ABSTRACT
Dysbiosis of the gut microbiome and a pathological immune response in intestinal tissues form the basis of Crohn’s disease (CD), which is a debilitating disease with relevant morbidity and mortality. It is increasing in childhood and adolescents, due to western life-style and nutrition and a large set of predisposing genetic factors. Crohn’s disease-associated genetic mutations play an essential role in killing pathogens, altering mucosal barrier function, and protecting the host microbiome, suggesting an important pathogenic link. The intestinal microbiome is highly variable and can be influenced by environmental factors. Changes in microbial composition and a reduction in species diversity have been shown to be central features of disease progression and are therefore the target of therapeutic approaches. In this review, we summarize the current literature on the role of the gut microbiome in childhood, adolescent, and adult CD, current therapeutic options, and their impact on the microbiome.
Introduction
Inflammatory bowel disease (IBD), with its main forms Crohn’s disease (CD) and Ulcerative Colitis (UC), is on the rise, with 25% of IBD diagnosed in children under 18 years.Citation1 In CD, discontinuous involvement of the entire gastrointestinal tract is possible, with the terminal ileum being a particularly typical site of manifestation. In addition, development of fistulas and stenosis and extraintestinal manifestations, e.g., in the hepatobiliary tract, eyes, joints or skin are also well recognized.Citation2–4
CD is an immunological disorder with a multifactorial etiology: a polygenetic predisposition underlies the disease, and in susceptible individuals, environmental triggers can lead to a sustained proinflammatory response that damages the gut and its interaction with its microbiome,Citation5 which finally shifts the bacterial composition. This is designated as dysbiosis, which is described as a change in the composition and function of the microbiota in diseased individuals compared to healthy individuals.Citation6 Three basic changes form the pillars of dysbiosis: the loss of beneficial microorganisms, the proliferation of potentially harmful microbes, and the loss of overall microbial diversity. However, since there is no single definition of what a healthy microbiome should look like, there is no universally accepted definition of dysbiosis.Citation7–9 The resulting dysbiosis is thus part of the pathogenesis of the disease and is maintained by the inflammatory response at the same time. Environmental triggers include Western dietary habits,Citation10 active and passive smoking,Citation11–13 use of antibiotics,Citation14,Citation15 NSAIDCitation16,Citation17 and oral contraceptives.Citation18
Numerous genetic loci encoding specific proteins of the body’s immune defenses have been identified and are associated with the development of CD.Citation19 Genetic mutations e.g. in the NOD2 gene, have been shown to lead to inadequate handling of bacterial pathogens and thus promote the development of CD.Citation20 In addition, the loss of an intact epithelial intestinal barrier is also discussed as an important step in pathogenesis. Innate immunity plays a crucial role in this process: it ensures the recognition and differentiation of pathogenic invaders from the commensal microbial intestinal flora.Citation21–23 Ideally, the host-specific microbiome is spared and pathogenic bacteria are eliminated with the help of mucosal defense mechanisms like defensins. Possible processes involved in immune tolerance are impaired in CD such as the aryl hydrocarbon receptor (AhR) in colonic tissue. AhR is a ligand-dependent transcription factor that is widely expressed in various immune cells, including dendritic cells (DCs). Its activation leads to decreased expression of proinflammatory cytokines and increased production of anti-inflammatory cytokines (IL-1β, IL-23, and IL-12). In addition, it leads to promotion of Treg cell differentiation and improvement of epithelial barrier function of the intestinal mucosa.Citation24,Citation25 In CD, these processes appear to be disturbed, leading to increased growth of pathogenic bacteria, i.e., also to dysbiosis, and subsequently to a local inflammatory reaction that can entertain the derailed immune response.Citation5 Thus, an imbalance in the innate immune system’s defenses is at the basis of the chronic inflammatory response. Other signaling pathways that may be impaired and thus promote the manifestation of CD include defects in the mucosal barrier (Mut2 and FUT2 genes), increased migration of immune cells to sites of inflammation mediated by remodeling of the extracellular matrix through the action of metalloproteins (MMP-1 and MMP-3), and overexpression of adhesion molecules such as MAcCAM-1 and integrin α4β4.Citation26
Whether dysbiosis itself is one of the initial causes or the consequence of the disease is still controversial. It is usually associated with a loss of diversity in the microbiome, with a concomitant increase in Proteobacteria (e.g., Pasteurellaceae, Neisseriaceae, Enterobacteriaceae), Fusobacteriaceae and Actinomycetota and depletion of commensal intestinal bacteria, particularly Firmicutes (specifically those from the Clostridium clusters XIVa and IV) and Bacteroidetes. Approximately one third of CD patients exhibit increased abundance of mucosa-associated adherent-invasive Escherichia coli (AIEC). Overall, not only the diversity but also the amount of bacteria in the gut decreases. Faecalibacterium prausnitzii, a commensal bacterium with anti-inflammatory properties, is reduced in CD. Patients with IBD exhibit also viral and fungal dysbiosis.Citation27–31
Treatment includes immunomodulators such as thiopurines (azathioprine, 6-mercaptopurine), methotrexate, but also corticosteroids (CS), nutritional therapy, especially exclusive enteral nutrition (EEN), monoclonal antibodies against selected cytokines such as tumor necrosis factor α (TNF-α), other proinflammatory interleukins or integrins (e.g. α4β4), and antibiotics.Citation29,Citation32,Citation33 Except for enteral nutrition therapy and, in some cases, antibiotics, other interventions do not directly aim to alter the gut microbiome, but rather to curb the inflammatory response and thereby improve symptoms. In addition to the direct influence of EEN on the intestinal microbiome, other mechanisms of action of this therapy also play a role in explaining its positive effects. It has been shown that EEN has an influence on the defense function of the intestinal mucosa as well as on the inflammatory response of the intestine. Thus, there is a decrease in proinflammatory cytokines (IL-1β, IL-2, IL-6, TNF-a, and INF-ƴ), an increase in anti-inflammatory cytokines (transforming growth factor (TGF) beta-1) and regulatory T cells that promote anti-inflammatory processes in the mucosa, and an improvement in intestinal permeability and the integrity of the mucosal layer. Normalization of inflammatory parameters (CRP, Erythrocyte sedimentation rate (ESR)) is also observed. Furthermore, EEN influences and increases the concentration of short-chain fatty acids (SCFAs) such as butyrate, acetate, and propionate, which in turn have an anti-inflammatory effect on the intestinal mucosa. However, the exact mechanism of remission induction by EEN in CD remains unclear, although there is evidence that certain foods (wheat, emulsifiers, and some fatty acids) have a negative effect on epithelial cell tight junctions and impair mucus function.Citation34–36 Fecal microbiota transplantation (FMT) has only been tested in small trials and is not yet an approved therapy for the treatment of CD. Like EEN, it aims to alter the gut microbiome and thus prevent disease progression.Citation37,Citation38 Antibiotics are used as adjunctive therapy for complications such as fistulas and intra-abdominal abscesses, but also have immunomodulatory effects.Citation39 In addition, antibiotics such as vancomycin are now being investigated in the treatment of primary sclerosing cholangitis (PSC), which is also a complication of UC and CD.Citation40 Their use in this context also affects the intestinal microbiome and can lead to both exacerbation and improvement of the dysbiotic situation.Citation32,Citation41 In addition, partial nutrition therapy is discussed as a supplement to an ad libitum diet for malabsorption and in recently the Crohn’s disease exclusion diet (CDED).Citation42,Citation43
In this review, we summarize the current literature on the role of the gut microbiome in CD, its interplay with the innate and acquired immune system, current therapeutic options, and their impact on the microbiome.
The healthy gut microbiome
The commensal inhabitants of the gut are as numerous as they are diverse: bacteria, viruses, archaea, and eukaryotesCitation44 together comprise the gut microbiome as 1014 microbes.Citation5 Among these, Firmicutes (e.g., Faecalibacterium, Roseburia, Ruminococcus, Eubacterium) and Bacteroidetes (e.g., Bacteroides, Alistipes, Prevotella) dominate, followed by Actinomycetota (e.g., Bifidobacterium, Collinsella) and Proteobacteria (e.g., Escherichia). These are accompanied to a much lesser extent by other phyla such as Synergistetes, Verrucomicrobia, Fusobacteria, and Euryarchaeota.Citation45 It should be noted, that the microbiome varies greatly depending on its location in the gastrointestinal tract (esophagus, stomach, small and large intestine), and sample material (biopsy, stool sample, luminal duodenal aspirate, mucosal brush sample). A stool sample is more likely to reflect the microbial composition of the luminal side of the gut, whereas a biopsy of the mucosa is more likely to represent a picture of the mucosa-associated microbiome in the large intestine.Citation46–49 Microbiome studies using stool samples show, that phyla such as Bacteroidetes, Firmicutes, Proteobacteria, and Actinomycetota dominate, while in biopsies of the mucosa, Firmicutes, Bacteroidetes, Fusobacteria and Proteobacteria are overrepresented. In the phylum of Firmicutes, Faecalibacterium, Lachnospiraceae, and Roseburia are the most abundant genera in stool samples, while Ruminococcus and Blautia are most present in mucosal biopsies.Citation45,Citation50
In addition to the differences in the gut microbiome mentioned above, there are large interindividual variations as well as intraindividual changes throughout life. Variations between the microbiome of individuals depend on circumstances at birth (intrapartum antibiotics, mode of delivery: cesarean/vaginal delivery, breastfeeding), environment (e.g., animal contact, siblings), geographical origin, diet, BMI (body-mass-index), gender, and any diseases and medication use (e.g., antibiotics).Citation51–54 Azad et al.Citation55 demonstrated that the microbiome of infants born to mothers who received antibiotic prophylaxis (against B streptococci or due to cesarean section) during delivery differed from the microbiome of infants without intrapartum antibiotic prophylaxis for months after birth. Thus, a loss of richness of the commensal microbiota was observed in infants at three months of age. Among others, there was a depletion of Bacteroidetes and an increased abundance of Firmicutes (genera Clostridium and Enterococcus). In children who were not breastfed, some of the changes in the microbiome persisted. Furthermore, in children who received breast milk, lower abundance of bacteria was observed even after three months. Similarly, an enrichment of Bifidobacteria and a lower abundance of Bacteroidetes and Clostridiales were observed compared to non-breastfed children. A higher diversity of the microbiota was reported at one year of age in children who were breastfed at very early life stages. However, this phenomenon was also dependent on the duration and exclusivity of breastfeeding and was independent of the mode of delivery. Ringel-Kulka et al.Citation56 showed that the microbiome of young children is not yet fully equivalent to that of adults. Children still have a gap in the microbiome, the so-called window-of-opportunity. This provides an opportunity for external influences to shape the still immature microbiome of children, helping to promote health and interrupt the potential development of disease. The study also showed that, on the one hand, there are phyla that become established as early as 4 years of age, such as Clostridium cluster XIVa (including Roseburia intestinalis, Ruminococcus obeum and Eubacterium rectale). On the other hand, there are phyla such as Bacteroidetes, Bifidobacteria, and Clostridium cluster IV (including F. prausnitzii) whose numbers change only gradually with age. Moreover, a significantly higher number of Bifidobacteria (3.7-fold) was found in children. In fact, among the Actinomycetota, the genus Bifidobacteria was the group most likely to show a separation between the microbiome of children and adults. Claesson et al.Citation57 compared the microbiome of younger (13, 40, 44–46 years) and older adults (65 years and older). The core microbiome of older adults differed from that of young adults: e.g., older adults had a greater proportion of Bacteroides spp., a lower proportion of Firmicutes, and different abundances of various Clostridia groups. This study also examined the extent to which the microbiome changes in both health and disease, and found that Proteobacteria, Actinomycetota, and Faecalibacterium in particular are subject to strong influence.
Biagi et al.Citation58 studied how the microbiome of centenarians (99-104) and semi-supercentenarians (105+) differs from that of young adults (average age 30.5 years) and showed that there is a core microbiome consisting of the families Ruminococcaceae, Lachnospiraceae (both belonging to the phylum Firmicutes) and Bacteroidaceae (phylum Bacteroidetes), but with an age-related decrease in cumulative abundance. Aging is also characterized by increasing abundance of subdominant species (Eggerthella, Akkermansia, Anaerotruncus, Synergistaceae, Bilophila, and Christensenellaceae). Among semi-supercentenarians, opportunistic and allochthonous bacteria have been identified that may support maintenance of health during aging. These include Akkermansia, Bifidobacterium, and Christensenellaceae, for example. In a previous study by Drago et al.,Citation59 a quantitative decrease in Enterobacteriaceae (phylum Proteobacteria), Bifidobacteria (phylum Actinomycetota), and Bacteroides (phylum Bacteroidetes) and an increase in Clostridia sensu stricto was observed. The numbers of Lactobacillus and Bifidobacterium were similar in the centenarians and young adults in the study, but the composition of the Lactobacillus subpopulation was different.
Physiological function of the gut microbiome
To understand how the microbiome may affect the development of CD and how the inflammatory response in CD in turn affects the microbiome, one must consider the physiological roles of the microbiome. The central roles of the gut microbiome are digestion, immune defense, and immune system development.Citation60 In addition, the gut microbiome is involved in the synthesis of important vitamins such as vitamin K and the vitamin B complex (Vit. B1, B2, B6, B12), thus contributing to the synthesis of coagulation factors II, VII, IX, X, hematopoiesis, energy metabolism, amino acid metabolism, and neurotransmitter synthesis.Citation61 ()
Figure 1. Physiological function of the intestinal microbiome: The gut microbiota support digestion, synthesis of important substances such as SCFAs and vitamins, immune development, and defenses.
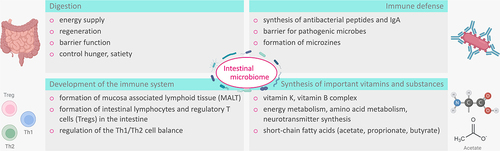
Infants whose gut microbiome is shaped by human milk produce metabolites for the synthesis of peripheral regulatory T cells. In addition, bacteria induce IgA+ -B cell production via activation of germinal centers. Bacterial molecules also provide for the formation of mucosa-associated lymphoid tissue (MALT) in the intestine, termed gut-associated lymphoid tissue (GALT), via Toll-like receptors (TLRs), thus contributing to the shaping of Th cell-mediated immunity of the gut. This promotes the activation and expansion of naive T cells into Th1 and/or Th17 effector cells that enter the systemic circulation and support the destruction of invading pathogens in the gut.Citation62,Citation63 Plant food components (starch, cell wall polysaccharides) as well as proteins are fermented in the intestine by bacteria (e.g., F. prausnitzii), and the resulting SCFAs have effects on host metabolism and immunity. Thus, butyrate serves as an energy source for colonocytes, maintains epithelial integrity in the gut, supports Treg differentiation, and has anti-inflammatory properties.Citation5,Citation61
The microbiome in CD
Microbial changes in adults and children
The fact that the microbiome of CD patients differs from that of healthy individuals has long been known and is summarized again here.
Right at the outset, it should be noted that newborns of mothers with IBD show reduced bacterial diversity and altered bacterial composition of the microbiome. For example, stool samples from these infants showed an enrichment of Gammaproteobacteria and a depletion of Bifidobacteria.Citation64 In addition, fecal calprotectin measurements have shown elevated levels in infants of mothers with IBD, indicating subclinical inflammation in early life, the long-term consequences of which are uncertain.Citation65 Gevers et al.Citation66 and Kansal et al.Citation67 have already shown a correlation between CD and an increasing abundance of Pasteurellaceae (Haemophilus spp.), Neisseriaceae, Enterobacteriaceae (all three belong to the phylum Proteobacteria), Veillonellaceae (phylum Firmicutes) and Fusobacteriaceae (phylum Fusobacteriota), with a simultaneous decrease of Bacteroides (phylum Bacteroidetes) and Clostridiales (phylum: Firmicutes) in mucosal biopsies of the ileum and the colon/rectum of children. Another study by Gevers et al.Citation66 on the abundance of several genera such as Faecalibacterium, Roseburia, Blautia, Ruminococcus, Coprococcus (all phylum Firmicutes) and a number of taxa within the families Ruminococcaceae and Lachnospiraceae also showed a decrease in abundance. Interestingly, some of these taxa were present in greater abundance in patients younger than 10 years than in older children, indicating an inverse relationship with age, including Pasteurellaceae and Neisseriaceae. In the stool samples also studied by Gevers et al.,Citation66 such a change was detected only to a small extent. In contrast, Lewis et al.Citation32 found in a study with stool samples from children on the one hand a reduced abundance of Prevotella, Odoribacter, Alistipes, Parabacteroides (all four phylum Bacteroidetes), Eubacterium, Roseburia, Coprococcus, Dorea, Ruminococcus (families of Lachnospiraceae, Ruminococcaceae, Eubacteriaceae, all phylum Firmicutes), Akkermansia (phylum Verrucomicrobiota) and, on the other hand, an increased abundance of Escherichia, Klebsiella (family Enterobacteriaceae, phylum Proteobacteria), Enterococcus and Veillonella (both phylum Firmicutes).
Takahashi et al.Citation68 investigated stool samples from adults with CD and found significantly reduced amounts of Bacteroides, Eubacterium, Faecalibacterium and Ruminococcus and significantly increased amounts of Actinomyces and Bifidobacterium. At the species level, Blautia faecis, Roseburia inulinivorans, Ruminococcus torques, Clostridium lavalense, Bacteroides uniformis and F. prausnitzii were significantly reduced ().
Figure 2. Microbiological changes in CD: Relative changes in abundance of taxa in CD patients compared to non-IBD subjects.
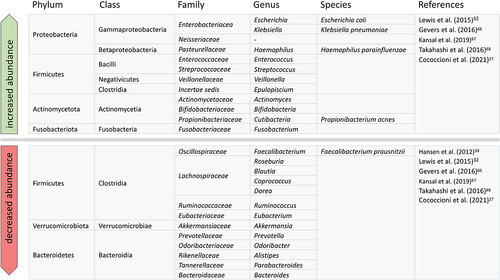
One special bacterium is F. prausnitzii (phylum Firmicutes), as it may play an important role in the manifestation of CD due to its anti-inflammatory properties and by providing energy for epithelial cells.Citation69,Citation70 Based on biopsies of the colon, F. prausnitzii appears to be present in significantly higher quantities in the early stages of the disease in children, although its quantity is significantly reduced in adult Crohn’s patients who have had the disease for some time.
Kansal et al.Citation67 reported similar findings: Patients were biopsied and divided into four groups according to disease stage: Crohn’s disease First Diagnosis (CDFD), Remission (CDRM), Relapse (CDRL) and a healthy control group (control samples without CD). F. prausnitzii was found to be present in almost 95% of all patients with an initial diagnosis of CD (CDFD) and in almost 99% of the healthy control group, with very similar mean frequencies. This contrasts with the lower prevalence of F. prausnitzii in the CDRM and CDRL groups as part of the manifesting dysbiosis of the microbiome.
It is possible that F. prausnitzii protects against progression of CD early, but its protective effect diminishes over time as the species decreases in numbers.Citation30 Caparrós et al.Citation71 also concluded that the gut microbiome of CD patients is more unstable over time during the acute phase of the disease. The loss of beneficial microorganisms (such as F. prausnitzii) seems to play a crucial role in the pathogenesis of CD.Citation72,Citation73
The type of sample material does not seem to play as much a significant role with regard to the altered composition of the microbiome in children.
The influence of genetics on the inflammatory response and mucosal barrier function in CD
In addition to environmental factors, numerous genes have been shown to influence the development of CD. A total of 140 susceptibility loci are known.Citation5 NOD2 and ATG16L1 mutations on chromosomes 16 and 2 are the best-known genes associated with an increased risk. The NOD2 gene encodes an intracellular pattern recognition receptor (PRR) that recognizes bacterial peptidoglycans (PAMPs = pathogen-associated molecular patterns and MAMPs = microbe-associated molecular patterns). In doing so, the PRR binds to muramyl dipeptide (MDP), a peptidoglycan found in the cell wall of bacteria. This leads to the activation of proinflammatory signaling pathways, such as NF-κB, resulting in the production of inflammatory cytokines and the release of antimicrobial peptides (e.g., defensins) ().
Figure 3. Schematic illustration of the interaction between the host microbiome, the intestinal barrier, and pathological agents under healthy conditions and in active CD. Anti-inflammatory cytokines (IL-10, TGF-β) are released via activation of regulatory T cells (Tregs), so that no activation of CD4+ T cells occurs. This mediates tolerance to symbiotic microorganisms. After pathogen invasion of the intestine, CD4+ T cells are activated by dendritic cells, and then B cells are activated by Th2 cells, which differentiate into antibody-producing plasma cells. In addition to activation of the acquired immune system, defense processes of the innate immune system also take place, e.g., by defensins. (A) dysbiosis promoted by genetic predisposition and/or environmental factors leads to an excessive proinflammatory immune response and increased permeability of the intestinal barrier. Differentiation into Th1, Th2 and Th17 cells occurs via activation of CD4+ T cells. The Th1 cell activates macrophages via cytokines, which produce reactive oxygen species (ROS) in addition to their phagocytotic function. Macrophages also maintain the Th1 response with the help of cytokines. The Th2 cells activate B cells to differentiate into antibody-producing plasma cells. The Th17 cell dominates alongside the Th1 cell in CD and promotes the inflammatory process and destruction of the mucosal barrier in the intestine via cytokines. Due to the genetic changes, there is insufficient defensin production, so that the innate immune defense is significantly impaired in its function. The ongoing inflammation promotes the aggravation of dysbiosis as part of an endless spiral (B).
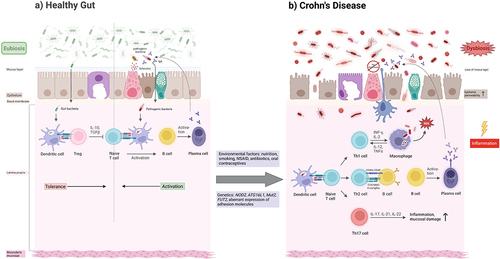
In contrast, the NOD2 mutation results in deficient activation of the NF-κB pathway by PAMPs, leading to insufficient activation of macrophages, Paneth cells, and dendritic cells and release of defensins, which in turn results in insufficient killing of pathogenic microbes. The consequences include an inflammatory response in the gut triggered by these bacteria,Citation74,Citation75 a breakdown of barrier function with invasion and spread of the dysbiotic microbiome, and impaired autophagy with inadequate killing of intracellular bacteriaCitation76 ().
It has not yet been clarified whether the impaired barrier function of the intestinal mucosa promotes the development of the disease or is a consequence of the disease. However, it can be deduced from the studies to date that the loss of an intact barrier perpetuates the inflammatory response associated with CD.
The ATG16L1 gene encodes an autophagy related 16-like 1 protein, which is characterized as a subunit of the autophagy-related ATG12-ATG5/ATG16 complex and is essential for LC3 lipidation and autophagosome formation to defend against invading pathogens. According to recent studies, the ATG16L1 protein mediates enhanced cytokine release due to increased NF-κB activation.Citation77 In this context, the ATG16L1 mutation also leads to deficient intracellular killing of pathogenic bacteria, thereby promoting the inflammatory response.Citation78
The role of the acquired immune system
In addition to the innate immune system, the acquired immune system also plays an important role, as it contributes significantly to the destruction of the intestinal mucosa. In CD, the Th17 and Th1 cells dominate, which take up antigens from pathogenic microbes showed to them by antigen-presenting cells (APC). Th1 cells secrete interferon gamma (INF-γ) and activate intestinal macrophages via the JAK-STAT pathway to produce bactericidal substances such as reactive oxygen species (ROS), which can also damage the mucosa. Th17 cells produce cytokines such as IL-17, IL-21, IL-22, etc., which contribute to the inflammatory response and mucosal damageCitation79 (). As the barrier function of the epithelium is disrupted, bacteria from the intestinal lumen may invade the epithelial cells and autoantibodies (including perinuclear antineutrophil cytoplasmic antibodies [pANCA]) may be formed as a result of cross-reaction with bacterial antigens in the intestine (in 10–15% of CD cases).Citation80 Similarly, formation of antimicrobial antibodies may occur, and the formation of autoantibodies against specific endogenous structures (e.g., intestinal goblet cells, tropomyosin) has also been described.Citation81
Therapeutic options
There are several therapeutic approaches aimed at either converting the dysbiotic microbiome to a non-dysbiotic microbiome through enteral nutrition therapy or fecal microbiota transplantation (FMT), or suppressing the proinflammatory immune response with antibodies, glucocorticoids or other immunosuppressants.Citation82,Citation83
Furthermore, a distinction must be made between the treatment of an acute flare and long-term therapy with the goal of remission. Treatment of the flare depends on the severity and extent of inflammation. Basic measure is enteral nutrition therapy, consisting of fully absorbable, low-fiber liquid nutrition, typically from a polymer formula.Citation84 Systemically administered glucocorticoids (e.g., prednisone) are used as therapeutic agents for severe acute activity, whereas topically applied glucocorticoids (e.g., budesonide per os) are used for mild inflammatory activity. Due to the high side effect profile, they are not used for remission maintenance therapy. In children and adolescents, its use can be associated with severe growth retardation, loss of bone mineral density and delayed pubertal development. Currently, immunosuppressants such as azathioprine, 6-mercaptopurine, and methotrexate as well as anti-TNF-α antibodies and other monoclonal antibodies such as ustekinumab are used for remission maintenance therapy, partial enteral nutrition (PEN) may play a role.Citation85
Azathioprine is a purine analogue that is metabolized in the body to 6-mercaptopurine and methylnitroimidazole. Azathioprine and its active metabolite 6-mercaptopurine inhibit lymphocyte differentiation and have an antiproliferative effect on CD8+ T lymphocytes, natural killer cells, and B lymphocytes. In addition, the secretion of TNF-α is reduced.Citation86
Methotrexate (MTX), as a folic acid antagonist, competitively and reversibly inhibits the enzyme dihydrofolate reductase (DHFR). This interferes with the formation of purines and thymidines, which are required for the de novo synthesis of DNA and RNA. It also inhibits other enzymes such as thymidilate synthase, which catalyzes metabolic steps of pyrimidine synthesis. Ultimately, MTX thus leads to an inhibition of cell proliferation.Citation87,Citation88
Clinically, antibiotics are often used to prevent or treat complications (abscesses, fistulas, stenosis, perianal disease) and treat potential infections (e.g., C.diff.) in pediatric IBD patients with heterogenous effects on disease activity and considerable side-effects in some cases. Antibiotics use is also associated with increased risk of new-onset IBD.Citation89–91
Enteral nutrition
There are three models of enteral nutrition therapy: exclusive enteral nutrition (EEN), partial enteral nutrition (PEN), and forms that imitate EENs effect with natural food, like CDED.
In EEN, the patient receives liquid enteral nutrition (polymer or elemental) for approximately eight weeks, either orally or through a feeding tube.Citation92,Citation93 In contrast to EEN, PEN is used for remission maintenance and therefore aimed to supplement natural food stuff for several years. With PEN and EEN, the patient receives nutritional compounds that have been shown to have a positive effect on disease symptomatology and the gut microbiomeCitation32,Citation92 (). EEN, in contrast to PEN, means that the patient meets more than 100% of estimated caloric needs with liquid enteral nutrition, whereas with PEN it is 35–50% and the remaining portion is met by the normal diet. Recently however, PEN alone or in combination with CDED in children and adults was investigated for remission induction.Citation43,Citation96,Citation100,Citation101 This stresses the notion, that one of the key mechanisms of EEN is withdrawal of food groups that may promote the pathological proinflammatory cycle. CDED is a diet that can be coupled with PEN, which excludes dietary components suspected of negatively affecting the microbiome (dysbiosis) or immune mechanisms in the gut.Citation43
Table 1. Changes in the gut microbiome during different types of therapy. Exclusive enteral nutrition (EEN); Crohn’s disease exclusion diet (CDED); partial enteral nutrition (PEN); infliximab (IFX); ustekinumab (UST).
In the microbiome of children, Lewis et al.Citation32 found that a change in the abundance of six genera was detectable as early as one week after the start of EEN treatment: a decrease in Haemophilus, Streptococcus, Dialister, Dorea, and Gordonibacter, and an increase in Alistipes. Such a pattern was not observed in patients treated with either PEN or anti-TNF, with the latter approaching the healthy controls within a week. However, complete elimination of dysbiosis was not achieved with either anti-TNF therapy or EEN. Thus, anti-TNF still showed a significant difference compared to healthy controls in the following taxa: Klebsiella, Prevotella, Escherichia, Odoribacter, Enterococcus and Fusobacterium. This fact may be due to a sustained host inflammatory response. However, Lewis et al.Citation32 generally noted a marked convergence of the responders’ microbiome with that of healthy controls and a decrease in the inflammatory response. A similar change in the microbiome of children receiving PEN was not observed, so Lewis et al.Citation32 assumed that the exclusion of foods on the table was critical here. They also concluded that dysbiosis is likely the result of the inflammatory response in CD because anti-TNF therapy is administered parenterally and thus can only indirectly affect the gut microbiome. In another study investigating the effect of EEN on the microbiome of children, some changes were observed in those who were in remission at the end of the study. For example, the microbiome of these children showed an increased abundance of Akkermansia muciniphila and Bacteroides (Bacteroides fragilis and Bacteroides ovatus), but also an increase in the families of Lachnospiraceae (e.g., Dorea) and Ruminococcaceae. In contrast, the abundance of Proteobacteria decreased.Citation94 However, the effect of EEN on the microbiome of children is not consistent, and its efficacy in inducing remission in CD can only be partially explained. In most EEN studies, diversity does not increase in all children with clinical response, in fact it even decreases in some of those. A potential explanation might be that EEN decreases both beneficial and potentially harmful microbes (such as E. coli and Shigella), with the loss of the latter outweighing the loss of the former.Citation102–104 When looking at the potentially “offending agents” in western diet, especially the “new dietary components” in ultra processed foods, like emulsifiers, preservatives, and antibacterial agents, are partly contained in the polymeric formula. Also compared to other dietary interventions described below in pediatric CD, the main effect is in restricting certain major food groups. It is extremely difficult to obtain this data from patients with IBD, but studies about reintroduction of food stuff give hints that need to be followed in further investigations in large cohorts.Citation105,Citation106
A recent study confirmed that EEN improved the relative expression of the phylum Firmicutes and the genera Flavonifractor and Clostridium V after treatment.Citation95
It is therefore not surprising that EEN is recommended as first line therapy for the treatment of acute relapses in children. It has significantly fewer adverse effects and long-term consequences than the administration of systemically acting glucocorticoids. Although clinical remission can also be achieved with systemically acting CS, EEN has been shown to be more beneficial in achieving endoscopic/histologic remission, well suited for a modern “treat-to-target” concept, aimed for deep remission types.Citation93,Citation107,Citation108
The study by Levine et al.Citation43 compared CDED in combination with PEN to EEN in children with mild to moderate CD. In both groups, a change in the microbiome and a decrease in inflammatory response with clinical remission was noted after only six weeks. A decrease of Proteobacteria such as Haemophilus spp. but also of other bacteria such as Veillonella spp., Anaerostipes spp. or Prevotella spp. could be observed. Likewise, an increase of Firmicutes such as Roseburia (butyrate producer) and Oscillibacter was noticed (). Over the next six weeks, the groups differed as the EEN returned first to the PEN diet and then to the free diet: While the microbiome in the CDED+PEN group continued to change, the microbiome in the EEN group returned to baseline at week 12 (“rebound effect”). While inflammatory markers (fecal calprotectin, C-reactive protein) increased again in the EEN group after six weeks, they continued to decrease in the CDED+PEN group. Thus, CDED+PEN develops as an important alternative to EEN as potential first-line therapy in children with mild to moderate active CD.
While EEN is a popular therapy in children, it appears to be less successful in adults: Multiple meta-analysis showed decreased efficacy of EEN vs corticosteroids in adults in the pastCitation109, the reason for this difference remains unclear. The basis of the analysis shows uniformly high drop-out rates in the adult population, compared to adolescents which may contribute to the effect. One reason could be the family situation with encouraging parents and peers that may be less present in early adulthood or (as we pediatric gastroenterologists like to think) our increased care for the patient by the multidisciplinary team, including variations in the way (e.g., nasogastric tube) the formula is provided. Another factor is the low acceptability in everyday life that may be more challenging in young adult life. Some studies showed high efficacy when these obstacles were surrounded by (nightly) tube feeding and low threshold for counseling with close follow-up in young adults.Citation110,Citation111 The low acceptance of this form of therapy has also been addressed in the pilot of CDED+PEN and CDED alone in young adults with clinical remission in 68% resp. 57% after 6 weeks, sustained by 80% over 24 weeks, 35% with endoscopic remission.Citation101
Fecal microbiota transplant (FMT)
FMT is a potential additional, experimental therapy for the treatment of CD, but so far it has only been investigated in small studies in adults and children with heterogeneous results. Some of these studies show a positive effect on the development of the microbiome after FMT and an associated decrease in proinflammatory activity. However, these studies generally include very few patients, which limits the ability to draw meaningful conclusions about the effect of FMT.Citation37,Citation38 In the few studies with a higher number of participants (174 subjects), positive results were also obtained in terms of clinical response and clinical remission, but further studies of this type are needed.Citation112 Currently, FMT remains a therapeutic option in the treatment of recurrent Clostridioides difficile infections.Citation113
Patients showed a significant increase in operational taxonomic units (OTU) and Shannon diversity index two weeks after FMT, indicating an increase in species richness. These results suggest that FMT shifts the taxonomic composition of the patients’ gut microbiome toward that of their respective donors. A second FMT revealed that although OTU richness continued to increase, the Shannon diversity index did not show further changes. It is recommended that a second FMT be performed in less than four months after the first FMT. Patients exhibited dysbiosis before FMT, as evidenced by low levels of Bacteroides, Eubacterium, Faecalibacterium, and Roseburia and higher levels of Clostridium, Cronobacter, Fusobacterium, and Streptococcus. Bacterial families associated with CD, such as Fusobacteriaceae, Enterobacteriaceae, and Veillonellaceae, showed no significant difference in relative abundance after treatment with FMT, but the relative abundance of Faecalibacterium increased significantly. In addition to improving disease status, clinical remission was achieved in an average of 64.3% of patients in the three studies.Citation114–116 For example, Xiang et al.Citation85 demonstrated in their study of 174 subjects that at final follow-up, 43.7% (76/174) of patients achieved a clinical response and 20.1% (35/174) of patients achieved sustained clinical remission. The median follow-up time in this study was 43 months.
However, it should be noted that an average of 38 subjects participated in these three studies, and significantly more study participants will be needed to make a meaningful statement in future studies.
To determine which species are transplanted during FMT, Suskind et al.Citation117 identified 31 species in nine patients that were found in the patient two weeks after FMT but not before FMT, and furthermore, all were detected in the respective donor. However, there were many factors that could explain the presence of these newly detected species independent of transplantation, so further studies are needed here. Regardless, FMT was well tolerated by patients and showed both clinical and laboratory improvements in the majority of patients. H. Wang et al.Citation118 demonstrated that FMT was generally well tolerated, with few adverse events such as frequent bowel movements, fever, abdominal pain, hematochezia, vomiting, flatulence, and herpes zoster in the first month after FMT. No adverse events were recorded in the subsequent months.
It should also be noted that as of yet, randomized controlled trials (RCT) on this topic have been published for adult patients only, although one with children is currently planned.Citation119
Biological therapy
Anti-TNF therapy in CD involves the use of various monoclonal antibodies such as infliximab (IFX), adalimumab and certolizumab and biosimilars. Among biologicals, TNF-α inhibitors are the currently established therapy for the treatment of pediatric CD.Citation120
In addition, there are antibodies against other proinflammatory cytokines like ustekinumab (Anti IL23/12) or anti-integrin antibodies such as vedolizumab, which selectively block certain integrins on the surface of activated lymphocytes and thus prevent lymphocytes from migrating into the tissue.Citation121,Citation122
Early initiation of infliximab therapy has already been shown to influence the natural course of disease and significantly reduce the likelihood of complications.Citation123 Recent studies have compared the effects of early initiation of IFX therapy in children with newly diagnosed CD with prednisolone or EEN (conventional treatment). As early as ten weeks after therapy initiation, the proportion of children in clinical and endoscopic remission was significantly higher in the group receiving first-line infliximab therapy than in the conventionally treated group. Clinical remission was maintained after one year of first-line infliximab therapy without the need for CS or additional biologicals. Although a portion of the conventionally treated group escalated with IFX, no such treatment success was subsequently achieved.Citation123 In another study, the effects of IFX therapy on the microbiome were investigated. This showed an increase in the diversity and abundance of short-chain fatty acid-producing bacteria and a concomitant decrease in pathogenic bacteria such as Fusobacterium, Enterobacter, and Escherichia. An increase in Lachnospiraceae (e.g., Roseburia and Lachnospira) and Blautia was associated with successful IFX therapy after six weeks. At the phylum level, an increase in Bacteroidetes was also noted. Measuring the stool microbiota could be useful as a biomarker for therapeutic success: using increase in these taxa as parameter, clinical response could be predicted at 14 and 30 weeks with 83.4% and 84.2% accuracy, respectively, and endoscopic response at 30 weeks with 89.1% accuracy.
Overall, dysbiosis was reduced by the IFX-induced alteration of the microbiome, leading to an improvement in disease progression.Citation97
A further study examined the changes in the microbiome and metabolic activities in children with CD who received IFX therapy with varying clinical outcomes (). Pediatric CD patients have a lower relative abundance of SCFA-producing bacteria (including Faecalibacterium, Clostridium clusters IV and XIVb, Roseburia, and Ruminococcus) and thus SCFAs. In addition, alteration of bile acid balance results in decreased relative abundance of Bifidobacterium and Clostridium clusters IV and XIVb, which contain genes for bile salt hydrolase (BSH). Treatment with IFX led to an accumulation of BSH-producing bacteria in patients. In addition, sustained response to IFX therapy was associated with higher numbers of Methylobacterium, Sphingomonas, Staphylococcus, and Streptococcus. Enrichment of SCFA- and BSH-producing bacteria can curb the inflammatory response and restore bile acid metabolism.Citation98
In addition, there are other monoclonal antibodies such as ustekinumab against interleukin-12 and IL-23 or risankizumab, guselkumab, mirikizumab and brazikumab against IL-23, the last three of which have so far only been tested in clinical trials and have not been approved as therapeutic agents for CD yet.Citation124
Since 2016, ustekinumab has been approved for the treatment of moderate to severe CD in adults. This is a human monoclonal antibody against the common p40 subunit of IL-12 and 23. IL-23 plays an important role in T cell activation and acts as an expansion driver and signaling agent for Th17 cell survival. Balzola et al.Citation121 investigated the effect of ustekinumab as a function of intravenous dose in a study of adult patients with moderate-to-severe CD who did not respond to treatment with TNF-α inhibitors. 526 patients were selected for the study and received either ustekinumab (1, 3 or 6 mg/kg body weight) or placebo at baseline. The 145 patients who responded to ustekinumab received a subcutaneous injection of ustekinumab (90 mg) or placebo after six weeks. The proportion of patients who met the primary endpoint at six weeks was 36.6%, 34.1%, and 39.7% for 1, 3, and 6 mg of ustekinumab per kilogram, respectively, compared with 23.5% for placebo. Ustekinumab therapy resulted in higher rates of clinical remission (41.7% vs. 27.4%) and response (69.4% vs. 42.5%) at 22 weeks compared with placebo. Patients with resistance to TNF-α inhibitors thus responded very well to treatment with ustekinumab.
Sandborn et al.Citation125 conducted a five-year study to evaluate the long-term efficacy, safety, and immunogenicity of subcutaneous ustekinumab maintenance therapy in patients with CD in the IM-UNITI trial and long-term extension (LTE). At week 252, 34.4% of patients who received an injection every eight weeks and 28.7% of patients who received an injection every 12 weeks were in clinical remission. The remission rate was even higher (44%) in patients not treated with TNF-α inhibitors.
Doherty et al.Citation99 examined the relationship between the microbiome and response to therapy in patients treated with ustekinumab (UST) in the phase 2 CERTIFI trial (). Overall, microbiome diversity increased significantly in those who responded to therapy throughout the 22-week period. On the one hand, there were operational taxonomic units (OTUs) that were ubiquitous among subjects throughout the period, such as Faecalibacterium, Escherichia, or Shigella. The latter two have been shown to be associated with CD and promote the local inflammatory response. On the other hand, patients who were in remission six weeks after treatment could be distinguished from those with active disease based on OTUs such as Blautia, Clostridium XIVa, Ruminococcaceae, Roseburia, Bacteroides, and Faecalibacterium. All these bacteria were present in higher median relative abundance in patients in remission than in patients with active disease. A decrease in frequency was observed for Escherichia/Shigella. The median diversity of the gut microbiome was also 1.7 times higher in these patients six weeks after treatment than in treated patients with active disease. It is worth noting that the microbiome at baseline showed differences between patients and may be considered as a possible prognostic factor: Two OTUs associated with Faecalibacterium and Bacteroides were significantly more common at baseline in patients in remission six weeks after treatment than in patients with active CD. Faecalibacterium was detectable at baseline in all patients in remission six weeks after UST therapy. This supports the theory established in other studies that Faecalibacterium has an impact on disease pathogenesis through its anti-inflammatory properties.Citation99
Risankizumab has been approved as a monoclonal antibody against the common p19 subunit of IL-23 as an induction therapy for CD in adults since November 2022. Risankizumab was evaluated for safety and efficacy prior to approval in studies by D’Haens et al.Citation126 and Ferrante et al.,Citation127 the results are presented here:
ADVANCE (A) (n = 931 patients) and MOTIVATE (M) (n = 618 patients) were randomized, double-blind, placebo-controlled phase 3 trials. Patients between 16 and 80 years of age with moderate-to-severe CD who had previously demonstrated intolerance or inadequate response to conventional therapy (ADVANCE) or to therapy with biologics (MOTIVATE) participated. These received either a single intravenous dose of 600 mg risankizumab (A: n = 373; M: n = 206) or 1200 mg risankizumab (A: n = 372; M: n = 205) or placebo (A: n = 373; M: n = 207) at weeks 0, 4, and 8. The study covered a total period of 12 weeks. The primary analysis population included 850 participants in ADVANCE and 569 participants in MOTIVATE. In both the ADVANCE study and the MOTIVATE study, the results were similar. Thus, clinical remission rates averaged 44% with risankizumab 600 mg (A: 152/336; M: 80/191) and 41% with risankizumab 1200 mg (A: 141/339; M: 77/191) compared to 22% (A: 43/175; M: 37/187) with placebo. Endoscopic remission rates averaged 36% (A: 135/336; M: 55/191) with risankizumab 600 mg and 33% with risankizumab 1200 mg (A: 109/339; M: 65/191) compared with 12% (A: 21/175; M: 21/187) with placebo. Overall, the therapy with risankizumab showed significant efficacy and was well tolerated by the patients as induction therapy.Citation126 Remarkably, the higher dosage of 1200 mg risankizumab did not seem to increase the efficacy of the therapy, but rather slightly decreased it.
In a third randomized study, FORTIFY sub-study 1, risankizumab was tested as remission maintenance therapy. In this study, patients received subcutaneous administration of 180 mg or 360 mg risankizumab or subcutaneous placebo every eight weeks. The study covered a total period of 52 weeks. When given 360 mg risankizumab, 52% (74/141) of patients achieved clinical remission and 47% (66/141) achieved endoscopic remission. In the placebo group, 41% (67/164) of patients achieved clinical remission and 22% (36/164) achieved endoscopic remission. 180 mg risankizumab resulted in clinical remission in 55% (87/141) of patients and endoscopic remission in 47% (74/157). The incidence of adverse events was similar in both groups, and the adverse events most often reported in all treatment groups were worsening disease activity, arthralgia, and headache.The results show that subcutaneously administered risankizumab is a safe and effective treatment for remission maintenance in patients with moderately to severely active CD. Thus, it offers a new therapeutic option for a broad spectrum of patients and could change the future course of the disease.Citation127 Now, multi-year studies are needed to evaluate the long-term effects of maintaining remission with risankizumab.
For both risankizumab and guselkumab, studies describing the effects of these therapies on the patient microbiome and the consequences for the disease process are not yet available. Given the effects of IFX and UST on the microbiome of diseased individuals, it remains to be seen whether similar results can be expected for risankizumab, guselkumab, and the other monoclonal antibodies.
Antibiotic therapy in CD and its effects on the microbiome
Antibiotics are used to treat complications such as abscesses, fistulas or PSC in CD and are usually only an adjunct to other therapies.
Ciprofloxacin, possibly in combination with metronidazole as an adjunct to biologic therapy, azithromycin, and rifaximin are used in this setting.Citation41,Citation128,Citation129 Vancomycin and metronidazole have been shown to be effective in the treatment of PSC.Citation130 The question of whether the administration of these antibiotics promotes or counteracts dysbiosis is controversial and cannot be answered unequivocally at present.
The therapies used in the studies to treat abscesses and fistulas focused on broad-spectrum antibiotics, usually consisting of metronidazole and ciprofloxacin for several weeks.Citation41,Citation131,Citation132 For example, a case report examining the use of ciprofloxacin and metronidazole showed that the intestinal mucosa recovered in patients with a severe endoscopic finding, and another study recorded a decrease in C-reactive protein.Citation133,Citation134 However, it was also observed that the symptoms reappeared after discontinuation of the antibiotics. In children, there are very few studies on this topic, which could be related to the many undesirable side effects.Citation135 A study examining the resistance pattern and clinical impact of ciprofloxacin in intra-abdominal abscesses from 78 Crohn’s patients found that more than two-thirds of the isolated Gram-negative aerobes were resistant to ciprofloxacin. Here, the high resistance patterns in the clinical setting represent a possible reason for non-response to therapy.Citation136
Primary sclerosing cholangitis is a chronic, inflammatory, fibrostenotic, and idiopathic liver disease characterized by dilatation and stricture of hepatic bile ducts leading to portal hypertension, cirrhosis, and liver failure with high morbidity and mortality.Citation137,Citation138 Up to 76% to 90% of PSC patients have concurrent IBD.Citation139 In a randomized double-blind study with 35 PSC patients, the effects of vancomycin and metronidazole were tested. For this purpose, patients were divided into four groups: Vancomycin 125 mg or 250 mg four times/day or metronidazole 250 mg or 500 mg three times/day for 12 weeks. The primary endpoint was defined by a decrease in alkaline phosphatase at week 12, which was achieved only by both vancomycin groups. The secondary endpoint was defined by a decrease in bilirubin and Mayo-PSC risk score (including variceal bleeding, age and biochemical tests; used by clinicians to advise patients with PSC regarding their prognosis) and a decrease in adverse events and pruritus. Both bilirubin levels and Mayo-PSC risk score decreased in the low-dose metronidazole and vancomycin groups.Citation140,Citation141 Pruritus decreased significantly in the high-dose metronidazole group. Adverse events requiring drug discontinuation occurred in six patients (four of whom received metronidazole). Both vancomycin and metronidazole were found to be well efficacious in this study, but long-term studies with larger numbers of patients are needed to better assess the efficacy and safety of both drugs.Citation130 In a triple-blind, randomized, placebo-controlled trial, oral vancomycin (125 mg, four times daily) was tested in 29 patients with PSC for 12 weeks. After the three months, there was a significant decrease in PSC Mayo risk score, alkaline phosphatase, and gamma-glutamyl transferase. Symptoms such as fatigue, pruritus, diarrhea, and loss of appetite also improved significantly. Since only low-dose vancomycin was used, no adverse side effects occurred in this study either. However, it should also be noted here that the number of patients participating in the study was small, so that only limited meaningful conclusions were possible.Citation137 In a 2020 randomized controlled trial, 16 children with acute severe colitis (ASC) were tested for two weeks on a cocktail of four antibiotics (amoxicillin, vancomycin, metronidazole, doxycycline/ciprofloxacin) administered concomitantly with intravenous CS (IVCS+AB). The comparison group, which included 12 children, received only intravenous CS (IVCS). The primary end point was the PUCAI score (Pediatric Ulcerative Colitis Activity Index) on day 5, which was ≥ 65 before treatment and averaged 25 ± 16.7 and 40.4 ± 20.4, respectively, on day 5. Before treatment, some of the children had dysbiosis of the microbiome with increased abundance of gamma proteobacteria such as Escherichia coli, Klebsiella pneumoniae, and Haemophilus parainfluenzae. Proteobacteria decreased in the IVCS-treated group; however, they continued to dominate in the IVCS+AB-treated group, and the diversity of the microbiome did not increase in either group, but actually decreased. Thus, in the IVCS+AB group, there was no association between the microbiome at baseline and clinical response five days later. One hypothesis of the study investigators is that the so-called “Jerusalem cocktail” led to a general decrease in bacterial diversity and did not affect individual species, so no relative changes were observed here. Secondary endpoints such as remission rate and calprotectin levels were numerically better in the intervention group but did not reach statistical significance in this small study.Citation142 Although this study only involved patients with ulcerative colitis, the Jerusalem cocktail is already being used in IBD situations, where previous studies have shown the benefit of antibiotics such as vancomycin, metronidazole, and ciprofloxacin.
As a non-absorbable antibiotic, rifaximin is an alternative to systemic antibiotics. Some studies have already tested and confirmed its efficacy in both adults and children with IBD and it is well tolerated.Citation131,Citation143,Citation144 Moreover, in combination with immunomodulators, it acts on the disrupted intestinal barrier at both microbiological and immunological levels. In studies, rifaximin was observed to increase the concentration of Bifidobacteria and F. prausnitzii, improve barrier function, and reduce visceral hypersensitivity.Citation145,Citation146 A meta-analysis suggests that antibiotic therapy can induce remission in active CD and prevent relapse in patients with quiescent CD.Citation147
It has already been shown that the antibiotics used can lead to an alteration in microbial metabolism with an increase in SCFAs, and the bacterial enzyme activities thus altered have been shown to correlate with the clinical response of patients. Antibiotics can also reduce bacterial invasion of tissues, heal microabscesses, and prevent bacterial translocation and systemic spread. In addition, antibiotics have been shown to have direct immunomodulatory properties, such as antagonizing the effect of TNF-α on epithelial cells.Citation146,Citation148,Citation149
Interestingly, the use of fluoroquinolones such as ciprofloxacin indirectly modulates the immune response by curbing the production of proinflammatory cytokines such as IL-1, IL-6, TNF-α, and IL-2, resulting in decreased activity of T and B lymphocytes. They also affect the expression of other cytokines and mediators related to the immune response.Citation150
Lewis et al.Citation32 documented that EEN led to an increase in Alistipes that was reversed by subsequent antibiotic therapy, raising the possibility that antibiotics antagonize the beneficial effects of EEN. Antibiotics are direct toxins to bacteria and therefore may promote fungal growth, which was also observed in this study. Thus, there was an increase in five fungi, including Candida, Clavispora, Cyberlindnera, Kluyveromyces, and Saccharomyces. This now leads to the hypothesis that antibiotics in the context of CD may promote bacterial dysbiosis, thereby supporting the growth of fungi and ultimately perpetuating the dysbiosis.Citation151
One possible explanation for the contradictory results regarding the effect of antibiotics could be the antibiotic used itself, but also the course of treatment, preexisting antibiotic resistance, and individual disease pathogenesis, which is influenced by external environmental factors, genetics, and gut microbial colonization, among other factors. All in all, it is difficult to draw firm conclusions from these studies, and therefore current guidelines rightly do not support the routine use of antibiotics in the treatment of CD and UC.Citation41
The juvenile and adolescent microbiome under the influence of therapy in CD
Kansal et al.Citation67 examined the microbiome of 204 children using biopsies from the ileum and colon/rectum. As described above, the authors divided the patients into groups and found several bacteria that differed significantly in number between CDFD and healthy controls, with Fusobacterium, Veillonella spp., Clostridium spp., Epulopiscium spp., Propionibacterium acnes, and H. parainfluenzae being the most prevalent. The CDRM group was dominated by Pseudoflavinofractor capillosus, Lactobacillus casei and Lactobacillus gasseri (the last two: phylum Firmicutes). Pseudoflavinofractor capillosus belongs to the healthy intestinal flora of humans, the other two have a positive effect on the intestinal flora and are also used in probiotics. In the CDRL group, two bacteria were strongly associated with relapse: Hespellia porcina (phylum: Firmicutes) (in 56.8% of all CDRL samples) and Eubacterium fissicatena (phylum: Firmicutes) (in 42.0% of all CDRL and in 45.8% of all CDRM samples). All patients in this study received therapy that included either oral corticosteroids or EEN for six weeks, followed by an immunomodulator such as azathioprine, 6-mercaptopurine or methotrexate or alternatively a biological (monoclonal antibodies). In a minority of patients, further therapy in the form of surgery was required. It is worth mentioning in this context that the microbiome of the patients from the CDRM group was not completely similar to that of the healthy control group. Thus, the microbiome of patients from the CDRL group also did not resemble the microbiome of the CDFD group because, among other things, Fusobacterium was not associated with the CDRL group but with the CDFD group. Rather, the microbiome at the time of relapse appears to be a consequence of factors such as ongoing inflammation and treatment, and cannot be used to predict potential relapse. Thus, therapy affects the microbiome both during remission and relapse, and dysbiosis is maintained to some degree by the drugs administered.Citation67
To-treat/with what to treat
The call for precision medicine and the increasing number of therapeutic interventions as described above, paired with scarcity of large-volume data from pediatric CD patients are not well suited to simplify the task of shared decision of treatment for the individual patient.
The problem of accessible complex data including microbiota and metabolomics is increased with combination of therapies including multimodal treatment (combination of immunomodulators/biologics/nutrition, that patients seem to benefit from and the risk of long-term complications with complex immunosuppression. Also timing of therapeutic intervention is crucial, with a (widely unknown) window of opportunity to achieve long-term steroid free remission. The current discussion focusses on reaching new, more objective, but also hard to reach targets (histological remission, transmural healing). This requires risk stratification, better phenotyping of patients and long-term data collection from real-world observation. The studies of the last years have led to the recommendation of early biologics in complicated cases (predictors of poor outcome), intensified dosing regimes of biologics and an earlier recommendation of surgery in isolated, stricturing L1 CD. It has also broadened the spectrum of nutritional interventions (e.g., CDED) with more acceptability to patients and parents. Also, the question of care in low to medium income countries has to be addressed, where biologics therapy may be costly and microbiota aimed nutrition is more accessible. The jury is still out, but they are gathering data, to soon improve care for all affected families.
Conclusion
The gut microbiome in inflammatory bowel diseases and especially CD remains highly relevant in western health care systems.
It is a key aspect in pathogenesis including its direct effects and metabolic interaction with the mucosa and associated immune response. Therapeutic manipulation of the gut microbiome, mainly by nutrition, immunomodulation and antibiotic treatment is increasingly in the focus of precision medicine approaches. It is important to keep these effects in mind when evaluating modern treatment options, that influence the microbiome through their targets in modulating the immune response. The treat-to-target concept has outlined the goal of deep remission that is not easily achieved by any known intervention alone. Currently combinations of different strategies are under evaluation in nutrition and medical treatment, stressing further the complexity for trial design and requirement of size and duration of observation. Understanding the role of the mucosal barrier and its interactions with the intestinal microbiome may enable specific medical interventions that may prove pivotal to disrupt pathogenic mechanisms in this major chronic inflammatory disease of modern times.
Author contribution
KF drafted the manuscript and designed the figures and tables. TH and JdL contributed in designing, reviewing, and editing the manuscript. MW, BO, MF and SS added intellectual content and critically revised the manuscript. The authors approved the final manuscript for publication.
Acknowledgments
KF thanks Tanja Fetter for her most valuable support in preparing the figures. Figures were created with BioRender and Microsoft PowerPoint 2019 and tables were created with Microsoft Excel 2019.
Disclosure statement
No potential conflict of interest was reported by the author(s).
Additional information
Funding
References
- Yu YR, Rodriguez JR. Clinical presentation of Crohn’s, ulcerative colitis, and indeterminate colitis: symptoms, extraintestinal manifestations, and disease phenotypes. Semin Pediatr Surg. 2017;26(6):349–23. doi:10.1053/j.sempedsurg.2017.10.003.
- Rahmani P, Rasti G, Gorgi M, Motamed F, Sharifi P. Extraintestinal manifestation of inflammatory bowel disease and associated factors in pediatric patients. Ann Med Surg. 2022;75(February):103363. doi:10.1016/j.amsu.2022.103363.
- Rogler G, Singh A, Kavanaugh A, Rubin DT. Extraintestinal manifestations of inflammatory bowel disease: current concepts, treatment, and implications for disease management. Gastroenterology. 2021;161(4):1118–1132. doi:10.1053/J.GASTRO.2021.07.042.
- de Laffolie J. Epidemiological research and treatment data analysis for chronic inflammatory bowel diseases: improvement in care of children and adolescents. Monatsschr Kinderheilkd. 2020;168(4):298–313. doi:10.1007/s00112-020-00852-9.
- Øyri SF, Muzes G, Sipos F. Dysbiotic gut microbiome: a key element of Crohn’s disease. Comp Immunol Microbiol Infect Dis. 2015;43:36–49. doi:10.1016/j.cimid.2015.10.005.
- Tiffany CR, Bäumler AJ. Dysbiosis: from fiction to function. Am J Physiol - Gastrointest Liver Physiol. 2019;317(5):G602–8. doi:10.1152/AJPGI.00230.2019.
- Levy M, Kolodziejczyk AA, Thaiss CA, Elinav E. Dysbiosis and the immune system. Nat Rev Immunol. 2017;17(4):219–232. doi:10.1038/nri.2017.7.
- Petersen C, Round JL. Defining dysbiosis and its influence on host immunity and disease. Cell Microbiol. 2014;16(7):1024–1033. doi:10.1111/cmi.12308.
- Proctor L. What‗s next for the human microbiome? Nature. 2019;569(7758):623. doi:10.1038/d41586-019-01654-0.
- Adolph TE, Meyer M, Schwärzler J, Mayr L, Grabherr F, Tilg H. The metabolic nature of inflammatory bowel diseases. Nat Rev Gastroenterol Hepatol. 2022;19(12):753–767. doi:10.1038/s41575-022-00658-y.
- Uchiyama K, Haruyama Y, Shiraishi H, Katahira K, Abukawa D, Ishige T, Tajiri H, Uchida K, Uchiyama K, Washio M, et al. Association between passive smoking from the mother and pediatric crohn’s disease: a Japanese multicenter study. Int J Environ Res Public Health. 2020;17(8):2926. doi:10.3390/ijerph17082926.
- Mahid SS, Minor KS, Stromberg AJ, Galandiuk S. Active and passive smoking in childhood is related to the development of inflammatory bowel disease. Inflamm Bowel Dis. 2007;13(4):431–438. doi:10.1002/ibd.20070.
- Salih A, Widbom L, Hultdin J, Karling P. Smoking is associated with risk for developing inflammatory bowel disease including late onset ulcerative colitis: a prospective study. Scand J Gastroenterol. 2018;53(2):173–178. doi:10.1080/00365521.2017.1418904.
- Troelsen FS, Jick S. Antibiotic use in childhood and adolescence and risk of inflammatory bowel disease: a case-control study in the UK clinical practice Research datalink. Inflamm Bowel Dis. 2020;26(3):440–447. doi:10.1093/ibd/izz137.
- Virta L, Auvinen A, Helenius H, Huovinen P, Kolho KL. Association of repeated exposure to antibiotics with the development of pediatric crohn’s disease - a nationwide, register-based Finnish case-control study. Am J Epidemiol. 2012;175(8):775–784. doi:10.1093/aje/kwr400.
- Korelitz BI. Role of nonsteroidal anti-inflammatory drugs in exacerbation of inflammatory bowel disease. J Clin Gastroenterol. 2016;50(2):97–98. doi:10.1097/MCG.0000000000000444.
- Ananthakrishnan AN, Higuchi LM, Huang ES, Khalili H, Richter JM, Fuchs CS, Chan AT. Aspirin, nonsteroidal anti-inflammatory drug use, and risk for Crohn disease and ulcerative colitis. Ann Intern Med. 2012;156(5):350–359. doi:10.7326/0003-4819-156-5-201203060-00007.
- Khalili H, Higuchi LM, Ananthakrishnan AN, Richter JM, Feskanich D, Fuchs CS, Chan AT. Oral contraceptives, reproductive factors and risk of inflammatory bowel disease. Gut. 2013;62(8):1153–1159. doi:10.1136/gutjnl-2012-302362.
- Ek WE, D’Amato M, Halfvarson J. The history of genetics in inflammatory bowel disease. Ann Gastroenterol. 2014;27:294–303.
- Van Limbergen J, Wilson DC, Satsangi J. The genetics of Crohn’s disease. Annu Rev Genom Hum Genet. 2009;10(1):89–116. doi:10.1146/annurev-genom-082908-150013.
- Thaiss CA, Zmora N, Levy M, Elinav E. The microbiome and innate immunity. Nature. 2016;535(7610):65–74. doi:10.1038/nature18847.
- Kaser A, Zeissig S, Blumberg RS. Inflammatory bowel disease. Annu Rev Immunol. 2010;28:573–621. doi:10.1146/annurev-immunol-030409-101225.
- Abraham C, Cho JH. T 2066. Bmj. 2009;361(21):2066–2078. doi:10.1056/NEJMra0804647.
- Cui X, Ye Z, Wang D, Yang Y, Jiao CH, Ma J, Tang N, Zhang H. Aryl hydrocarbon receptor activation ameliorates experimental colitis by modulating the tolerogenic dendritic and regulatory T cell formation. Cell & Biosci. 2022;12(1):1–13. doi:10.1186/s13578-022-00780-z.
- Davidson M, Saiki J. A novel gut-restricted aryl hydrocarbon receptor agonist with activity in the dextran sodium sulfate colitis murine model. Inflamm Bowel Dis. 2023;29(Supplement_1):S3–S3. doi:10.1093/ibd/izac247.006.
- Petagna L, Antonelli A, Ganini C, Bellato V, Campanelli M, Divizia A, Efrati C, Franceschilli M, Guida AM, Ingallinella S, et al. Pathophysiology of Crohn’s disease inflammation and recurrence. Biol Direct. 2020;15(1):1–10. doi:10.1186/s13062-020-00280-5.
- Cococcioni L, Panelli S, Varotto-Boccazzi I, Carlo D, Pistone D, Leccese G, Zuccotti GV, Comandatore F. Ibds and the pediatric age: their peculiarities and the involvement of the microbiota. Diges Liver Dis. 2021;53(1):17–25. doi:10.1016/j.dld.2020.10.033.
- de Alencar Junior H, Paiotti APR, de Araújo Filho HB, Oshima CTF, Miszputen SJ, Ambrogini-Júnior O. The relationship between the commensal microbiota levels and Crohn’s disease activity. JGH Open. 2020;4(5):784–789. doi:10.1002/jgh3.12338.
- Torres J, Mehandru S, Colombel JF, Peyrin-Biroulet L. Crohn’s disease. Lancet. 2017;389(10080):1741–1755. doi:10.1016/S0140-6736(16)31711-1.
- Hansen R, Russell RK, Reiff C, Louis P, McIntosh F, Berry SH, Mukhopadhya I, Bisset MW, Barclay AR, Bishop J, et al. Microbiota of de-novo pediatric IBD: increased faecalibacterium prausnitzii and reduced bacterial diversity in Crohn’s but not in ulcerative colitis. Am J Gastroenterol. 2012;107(12):1913–1922. doi:10.1038/ajg.2012.335.
- Frank DN, St. Amand AL, Feldman RA, Boedeker EC, Harpaz N, Pace NR. Molecular-phylogenetic characterization of microbial community imbalances in human inflammatory bowel diseases. Proc Natl Acad Sci USA. 2007;104(34):13780–13785. doi:10.1073/pnas.0706625104.
- Lewis JD, Chen EZ, Baldassano RN, Otley AR, Griffiths AM, Lee D, Bittinger K, Bailey A, Friedman E, Hoffmann C, et al. Inflammation, antibiotics, and diet as environmental stressors of the gut microbiome in pediatric Crohn’s disease. Cell Host & Microbe. 2015;18(4):489–500. doi:10.1016/j.chom.2015.09.008.
- Gastroenterol WJ. Emerging therapeutic options in inflammatory bowel disease. Contents. 2021;9327(48):8242–8261).
- Ashton JJ, Gavin J, Beattie RM. Exclusive enteral nutrition in Crohn’s disease: evidence and practicalities. Clin Nutr. 2019;38(1):80–89. doi:10.1016/j.clnu.2018.01.020.
- Heuschkel R, Pender S, Paintin M, Arnaud-Battandier F, Walker J, MacDonald T. Imbalance of stromelysin-1 and timp-1 mRNA and protein in the mucosal lesions of children with inflammatory bowel disease. J Pediatr Gastroenterol Nutr. 1999;28(5):557. doi:10.1097/00005176-199905000-00073.
- Xiao F, Gao X, Hu H, Le J, Chen Y, Shu X, Liang Z, Xu Y, Wang Y, Zhang T. Exclusive enteral nutrition exerts anti-inflammatory effects through modulating microbiota, bile acid metabolism, and immune activities. Nutr. 2022;14(21):4463. doi:10.3390/nu14214463.
- Weingarden AR, Vaughn BP. Intestinal microbiota, fecal microbiota transplantation, and inflammatory bowel disease. Gut Microbes. 2017;8(3):238–252. doi:10.1080/19490976.2017.1290757.
- Khanna S, Raffals LE. The microbiome in Crohn’s disease: role in pathogenesis and role of microbiome replacement therapies. Gastroenterol Clin North Am. 2017;46(3):481–492. doi:10.1016/j.gtc.2017.05.004.
- Gruszecka J, Filip R. Does anti-TNF-α therapy affect the bacteriological profile of specimens collected from perianal lesions? A retrospective analysis in patients with Crohn’s disease. Int J Environ Res Public Health. 2022;19(5):2892. doi:10.3390/ijerph19052892.
- Fousekis FS, Theopistos VI, Tsianos KH, Katsanos EV, Christodoulou DK. Hepatobiliary manifestations and complications in inflammatory bowel disease: a review. Gastroenterol Res. 2018;11(2):83–94. doi:10.14740/gr990w.
- Ledder O, Turner D. Antibiotics in IBD: still a role in the biological era? Inflamm Bowel Dis. 2018;24(9):1676–1688. doi:10.1093/ibd/izy067.
- Wilschanski M, Sherman P, Pencharz P, Davis L, Corey M, Griffiths A. Supplementary enteral nutrition maintains remission in paediatric Crohn’s disease. Gut. 1996;38(4):543–548. doi:10.1136/gut.38.4.543.
- Levine A, Wine E, Assa A, Sigall Boneh R, Shaoul R, Kori M, Cohen S, Peleg S, Shamaly H, On A, et al. Crohn’s disease exclusion diet plus partial enteral nutrition induces sustained remission in a randomized controlled trial. Gastroenterol. 2019;157(2):440–450.e8. doi:10.1053/j.gastro.2019.04.021.
- Hollister EB, Gao C, Versalovic J. Compositional and functional features of the gastrointestinal microbiome and their effects on human health. Gastroenterol. 2014;146(6):1449–1458. doi:10.1053/j.gastro.2014.01.052.
- Arumugam M, Raes J, Pelletier E, Paslier D, Yamada T, Mende DR, Fernandes GR, Tap J, Bruls T, Batto JM, et al. Enterotypes of the human gut microbiome. Nature. 2011;473(7346):174–180. doi:10.1038/nature09944.
- Eckburg PB, Bik EM, Bernstein CN, Purdom E, Dethlefsen L, Sargent M, Gill SR, Nelson KE, Relman DA. Diversity of the human intestinal microbial flora. Sci. 2005;308(5728):1635–1638. doi:10.1126/science.1110591.
- Harrell L, Wang Y, Antonopoulos D, Young V, Lichtenstein L, Huang Y, Hanauer S, Chang E. Standard colonic lavage alters the natural state of mucosal-associated microbiota in the human colon. PLoS One. 2012;7(2):e32545. doi:10.1371/journal.pone.0032545.
- Maharshak N, Ringel Y, Katibian D, Lundqvist A, Sartor RB, Carroll IM, Ringel-Kulka T. Fecal and mucosa-associated intestinal microbiota in patients with diarrhea-predominant irritable bowel syndrome. Dig Dis Sci. 2018;63(7):1890–1899. doi:10.1007/s10620-018-5086-4.
- Klymiuk I, Singer G, Castellani C, Trajanoski S, Obermüller B, Till H. Characterization of the luminal and mucosa-associated microbiome along the gastrointestinal tract: results from surgically treated preterm infants and a murine model. Nutr. 2021;13(3):1030. doi:10.3390/nu13031030.
- Kashiwagi S, Naito Y, Inoue R, Takagi T, Nakano T, Inada Y, Fukui A, Katada K, Mizushima K, Kamada K, et al. Mucosa-associated microbiota in the gastrointestinal tract of healthy Japanese subjects. Digest. 2020;101(2):107–120. doi:10.1159/000496102.
- Kim H, Sitarik AR, Woodcroft K, Johnson CC, Zoratti E. Birth mode, breastfeeding, pet exposure, and antibiotic use: associations with the gut microbiome and sensitization in children. Curr Allergy Asthma Rep. 2019;19(4). doi:10.1007/s11882-019-0851-9.
- Haro C, Rangel-Zúñiga OA, Alcalá-Díaz JF, Gómez-Delgado F, Pérez-Martínez P, Delgado-Lista J, Quintana-Navarro GM, Landa BB, Navas-Cortés JA, Tena-Sempere M, et al. Intestinal microbiota is influenced by gender and body mass index. PLoS One. 2016;11(5):1–16. doi:10.1371/journal.pone.0154090.
- Koliada A, Syzenko G, Moseiko V, Budovska L, Puchkov K, Perederiy V, Gavalko Y, Dorofeyev A, Romanenko M, Tkach S, et al. Association between body mass index and Firmicutes/Bacteroidetes ratio in an adult Ukrainian population. BMC Microbiol. 2017;17(1):4–9. doi:10.1186/s12866-017-1027-1.
- Yun Y, Kim HN, Kim SE, Heo SG, Chang Y, Ryu S, Shin H, Kim H-L. Comparative analysis of gut microbiota associated with body mass index in a large Korean cohort. BMC Microbiol. 2017;17(1):1–9. doi:10.1186/s12866-017-1052-0.
- Azad MB, Konya T, Persaud RR, Guttman DS, Chari RS, Field CJ, Sears MR, Mandhane PJ, Turvey SE, Subbarao P, et al. Impact of maternal intrapartum antibiotics, method of birth and breastfeeding on gut microbiota during the first year of life: a prospective cohort study. BJOG: Int J Obstet Gy. 2016;123(6):983–993. doi:10.1111/1471-0528.13601.
- Ringel-Kulka T, Cheng J, Ringel Y, Salojärvi J, Carroll I, Palva A, de Vos WM, Satokari R. Intestinal microbiota in healthy U.S. Young children and adults—a high throughput microarray analysis. PLoS One. 2013;8(5):e64315. doi:10.1371/journal.pone.0064315.
- Claesson MJ, Cusack S, O’Sullivan O, Greene-Diniz R, De Weerd H, Flannery E, Marchesi JR, Falush D, Dinan T, Fitzgerald G, et al. Composition, variability, and temporal stability of the intestinal microbiota of the elderly. Proc Natl Acad Sci USA. 2011;108(SUPPL. supplement_1):4586–4591. doi:10.1073/pnas.1000097107.
- Biagi E, Franceschi C, Rampelli S, Severgnini M, Ostan R, Turroni S, Consolandi C, Quercia S, Scurti M, Monti D, et al. Gut microbiota and extreme longevity. Curr Biol. 2016;26(11):1480–1485. doi:10.1016/j.cub.2016.04.016.
- Drago L, Toscano M, Rodighiero V, De Vecchi E, Mogna G. Cultivable and pyrosequenced fecal microflora in centenarians and young subjects. J Clin Gastroenterol. 2012;46(SUPPL. 1):81–84. doi:10.1097/MCG.0b013e3182693982.
- Nishida A, Inoue R, Inatomi O, Bamba S, Naito Y, Andoh A. Gut microbiota in the pathogenesis of inflammatory bowel disease. Clin J Gastroenterol. 2018;11(1):1–10. doi:10.1007/s12328-017-0813-5.
- Dominguez-Bello MG, Godoy-Vitorino F, Knight R, Blaser MJ. Role of the microbiome in human development. Gut. 2019;68(6):1108–1114. doi:10.1136/gutjnl-2018-317503.
- Koboziev I, Karlsson F, Grisham MB. Gut-associated lymphoid tissue, T cell trafficking, and chronic intestinal inflammation. Ann N Y Acad Sci. 2010;1207(SUPPL.1):86–93. doi:10.1111/j.1749-6632.2010.05711.x.
- Agace WW. T-cell recruitment to the intestinal mucosa. Trends Immunol. 2008;29(11):514–522. doi:10.1016/j.it.2008.08.003.
- Torres J, Hu J, Seki A, Eisele C, Nair N, Huang R, Tarassishin L, Jharap B, Cote-Daigneault J, Mao Q, et al. Infants born to mothers with IBD present with altered gut microbiome that transfers abnormalities of the adaptive immune system to germ-free mice. Gut. 2020;69(1):42–51. doi:10.1136/gutjnl-2018-317855.
- Kim ES, Tarassishin L, Eisele C, Barre A, Nair N, Rendon A, Hawkins K, Debebe A, White S, Thjømøe A, et al. Longitudinal changes in Fecal calprotectin levels among pregnant women with and without inflammatory bowel disease and their babies. Gastroenterol. 2021;160(4):1118–1130.e3. doi:10.1053/J.GASTRO.2020.11.050.
- Gevers D, Kugathasan S, Denson LA, Vázquez-Baeza Y, Van Treuren W, Ren B, Schwager E, Knights D, Song S, Yassour M, et al. The treatment-naive microbiome in new-onset Crohn’s disease. Cell Host & Microbe. 2014;15(3):382–392. doi:10.1016/j.chom.2014.02.005.
- Kansal S, Catto-Smith AG, Boniface K, Thomas S, Cameron DJ, Oliver M, Alex G, Kirkwood C, Wagner J. The microbiome in paediatric Crohn’s disease—a longitudinal, prospective, single-centre study. J Crohns Colitis. 2019;13(8):1044–1054. doi:10.1093/ecco-jcc/jjz016.
- Takahashi K, Nishida A, Fujimoto T, Fujii M, Shioya M, Imaeda H, Inatomi O, Bamba S, Andoh A, Sugimoto M. Reduced abundance of butyrate-producing bacteria species in the Fecal microbial community in Crohn’s disease. Digestion. 2016;93(1):59–65. doi:10.1159/000441768.
- Miquel S, Martín R, Rossi O, Bermúdez-Humarán LG, Chatel JM, Sokol H, Thomas M, Wells JM, Langella P. Faecalibacterium prausnitzii and human intestinal health. Curr Opin Microbiol. 2013;16(3):255–261. doi:10.1016/j.mib.2013.06.003.
- Sokol H, Pigneur B, Watterlot L, Lakhdari O, Bermúdez-Humarán LG, Gratadoux JJ, Blugeon S, Bridonneau C, Furet J-P, Corthier G, et al. Faecalibacterium prausnitzii is an anti-inflammatory commensal bacterium identified by gut microbiota analysis of Crohn disease patients. Proc Natl Acad Sci U S A. 2008;105(43):16731–16736. doi:10.1073/pnas.0804812105.
- Caparrós E, Wiest R, Scharl M, Rogler G, Gutiérrez Casbas A, Yilmaz B, Wawrzyniak M, Francés R. Dysbiotic microbiota interactions in Crohn’s disease. Gut Microbes. 2021;13(1):1–19. doi:10.1080/19490976.2021.1949096.
- Pascal V, Pozuelo M, Borruel N, Casellas F, Campos D, Santiago A, Martinez X, Varela E, Sarrabayrouse G, Machiels K, et al. A microbial signature for Crohn’s disease. Gut. 2017;66(5):813–822. doi:10.1136/gutjnl-2016-313235.
- Zhao H, Xu H, Chen S, He J, Zhou Y, Nie Y. Systematic review and meta-analysis of the role of faecalibacterium prausnitzii alteration in inflammatory bowel disease. J Gastroenterol Hepatol. 2021;36(2):320–328. doi:10.1111/jgh.15222.
- Barnich N, Aguirre JE, Reinecker HC, Xavier R, Podolsky DK. Membrane recruitment of NOD2 in intestinal epithelial cells is essential for nuclear factor-ΚB activation in muramyl dipeptide recognition. J Cell Biol. 2005;170(1):21–26. doi:10.1083/jcb.200502153.
- Wehkamp J, Stange EF. An update review on the Paneth cell as key to ileal Crohn’s disease. Front Immunol. 2020;11(April). doi:10.3389/fimmu.2020.00646.
- Ashton JJ, Seaby EG, Beattie RM, Ennis S. NOD2 in Crohn’s disease—unfinished business. J Crohns Colitis. 2023;17(3):450–458. doi:10.1093/ecco-jcc/jjac124.
- Gao P, Liu H, Huang H, Sun Y, Jia B, Hou B, Zhou X, Strober W, Zhang F. The Crohn disease-associated ATG16L1T300A polymorphism regulates inflammatory responses by modulating TLR- and NLR-mediated signaling. Autophagy. 2022;18(11):2561–2575. doi:10.1080/15548627.2022.2039991.
- Martinez-Medina M, Aldeguer X, Lopez-Siles M, González-Huix F, López-Oliu C, Dahbi G, Blanco JE, Blanco J, Garcia-Gil JL, Darfeuille-Michaud A. Molecular diversity of Escherichia coli in the human gut: new ecological evidence supporting the role of adherent-invasive E. coli (AIEC) in Crohnʼs disease. Inflamm Bowel Dis. 2009;15(6):872–882. doi:10.1002/ibd.20860.
- Jiang W, Su J, Zhang X, Cheng X, Zhou J, Shi R, Zhang H. Elevated levels of Th17 cells and Th17-related cytokines are associated with disease activity in patients with inflammatory bowel disease. Inflamm Res. 2014;63(11):943–950. doi:10.1007/s00011-014-0768-7.
- Mitsuyama K, Niwa M, Takedatsu H, Yamasaki H, Kuwaki K, Yoshioka S, Yamauchi R, Fukunaga S, Torimura T. Antibody markers in the diagnosis of inflammatory bowel disease. World J Gastroenterol. 2016;22(3):1304–1310. doi:10.3748/wjg.v22.i3.1304.
- Wang JQ, Huang Y. Serological markers of inflammatory bowel disease. World Chinese J Dig. 2013;21(36):4110–4115. doi:10.11569/wcjd.v21.i36.4110.
- Zimmerman L, Bousvaros A. The pharmacotherapeutic management of pediatric Crohn’s disease. Expert Opin Pharmacother. 2019;20(17):2161–2168. doi:10.1080/14656566.2019.1659778.
- Akobeng AK. Crohn’s disease: current treatment options. Arch Dis Child. 2008;93(9):787–792. doi:10.1136/adc.2007.128751.
- Schwermer M, Fetz K, Längler A, Ostermann T, Zuzak TJ. Complementary, alternative, integrative and dietary therapies for children with Crohn’s disease – a systematic review. Complement Ther Med. 2020;52(June):102493. doi:10.1016/j.ctim.2020.102493.
- Brookes MJ, Green JRB. Maintenance of remission in Crohn’s disease: current and emerging therapeutic options. Drugs. 2004;64(10):1069–1089. doi:10.2165/00003495-200464100-00004.
- Bradford K, Shih DQ. Optimizing 6-mercaptopurine and azathioprine therapy in the management of inflammatory bowel disease. World J Gastroenterol. 2011;17(37):4166–4173. doi:10.3748/wjg.v17.i37.4166.
- Dubinsky MC. Azathioprine 6-mercaptopurine in inflammatory bowel disease: pharmacology, efficacy, and safety. Clin Gastroenterol Hepatol. 2004;2(9):731–743. doi:10.1016/S1542-3565(04)00344-1.
- Cronstein BN. The mechanism of action of methotrexate. Rheum Dis Clin North Am. 1997;23(4):739–755. doi:10.1016/S0889-857X(05)70358-6.
- Ludvigsson JF. Antibiotic use and inflammatory bowel disease: number needed to harm? Gut. 2023;(0):gutjnl-2023–329575. doi:10.1136/gutjnl-2023-329575.
- Weisband YL. Association of antibiotic use with durability of biologic agents in inflammatory bowel disease. A Report From The Epi-IIRN. 2023;jjad070(April):1–8.
- Bogatic D, Bryant RV, Lynch KD, Costello SP. Systematic review: microbial manipulation as therapy for primary sclerosing cholangitis. Aliment Pharmacol Ther. 2023;57(1):23–36. doi:10.1111/apt.17251.
- Lafferty L, Tuohy M, Carey A, Sugrue S, Hurley M, Hussey S. Outcomes of exclusive enteral nutrition in paediatric Crohn’s disease. Eur J Clin Nutr. 2017;71(2):185–191. doi:10.1038/ejcn.2016.210.
- Grover Z, Lewindon P. Two-year outcomes after exclusive enteral nutrition induction are superior to corticosteroids in pediatric Crohn’s disease treated early with thiopurines. Dig Dis Sci. 2015;60(10):3069–3074. doi:10.1007/s10620-015-3722-9.
- Dunn KA, Moore-Connors J, Macintyre B, Stadnyk AW, Thomas NA, Noble A, Mahdi G, Rashid M, Otley AR, Bielawski JP, et al. Early changes in microbial community structure are associated with sustained remission after nutritional treatment of pediatric Crohnʼs disease. Inflamm Bowel Dis. 2016;22(12):2853–2862. doi:10.1097/MIB.0000000000000956.
- Lv Y, Lou Y, Liu A, Cheng Q, Yang G, Xu C, Luo Y, Lou J, Yu J, Fang Y, et al. The impact of exclusive enteral nutrition on the gut microbiome and bile acid metabolism in pediatric Crohn’s disease. Clin Nutr. 2023;42(2):116–128. doi:10.1016/J.CLNU.2022.11.018.
- Verburgt CM, Dunn KA, Ghiboub M, Lewis JD, Wine E, Sigall Boneh R, Gerasimidis K, Shamir R, Penny S, Pinto DM, et al. Successful dietary therapy in paediatric Crohn’s disease is associated with shifts in bacterial dysbiosis and inflammatory metabotype towards healthy controls. J Crohns Colitis. 2023;17(1):61–72. doi:10.1093/ecco-jcc/jjac105.
- Zhuang X, Tian Z, Feng R, Li M, Li T, Zhou G, Qiu Y, Chen B, He Y, Chen M, et al. Fecal microbiota alterations associated with clinical and endoscopic response to infliximab therapy in crohn’s disease. Inflamm Bowel Dis. 2020;26(11):1636–1647. doi:10.1093/ibd/izaa253.
- Wang A, Zhang Z, Ding Q, Yang Y, Bindelle J, Ran C, Zhou Z. Intestinal cetobacterium and acetate modify glucose homeostasis via parasympathetic activation in zebrafish. Gut Microbes. 2021;13(1):1–15. doi:10.1080/19490976.2021.1900996.
- Doherty MK, Ding T, Koumpouras C, Telesco SE, Monast C, Das A, Brodmerkel C, Schloss PD. Fecal microbiota signatures are associated with response to ustekinumab therapy among crohn’s disease patients. MBio. 2018;9(2). doi:10.1128/mBio.02120-17.
- González-Torres L, Moreno-Álvarez A, Fernández-Lorenzo AE, Leis R, Solar-Boga A. The role of partial enteral nutrition for induction of remission in Crohn’s disease: a systematic review of controlled trials. Nutrients. 2022;14(24):5263. doi:10.3390/nu14245263.
- Yanai H, Levine A, Hirsch A, Boneh RS, Kopylov U, Eran HB, Cohen NA, Ron Y, Goren I, Leibovitzh H, et al. The Crohn’s disease exclusion diet for induction and maintenance of remission in adults with mild-to-moderate Crohn’s disease (CDED-AD): an open-label, pilot, randomised trial. Lancet Gastroenterol Hepatol. 2022;7(1):49–59. doi:10.1016/S2468-1253(21)00299-5.
- Hart L, Verburgt CM, Wine E, Zachos M, Poppen A, Chavannes M, Van Limbergen J, Pai N. Nutritional therapies and their influence on the intestinal microbiome in pediatric inflammatory bowel disease. Nutr. 2022;14(1):4–22. doi:10.3390/nu14010004.
- Ding Z, Ninan K, Johnston BC, Moayyedi P, Sherlock M, Zachos M. Microbiota signatures and mucosal healing in the use of enteral nutrition therapy versus corticosteroids for the treatment of children with Crohn’s disease: a systematic review and meta-analysis. Br J Nutr. 2023;16–19. doi:10.1017/S0007114523000405.
- Melton SL, Taylor KM, Gibson PR, Halmos EP. Review article: mechanisms underlying the effectiveness of exclusive enteral nutrition in Crohn’s disease. Aliment Pharmacol Ther. 2023 October;57(9):932–947. doi:10.1111/apt.17451.
- Gkikas K, Logan M, Nichols B, Ijaz UZ, Clark CM, Svolos V, Gervais L, Duncan H, Garrick V, Curtis L, et al. Dietary triggers of gut inflammation following exclusive enteral nutrition in children with Crohn’s disease: a pilot study. BMC Gastroenterol. 2021;21(1):1–11. doi:10.1186/s12876-021-02029-4.
- Gerasimidis K, Gkikas K, Stewart C, Neelis E, Svolos V. Microbiome and paediatric gut diseases. Arch Dis Child. 2022;107(9):784–789. doi:10.1136/archdischild-2020-320875.
- Pigneur B, Lepage P, Mondot S, Schmitz J, Goulet O, Doré J, Ruemmele FM. Mucosal healing and bacterial composition in response to enteral nutrition vs steroid-based induction therapy—A randomised prospective clinical trial in children with Crohn’s disease. J Crohn’s Colitis. 2019;13(7):846–855. doi:10.1093/ecco-jcc/jjy207.
- Soo J, Malik BA, Turner JM, Persad R, Wine E, Siminoski K, Huynh HQ. Use of exclusive enteral nutrition is just as effective as corticosteroids in newly diagnosed pediatric Crohn’s disease. Dig Dis Sci. 2013;58(12):3584–3591. doi:10.1007/s10620-013-2855-y.
- Narula N, Dhillon A, Zhang D, Sherlock ME, Tondeur M, Zachos M. Enteral nutritional therapy for induction of remission in Crohn’s disease. Cochrane Database Syst Rev. 2018;2018(4). doi:10.1002/14651858.CD000542.pub3.
- Morain CO, Segal AW, Levi AJ. Elemental diet as primary treatment of acute Crohn’s disease: a controlled trial. Br Med J. 1984;288(6434):1859–1862. doi:10.1136/bmj.288.6434.1859.
- Okada M, Yao T, Yamamoto T, Takenaka K, Imamura K, Maeda KF, Fujita K. Controlled trial comparing an elemental diet with prednisolone in the treatment of active Crohn’s disease. Hepatogastroenterol. 1990;37:72–80.
- Xiang L, Ding X, Li Q, Wu X, Dai M, Long C, He Z, Cui B, Zhang F. Efficacy of faecal microbiota transplantation in Crohn’s disease: a new target treatment? Microb Biotechnol. 2020;13(3):760–769. doi:10.1111/1751-7915.13536.
- Jarmo O, Veli-Jukka A, Eero M. Treatment of clostridioides (clostridium) difficile infection. Ann Med. 2020;52(1–2):12–20. doi:10.1080/07853890.2019.1701703.
- Li P, Zhang T, Xiao Y, Tian L, Cui B, Ji G, Liu YY, Zhang F. Timing for the second fecal microbiota transplantation to maintain the long-term benefit from the first treatment for Crohn’s disease. Appl Microbiol Biotechnol. 2019;103(1):349–360. doi:10.1007/s00253-018-9447-x.
- Vaughn BP, Vatanen T, Allegretti JR, Bai A, Xavier RJ, Korzenik J, Gevers D, Ting A, Robson SC, Moss AC. Increased intestinal microbial diversity following Fecal microbiota transplant for active Crohnʼs disease. Inflamm Bowel Dis. 2016;22(9):2182–2190. doi:10.1097/MIB.0000000000000893.
- Yang Z, Bu C, Yuan W, Shen Z, Quan Y, Wu S, Zhu C, Wang X. Fecal microbiota transplant via endoscopic delivering through small intestine and colon: no difference for Crohn’s disease. Dig Dis Sci. 2020;65(1):150–157. doi:10.1007/s10620-019-05751-y.
- Suskind DL, Brittnacher MJ, Wahbeh G, Shaffer ML, Hayden HS, Qin X, Singh N, Damman CJ, Hager KR, Nielson H, et al. Fecal microbial transplant effect on clinical outcomes and Fecal microbiome in active Crohnʼs disease. Inflamm Bowel Dis. 2015;21(3):556–563. doi:10.1097/MIB.0000000000000307.
- Wang H, Cui B, Li Q, Ding X, Li P, Zhang T, Yang X, Ji G, Zhang F. The safety of fecal microbiota transplantation for Crohn’s disease: findings from a long-term study. Adv Ther. 2018;35(11):1935–1944. doi:10.1007/s12325-018-0800-3.
- Pai N, Popov J, Hill L, Hartung E. Protocol for a double-blind, randomised, placebo-controlled pilot study for assessing the feasibility and efficacy of faecal microbiota transplant in a paediatric Crohn’s disease population: PediCRaFT trial. BMJ Open. 2019;9(11):1–11. doi:10.1136/bmjopen-2019-030120.
- Aardoom MA, Veereman G, de Ridder L. A review on the use of anti-TNF in children and adolescents with inflammatory bowel disease. Int J Mol Sci. 2019;20(10). doi:10.3390/ijms20102529.
- Balzola F, Cullen G, Ho GT, Russell RK, Wehkamp J. Ustekinumab induction and maintenance therapy in refractory Crohn’s disease: commentary. Inflamm Bowel Dis Monit. 2012;13(2):69. doi:10.1056/NEJMoa1203572.
- Balzola F, Cullen G, Ho GT, Russell R. Vedolizumab as induction and maintenance therapy for Crohn’s disease: commentary. Inflamm Bowel Dis Monit. 2014;14(2):55–56. doi:10.1056/NEJMoa1215739.
- Jongsma MME, Aardoom MA, Cozijnsen MA, Van Pieterson M, De Meij T, Groeneweg M, Norbruis OF, Wolters VM, van Wering HM, Hojsak I, et al. First-line treatment with infliximab versus conventional treatment in children with newly diagnosed moderate-to-severe Crohn’s disease: an open-label multicentre randomised controlled trial. Gut. 2022;71(1):34–42. doi:10.1136/gutjnl-2020-322339.
- Parigi TL, Iacucci M, Ghosh S. Blockade of IL-23: What is in the pipeline? J Crohn’s Colitis. 2022;16(Supplement_2):ii64–ii72. doi:10.1093/ecco-jcc/jjab185.
- Sandborn WJ, Rebuck R, Wang Y, Zou B, Adedokun OJ, Gasink C, Sands BE, Hanauer SB, Targan S, Ghosh S, et al. Five-year efficacy and safety of ustekinumab treatment in Crohn’s disease: The IM-UNITI trial. Clin Gastroenterol Hepatol. 2022;20(3):578–590.e4. doi:10.1016/j.cgh.2021.02.025.
- D’Haens G, Panaccione R, Baert F, Bossuyt P, Colombel JF, Danese S, Dubinsky M, Feagan BG, Hisamatsu T, Lim A, et al. Risankizumab as induction therapy for Crohn’s disease: results from the phase 3 ADVANCE and MOTIVATE induction trials. Lancet. 2022;399(10340):2015–2030. doi:10.1016/S0140-6736(22)00467-6.
- Ferrante M, Panaccione R, Baert F, Bossuyt P, Colombel JF, Danese S, Dubinsky M, Feagan BG, Hisamatsu T, Lim A, et al. Risankizumab as maintenance therapy for moderately to severely active Crohn’s disease: results from the multicentre, randomised, double-blind, placebo-controlled, withdrawal phase 3 FORTIFY maintenance trial. Lancet. 2022;399(10340):2031–2046. doi:10.1016/S0140-6736(22)00466-4.
- Thia KT, Mahadevan U, Feagan BG, Wong C, Cockeram A, Bitton A, Bernstein CN, Sandborn WJ. Ciprofloxacin or metronidazole for the treatment of perianal fistulas in patients with Crohnʼs disease: a randomized, double-blind, placebo-controlled pilot study. Inflamm Bowel Dis. 2009;15(1):17–24. doi:10.1002/ibd.20608.
- Breton J, Tanes C, Tu V, Albenberg L, Rowley S, Devas N, Hwang R, Kachelries K, Wu GD, Baldassano RN, et al. A microbial signature for paediatric perianal Crohn’s disease. J Crohns Colitis. 2022;16(8):1281–1292. doi:10.1093/ecco-jcc/jjac032.
- Tabibian JH, Weeding E, Jorgensen RA, Petz JL, Keach JC, Talwalkar JA, Lindor KD. Randomised clinical trial: vancomycin or metronidazole in patients with primary sclerosing cholangitis - a pilot study. Aliment Pharmacol Ther. 2013;37(6):604–612. doi:10.1111/apt.12232.
- Nitzan O, Elias M, Peretz A, Saliba W. Role of antibiotics for treatment of inflammatory bowel disease. World J Gastroenterol. 2016;22(3):1078–1087. doi:10.3748/wjg.v22.i3.1078.
- Wise PE, Schwartz DA. Management of perianal Crohn’s disease. Clin Gastroenterol Hepatol. 2006;4(4):426–430. doi:10.1016/j.cgh.2006.02.001.
- Elliott PR, Moore GTC, Bell SJ, Connell WR. Severe recurrent Crohn’s disease of the ileocolonic anastomosis disappearing completely with antibacterial therapy [2]. Gut. 2005;54(12):1818–1819. doi:10.1136/gut.2005.078568.
- Levine A, Turner D. Combined azithromycin and metronidazole therapy is effective in inducing remission in pediatric Crohn’s disease. J Crohn’s Colitis. 2011;5(3):222–226. doi:10.1016/j.crohns.2011.01.006.
- Strong SA. Perianal Crohn’s disease. Semin Pediatr Surg. 2007;16(3):185–193. doi:10.1053/j.sempedsurg.2007.04.007.
- Park SK, Kim KJ, Lee SO, Yang DH, Jung KW, Duk Ye B, Byeon JS, Myung SJ, Yang SK, Kim JH, et al. Ciprofloxacin usage and bacterial resistance patterns in Crohn’s disease patients with abscesses. J Clin Gastroenterol. 2014;48(8):703–707. doi:10.1097/MCG.0000000000000024.
- Rahimpour S, Nasiri-Toosi M, Khalili H, Daryani NE, Taromlou MKN, Azizi Z. A triple blinded, randomized, placebo-controlled clinical trial to evaluate the efficacy and safety of oral vancomycin in primary sclerosing cholangitis: a pilot study. J Gastrointest Liver Dis. 2016;25(4):457–464. doi:10.15403/jgld.2014.1121.254.rah.
- Chandok N, Hirschfield GM. Management of primary sclerosing cholangitis: conventions and controversies. Can J Gastroenterol. 2012;26(5):261–268. doi:10.1155/2012/426430.
- Kucharska M, Daniluk U, Kwiatek-Średzińska KA, Wasilewska N, Filimoniuk A, Jakimiec P, Zdanowicz K, Lebensztejn D. Hepatobiliary manifestations of inflammatory bowel disease in children. Clin Exp Hepatol. 2019;5(3):203–209. doi:10.5114/ceh.2019.87632.
- Ni Mhuircheartaigh JM, Lee KS, Curry MP, Pedrosa I, Mortele KJ. Early peribiliary hyperenhancement on MRI in patients with primary sclerosing cholangitis: significance and Association with the mayo risk score. Abdom Radiol. 2017;42(1):152–158. doi:10.1007/s00261-016-0847-z.
- Khoshpouri P, Ameli S, Ghasabeh MA, Pandey A, Zarghampour M, Varzaneh FN, Jacob A, Pandey P, Luo Y, Kamel IR, et al. Correlation between quantitative liver and spleen volumes and disease severity in primary sclerosing cholangitis as determined by mayo risk score. Eur J Radiol. 2018;108:254–260. doi:10.1016/J.EJRAD.2018.10.006.
- Turner D, Bishai J, Reshef L, Abitbol G, Focht G, Marcus D, Ledder O, Lev-Tzion R, Orlanski-Meyer E, Yerushalmi B, et al. Antibiotic cocktail for pediatric acute severe colitis and the microbiome: the PRASCO randomized controlled trial. Inflamm Bowel Dis. 2020;26(11):1733–1742. doi:10.1093/ibd/izz298.
- Guslandi M. Rifaximin in the treatment of inflammatory bowel disease. World J Gastroenterol. 2011;17(42):4643–4646. doi:10.3748/wjg.v17.i42.4643.
- Muniyappa P, Gulati R, Mohr F, Hupertz V. Use and safety of rifaximin in children with inflammatory bowel disease. J Pediatr Gastroenterol Nutr. 2009;49(4):400–404. doi:10.1097/MPG.0b013e3181a0d269.
- Lopetuso LR, Napoli M, Rizzatti G, Gasbarrini A. The intriguing role of rifaximin in gut barrier chronic inflammation and in the treatment of Crohn’s disease. Expert Opin Investig Drugs. 2018;27(6):543–551. doi:10.1080/13543784.2018.1483333.
- Maccaferri S, Vitali B, Klinder A, Kolida S, Ndagijimana M, Laghi L, Calanni F, Brigidi P, Gibson GR, Costabile A, et al. Rifaximin modulates the colonic microbiota of patients with Crohn’s disease: an in vitro approach using a continuous culture colonic model system. J Antimicrob Chemother. 2010;65(12):2556–2565. doi:10.1093/jac/dkq345.
- Khan KJ, Ullman TA, Ford AC, Abreu MT, Abadir A, Marshall JK, Talley NJ, Moayyedi P. Antibiotic therapy in inflammatory bowel disease: a systematic review and meta-analysis. Am J Gastroenterol. 2011;106(4):661–673. doi:10.1038/ajg.2011.72.
- Mishima Y, Sartor RB. Manipulating resident microbiota to enhance regulatory immune function to treat inflammatory bowel diseases. J Gastroenterol. 2020;55(1):4–14. doi:10.1007/s00535-019-01618-1.
- Eindor-Abarbanel A, Healey GR, Jacobson K. Therapeutic advances in gut microbiome modulation in patients with inflammatory bowel disease from pediatrics to adulthood. Int J Mol Sci. 2021;22(22). doi:10.3390/ijms222212506.
- Assar S, Nosratabadi R, Khorramdel Azad H, Masoumi J, Mohamadi M, Hassanshahi G. A review of immunomodulatory effects of fluoroquinolones. Immunol Invest. 2021;50(8):1007–1026. doi:10.1080/08820139.2020.1797778.
- Sprockett D, Fischer N, Boneh RS, Turner D, Kierkus J, Sladek M, Escher JC, Wine E, Yerushalmi B, Dias JA, et al. Treatment-specific composition of the gut microbiota is associated with disease remission in a pediatric crohn’s disease cohort. Inflamm Bowel Dis. 2019;25(12):1927–1938. doi:10.1093/ibd/izz130.