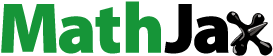
ABSTRACT
Microbiota-derived short-chain fatty acids, including butyrate (BA), have multiple beneficial health effects. In the colon, BA concentrations range from 10 to 20 mM and up to 95% is utilized as energy by the mucosa. BA plays a key role in epithelial-barrier regulation and anti-inflammation, and regulates cell growth and differentiation, at least in part, due to its direct influence on stabilization of the transcription factor hypoxia-inducible factor (HIF). It remains unclear whether BA is the optimal metabolite for such a response. In this study, we explored metabolite mimicry as an attractive strategy for the biological response to HIF. We discovered that 4-mercapto butyrate (MBA) stabilizes HIF more potently and has a longer biological half-life than BA in intestinal epithelial cells (IECs). We validated the MBA-mediated HIF transcriptional activity through the induction of classic HIF gene targets in IECs and enhanced epithelial barrier formation in vitro. In-vivo studies with MBA revealed systemic HIF stabilization in mice, which was more potent than its parent BA metabolite. Mechanistically, we found that MBA enhances oxygen consumption and that the sulfhydryl group is essential for HIF stabilization, but exclusively as a four-carbon SCFA. These findings reveal a combined biochemical mechanism for HIF stabilization and provide a foundation for the discovery of potent metabolite-like scaffolds.
Introduction
Short-chain fatty acids (SCFAs) are the primary products of complex carbohydrate fermentation in the gut microbiota, primarily in the colon. They represent the major source of carbon from diet, through the microbiome to the host.Citation1 The three major SCFAs produced by gut bacteria are acetate, propionate, and butyrate,Citation2 which are aliphatic and saturated carboxylic acids of various carbon lengths. Based on the availability of dietary substrates, SCFAs can reach tolerable high concentrations of up to 150 mM in the colon at relative molar ratios of acetate-propionate-butyrate of approximately 60-20-20, respectively.Citation3–5 Apart from these major SCFAs, the gut microbiota ferments branched amino acids into isobutyrate, isovalerate, and 2-methyl butyrate, known as branched short chain fatty acids (BCFAs).
Among all SCFAs, butyrate (BA) has received the most attention due to its beneficial effects on cellular energy metabolism and intestinal homeostasis.Citation6–8 BA regulates biological responses to maintain gastrointestinal health by acting as a powerful histone deacetylase (HDAC) inhibitorCitation9,Citation10 and through binding to several specific G protein-coupled receptors (GPCRs).Citation11 As the major source of energy in the colon, up to 95% of BA is absorbed by colonocytes and quickly transformed into fuel via β-oxidation and the tricarboxylic acid cycle.Citation12 Furthermore, various studies have shown that BA modulates immune and inflammatory responses and regulates the intestinal barrier.Citation13,Citation14 Changes in the microbial composition of intestinal bowel disease (IBD) patients lead to decreases in BA and BA-producing bacteria, considered hallmarks of intestinal dysbiosis.Citation1,Citation2
The beneficial influences of BA also include the stabilization of hypoxia-inducible factors (HIF).Citation15,Citation16 HIF is a master transcriptional regulator of multiple genes involved in erythropoiesis, angiogenesis, energy metabolism, antimicrobial, and anti-inflammatory processes.Citation17–19 Three HIF-α isoforms are known (HIF1α, HIF2α, HIF3α), where HIF1α and HIF2α are the most studied, exhibiting similar structures and functions.Citation17 HIF-α subunits are intimately regulated by oxygen availability through a family of iron-dependent enzymes, prolyl hydroxylases (PHDs), where PHD2 is the most studied. PHDs use oxygen and 2-oxoglutarate (2-OG) as co-substrates, and Fe2+ and ascorbate as cofactors.Citation20 Under well oxygenated conditions, PHDs hydroxylate proline residues within the oxygen-dependent domain (ODD) of HIF-α substrates, a carefully executed biochemical reaction where molecular oxygen is split with one oxygen atom inserted into the prolyl residue and the other bonded to 2-OG, producing succinate and CO2. This reaction is strongly catalyzed by Fe2+ which is oxidized to Fe3+ and quickly reverts to its active form by ascorbate. Hydroxylated HIF-α substrates bind to the von Hippel-Lindau tumor suppressor (pVHL), the recognition element of the E3 ubiquitin ligase that polyubiquitinates HIF-α for proteasomal degradation.Citation20–22 When oxygen is limited or PHDs are chemically inhibited, HIF-α substrates are stabilized and form a heterodimeric complex with HIF-1β in the nucleus to bind hypoxia responsive elements (HRE) in the promoter region of hundreds of target genes designed for cell survival.Citation23 Interestingly, β-oxidation of BA to meet energy demands accounts for > 70% of cellular oxygen consumption in the distal colonCitation24 and this shift in oxygen availability can stabilize HIF.Citation25 Further studies in our laboratory demonstrated that BA is also an endogenous and noncompetitive PHD inhibitor that stabilizes HIF through an oxygen-independent mechanism or possibly through a synergistic effect.Citation16
A potential clinical limitation of butyrate is that it is rapidly metabolized as an energy source, thus diminishing its potency in other functions, such as stabilizing HIF. Microbial metabolite mimicry has been reported as an attractive strategy to improve drug discovery, resulting in relatively nontoxic drugs.Citation26,Citation27 Metabolite-like scaffolds are already seen in many successful drugs.Citation28 Metabolite scaffold mimicry could potentially result in more potent and specific biological responses. With this in mind, we explored endogenous and non-endogenous butyrate derivatives with specific functional groups at different positions within the small molecule and assessed their effects on HIF stabilization. Here, we present a non-endogenous butyrate-mimicking derivative that can more potently stabilize HIF in-vitro and in-vivo. These findings contribute to the search for more tolerable HIF stabilizers as potential therapeutic agents.
Results
HIF is stabilized despite structural modifications on butyrate
As a foundation for our studies, a small library of butyrate derivatives was explored () using an electrochemiluminescence-based assay to quantify HIF1α protein. Human intestinal epithelial cells (IECs) Caco-2 and T84 were exposed to a physiologically relevant concentration (5 mM) of each butyrate derivative for 6 h. A bona fide PHD2 inhibitor (IOX4) was used to stabilize HIF1α as a positive control. Various butyrate derivatives increased HIF1α relative concentration in both cell lines compared to the low signals detected in untreated lysates. Surprisingly, however, one of the butyrate derivatives exceeded HIF1α stabilization in Caco-2 cells compared to the potent IOX4 PHD2 inhibitor (albeit at higher concentrations) identified as 4-mercapto butyrate (MBA) (Supplemental ). This result was confirmed by immunoblotting for HIF1α (), which showed that MBA stabilized HIF1α at least as much as IOX4 (10 μM) in Caco-2 cells and exceeded the capabilities of butyrate at an equimolar concentration in both cell lines. In T84 cells specifically (), MBA was the only butyrate derivative that significantly increased HIF1α accumulation, we thus decided to focus on this butyrate-mimicking derivative for further studies. A dose response study between MBA and BA revealed that MBA can stabilize HIF more potently at all doses tested and up to the sub-millimolar range, demonstrating an important characteristic that could be clinically relevant (). We assessed potential toxicity in T84 cells exposed to doses of BA and MBA as high as 10 mM by a lactate dehydrogenase (LDH) assay (see supplemental ). LDH is a cytosolic enzyme present in many cell types and release of LDH in media is well correlated with membrane disruption and cell death.Citation29 We observed a low cytotoxicity of ~ 9% in BA and ~ 11% in MBA incubated cells and there was no significant difference in toxicity between MBA and its parent natural metabolite in this specific cell line. Gathering these results and for a fair comparison, 5 mM concentrations of MBA and BA were used in further studies unless otherwise noted.
Figure 1. MBA stabilizes HIF.
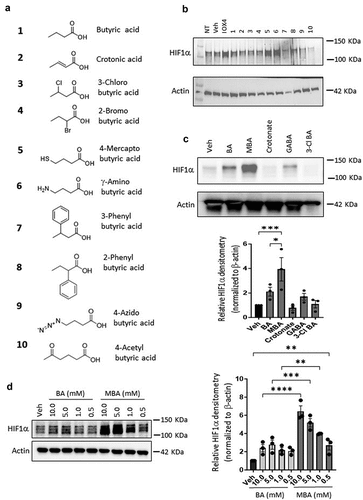
Figure 2. Time course studies of MBA-HIF stabilization.
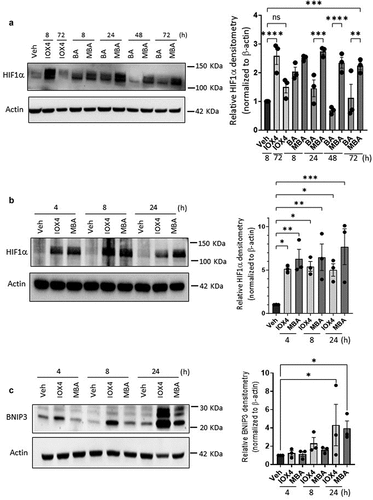
4-mercapto butyrate extends HIF1α protein half-life in vitro compared to native butyrate
HIF1α is highly unstable under normoxic conditions, with a half-life of less than 5 min.Citation30 To elucidate the effectiveness of MBA to stabilize HIF1α over time and possibly shine light on playing a potential role in metabolism similar to its parent-metabolite BA, time-course studies were performed between BA and MBA, IOX4 was used as a positive control. As observed in , HIF1α was no longer significantly stabilized after 24 h when T84 cells were exposed to BA, likely due to its rapid metabolism.Citation9 By contrast, the influence of MBA on HIF stabilization was observed as late as 72 h. IOX4 function was lost within 48 h of exposure. As a proof of concept and employing a HIF1α Mesoscale assay for protein concentration, we screened Caco-2 cells exposed to MBA (5 mM) for various time points. We observed that HIF1α was rapidly stabilized (3 h), and its relative concentration steadily increased over time for up to 72 h, supporting its prolonged stabilization mechanism (Supplemental ). This study was followed by immunoblotting Caco-2 cells exposed to IOX4 and MBA, supporting HIF1α accumulation at 4 h and up to at least 24 h (). BNIP3 is involved in cell survival and is an established HIF1α target that is induced through the chemical stabilization of HIF or hypoxia. BNIP3 promoter comprises a functional hypoxia response element (HRE) and can be activated by HIF1α.Citation31 Parallel to HIF stabilization time course studies, we assessed the transcriptional activity of the stabilized protein over time. BNIP3 Immunoblotting of Caco-2 cell lysates exposed to IOX4 and MBA over various time points supported active HIF1α transcription leading to translation of BNIP3, more specifically at longer time points as expected ().
Figure 3. MBA transactivates HIF target genes.
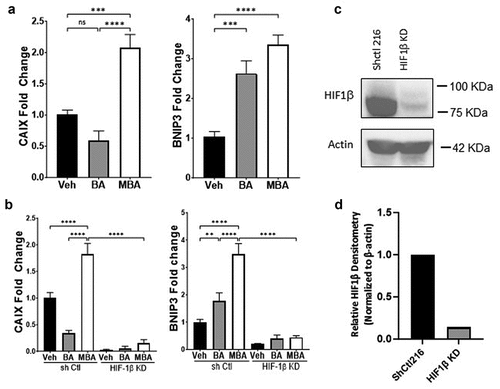
HIF dependent transcription of target genes after exposure to 4-mercapto butyrate
We further explored whether MBA-induced stabilization of HIF was reflected in the transcriptional regulation of target genes. We utilized real-time PCR to study the influence of MBA mediated HIF stabilization in the induction of two established HIF target genes, hbnip3 (autophagy regulator)Citation32 and hcaix (pH regulation and maintenance).Citation33 BA was used both for comparison and as a positive control in these experiments since it has been previously demonstrated to significantly induce various HIF targets.Citation25 As shown in , IECs exposed to 5 mM MBA or BA for 0 and 16 h resulted in significant induction of hbnip3 and hcaix, more specifically when exposed to MBA.
Furthermore, to confirm transcriptional activity through the HIF pathway, we quantified the induction of hbnip3 and hcaix in lentiviral shRNA-mediated knockdown of HIF1β relative to non-targeting shRNA controls exposed to BA or MBA (5 mM, 0 and 16 h). As shown in , there was a complete loss of induction of both target genes in cells lacking HIF1β when exposed to BA or MBA. In contrast, the shRNA control exhibited a profile almost identical to that of the wild-type treatments. show HIF1β depletion by approximately 84% in T84 KD cells compared with the control. These findings confirmed that MBA stabilizes HIF, leading to its transcriptional activity. Furthermore, MBA allows a more prominent induction of these two HIF target genes than BA.
Potential dual functionality of 4-mercapto butyrate in HIF stabilization mechanism
To investigate the potential biochemical mechanism of MBA-induced HIF stabilization, we first explored intracellular oxygen consumption. Sulfur is a common oxidation site in thiol derivatives. Many oxidizing agents oxidize thiols to disulfides, including molecular oxygens. Thiol compounds also react with oxygen in coordination with variable-valence metals as a basis for the action of many metalloproteins (e.g., iron-dependent proteins).Citation34,Citation35 Using OxoDish assaysCitation15,Citation16 we monitored oxygen consumption in clones of Caco-2 cells (c2bbe), a colorectal adenocarcinoma cell line previously described by our laboratory,Citation36,Citation37 exposed to buffer, BA, or MBA. Indeed, we observed that in a period of 2 h, MBA significantly accelerated oxygen consumption in the media by approximately 3.5% compared to our controls (). We then explored oxygen consumption in c2bbe cells pretreated with methylene cyclopropyl acetic acid (MCPA). As previously described by our laboratory, MCPA inhibits natural β-oxidation of butyrate, allowing the distinction of fatty acid oxidation and other actions of butyrate.Citation16 C2bbe cells were pretreated with 1 mM MCPA (2 h) followed by exposure to MBA or BA, and %O2 was measured over a period of 120 min. As shown in , BA and MBA significantly increased O2 consumption compared to the vehicle-treated cells. Notably, MCPA abolished the increase in O2 consumption by BA, but did not have a significant influence on MBA-mediated increases in O2 consumption. Furthermore, we investigated the relationship between oxygen consumption and HIF stabilization when the IECs were exposed to BA or MBA. As depicted in , BA-induced HIF stabilization was reduced in the presence of MCPA, whereas MBA-induced HIF stabilization appeared to be independent of oxygen consumption. These results suggest that BA and MBA display differential dependence on O2 consumption, as they are related to HIF stabilization.
Figure 4. MBA stabilizes HIF independent of oxygen consumption through a sulfhydryl involved mechanism.
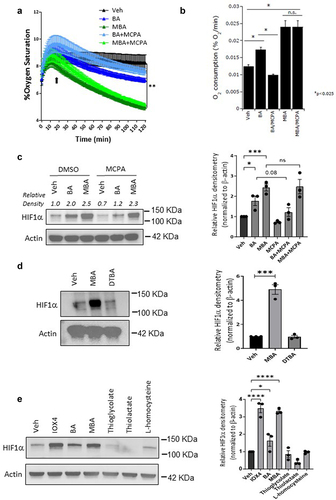
Next, we investigated the role of the sulfhydryl functional group in HIF stabilization. It has been shown that small molecules exhibiting sulfhydryl functional groups in their reduced form can chelate various metals including Cu, Zn, Pb, Hg, and Fe.Citation38,Citation39 We exposed IECs to equimolar concentrations of MBA (−SH) or its oxidized form 4,4-dithiobutyrate (DTBA S-S) for 0 and 6 h. As observed in , DTBA was not capable of stabilizing HIF, thus supporting the vital role of the sulfhydryl functional group in this mechanism. To determine whether HIF stabilization was achieved through a thiol-induced mechanism, T84 cells were treated with equal concentrations of several thiol-containing small molecules similar to BA (). Surprisingly, none of these thiol derivatives (except for MBA) stabilized HIF, demonstrating an important role for both the fatty acid structure and thiol in enhanced HIF stabilization.
MBA enhances epithelial barrier function
Studies have indicated that HIF orchestrates the regulation of various genes responsible for epithelial barrier function and barrier-adaptive responses.Citation40 Sodium butyrate is an established pro-barrier factor, in part due to HIF stabilization.Citation9,Citation25,Citation41 Therefore, we studied the influences of various butyrate derivatives in epithelial cell barrier formation. Epithelial barrier integrity was determined by measuring transepithelial electrical resistance (TEER), a typical assay for the quantitation of epithelial barrier strength.Citation42 c2bbe cells were exposed to butyrate-mimicking derivatives (5 mM) and TEERS were measured daily. Various derivatives demonstrated a considerable influence on barrier formation compared with the vehicle (Supplemental ). Interestingly, both MBA and BA produced a significant increase in the barrier by approximately two-fold compared to the vehicle controls. It is notable that, possibly through consistent extended HIF stabilization, MBA elicited a sustained barrier maintenance influence over the course of the experiment (). These results indicate that, in addition to the molecular regulation of HIF, MBA elicits an enhanced functional response in IEC.
Figure 5. Epithelial barrier formation response to MBA and BA.
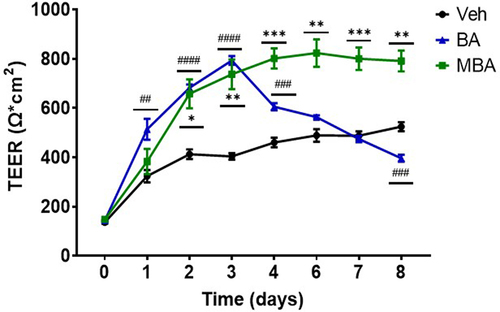
In vivo actions of MBA
A prototypical physiological response to hypoxia is increased red blood cell production. HIF is responsible for this response by regulating cell-type specific gene expression that leads to increased levels of the hormone erythropoietin (EPO).Citation43 Thus, we explored the in vivo effects of MBA in mediating the induction of genes fundamentally regulated by HIF. C57BL/6 mice received an intraperitoneal injection of equimolar amounts of BA (73 mg/kg), MBA (80 mg/kg), or the established in vivo PHD inhibitor, DMOG (117 mg/kg).Citation44,Citation45 PBS (200 µL) was used as control. After 24 h of treatment, the mice were sacrificed and rapidly exsanguinated to measure the EPO levels in the serum. As shown in , MBA-treated mice exhibited significantly higher circulating levels of EPO than vehicle, DMOG, or BA. Furthermore, colon tissue was collected to probe for HIF1α protein accumulation; as observed in supplemental all treated murine samples exhibited a degree of HIF stabilization compared to PBS treated mice. Of note, both DMOG and MBA exhibited significant stabilization of HIF1α. Furthermore, we studied the induction of mGLUT1, which is another classic HIF target responsible for the transport of various monosaccharides. Kidney and colon tissues were analyzed using real-time PCR to compare BA and MBA head-to-head. As observed in , both treatments resulted in the induction of mGLUT1 in the kidney and a significant increase in colon tissue. These findings support the ability of MBA to stabilize HIF in vivo leading to the induction of mGLUT1 and transcription of the tightly regulated EPO protein.
Figure 6. MBA induces HIF regulated targets in vivo.
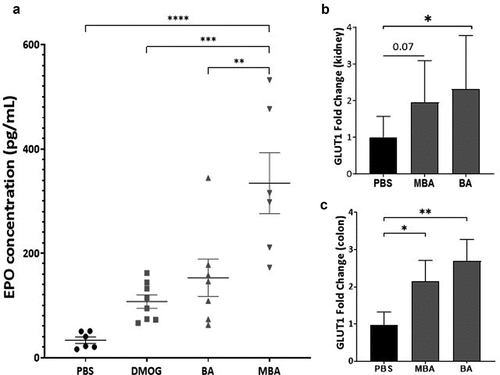
Discussion
Multiple lines of evidence implicate microbially derived butyrate in promoting intestinal homeostasis, and deficiencies in butyrate production/utilization are associated with disease states, most notably IBD.Citation46–48 In recent studies, the protective roles of butyrate are attributable to the stabilization of HIF.Citation8,Citation15,Citation16 HIF orchestrates gut homeostasis through the regulation of a number of genes, including tight junction proteins (e.g., CLDN1) and mucin-2 (MUC2) as the major component of the mucus layer, as well as antimicrobial peptides.Citation19,Citation49 Previous studies have established the HIF pathway in the development and maintenance of the epithelial barrier, the loss of which is a hallmark of IBD.Citation50,Citation51 Evolving literature has demonstrated that HIF is overall protective in the mucosa and most specifically in disease states.Citation52–54 The primary role of butyrate is to provide energy to colonocytes, where it is rapidly oxidized to CO2 via mitochondrial oxidative phosphorylation as a means for ATP production. In homeostasis, this mechanism allows for the procurement of 70–80% of the required energy in colonocytes.Citation55,Citation56 Interestingly, butyrate-mediated temporal stabilization of HIF may play a major role in priming the colon tissue toward butyrate metabolism by shifting acetyl-CoA production via β-oxidation of butyrate.Citation16,Citation57,Citation58 Thus, the rapid metabolism and various mechanisms of action of butyrate in conjunction with decreased levels in disease states may prove to limit its clinical applications. To overcome this limitation, biomimicry provides potent and safe drug-like small molecules based on their structural similarity to the parent metabolite.Citation26,Citation27,Citation59 Here, we report the discovery of butyrate-mimicking MBA as a potent HIF stabilizer. We demonstrated enhanced HIF stabilization over extended periods relative to the parent metabolite. We further report MBA-mediated transactivation of HIF target genes, specifically through the HIF pathway. We propose a combined mechanism of HIF stabilization involving oxygen shifts, PHD inhibition, and iron chelation. We identified MBA as a significant factor in the establishment and maintenance in vitro. Lastly, we report the ability of MBA to transactivate HIF genes in vivo with enhanced potency over both butyrate and a classic HIF stabilizing agent, as demonstrated specifically by the significant levels of kidney-derived EPO in the circulation.
As a continuation of previous findings, we pursued the discovery of butyrate-mimicking derivatives that might stabilize HIF more selectively. Previously reported nuclear magnetic resonance studies have established the specificity of butyrate for binding and inhibiting PHDs. Furthermore, it was reported that butyrate exhibited a physiologically relevant true half maximal inhibitory concentration (IC50) of 5 mM and that by functionalizing carbon 2 and 4, PHD2 inhibition was greatly reduced to an IC50 of ~220 mM.Citation16 In agreement with these findings, a small library of butyrate derivatives was designed with electron-withdrawing groups of various atomic sizes in all positions of the small molecule (e.g., 2-bromo, 3-chloro, 4-amino butyrate, 2-phenyl butyrate) in the hope of creating a carboxylate stabilizing effect. Among other compounds, we tested isomers of butyrate, unsaturated butyrate-mimicking compounds (crotonic acid), and a butyrate-mimicking derivative exhibiting an azide at the 4 position, a classic functional group in click chemistry (see ). In screening this library by monitoring HIF1α protein levels in IECs, we discovered MBA to be a superior butyrate derivative in HIF1α stabilization among all compounds in the two intestinal epithelial cell lines. Furthermore, through dose response studies, it was discovered that, in contrast to BA, MBA can stabilize HIF in the sub-millimolar region, increasing its physiological relevance. It was also observed that MBA can mediate a prolonged HIF stabilization influence in different IECs, possibly because butyrate is rapidly used as an energy source. The MBA-mediated transcriptional activity of HIF was analyzed using qPCR. Well-established HIF target genes, carbonic anhydrase IX (caix) involved in differentiation, proliferation, and pH regulationCitation60 as well as an autophagy regulator bnip3 were analyzed.Citation32,Citation36 These targets were induced significantly higher by MBA compared to the parent metabolite in IECs in-vitro. A complete loss of induction was observed in HIF1β knockdown cells when exposed to the same conditions and compared to the corresponding shRNA controls, establishing an MBA-mediated HIF stabilization pathway.
The present observations led us to propose a possible biochemical mechanism of HIF stabilization by MBA. We propose that MBA influences PHD activity in several ways (). First, the enhanced oxygen consumption of IECs in the presence of thiol derivatives significantly diminished the availability of oxygen as a substrate for PHD enzymes. When IECs were pretreated with MCPA (an ββ-oxidation inhibitor) followed by exposure to butyrate, we observed a decrease in oxygen consumption and reduced HIF stabilization. In contrast, MCPA did not significantly influence O2 consumption or HIF accumulation in MBA-treated cells. These findings led us to conclude that MBA is not metabolized through fatty acid β oxidation, and that it consumes oxygen through natural auto-oxidation, probably in the presence of iron. Furthermore, we exposed IECs to the oxidized version of MBA (DTBA S-S) and compared it with freshly reduced MBA (incubated in the solid supported reducing agent tris[2-carboxyethyl]phosphine TCEP) to observe a complete loss of HIF stabilization in the oxidized version (DTBA). This result indicated the importance of the free thiol present in the molecule for a rapid mechanism of action. Surprisingly, shorter thiol-SCFAs (thioglycolate and thiolactate) or L-homocysteine (a non-proteogenic homologue of cysteine) did not stabilize HIF. This result suggested that although oxygen consumption might prime cells for HIF stabilization, there is an intimate interaction between MBA and PHDs that ultimately leads to HIF accumulation. We believe MBA is not only consuming O2, a substrate required in the hydroxylation of HIF, but it is binding to PHD similar to butyrate.Citation16 MBA is “in essence” butyrate with the replacement of a hydrogen atom and the insertion of SH at C4, thus it is very possible its PHD binding affinities are retained. Furthermore, based on the metal scavenging ability of thiols, we propose that MBA chelates require cofactor iron possibly in a bidentate manner since both the carboxylate and thiol groups have the ability to strongly coordinate with the metal.Citation38,Citation39 Lastly, we speculate that when oxidized, MBA can regenerate to its active form, possibly catalyzed by NADPH-dependent reductases.Citation61 Thus, clearly making MBA a superior derivative when compared to its parent metabolite butyrate by utilizing the available tools, the thiol functional group confers (see ).
Figure 7. Proposed biochemical mechanism of MBA mediated HIF stabilization.
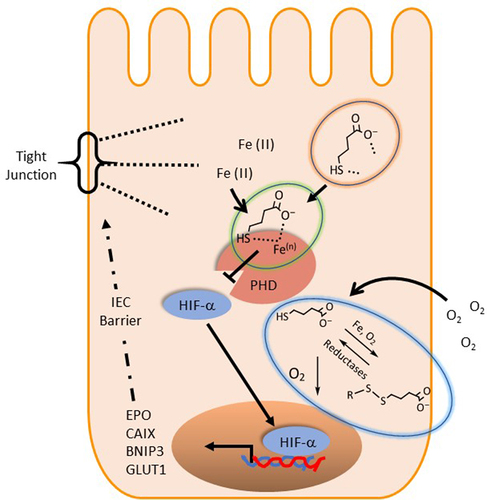
IECs provide a selective barrier and their constant turnover requires the ready availability of energy substrates.Citation62 In addition to energy provision, butyrate coordinates the control of barrier function through selective regulation of actin-associated genes (e.g. synaptopodin), via HDAC inhibition.Citation9 Also, butyrate enhances barrier through HIF stabilization as previously demonstrated by loss of barrier function in HIF1β knockdown IECs.Citation25 Epithelial HIF stabilization contributes at many levels to enhanced barrier function in vitro and in vivo.Citation63 Some examples include controlled cell renewal, maintenance of intercellular junctions, and secretion of barrier proteins.Citation64 We validated a biological response of MBA in the formation and maintenance of epithelial barrier in vitro. In our study, MBA-exposed IECs exhibited a slower response in barrier formation compared to BA; this could be attributed to a rapid response to BA due to energy supply (~24 h) compared to a slower HIF pathway response involving the transcription and translation of regulatory proteins when treated with MBA (~48 h). Nevertheless, MBA induces barrier formation as strongly as its parent metabolite and exhibits a sustained influence far beyond that of BA, possibly through longer HIF stabilization. Finally, we investigated MBA-mediated HIF stabilization in vivo through HIF1α protein accumulation and the induction of classic targets at the protein and gene levels. In our studies, HIF1α protein accumulation was significantly observed in colon tissue in the DMOG and MBA treated groups. Furthermore, quantification of serum EPO protein concentration in C57BL/6 mice revealed that a single dose of MBA significantly induced the accumulation of this hormone in circulation compared to all treatments. mGLUT1 expression was also increased in kidney and colon tissues. These results support a systemic and enhanced HIF stabilization in vivo mediated through a butyrate-mimicking compound and lay the foundation for future potential applications in mouse models of intestinal disease.
An important consideration is whether butyrate mimetics might have place in the clinic. It is notable that the inflammatory tissue microenvironment in disorders such as IBD shifts toward a less tolerant state for strict anaerobes (e.g., butyrate producers such as Bacteroidetes and Firmicutes) toward a microbiota that is less diverse and supportive of facultative anaerobes (e.g., more virulent Proteobacteria).Citation65 Insight into the role of dysbiosis has been gained through longitudinal studies, where dysbiosis improves with treatment but persists even in patients that have achieved full mucosal healing.Citation66 Given the complexity of IBD symptoms and the plethora of butyrate functions in the mucosa,Citation46–48 such observations provide opportunities for supplementation with butyrate mimetics that may be tailored to specific patients under specific disease conditions.
In summary, the mammalian intestine has evolved a complex mechanism by which microbial-derived butyrate inhibits PHDs to stabilize HIF, thereby contributing to intestinal homeostasis. However, this metabolite must be carefully distributed to play various roles, primarily to maintain energy demands. Microbial metabolite mimicry is an attractive alternative to provide more specific biological responses in the intestine. These results support the discovery of a simple small molecule that closely resembles a natural metabolite with more potent influences and a possible novel mechanism of action, laying the foundation for the discovery of more tolerable HIF stabilizers and adding to the growing field of metabolite mimicry research.
Experimental procedures
Cell culture and treatments- T84 cells (ATCC #CCL-248) were cultured in DMEM/F-12 1:1 (Thermo Fisher Scientific) containing Pen/Strep, GlutaMAX, and 10% (v/v) heat-inactivated bovine calf serum (BCS, Hyclone). Caco-2 cells (ATCC #HTB-37) were grown in IMDM (Corning) supplemented with Pen/Strep, GlutaMAX, and 10% BCS. Clones of Caco-2 cells (c2bbe, ATCC #CRL2102)Citation67 were grown in IMDM (Corning) containing Pen/Strep and Glutamax + 10% BCS. All the cell lines were maintained at 37°C and 5% CO2 in a humidified incubator. Cells were passaged approximately every 7 days with trypsin (Thermo Fisher Scientific). Cells were plated on 6-well or 24-well plates, as required. Sodium butyrate, 4-mercapto butyric acid, crotonic acid, 3-chloro butyric acid, 2-bromo butyric acid, gamma-amino butyric acid, 3-phenyl butyric acid, 4-acetyl butyric acid, thioglycolic acid, thiolactic acid, and l-homocysteine were acquired from Sigma Aldrich; 2-phenyl butyric acid (Millipore Sigma); and 4-azido butyric acid (Aurum Pharmaceuticals). The reduction of 4-mercapto butyric acid was ensured by incubating immobilized tris(2-carboxyethyl)phosphine (TCEP) disulfide reducing gel (Thermo Fisher Scientific) for 1 h at room temperature, followed by centrifugation and recovery of reduced MBA for immediate use. Unless otherwise noted cells were exposed to a final concentration of 5 mM BA or MBA by adding appropriate volumes of 50 mM solutions prepared in Hanks’ balanced salt solution + HEPES, pH 7.4 (HBSS+ sterile filtered at 0.22 μm Millipore Sigma) directly in media and HBSS+ used as no treatment control. All MCPA experiments included a 2 h pretreatment with MCPA, as previously described.Citation16
Generation of HIF1β KD cell line- To generate HIF-1β KD cell lines, lentiviral particles encoding shRNA directed against HIF1β or a non-targeting control (MISSION™ TRC shRNA, Sigma) were used to transduce T84 cells using standard protocols as described previously.Citation37 Stable integration was achieved by puromycin selection (6 μg/ml). Knockdown was confirmed by western blot analysis, which indicated a KD of approximately 86%.
Protein analysis and Immunoblotting- In experiments, HIF1α protein levels were quantified using the HIF1α MSD whole cell lysate kit and lysis buffer, and the protocol was followed without modifications (Mesoscale Discovery). Total protein was quantified for normalization using the Pierce BCA Protein Assay Kit (Thermo Fisher Scientific). The erythropoietin (EPO) protein concentration was quantified using the U-Plex mouse EPO assay and compared to the calibration curve (Mesoscale Discovery). For western blotting, cells were immediately placed on ice and cell lysates were prepared by scraping in ice-cold 1X Laemmli buffer (Bio-Rad) containing 1X HALT protease inhibitor (thermo scientific), 5 mM EDTA, and 100 mM DTT, each added immediately prior to use (450 μL per well in a 6 well-plate). The obtained lysate solution was sonicated until its viscosity was similar to that of water. For HIF1α analysis, the samples were not boiled. SDS-PAGE samples were run on Mini-PROTEAN TGX precast gels 10% (Bio-Rad) and transferred to 0.2 μm PVDF membranes using a Bio-Rad Transblot Turbo system. Blots were blocked for 1 h at room temperature using blocking buffer 5% milk (Bio-Rad) in Tris-buffered saline (TBST) (25 mM Tris-HCl, 150 mM NaCl, 0.1% Tween-20) and then incubated overnight in primary antibody diluted in 1% milk in TBST. The primary antibodies used were anti-β-actin (Abcam #ab8227, 1/5000), anti-HIF1α (BD Biosciences #610959), anti-HIF1 β (BD Biosciences #611079), and anti-BNIP3 (Abcam #ab109362, 1/1000). Blots were then washed with TBST and incubated with corresponding HRP-conjugated secondary antibody diluted in 1% milk (MP Biomedical #0855676, 0855550; 1/10000). Blots were washed thoroughly with TBST, developed using Clarity MAX ECL reagent (Bio-Rad), and imaged using Bio-Rad ChemiDoc MP.
RNA isolation, cDNA synthesis, and qPCR- RNA was isolated from cells or homogenized tissue samples using TRIzol reagent (Thermo Scientific) according to the manufacturer’s instructions. Isolated RNA was then reverse transcribed to cDNA using the iScript Supermix reagent (Bio-Rad), and analyzed using Power SYBR Green Master Mix reagent (Thermo Scientific) in an ABI 7300 or Quant Studio 5 Applied Biosystems Real-Time PCR (Thermo Fisher Scientific). Transcript quantities were calculated using an on-plate standard curve and were normalized to β that of β-actin. The following primer sequences were used for real-time PCR analysis: hactb, forward − 5’ – CATGTACGTTGCTATCCAGGC − 3’, reverse, 5’ - CTCCTTAATGTCACGCACGAT − 3’; hbnip3, forward − 5’ – AGCATGAGTCTGGACGGAGTAG − 3’, reverse, 5’ – CCTGTTGGTATCTTGTGGTGTCTG − 3’; hcaix, forward − 5’ – GGATCTACCTACTGTTGAGGCT − 3’, reverse, 5’ – CATAGCGCCAATGACTCTGGT − 3’; mGLUT1, forward − 5’ – TCTCGGCTTAGGGCATGGAT − 3’, reverse, 5’ – TCTATGACGCCGTGATAGCAG − 3’; mACTB, forward − 5’ – GGCTGTATTCCCCTCCATCG − 3’, reverse, 5 CCAGTTGGTAACAATGCCATGT − 3’.
Real time oxygen consumption- A SensorDish Reader from Applikon Biotechnology and oxodish plates were used as previously described.Citation68 Briefly, c2bbe monolayers were grown to confluency on 0.33 cm2 inserts with 0.4 μm pore size (Corning). Once confluent, the medium was aspirated, and inserts were transferred to a 24-well oxodish plate with an oxygen sensor at the bottom center of each well. One milliliter of medium was added basolaterally and 250 μl to the apical side and allowed to equilibrate for 1 h at 37°C incubator. Corresponding treatments were added directly to the media, and the oxodish was immediately placed on the sensordish reader on a rotating platform at 37°C. The plate was not sealed to allow for reoxygenation of the media. The oxygen percentage in the media was continuously measured at 1-min intervals. O2 consumption rates were calculated by linear regression of individual plots following a 20-min equilibration period.
Assessment of epithelial barrier function- Caco-2 c2bbe cells were plated on 24-well transwell inserts (Corning), 0.33 cm2 inserts with 0.4 μm pore size. All treatments were applied the day after plating. Transepithelial electrical resistance (TEERs) was monitored every 24 h using an EVOM2 epithelial voltmeter (World Precision Instruments).
Mouse studies- C57BL/6 mice were purchased from Jackson Laboratories. Animals were maintained and bred in standard housing conditions under 24 h/day, 7 days/week veterinary care at the University of Colorado Anschutz Medical Campus (AMC) animal facility. Animal procedures and care were reviewed and approved by the Institutional Animal Care and Use Committee of AMC (protocol number 00182). Seven-to 12-week-old male and female mice received an intraperitoneal injection of equimolar amounts of butyrate (73 mg/kg), 4-mercapto butyrate (80 mg/kg), an established in-vivo PHD inhibitor, dimethyl-oxalylglycine (DMOG) (117 mg/kg)Citation44,Citation45 and PBS (200 µL). Mice were sacrificed after 24 h of treatment, and blood was immediately collected by cardiac puncture, allowed to naturally clot overnight at 4°C followed by centrifuged for serum collection for immediate use. The distal colon (~3 cm) and right kidney were collected for RNA analysis by placing them in TRIzol, followed by tissue homogenization. For protein analysis, colon tissue was solubilized in 500 l of ice-cold radioimmunoprecipitation assay buffer (RIPA, 50 mM Tris-HCl pH 8.0, 1 mM EDTA, 1% Triton-X100, 10% SDS, 0.5% sodium deoxycholate, 150 mM NaCl) containing 1X HALT protease inhibitor. Then, 63 uL of ice-cold colon lysates in RIPA were added to 37
l of Laemmli cocktail (1X Laemmli buffer containing 1X HALT protease inhibitor, 5 mM EDTA, and 100 mM DTT). Samples were spun down before loading. For better HIF1
immunoblotting results, samples were not heated. Blotting was performed as previously described in protein analysis and immunoblotting section.
Cytotoxicity assay- A CyQUANT LDH cytotoxicity assay (Thermofisher scientific) was used to quantify membrane disruption in IECs when exposed to BA and MBA. The assay protocol was followed without modifications. Briefly, T84 cells were plated on a 96-well plate with 200 l of M4 media and incubated at 37°C overnight. Cells were then exposed to various doses of BA and MBA (10 mM, 5 mM, 1 mM, and 0.5 mM in HBSS buffer) for 6 h in triplicates. Control wells contained 1X Triton lysis buffer for maximum LDH release and HBSS for spontaneous LDH release. 50
l of supernatant was recovered from each treatment and transferred to a clear flat-bottom 96 well-plate for analysis. 50
l of the provided kit reaction mixture was mixed in each well and incubated for 30 minutes away from light. Lastly, 50
l of Stop solution was mixed in and the absorbance was immediately measured (490 and 680 nm). 680 nm absorbance was subtracted from 490 nm reads to eliminate background. %Cytotoxicity was calculated as [(Compound treated activity-Spontaneous activity)/(Maximum-Spontaneous)]*100.
Statistical and graphical presentation of data - Statistical analysis and figure generation were performed using GraphPad Prism. Statistical analyses were performed using either One-way or Two-way ANOVA with post-hoc corrections, as indicated in the figures. The p value was specified if the results were significant (p < .05).
Supplemental Material
Download Zip (178.3 KB)Disclosure statement
No potential conflict of interest was reported by the author(s).
Data availability statement
All data generated in this study are included in this article and the supplementary information or are available from the corresponding author on reasonable request.
Supplementary material
Supplemental data for this article can be accessed online at https://doi.org/10.1080/19490976.2023.2267706
Additional information
Funding
References
- Morrison DJ, Preston T. Formation of short chain fatty acids by the gut microbiota and their impact on human metabolism. Gut Microbes. 2016;7(3):189–16. doi:10.1080/19490976.2015.1134082.
- Parada Venegas D, De la Fuente MK, Landskron G, González MJ, Quera R, Dijkstra G, Harmsen HJM, Faber KN, Hermoso MA. Short Chain Fatty Acids (SCFAs)-mediated gut epithelial and immune regulation and its relevance for inflammatory bowel diseases. Front Immunol. 2019;10:227. doi:10.3389/fimmu.2019.01486.
- Deleu S, Machiels K, Raes J, Verbeke K, Vermeire S. Short chain fatty acids and its producing organisms: an overlooked therapy for IBD? EBioMedicine. 2021;66:103293. doi:10.1016/j.ebiom.2021.103293.
- Topping DL, Clifton PM. Short-chain fatty acids and human colonic function: roles of resistant starch and nonstarch polysaccharides. Physiol Rev. 2001;81(3):1031–1064. doi:10.1152/physrev.2001.81.3.1031.
- Cummings JH, Pomare EW, Branch WJ, Naylor CP, Macfarlane GT. Short chain fatty acids in human large intestine, portal, hepatic and venous blood. Gut. 1987;28(10):1221–1227. doi:10.1136/gut.28.10.1221.
- Guilloteau P, Martin L, Eeckhaut V, Ducatelle R, Zabielski R, Van Immerseel F. From the gut to the peripheral tissues: the multiple effects of butyrate. Nutr Res Rev. 2010;23(2):366–384. doi:10.1017/s0954422410000247.
- Liu H, Wang J, He T, Becker S, Zhang G, Li D, Ma X. Butyrate: a Double-edged sword for health? Adv Nutr. 2018;9(1):21–29. doi:10.1093/advances/nmx009.
- Ornelas A, Dowdell AS, Lee JS, Colgan SP. Microbial metabolite regulation of epithelial cell-cell Interactions and barrier function. Cells. 2022;11(6):944. doi:10.3390/cells11060944.
- Wang RX, Lee JS, Campbell EL, Colgan SP. Microbiota-derived butyrate dynamically regulates intestinal homeostasis through regulation of actin-associated protein synaptopodin. Proc Natl Acad Sci. 2020;117(21):11648–11657. doi:10.1073/pnas.1917597117.
- Davie JR. Inhibition of histone deacetylase activity by butyrate. J Nutr. 2003;133(7):2485S–2493S. doi:10.1093/jn/133.7.2485s.
- de Clercq NC, Groen AK, Romijn JA, Nieuwdorp M. Gut microbiota in obesity and undernutrition. Adv Nutr. 2016;7(6):1080–1089. doi:10.3945/an.116.012914.
- Salvi PS, Cowles RA. Butyrate and the intestinal epithelium: modulation of proliferation and inflammation in homeostasis and disease. Cells. 2021;10(7):1775. doi:10.3390/cells10071775.
- Tan J, McKenzie C, Potamitis M, Thorburn AN, Mackay CR, Macia L. The role of short-chain fatty acids in health and disease. Adv Immunol. 2014;121:91–119. doi:10.1016/b978-0-12-800100-4.00003-9.
- Hamer HM, Jonkers D, Venema K, Vanhoutvin S, Troost FJ, Brummer RJ. Review article: the role of butyrate on colonic function. Aliment Pharmacol Ther. 2007;27(2):104–119. doi:10.1111/j.1365-2036.2007.03562.x.
- Kelly C, Zheng L, Campbell E, Saeedi B, Scholz C, Bayless A, Wilson K, Glover L, Kominsky D, Magnuson A, et al. Crosstalk between microbiota-derived Short-chain fatty acids and intestinal epithelial HIF augments tissue barrier function. Cell Host & Microbe. 2015;17(5):662–671. doi:10.1016/j.chom.2015.03.005.
- Wang RX, Henen MA, Lee JS, Vögeli B, Colgan SP. Microbiota-derived butyrate is an endogenous HIF prolyl hydroxylase inhibitor. Gut Microbes. 2021;13(1):1938380. doi:10.1080/19490976.2021.1938380.
- Giaccia A, Siim BG, Johnson RS. HIF-1 as a target for drug development. Nat Rev Drug Discov. 2003;2(10):803–811. doi:10.1038/nrd1199.
- Palazon A, Goldrath Ananda W, Nizet V, Johnson Randall S. HIF transcription factors, inflammation, and Immunity. Immunity. 2014;41(4):518–528. doi:10.1016/j.immuni.2014.09.008.
- Kelly CJ, Glover LE, Campbell EL, Kominsky DJ, Ehrentraut SF, Bowers BE, Bayless AJ, Saeedi BJ, Colgan SP. Fundamental role for HIF-1alpha in constitutive expression of human beta defensin-1. Mucosal Immunol. 2013;6(6):1110–1118. doi:10.1038/mi.2013.6.
- Fong GH, Takeda K. Role and regulation of prolyl hydroxylase domain proteins. Cell Death Differ. 2008;15(4):635–641. doi:10.1038/cdd.2008.10.
- Kaelin WG. PROLINE HYDROXYLATION and GENE EXPRESSION. Annu Rev Biochem. 2005;74(1):115–128. doi:10.1146/annurev.biochem.74.082803.133142.
- Semenza GL. Hypoxia-inducible factors in physiology and medicine. Cell. 2012;148(3):399–408. doi:10.1016/j.cell.2012.01.021.
- Kaelin WG, Ratcliffe PJ. Oxygen sensing by Metazoans: the central role of the HIF hydroxylase pathway. Mol Cell. 2008;30(4):393–402. doi:10.1016/j.molcel.2008.04.009.
- Roediger WE. Role of anaerobic bacteria in the metabolic welfare of the colonic mucosa in man. Gut. 1980;21(9):793–798. doi:10.1136/gut.21.9.793.
- Kelly CJ, Zheng L, Campbell EL, Saeedi B, Scholz CC, Bayless AJ, Wilson KE, Glover LE, Kominsky DJ, Magnuson A, et al. Crosstalk between microbiota-derived Short-chain fatty acids and intestinal epithelial HIF augments tissue barrier function. Cell Host Microbe. 2015;17(5):662–671. doi:10.1016/j.chom.2015.03.005.
- Mani S. Microbial metabolite mimicry: one step closer to drug discovery. Oncotarget. 2020;11(19):1680. doi:10.18632/oncotarget.27591.
- Dvořák Z, Kopp F, Costello CM, Kemp JS, Li H, Vrzalová A, Štěpánková M, Bartoňková I, Jiskrová E, Poulíková K, et al. Targeting the pregnane X receptor using microbial metabolite mimicry. EMBO Mol Med. 2020;12(4):e11621. doi:10.15252/emmm.201911621.
- Nuzzo A, Brown JR. Microbiome metabolite mimics accelerate drug discovery. Trends Mol Med. 2020;26(5):435–437. doi:10.1016/j.molmed.2020.03.006.
- Parajuli P, Gokulan K, Khare S. Preclinical in vitro model to assess the changes in permeability and cytotoxicity of polarized intestinal epithelial cells during exposure mimicking oral or intravenous routes: an example of arsenite exposure. Int J Mol Sci. 2022;23(9):4851. doi:10.3390/ijms23094851.
- Heikal L, Ghezzi P, Mengozzi M, Ferns G. Assessment of HIF-1α expression and release following endothelial injury in-vitro and in-vivo. Mol Med. 2018;24(1):22. doi:10.1186/s10020-018-0026-5.
- Zhang Y, Liu D, Hu H, Zhang P, Xie R, Cui W. HIF-1α/BNIP3 signaling pathway-induced-autophagy plays protective role during myocardial ischemia-reperfusion injury. Biomed Pharmacother. 2019;120:109464. doi:10.1016/j.biopha.2019.109464.
- Dowdell AS, Cartwright IM, Kitzenberg DA, Kostelecky RE, Mahjoob O, Saeedi BJ, Welch N, Glover LE, Colgan SP. Essential role for epithelial HIF-mediated xenophagy in control of salmonella infection and dissemination. Cell Rep. 2022;40(13):111409. doi:10.1016/j.celrep.2022.111409.
- Potter C, Harris AL. Hypoxia inducible carbonic anhydrase IX, marker of tumour hypoxia, survival pathway and therapy target. Cell Cycle. 2004;3(2):164–167. doi:10.4161/cc.3.2.618.
- Bagiyan GA, Koroleva IK, Soroka NV, Ufimtsev AV. Ufimtsev AV. Russian Chem Bulletin. 2003;52(5):1135–1141. doi:10.1023/a:1024761324710.
- Beinert H, Kennedy MC, Stout CD. Aconitase as iron−Sulfur protein, Enzyme, and iron-regulatory protein. Chem Rev. 1996;96(7):2335–2374. doi:10.1021/cr950040z.
- Dowdell AS, Cartwright IM, Goldberg MS, Kostelecky R, Ross T, Welch N, Glover LE, Colgan SP, Yap A. The HIF target ATG9A is essential for epithelial barrier function and tight junction biogenesis. Mol Biol Cell. 2020;31(20):2249–2258. doi:10.1091/mbc.e20-05-0291.
- Glover LE, Bowers BE, Saeedi B, Ehrentraut SF, Campbell EL, Bayless AJ, Dobrinskikh E, Kendrick AA, Kelly CJ, Burgess A, et al., Control of creatine metabolism by HIF is an endogenous mechanism of barrier regulation in colitis, Proc Natl Acad Sci U S A. 2013;110(49):19820–19825. Epub 2013 Nov 18. doi:10.1073/pnas.1302840110.
- Persson HL, Svensson AI, Brunk UT. A-Lipoic acid and a-lipoamide prevent oxidant-induced lysosomal rupture and apoptosis. Redox Report. 2001;6(5):327–334. doi:10.1179/135100001101536472.
- Flora SJS. Structural, chemical and biological aspects of antioxidants for strategies against metal and metalloid exposure. Oxid Med Cell Longev. 2009;2(4):191–206. doi:10.4161/oxim.2.4.9112.
- Glover LE, Colgan SP. Epithelial barrier regulation by Hypoxia-Inducible Factor. Ann Am Thorac Soc. 2017;14(Supplement_3):S233–S236. doi:10.1513/AnnalsATS.201608-610MG.
- Zheng L, Kelly CJ, Battista KD, Schaefer R, Lanis JM, Alexeev EE, Wang RX, Onyiah JC, Kominsky DJ, Colgan SP. Microbial-derived butyrate promotes epithelial barrier function through IL-10 receptor-dependent repression of claudin-2. J Immunol. 2017;199(8):2976–2984. doi:10.4049/jimmunol.1700105.
- Curtis VF, Ehrentraut SF, Campbell EL, Glover LE, Bayless A, Kelly CJ, Kominsky DJ, Colgan SP. Stabilization of HIF through inhibition of cullin-2 neddylation is protective in mucosal inflammatory responses. FASEB J. 2015;29(1):208–215. doi:10.1096/fj.14-259663.
- Haase VH. Regulation of erythropoiesis by hypoxia-inducible factors. Blood Rev. 2013;27(1):41–53. doi:10.1016/j.blre.2012.12.003.
- Marchbank T, Mahmood A, Harten S, Maxwell PH, Playford RJ. Dimethyloxalyglycine stimulates the early stages of gastrointestinal repair processes through VEGF-dependent mechanisms. Lab Invest. 2011;91(12):1684–1694. doi:10.1038/labinvest.2011.129.
- Song YR, You SJ, Lee Y-M, Chin HJ, Chae D-W, Oh YK, Joo KW, Han JS, Na KY. Activation of hypoxia-inducible factor attenuates renal injury in rat remnant kidney. Nephrol Dial Transpl. 2009;25(1):77–85. doi:10.1093/ndt/gfp454.
- Colgan SP, Wang RX, Hall CHT, Bhagavatula G, Lee JS. Revisiting the “starved gut” hypothesis in inflammatory bowel disease. Immunometabolism (Cobham). 2023;5(1):e0016. doi:10.1097/IN9.0000000000000016.
- Hamer HM, Jonkers D, Venema K, Vanhoutvin S, Troost FJ, Brummer RJ. Review article: the role of butyrate on colonic function. Aliment Pharmacol Ther. 2008;27(2):104–119. doi:10.1111/j.1365-2036.2007.03562.x.
- Machiels K, Joossens M, Sabino J, Preter VD, Arijs I, Eeckhaut V, Ballet V, Claes K, Immerseel FV, Verbeke K, et al. A decrease of the butyrate-producing species roseburia hominis and faecalibacterium prausnitzii defines dysbiosis in patients with ulcerative colitis. Gut. 2014;63(8):1275–1283. doi:10.1136/gutjnl-2013-304833.
- Saeedi BJ, Kao DJ, Kitzenberg DA, Dobrinskikh E, Schwisow KD, Masterson JC, Kendrick AA, Kelly CJ, Bayless AJ, Kominsky DJ, et al. HIF-dependent regulation of claudin-1 is central to intestinal epithelial tight junction integrity. Mol Biol Cell. 2015;26(12):2252–2262. doi:10.1091/mbc.E14-07-1194.
- Ramakrishnan SK, Shah YM. Role of intestinal HIF-2α in health and disease. Annu Rev Physiol. 2016;78(1):301–325. doi:10.1146/annurev-physiol-021115-105202.
- Cummins EP, Crean D. Hypoxia and inflammatory bowel disease. Microbes Infect. 2017;19(3):210–221. doi:10.1016/j.micinf.2016.09.004.
- Lei X, Teng W, Fan Y, Zhu Y, Yao L, Li Y, Zhu S, Pileggi M. The protective effects of HIF-1α activation on sepsis induced intestinal mucosal barrier injury in rats model of sepsis. PloS One. 2022;17(5):e0268445. doi:10.1371/journal.pone.0268445.
- Karhausen J, Furuta GT, Tomaszewski JE, Johnson RS, Colgan SP, Haase VH. Epithelial hypoxia-inducible factor-1 is protective in murine experimental colitis. J Clin Invest. 2004;114(8):1098–1106. doi:10.1172/JCI200421086.
- Taylor CT, Scholz CC. The effect of HIF on metabolism and immunity. Nat Rev Nephrol. 2022;18(9):573–587. doi:10.1038/s41581-022-00587-8.
- DeGruttola AK, Low D, Mizoguchi A, Mizoguchi E. Current understanding of dysbiosis in disease in human and Animal models. Inflamm Bowel Dis. 2016;22(5):1137–1150. doi:10.1097/mib.0000000000000750.
- Gasaly N, Hermoso MA, Gotteland M. Butyrate and the fine-tuning of colonic homeostasis: implication for inflammatory bowel diseases. Int J Mol Sci. 2021;22(6):3061. doi:10.3390/ijms22063061.
- Kim JW, Tchernyshyov I, Semenza GL, Dang CV. HIF-1-mediated expression of pyruvate dehydrogenase kinase: a metabolic switch required for cellular adaptation to hypoxia. Cell Metab. 2006;3(3):177–185. doi:10.1016/j.cmet.2006.02.002.
- Lu CW, Lin SC, Chen KF, Lai YY, Tsai SJ. Induction of pyruvate dehydrogenase kinase-3 by hypoxia-inducible factor-1 promotes metabolic switch and drug resistance. J Biol Chem. 2008;283(42):28106–28114. doi:10.1074/jbc.M803508200.
- Li H, Ranhotra HS, Mani S, Dvořák Z, Sokol H, Müller R. Human microbial metabolite mimicry as a strategy to expand the chemical space of potential drugs. Drug Discov Today. 2020;25(9):1575–1579. doi:10.1016/j.drudis.2020.06.007.
- Liao S-Y, Lerman MI, Stanbridge EJ. Expression of transmembrane carbonic anhydrases, CAIX and CAXII, in human development. BMC Dev Biol. 2009;9(1):22. doi:10.1186/1471-213X-9-22.
- Ulrich K, Jakob U. The role of thiols in antioxidant systems. Free Radic Biol Med. 2019;140:14–27. doi:10.1016/j.freeradbiomed.2019.05.035.
- Van Der Schoor SRD, Reeds PJ, Stoll B, Henry JF, Rosenberger JR, Burrin DG, Van Goudoever JB. The high metabolic cost of a functional gut. Gastroenterology. 2002;123(6):1931–1940. doi:10.1053/gast.2002.37062.
- Glover LE, Colgan SP. Hypoxia and metabolic factors that influence inflammatory bowel disease pathogenesis. Gastroenterology. 2011;140(6):1748–1755. doi:10.1053/j.gastro.2011.01.056.
- Steiner CA, Cartwright IM, Taylor CT, Colgan SP. Hypoxia-inducible factor as a bridge between healthy barrier function, wound healing, and fibrosis. Am J Physiol-Cell Ph. 2022;323(3):C866–C878. doi:10.1152/ajpcell.00227.2022.
- Sandborn WJ, Ghosh S, Panes J, Schreiber S, D’Haens G, Tanida S, Siffledeen J, Enejosa J, Zhou W, Othman AA, et al. Efficacy of upadacitinib in a randomized trial of patients with active ulcerative colitis. Gastroenterology. 2020;158(8):2139–2149 e2114. doi:10.1053/j.gastro.2020.02.030.
- D’Haens G, Panes J, Louis E, Lacerda A, Zhou Q, Liu J, Loftus EV Jr. Upadacitinib was Efficacious and well-tolerated over 30 months in patients with Crohn’s disease in the CELEST Extension study. Clin Gastroenterol Hepatol. 2021;20(10):2337–2346.e3. doi:10.1016/j.cgh.2021.12.030.
- Peterson MD, Mooseker MS. Characterization of the enterocyte-like brush border cytoskeleton of the C2BBe clones of the human intestinal cell line, Caco-2. J Cell Sci. 1992;102(Pt 3):581–600. doi:10.1242/jcs.102.3.581.
- Campbell EL, Bruyninckx WJ, Kelly CJ, Glover LE, McNamee EN, Bowers BE, Bayless AJ, Scully M, Saeedi BJ, Golden-Mason L, et al. Transmigrating neutrophils shape the mucosal microenvironment through localized oxygen depletion to influence resolution of inflammation. Immunity. 2014;40(1):66–77. doi:10.1016/j.immuni.2013.11.020.