2020.1826939.
- Kutsukake K, Ohya Y, Iino T. Transcriptional analysis of the flagellar regulon of Salmonella typhimurium. J Bacteriol. 1990;172(2):741‒747. doi:10.1128/jb.172.2.741-747.1990.
- Osterman IA, Dikhtyar YY, Bogdanov AA, Dontsova OA, Sergiev PV. Regulation of flagellar gene expression in bacteria. Biochemistry (Mosc). 2015;80(11):1447‒1456. doi:10.1134/S000629791511005X.
- Liu X, Matsumura P. The FlhD/FlhC complex, a transcriptional activator of the Escherichia coli flagellar class II operons. J Bacteriol. 1994;176(23):7345‒7351. doi:10.1128/jb.176.23.7345-7351.1994.
- Ohnishi K, Kutsukake K, Suzuki H, Iino T. Gene fliA encodes an alternative sigma factor specific for flagellar operons in Salmonella typhimurium. Mol Gen Genet. 1990;221(2):139‒147. doi:10.1007/BF00261713.
- Chadsey MS, Karlinsey JE, Hughes KT. The flagellar anti-sigma factor FlgM actively dissociates Salmonella typhimurium sigma28 RNA polymerase holoenzyme. Genes Dev. 1998;12(19):3123‒3136. doi:10.1101/gad.12.19.3123.
- Hughes KT, Gillen KL, Semon MJ, Karlinsey JE. Sensing structural intermediates in bacterial flagellar assembly by export of a negative regulator. Science. 1993;262(5137):1277‒1280. doi:10.1126/science.8235660.
- Ongeng D, Geeraerd AH, Springael D, Ryckeboer J, Muyanja C, Mauriello G. Fate of Escherichia coli O157:H7 and Salmonella enterica in the manure-amended soil-plant ecosystem of fresh vegetable crops: a review. Crit Rev Microbiol. 2015;41(3):273‒294. doi:10.3109/1040841X.2013.829415.
- Lackraj T, Kim JI, Tran SL, Barnett Foster DE. Differential modulation of flagella expression in enterohaemorrhagic Escherichia coli O157:H7 by intestinal short-chain fatty acid mixes. Microbiol (Reading). 2016;162(10):1761‒1772. doi:10.1099/mic.0.000357.
- Tobe T, Nakanishi N, Sugimoto N, Bliska JB. Activation of motility by sensing short-chain fatty acids via two steps in a flagellar gene regulatory cascade in enterohemorrhagic Escherichia coli. Infect Immun. 2011;79(3):1016‒1024. doi:10.1128/IAI.00927-10.
- Kim JC, Yoon JW, Kim CH, Park MS, Cho SH. Repression of flagella motility in enterohemorrhagic Escherichia coli O157:H7 by mucin components. Biochem Biophys Res Commun. 2012;423(4):789‒792. doi:10.1016/j.bbrc.2012.06.041.
- Hamner S, McInnerney K, Williamson K, Franklin MJ, Ford TE, Ahmed N. Bile salts affect expression of Escherichia coli O157:H7 genes for virulence and iron acquisition, and promote growth under iron limiting conditions. PLoS One. 2013;8(9):e74647. doi:10.1371/journal.pone.0074647.
- Moreira CG, Sperandio V. The epinephrine/norepinephrine/autoinducer-3 interkingdom signaling system in Escherichia coli O157:H7. Adv Exp Med Biol. 2016;874:247‒261. doi:10.1007/978-3-319-20215-0_12.
- Bansal T, Englert D, Lee J, Hegde M, Wood TK, Jayaraman A. Differential effects of epinephrine, norepinephrine, and indole on Escherichia coli O157:H7 chemotaxis, colonization, and gene expression. Infect Immun. 2007;75(9):4597‒4607. doi:10.1128/IAI.00630-07.
- Shimizu T, Ichimura K, Noda M, Payne SM. The surface sensor NlpE of enterohemorrhagic Escherichia coli contributes to regulation of the type III secretion system and flagella by the Cpx response to adhesion. Infect Immun. 2016;84(2):537‒549. doi:10.1128/IAI.00881-15.
- Yang B, Wang S, Huang J, Yin Z, Jiang L, Hou W, Li X, Feng L. Transcriptional activator GmrA,encoded in genomic island OI-29, controls the motility of enterohemorrhagic Escherichia coli O157:H7. Front Microbiol. 2018;9:338. doi:10.3389/fmicb.2018.00338.
- Andreozzi E, Uhlich GA. PchE regulation of Escherichia coli O157:H7 flagella, controlling the transition to host cell attachment. Int J Mol Sci. 2020;21(13):4592. doi:10.3390/ijms21134592.
- Xu Y, Xu X, Lan R, Xiong Y, Ye C, Ren Z, Liu L, Zhao A, Wu LF, Xu J. An O island 172 encoded RNA helicase regulates the motility of Escherichia coli O157:H7. PLoS One. 2013;8(6):e64211. doi:10.1371/journal.pone.0064211.
- Sharma VK, Bearson BL. Hha controls Escherichia coli O157:H7 biofilm formation by differential regulation of global transcriptional regulators FlhDC and CsgD. Appl Environ Microbiol. 2013;79(7):2384‒2396. doi:10.1128/AEM.02998-12.
- Sharma VK, Casey TA, Ibekwe AM. Determining the relative contribution and hierarchy of hha and qseBC in the regulation of flagellar motility of Escherichia coli O157:H7. PLoS One. 2014;9(1):e85866. doi:10.1371/journal.pone.0085866.
- Iyoda S, Koizumi N, Satou H, Lu Y, Saitoh T, Ohnishi M, Watanabe H. The GrlR-GrlA regulatory system coordinately controls the expression of flagellar and LEE-encoded type III protein secretion systems in enterohemorrhagic Escherichia coli. J Bacteriol. 2006;188(16):5682‒5692. doi:10.1128/JB.00352-06.
- Allison SE, Silphaduang U, Mascarenhas M, Konczy P, Quan Q, Karmali M, Coombes BK. Novel repressor of Escherichia coli O157:H7 motility encoded in the putative fimbrial cluster OI-1. J Bacteriol. 2012;194(19):5343‒5352. doi:10.1128/JB.01025-12.
- Yona-Nadler C, Umanski T, Aizawa SI, Friedberg D, Rosenshine I. Integration host factor (IHF) mediates repression of flagella in enteropathogenic and enterohaemorrhagic Escherichia coli. Microbiol (Reading). 2003;149:877‒884. doi:10.1099/mic.0.25970-0.
- Kanamaru K, Kanamaru K, Tatsuno I, Tobe T, Sasakawa C. SdiA, an Escherichia coli homologue of quorum-sensing regulators, controls the expression of virulence factors in enterohaemorrhagic Escherichia coli O157:H7. Mol Microbiol. 2000;38(4):805‒816. doi:10.1046/j.1365-2958.2000.02171.x.
- Sharma VK, Bearson SMD, Bearson BL. Evaluation of the effects of sdiA, a luxR homologue, on adherence and motility of Escherichia coli O157:H7. Microbiol (Reading). 2010;156:1303‒1312. doi:10.1099/mic.0.034330-0.
- Vazquez-Juarez RC, Kuriakose JA, Rasko DA, Ritchie JM, Kendall MM, Slater TM, Sinha M, Luxon BA, Popov VL, Waldor MK, et al. CadA negatively regulates Escherichia coli O157:H7 adherence and intestinal colonization. Infect Immun. 2008;76(11):5072‒5081. doi:10.1128/IAI.00677-08.
- Beckham KS, Connolly JP, Ritchie JM, Wang D, Gawthorne JA, Tahoun A, Gally DL, Burgess K, Burchmore RJ, Smith BO, et al. The metabolic enzyme AdhE controls the virulence of Escherichia coli O157:H7. Mol Microbiol. 2014;93(1):199‒211. doi:10.1111/mmi.12651.
- Morgan JK, Vendura KW, Stevens SM, Riordan JT. RcsB determines the locus of enterocyte effacement (LEE) expression and adherence phenotype of Escherichia coli O157:H7 spinach outbreak strain TW14359 and coordinates bicarbonate-dependent LEE activation with repression of motility. Microbiol (Reading). 2013;159:2342‒2353. doi:10.1099/mic.0.070201-0.
- Parker CT, Russell R, Njoroge JW, Jimenez AG, Taussig R, Sperandio V, Silhavy TJ. Genetic and mechanistic analyses of the periplasmic domain of the enterohemorrhagic Escherichia coli QseC histidine sensor kinase. J Bacteriol. 2017;199(8):e00861–16. doi:10.1128/JB.00861-16.
- Sperandio V, Torres AG, Kaper JB. Quorum sensing Escherichia coli regulators B and C (QseBC): a novel two-component regulatory system involved in the regulation of flagella and motility by quorum sensing in E. coli. Mol Microbiol. 2002;43(3):809‒821. doi:10.1046/j.1365-2958.2002.02803.x.
- Clarke MB, Sperandio V. Transcriptional regulation of flhDC by QseBC and sigma (FliA) in enterohaemorrhagic Escherichia coli. Mol Microbiol. 2005;57(6):1734‒1749. doi:10.1111/j.1365-2958.2005.04792.x.
- Morgan JK, Ortiz JA, Riordan JT. The role for TolA in enterohemorrhagic Escherichia coli pathogenesis and virulence gene transcription. Microb Pathog. 2014;77:42‒52. doi:10.1016/j.micpath.2014.10.010.
- Hirakawa H, Suzue K, Takita A, Awazu C, Kurushima J, Tomita H. Roles of the Tol-Pal system in the Type III secretion system and flagella-mediated virulence in enterohemorrhagic Escherichia coli. Sci Rep. 2020;10(1):15173. doi:10.1038/s41598-020-72412-w.
- Xu X, Zhang H, Huang Y, Zhang Y, Wu C, Gao P, Teng Z, Luo X, Peng X, Wang X, et al. Beyond a ribosomal RNA methyltransferase, the wider role of MraW in DNA methylation, motility and colonization in Escherichia coli O157:H7. Front Microbiol. 2019;10:2520. doi:10.3389/fmicb.2019.02520.
- Wang S, Yang F, Yang B. Global effect of CsrA on gene expression in enterohemorrhagic Escherichia coli O157:H7. Res Microbiol. 2017;168(8):700‒709. doi:10.1016/j.resmic.2017.08.003.
- Kitagawa R, Takaya A, Yamamoto T. Dual regulatory pathways of flagellar gene expression by ClpXP protease in enterohaemorrhagic Escherichia coli. Microbiol (Reading). 2011;157:3094‒3103. doi:10.1099/mic.0.051151-0.
- Kendall MM, Gruber CC, Rasko DA, Hughes DT, Sperandio V. Hfq virulence regulation in enterohemorrhagic Escherichia coli O157:H7 strain 86-24. J Bacteriol. 2011;193(24):6843‒6851. doi:10.1128/JB.06141-11.
- Hansen AM, Kaper JB. Hfq affects the expression of the LEE pathogenicity island in enterohaemorrhagic Escherichia coli. Mol Microbiol. 2009;73(3):446‒465. doi:10.1111/j.1365-2958.2009.06781.x.
- Sudo N, Lee K, Sekine Y, Ohnishi M, Iyoda S. RNA-binding protein Hfq downregulates locus of enterocyte effacement-encoded regulators independent of small regulatory RNA in enterohemorrhagic Escherichia coli. Mol Microbiol. 2022;117(1):86‒101. doi:10.1111/mmi.14799.
- Jia T, Liu B, Mu H, Qian C, Wang L, Li L, Lu G, Zhu W, Guo X, Yang B, et al. A novel small RNA promotes motility and virulence of enterohemorrhagic Escherichia coli O157:H7 in response to ammonium. mBio. 2021;12(2):e03605–20. doi:10.1128/mBio.03605-20.
- Han R, Xu L, Wang T, Liu B, Wang L. A small regulatory RNA contributes to the preferential colonization of Escherichia coli O157:H7 in the large intestine in response to a low DNA concentration. Front Microbiol. 2017;8:274. doi:10.3389/fmicb.2017.00274.
- Sudo N, Soma A, Muto A, Iyoda S, Suh M, Kurihara N, Abe H, Tobe T, Ogura Y, Hayashi T, et al. A novel small regulatory RNA enhances cell motility in enterohemorrhagic Escherichia coli. J Gen Appl Microbiol. 2014;60(1):44‒50. doi:10.2323/jgam.60.44.
- Sudo N, Soma A, Iyoda S, Oshima T, Ohto Y, Saito K, Sekine Y. Small RNA Esr41 inversely regulates expression of LEE and flagellar genes in enterohaemorrhagic Escherichia coli. Microbiol (Reading). 2018;164(5):821‒834. doi:10.1099/mic.0.000652.
- Sauder AB, Kendall MM. A pathogen-specific sRNA influences enterohemorrhagic Escherichia coli fitness and virulence in part by direct interaction with the transcript encoding the ethanolamine utilization regulatory factor EutR. Nucleic Acids Res. 2021;49(19):10988‒11004. doi:10.1093/nar/gkab863.
- Cummings JH, Macfarlane GT. The control and consequences of bacterial fermentation in the human colon. J Appl Bacteriol. 1991;70(6):443‒459. doi:10.1111/j.1365-2672.1991.tb02739.x.
- Dalile B, Van Oudenhove L, Vervliet B, Verbeke K. The role of short-chain fatty acids in microbiota-gut-brain communication. Nat Rev Gastroenterol Hepatol. 2019;16(8):461‒478. doi:10.1038/s41575-019-0157-3.
- Ringot-Destrez B, Kalach N, Mihalache A, Gosset P, Michalski JC, Leonard R, Robbe-Masselot C. How do they stick together? Bacterial adhesins implicated in the binding of bacteria to the human gastrointestinal mucins. Biochem Soc Trans. 2017;45(2):389‒399. doi:10.1042/BST20160167.
- Ye J, Song L, Liu Y, Pan Q, Zhong X, Li S, Shang Y, Tian Y, He Y, Chen L, et al. Core 2 mucin-type O-glycan is related to EPEC and EHEC O157:H7 adherence to human colon carcinoma HT-29 epithelial cells. Dig Dis Sci. 2015;60(7):1977‒1990. doi:10.1007/s10620-015-3548-5.
- Gunn JS. Mechanisms of bacterial resistance and response to bile. Microbes Infect. 2000;2(8):907‒913. doi:10.1016/s1286-4579(00)00392-0.
- Begley M, Gahan CG, Hill C. The interaction between bacteria and bile. FEMS Microbiol Rev. 2005;29(4):625‒651. doi:10.1016/j.femsre.2004.09.003.
- Mukherjee S, Bassler BL. Bacterial quorum sensing in complex and dynamically changing environments. Nat Rev Microbiol. 2019;17(6):371‒382. doi:10.1038/s41579-019-0186-5.
- Bansal T, Alaniz RC, Wood TK, Jayaraman A. The bacterial signal indole increases epithelial-cell tight-junction resistance and attenuates indicators of inflammation. Proc Natl Acad Sci U S A. 2010;107(1):228‒233. doi:10.1073/pnas.0906112107.
- Domka J, Lee J, Wood TK. YliH (BssR) and YceP (BssS) regulate Escherichia coli K-12 biofilm formation by influencing cell signaling. Appl Environ Microbiol. 2006;72(4):2449‒2459. doi:10.1128/AEM.72.4.2449-2459.2006.
- Karlin DA, Mastromarino AJ, Jones RD, Stroehlein JR, Lorentz O. Fecal skatole and indole and breath methane and hydrogen in patients with large bowel polyps or cancer. J Cancer Res Clin Oncol. 1985;109(2):135‒141. doi:10.1007/BF00391888.
- Belas R. Biofilms, flagella, and mechanosensing of surfaces by bacteria. Trends Microbiol. 2014;22(9):517‒527. doi:10.1016/j.tim.2014.05.002.
- Belas R. When the swimming gets tough, the tough form a biofilm. Mol Microbiol. 2013;90(1):1‒5. doi:10.1111/mmi.12354.
- Armbruster CR, Lee CK, Parker-Gilham J, de Anda J, Xia A, Zhao K, Murakami K, Tseng BS, Hoffman LR, Jin F, et al. Heterogeneity in surface sensing suggests a division of labor in Pseudomonas aeruginosa populations. Elife. 2019;8. doi:10.7554/eLife.45084.
- Durso LM, Smith D, Hutkins RW. Measurements of fitness and competition in commensal Escherichia coli and E. coli O157:H7 strains. Appl Environ Microbiol. 2004;70(11):6466‒6472. doi:10.1128/AEM.70.11.6466-6472.2004.
- Aldridge P, Hughes KT. Regulation of flagellar assembly. Curr Opin Microbiol. 2002;5(2):160‒165. doi:10.1016/s1369-5274(02)00302-8.
- Perna NT, Plunkett G 3rd, Burland V, Mau B, Glasner JD, Rose DJ, Mayhew GF, Evans PS, Gregor J, Kirkpatrick HA, et al. Genome sequence of enterohaemorrhagic Escherichia coli O157:H7. Nature. 2001;409(6819):529‒533. doi:10.1038/35054089.
- Iyoda S, Watanabe H. Positive effects of multiple pch genes on expression of the locus of enterocyte effacement genes and adherence of enterohaemorrhagic Escherichia coli O157:H7 to HEp-2 cells. Microbiol (Reading). 2004;150:2357‒2371. doi:10.1099/mic.0.27100-0.
- Madrid C, Balsalobre C, Garcia J, Juarez A. The novel Hha/YmoA family of nucleoid-associated proteins: use of structural mimicry to modulate the activity of the H-NS family of proteins. Mol Microbiol. 2007;63(1):7‒14. doi:10.1111/j.1365-2958.2006.05497.x.
- Nagel de Zwaig R, Luria SE. Genetics and physiology of colicin-tolerant mutants of Escherichia coli. J Bacteriol. 1967;94(4):1112‒1123. doi:10.1128/jb.94.4.1112-1123.1967.
- Deprez C, Lloubes R, Gavioli M, Marion D, Guerlesquin F, Blanchard L. Solution structure of the E. coli TolA C-terminal domain reveals conformational changes upon binding to the phage g3p N-terminal domain. J Mol Biol. 2005;346(4):1047‒1057. doi:10.1016/j.jmb.2004.12.028.
- Szczepaniak J, Press C, Kleanthous C. The multifarious roles of Tol-Pal in gram-negative bacteria. FEMS Microbiol Rev. 2020;44(4):490‒506. doi:10.1093/femsre/fuaa018.
- Barba J, Bustamante VH, Flores-Valdez MA, Deng W, Finlay BB, Puente JL. A positive regulatory loop controls expression of the locus of enterocyte effacement-encoded regulators Ler and GrlA. J Bacteriol. 2005;187(23):7918‒7930. doi:10.1128/JB.187.23.7918-7930.2005.
- Saitoh T, Iyoda S, Yamamoto S, Lu Y, Shimuta K, Ohnishi M, Terajima J, Watanabe H. Transcription of the ehx enterohemolysin gene is positively regulated by GrlA, a global regulator encoded within the locus of enterocyte effacement in enterohemorrhagic Escherichia coli. J Bacteriol. 2008;190(14):4822‒4830. doi:10.1128/JB.00231-08.
- Pozdeev G, Beckett MC, Mogre A, Thomson NR, Dorman CJ. Reciprocally rewiring and repositioning the Integration Host Factor (IHF) subunit genes in Salmonella enterica serovar typhimurium: impacts on physiology and virulence. Microb Genom. 2022;8(2):000768. doi:10.1099/mgen.0.000768.
- Fuqua WC, Winans SC, Greenberg EP. Quorum sensing in bacteria: the LuxR-LuxI family of cell density-responsive transcriptional regulators. J Bacteriol. 1994;176(2):269‒275. doi:10.1128/jb.176.2.269-275.1994.
- Pony P, Rapisarda C, Terradot L, Marza E, Fronzes R. Filamentation of the bacterial bi-functional alcohol/aldehyde dehydrogenase AdhE is essential for substrate channeling and enzymatic regulation. Nat Commun. 2020;11(1):1426. doi:10.1038/s41467-020-15214-y.
- Majdalani N, Gottesman S. The Rcs phosphorelay: a complex signal transduction system. Annu Rev Microbiol. 2005;59:379‒405. doi:10.1146/annurev.micro.59.050405.101230.
- Gottesman S, Trisler P, Torres-Cabassa A. Regulation of capsular polysaccharide synthesis in Escherichia coli K-12: characterization of three regulatory genes. J Bacteriol. 1985;162(3):1111‒1119. doi:10.1128/jb.162.3.1111-1119.1985.
- Francez-Charlot A, Laugel B, Van Gemert A, Dubarry N, Wiorowski F, Castanie-Cornet MP, Gutierrez C, Cam K. RcsCDB His-Asp phosphorelay system negatively regulates the flhDC operon in Escherichia coli. Mol Microbiol. 2003;49(3):823‒832. doi:10.1046/j.1365-2958.2003.03601.x.
- Berne C, Ellison CK, Ducret A, Brun YV. Bacterial adhesion at the single-cell level. Nat Rev Microbiol. 2018;16(10):616‒627. doi:10.1038/s41579-018-0057-5.
- Lehnen D, Blumer C, Polen T, Wackwitz B, Wendisch VF, Unden G. LrhA as a new transcriptional key regulator of flagella, motility and chemotaxis genes in Escherichia coli. Mol Microbiol. 2002;45(2):521‒532. doi:10.1046/j.1365-2958.2002.03032.x.
- Kimura S, Suzuki T. Fine-tuning of the ribosomal decoding center by conserved methyl-modifications in the Escherichia coli 16S rRNA. Nucleic Acids Res. 2010;38(4):1341‒1352. doi:10.1093/nar/gkp1073.
- Sauder AB, Kendall MM, Margolin W. After the fact(or): posttranscriptional gene regulation in enterohemorrhagic Escherichia coli O157:H7. J Bacteriol. 2018;200(19):e00228–18. doi:10.1128/JB.00228-18.
- Felden B, Augagneur Y. Diversity and versatility in small RNA-mediated regulation in bacterial pathogens. Front Microbiol. 2021;12:719977. doi:10.3389/fmicb.2021.719977.
- Quendera AP, Seixas AF, Dos Santos RF, Santos I, Silva JPN, Arraiano CM, Andrade JM. RNA-binding proteins driving the regulatory activity of small non-coding RNAs in bacteria. Front Mol Biosci. 2020;7:78. doi:10.3389/fmolb.2020.00078.
- Kapuy O, Barik D, Sananes MR, Tyson JJ, Novak B. Bistability by multiple phosphorylation of regulatory proteins. Prog Biophys Mol Biol. 2009;100(1–3):47‒56. doi:10.1016/j.pbiomolbio.2009.06.004.
- Strumillo M, Beltrao P. Towards the computational design of protein post-translational regulation. Bioorg Med Chem. 2015;23(12):2877‒2882. doi:10.1016/j.bmc.2015.04.056.
- Burrowes E, Baysse C, Adams C, O’Gara F. Influence of the regulatory protein RsmA on cellular functions in Pseudomonas aeruginosa PAO1, as revealed by transcriptome analysis. Microbiol (Reading). 2006;152:405‒418. doi:10.1099/mic.0.28324-0.
- Lawhon SD, Frye JG, Suyemoto M, Porwollik S, McClelland M, Altier C. Global regulation by CsrA in Salmonella typhimurium. Mol Microbiol. 2003;48(6):1633‒1645. doi:10.1046/j.1365-2958.2003.03535.x.
- Wachter S, Bonazzi M, Shifflett K, Moses AS, Raghavan R, Minnick MF, Henkin TM. A CsrA-Binding, trans -Acting sRNA of Coxiella burnetii is necessary for optimal intracellular growth and vacuole formation during early infection of host cells. J Bacteriol. 2019;201(22):e00524–19. doi:10.1128/JB.00524-19.
- Wei BL, Brun-Zinkernagel AM, Simecka JW, Pruss BM, Babitzke P, Romeo T. Positive regulation of motility and flhDC expression by the RNA-binding protein CsrA of Escherichia coli. Mol Microbiol. 2001;40(1):245‒256. doi:10.1046/j.1365-2958.2001.02380.x.
- Gottesman S, Roche E, Zhou Y, Sauer RT. The ClpXP and ClpAP proteases degrade proteins with carboxy-terminal peptide tails added by the SsrA-tagging system. Genes Dev. 1998;12(9):1338‒1347. doi:10.1101/gad.12.9.1338.
- Gottesman S. Trouble is coming: signaling pathways that regulate general stress responses in bacteria. J Biol Chem. 2019;294(31):11685‒11700. doi:10.1074/jbc.REV119.005593.
- Ortega J, Singh SK, Ishikawa T, Maurizi MR, Steven AC. Visualization of substrate binding and translocation by the ATP-dependent protease, ClpXP. Mol Cell. 2000;6(6):1515‒1521. doi:10.1016/s1097-2765(00)00148-9.
- Vasil’eva Iu M, Garber MB. The regulatory role of the Hfq protein in bacterial cells. Mol Biol (Mosk). 2002;36:970‒977.
- Link TM, Valentin-Hansen P, Brennan RG. Structure of Escherichia coli Hfq bound to polyriboadenylate RNA. Proc Natl Acad Sci U S A. 2009;106(46):19292‒19297. doi:10.1073/pnas.0908744106.
- Storz G, Vogel J, Wassarman KM. Regulation by small RNAs in bacteria: expanding frontiers. Mol Cell. 2011;43(6):880‒891. doi:10.1016/j.molcel.2011.08.022.
- Jorgensen MG, Pettersen JS, Kallipolitis BH. sRNA-mediated control in bacteria: an increasing diversity of regulatory mechanisms. Biochim Biophys Acta Gene Regul Mech. 2020;1863(5):194504. doi:10.1016/j.bbagrm.2020.194504.
- Yang B, Feng L, Wang F, Wang L. Enterohemorrhagic Escherichia coli senses low biotin status in the large intestine for colonization and infection. Nat Commun. 2015;6:6592. doi:10.1038/ncomms7592.
- Otaka H, Ishikawa H, Morita T, Aiba H. PolyU tail of rho-independent terminator of bacterial small RNAs is essential for Hfq action. Proc Natl Acad Sci U S A. 2011;108(32):13059‒13064. doi:10.1073/pnas.1107050108.
- Chaban B, Hughes HV, Beeby M. The flagellum in bacterial pathogens: for motility and a whole lot more. Semin Cell Dev Biol. 2015;46:91‒103. doi:10.1016/j.semcdb.2015.10.032.
- Wolfson EB, Elvidge J, Tahoun A, Gillespie T, Mantell J, McAteer SP, Rossez Y, Paxton E, Lane F, Shaw DJ, et al. The interaction of Escherichia coli O157:H7 and Salmonella Typhimurium flagella with host cell membranes and cytoskeletal components. Microbiol (Reading). 2020;166(10):947‒965. doi:10.1099/mic.0.000959.
- Subramanian S, Kearns DB. Functional regulators of bacterial flagella. Annu Rev Microbiol. 2019;73:225‒246. doi:10.1146/annurev-micro-020518-115725.
- Vijayan A, Rumbo M, Carnoy C, Sirard JC. Compartmentalized antimicrobial defenses in response to flagellin. Trends Microbiol. 2018;26(5):423‒435. doi:10.1016/j.tim.2017.10.008.
- Liu Y, Liu B, Yang P, Wang T, Chang Z, Wang J, Wang Q, Li W, Wu J, Huang D, et al. LysR-type transcriptional regulator OvrB encoded in O island 9 drives enterohemorrhagic Escherichia coli O157:H7 virulence. Virulence. 2019;10(1):783‒792. doi:10.1080/21505594.2019.1661721.
- Frenzen PD, Drake A, Angulo FJ. Emerging Infections Program FoodNet Working G. Economic cost of illness due to Escherichia coli O157 infections in the United States. J Food Prot. 2005;68(12):2623‒2630. doi:10.4315/0362-028x-68.12.2623.
- Mir RA, Kudva IT. Antibiotic-resistant Shiga toxin-producing Escherichia coli: an overview of prevalence and intervention strategies. Zoonoses Public Health. 2019;66(1):1‒13. doi:10.1111/zph.12533.
- Ho NK, Henry AC, Johnson-Henry K, Sherman PM. Pathogenicity, host responses and implications for management of enterohemorrhagic Escherichia coli O157:H7 infection. Can J Gastroenterol. 2013;27(5):281‒285. doi:10.1155/2013/138673.