ABSTRACT
The advent of highthroughput ‘omics’ technologies has improved our knowledge of gut microbiome in human health and disease, including Alzheimer’s disease (AD), a neurodegenerative disorder. Frequent bidirectional communications and mutual regulation exist between the gastrointestinal tract and the central nervous system through the gut-brain axis. A large body of research has reported a close association between the gut microbiota and AD development, and restoring a healthy gut microbiota may curb or even improve AD symptoms and progression. Thus, modulation of the gut microbiota has become a novel paradigm for clinical management of AD, and emerging effort has focused on developing potential novel strategies for preventing and/or treating the disease. In this review, we provide an overview of the connection and causal relationship between gut dysbiosis and AD, the mechanisms of gut microbiota in driving AD progression, and the successes and challenges of implementing available gut microbiome-targeted therapies (including probiotics, prebiotics, synbiotics, postbiotics, and fecal microbiota transplantation) in preventive and/or therapeutic preclinical and clinical intervention studies of AD. Finally, we discuss the future directions in this field.
Introduction
Alzheimer’s disease (AD) is one of the most common forms of dementia that can range in severity from cognitive impairment to incapacitation. It is a neurological disorder characterized by the accumulation of β-amyloid-containing extracellular plaques and tau-containing intracellular neurofibrillary tangles.Citation1 Around 6.07 million people aged 65 and older in the United States experienced AD in 2020, which is predicted to climb to 13.85 million in 2060.Citation2 The mortality of AD is 121,499 individuals in 2019, making it the sixth-leading cause of death in the United States.Citation3 The short life expectancy, low quality of life, and high health-care costs incurred by the AD have triggered a heavy social burden.Citation3 Although the pathogenesis of AD remains incompletely understood, multiple confounding factors that contribute to disease progression have been determined.Citation4 Age, genetics, and family history are the well-established risk factors for AD. Individuals of 65 y of age and older exhibit greater susceptibility to AD than younger people.Citation5 A recent genome-wide association study has identified 38 susceptibility loci regulating AD risk, acting via immune dysregulation and protein catabolism,Citation6 of which the apolipoprotein E (APOE) alleles have the strongest association with the disease, especially APOE4 allele.Citation7 Parental history of dementia has also been linked to an increased risk of dementia.Citation8 Modifiable risk factors (air pollution, lack of education, hearing loss, smoking, etc.) for AD in addition to the aforementioned events also deserve emphasis.Citation9 Although the major strides in the pathophysiology of AD have been made, no ‘magic bullet’ therapies are available. Of note, aducanumab, a monoclonal antibody targeting amyloid β (Aβ), has been granted accelerated approval by the United Food and Drug Administration for treating AD in 2021.Citation10 The divergence related to real-world effectiveness and safety over this first-in-class drug, however, causes anxiety for clinicians and patients.Citation11 Thus, concerted effort to expand the limited treatment options would tremendously aid clinical management of AD.
Although the clinical manifestations of gastrointestinal dysfunction associated with the pathogenesis of Parkinson’s disease,Citation12 another clinicopathological entity of neurodegenerative diseases, are not established in patients with AD, accumulating evidence suggests that the gut microbiota (see Box 1 for definitions)Citation13–19 plays an important role in the onset and progression of AD.Citation20 Alterations in the gut microbiome composition and functionality have been observed both in animal and human studies,Citation21 and the results of fecal microbiota transplantation (FMT) intervention studies conducted in both germ-free (GF) mice and antibiotic-treated pseudo-GF mice further suggested that the gut microbiome is a key driver of AD development.Citation22 The establishment of a causality relationship between alterations in gut microbiota and the progress of AD forms the basis for designing novel therapeutic strategies targeting the gut microbiome, such as probiotics, prebiotics, synbiotics, postbiotics, and FMT.
This review summarizes the current knowledge of the mechanistic role of the gut microbiome in the development of AD and potential gut microbiome-targeting therapies in managing the disease. Finally, we discuss future directions of translating the gut microbiome research into clinical practice.
The gut microbiome and AD
Aberrant gut microbiota in AD patients
Nineteen next-generation sequencing technology-based observational studies have reported the observation of an altered gut microbiota in patients diagnosed with AD compared with healthy controls (Supplementary Table S1). At least 11 phyla, 15 classes, 14 orders, 38 families, 137 genera, and 51 species were regarded as significantly differential bacterial taxa between healthy and diseased individuals, though only a low proportion (26/266, 9.77%) of them altered in one study showed consistent changes in another study (Supplementary Table S2). The most frequently reported differentially abundant families and genera are: the families Enterococcaceae (four studies), Lachnospiraceae (four studies), and Ruminococcaceae (four studies); the genera Bifidobacterium (seven studies), Bacteroides (six studies), Blautia (six studies), Dorea (four studies), Eubacterium (four studies), and Parabacteroides (four studies). However, for most reported differentially abundant taxa, contradictory results are seen between studies. For example, five studies detected more gene sequences representing Bifidobacterium in the gut microbiota of patients with AD, while two studies found an opposite trend of difference. Only few families and genera exhibit a consistent trend of difference between patients with AD and healthy subjects (Supplementary Table S2), including: an increase representation in the families Enterobacteriaceae (two studies), Erysipelotrichaceae (two studies), and Gemellaceae (two studies), but a decrease representation in the family Lachnospiraceae (four studies) and Clostridiaceae (two studies) in the gut microbiota of patients with AD; an increase representation in the genera Akkermansia (three studies), Anaerotruncus (three studies), Collinsella (three studies), Bilophila (two studies), Escherichia-Shigella (two studies), Flavobacterium (two studies), Gemella (two studies), Lactobacillus (two studies), and Solobacterium (two studies), but a decrease representation in the genera Roseburia (three studies), Anaerostipes (two studies), Butyricicoccus (two studies), Coprococcus (two studies), Lachnoclostridium (two studies), Lachnospira (two studies), Megamonas (two studies), Parasutterella (two studies), and Ruminococcus (two studies) in the gut microbiota of patients with AD.
Although the gut microbiota profile in patients with AD represents substantial heterogeneity, patients with AD seem to have a distinct intestinal microbial landscape from healthy individuals, and the role of the differential abundant taxa in the development and pathogenesis in AD are still obscure, which warrants further confirmation and investigation.
Dysregulated gut microbiota in animal models of AD
Similarly, alterations in the gut microbiota composition have been reported in animal models of AD (Supplementary Table S3). One hundred and forty-three bacterial taxa were reported to show statistical significance between the gut microbiota of healthy and AD model mice in 11 observational studies, including seven phyla, four classes, eight orders, 35 families, 69 genera, and 20 species (Supplementary Table S4). At least 14 of these 143 bacterial taxa showed consistent results between at least two independent studies, particularly, the genus Ruminococcus was concordantly reported to be decreased in AD model mice in four unrelated studies. In addition, the genera Desulfovibrio and Lactobacillus were concordantly reported to be increased in AD model mice in three unrelated studies. Notably, the phyla Proteobacteria (five studies) and Verrucomicrobia (four studies), the family Erysipelotrichaceae (four studies), the genus Allobaculum (five studies) and Ruminococcus (four studies) were most frequently reported differentially abundant bacterial taxa in the selected studies. Altogether, these data suggested that the gut microbiota in AD model mice is distinct from those of the healthy controls, and some common differential bacterial taxa have been captured across studies.
It is worth mentioning that the AD differential microbial taxa identified in clinical and preclinical studies are largely non-overlapping (). Such finding represents an enormous challenge of translational gut microbiome research, which is likely due to the substantial intrinsic difference between the mouse and human gut microbiome configurations.Citation23 In addition, 77.8% (21/27) and 75% (9/12) of the most frequently reported differentially abundant bacterial taxa (reported in at least three studies) showed inconsistent results in human and animal observational studies, respectively, suggesting that there are intrinsic differences both among humans and among animals, not just the differences between humans and animals. The variability of the human and animal microbiota results may be attributed to differences in methodology applied (such as fecal sample preservation, sequencing methods and bioinformatic analyses). Furthermore, factors that influence the gut microbiome should not be ignored, especially diet and lifestyle, as they are crucial determinants of overall gut microbial composition and function.Citation24 Together, these various confounders affecting the heterogeneity between studies should be taken into account in future studies, which will greatly facilitate the repeatability and reproducibility of data between laboratories.
Figure 1. An overview of the most frequently reported differentially abundant gut taxa (reported in at least three studies) of Alzheimer’s disease (AD) in (a) humans and (b) animals.
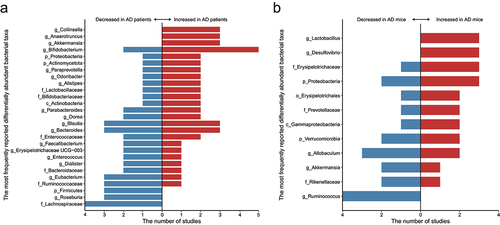
Relationship between the gut microbiome and AD
An overwhelming amount of observational data from animal and human studies have consistently pointed to a close association between the gut microbiota and AD development, and, in recent years, the field of gut microbiome research in AD has started to move rapidly from correlation to causation.
The first generation of studies that disentangle the causality of gut microbiome in AD is mainly achieved by depleting the indigenous gut microbiota in transgenic (Tg) mouse models with AD phenotypes via implementing broad-spectrum combination antibiotics. For example, both short- and long-term antibiotic treatment-induced perturbations in the gut microbiota could lead to decreased Aβ plaque deposition and attenuated plaque-localized glial reactivity in male APP/PS1 mice.Citation25,Citation26 However, the latter effect was not obvious in female APP/PS1 mice,Citation26 suggesting that the gut microbiome played a sex-specific role in brain Aβ amyloidosis and microglial homeostasis.Citation27 Yet it is worth noting that disrupting the gut microbiota by individual antibiotics (rather than a wide-spectrum antibiotic cocktail) was insufficient to reproduce these desired outcomes.Citation28
The second generation of studies dissecting the causal relationship of the gut microbiota in AD development and AD pathology are conducted mainly through FMT experiments, which will be discussed later in the FMT section. Results obtained from the emerging explanatory research have started to present compelling and converging evidence supporting that the gut microbiome is a major determinant driving AD pathology.
Mechanisms of action of gut microbiota in driving AD progression
There is growing evidence that close connections and bidirectional communications exist between the brain and the gut through the gut-brain axis via the immune, endocrine/systemic, and neuronal pathways.Citation29 The following sections discuss new insights into the mechanisms by which the gut microbiota contributes to AD ().
Figure 2. Mechanistic insights of gut microbiota in Alzheimer’s disease (AD) development.
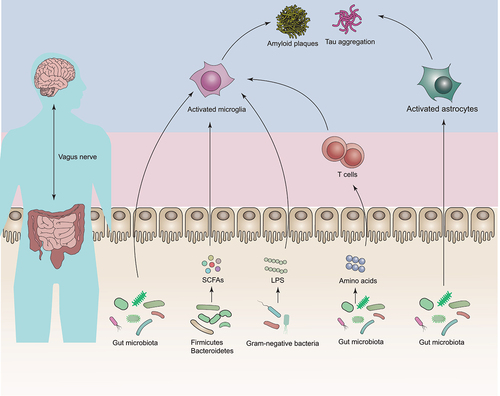
Gut microbiota-microglia connections
Microglia are the resident macrophages of the central nervous system (CNS)Citation30. They are of paramount importance to ensure a healthy brain development and homeostasis under normal conditions. In AD, dynamic changes in microglial activation can affect disease progressionCitation31,Citation32, playing a central role for promoting disease pathogenesis.
It is known that microglia can be orchestrated by gut microbiota. This notion is supported by a groundbreaking animal study, which reported the observations of global defects in microglia in GF mice and severely changed microglia properties in antibiotic-treated specific pathogen-free (SPF) mice and the defective phenotype could be, at least in part, reversed by a co-housing experiment.Citation33 Intriguingly, two other studies further substantiated that the impact of gut microbiota on microglia,Citation34,Citation35 meanwhile reporting that such effect was temporal- and sex-specific.Citation35 Furthermore, the increased gut barrier permeability driven by the gut microbiota during aging results in increased translocation of gut-derived N6-carboxymethyllysine into the brain, consequently triggering oxidative stress and mitochondrial dysfunction in the microglia.Citation36 In fact, it has also been shown that microglia were required in the process of gut microbiota-driven brain Aβ plaque deposition in a mouse model of AD.Citation37,Citation38 Additionally, single-nucleus RNA-sequencing of brain cells of GF, SPF, and colonized-GF mice revealed that the absence of gut microbiota could lead to cell-specific transcriptomic changes, preferentially in microglia; and such cellular alterations were associated with AD and could be effectively reversed by microbial colonization.Citation39 Another study conducted by Seo et al. (2023) found that GF or antibiotic-treated genetically engineered mice of tauopathy expressing human APOE isoforms exhibited reduced gliosis, tau pathology, and neurodegeneration in a sex- and APOE isoform-dependent manner, providing direct evidence of the role of the gut microbiota in interactive regulation of neuroinflammation and tau-mediated neurodegeneration.Citation40
Gut microbiota-astrocytes connections
Astrocytes are the predominant type of glial cells in the CNS, which is known to play a part in the cellular phase of AD.Citation41 Although several recent studies have further reinforced the essential role of astrocytes in AD pathogenesis,Citation42–44 the connections between astrocytes and intestinal microbiota are less explored compared with microglia.
One previous work found that transferring the feces from 16-month-old APP/PS1 mice to antibiotic-pretreated 3-month-old APP/PS1 mice for 7 d could lead to increased Aβ deposition, presumably through inhibiting the activation of astrocytes around Aβ plaques.Citation45 In a mouse model of multiple sclerosis, another nervous system disease, the combined application of microbial metabolites of tryptophan and type I interferons could activate aryl hydrocarbon receptor signaling in astrocytes and suppress CNS inflammation.Citation46 In an experimental autoimmune encephalomyelitis model, it was found that gut microbiome-driven production of interferon-γ by meningeal natural killer cells could upregulate the expression of the tumor necrosis factor-related apoptosis inducing ligand in astrocytes, thereby limiting CNS inflammation through inducing T cell apoptosis.Citation47 Together, these limited data suggest that the gut microbiota has the capacity to modulate astrocyte function, and whether and how these astrocyte-based anti-inflammatory mechanisms affect AD remains to be further investigated.
Gut microbiota-T cell connections
The gut microbiota can manipulate immune cells to influence disease manifestation, such as strokeCitation48 and multiple sclerosis.Citation47 Thus, apart from the CNS immune cells, such as microglia and astrocytes, brain-infiltrated peripheral immune cells may also play a part in AD neuropathogenesis.Citation49–52
A previous study found that, the shift in the gut microbiota during AD progression led to the peripheral accumulation of phenylalanine and isoleucine, stimulating the differentiation and proliferation of pro-inflammatory T helper 1 (Th1) cells. Pro-inflammatory Th1 cells were associated with the M1 microglia activation, contributing to AD-associated neuroinflammation and cognitive impairment. Moreover, healthy control mice exhibited an increase in Th1 cell infiltration and M1 microglia activation when exposed to fecal bacteria of AD mice by co-housing or FMT, and FMT of WT mice feces into AD mice could reduce Th1 cells of the recipient mice.Citation53 The study supported that gut dysbiosis is a driver for AD progression. Given the interconnectedness of the gut microbiota and immune system in neurodegenerative diseases, continual efforts should be made to decipher the precise role of T cells and other immune cells in the development of AD through the control of the gut microbiota.Citation54
In addition, it is noteworthy that gut-derived metabolites are integral to AD development.Citation55 Although changes in metabolite profile with respect to AD have been identified with the help of metabolomics, including but not limited to short-chain fatty acids (SCFAs),Citation56 lipopolysaccharides (LPS),Citation56 bile acids,Citation57 trimethylamine-N-oxideCitation58 and amino acids,Citation59 few studies have investigated the causative links between these bioactive compounds and AD progression. Two distinct mouse models of AD have provided elegant examples of cause- and- effect relationships between the SCFAs and the disease progress. In these studies, it was shown that supplementing SCFAs to either GF APP/PS1 or 5×FAD mice could lead to increased Aβ plaque deposition through modulating plaque-associated microglial functions, compared to their SPF.Citation60,Citation61 Akin to the notion that SCFA supplementation could modulate microglia and worsen disease progression in a mouse model of Parkinson’s disease.Citation62 These converging studies suggest a detrimental effect of SCFAs on neurodegenerative diseases. Conversely, SCFAs have also been shown to be beneficial for AD in vitro and in vivo.Citation63–65 In addition, LPS, pro-inflammatory compounds produced by Gram-negative bacterial membranes, could also lead to amyloid and tau pathology in animal models.Citation66,Citation67 The role of other metabolites in AD needs to be further validated and tested in mechanistic models.
Collectively, studies dissecting the potential mechanisms by which the gut microbiota and their metabolites influence AD remain in its infancy, and additional research is needed to explore whether other action pipelines exist.
Gut microbiome-targeted therapies
Given that the tight connection between the gut microbiota and AD, and the mounting evidence supporting that microbial dysbiosis is a driver of the onset and progression of AD, attempts to circumvent AD through microbiome modification that restores gut homeostasis hold great promise. In the following sections, we summarize available strategies that target the gut microbiota, including probiotics, prebiotics, synbiotics, postbiotics, and FMT (), and current challenges.
Figure 3. Microbiome-based therapeutics in Alzheimer’s disease (AD).
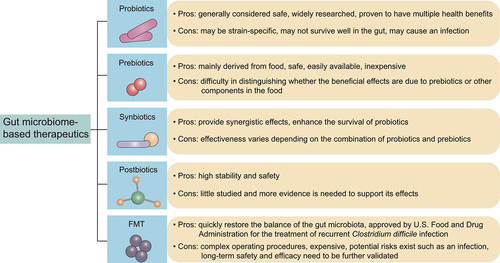
Probiotics
The excellent ability of probiotics to modulate the gut microbiota has motivated their widespread use in various disease management, ranging from intestinal diseases to extraintestinal diseases, including AD.
Preclinical evidence
While experimental animal models of AD might not accurately recapitulate clinical scenarios, they are essential for us to understand AD pathophysiology and related drug development. Animal models of AD include Tg and non-Tg models, of which Tg mouse models are most commonly used.Citation68 Probiotic intervention studies in AD Tg rodent models are summarized in .Citation69–95
Table 1. Comprehensive summary of probiotic intervention studies of Alzheimer’s disease (AD) using transgenic rodent models.
The pioneering work of Bonfili et al. (2017) provided evidence supporting the improvement effect of SLAB51 probiotic formulation in reducing cognitive decline, Aβ plaque and brain damages through restoring the gut microbial homeostasis in 3×Tg AD mice, providing a paradigm for managing AD with probiotic-based therapeutics.Citation69 Over the ensuing years, the neuroprotective effects of a multitude of probiotics on AD were investigated, including not only well-known probiotic species belonging to the genera Lactobacillus and Bifidobacterium, such as Lactobacillus plantarum, Citation71,Citation80,Citation85 Bifidobacterium longum, Citation72,Citation81 Lactobacillus lactis subsp. cremoris, Citation74 Bifidobacterium lactis, Citation82 and Bifidobacterium breve, Citation89,Citation91,Citation95 but also less conventional probiotic species including Akkermansia muciniphila, Citation77 Clostridium butyricum, Citation78 Agathobaculum butyriciproducens, Citation83 and Saccharomyces boulardii. Citation93 Besides single-strain probiotics, some intervention trials evaluated the beneficial effects of multi-strain probiotics in AD.Citation69,Citation70,Citation73,Citation75,Citation76,Citation79,Citation84,Citation87,Citation88,Citation90,Citation92,Citation94
Most of these animal-based probiotic intervention trials have observed some extent of beneficial or even therapeutic effects on AD after the intervention with only few exceptions, although different study parameters were used, including probiotic strain used, probiotic dose, treatment duration, and the animal model. For example, orally administered Saccharomyces boulardii HANSEN CBS 5926 had the potential to attenuate cognitive impairment of APP/PS1 mice by regulation of the fungal microbiota-gut-brain axis.Citation93 Conversely, administering Lactobacillus plantarum PS128 did not significantly change the cognitive dysfunction, levels of Aβ- and tau-related proteins, astrocyte activation state, synaptic plasticity, and cognition-related neuronal loss in 3×Tg AD mice.Citation85
Strain specificity is one of the most influential factors contributing to the observed heterogeneity in therapeutic effects across studies. A previous study investigated how the physiological characteristics of different Lactobacillus plantarum were related to their ulcerative colitis-alleviating function, and, after extensive experimentation, it was discovered that conjugated linoleic acid synthesizing capacity could be a determining factor associating with ulcerative colitis alleviation.Citation96 Beck et al. (2022) reported that probiotic strain-specificity is an important driver of gut microbiome development in preterm infants, influencing the host-microbe interaction in human gut in the very early stage of life.Citation97 The implementation of genetically engineered probiotics that express a specific functional molecule against AD, such as p62 protein, a multi-functional signaling protein involved in regulating protein turnover via the ubiquitin-proteasome system and autophagy, cell proliferation and death, oxidative stress, inflammation and immune response has been demonstrated to be a safe, non-pathogenic, and noninvasive approach to deliver therapeutics to mice with AD.Citation74 Genetically designed AD-targeting therapeutic probiotics may represent a novel paradigm for realistic therapeutic management in AD compared to traditional natural probiotics.
Furthermore, animal models also seem to be a vital determinant of discordant results, as there were minimal to no preclinical benefits from Lactobacillus plantarum PS128 consumption in 3×Tg mice, whereas these preclinical benefits were reversed when mice were injected intracerebroventricularly with streptozotocin, a chemical that is extensively applied in constructing diabetic animal models.Citation85
Sexual dimorphism appears to be a characteristic in AD, contributing to a divergent therapeutic effect between male and female subjects. For instance, VSL#3 supplementation was correlated with reduced Aβ plaque load, improved memory, and decreased microgliosis in female AppNL-G-F but not male mice, pointing to a sex-dependent probiotic effect.Citation86 This interesting finding not only explains part of the discrepant probiotic therapeutic effects observed between studies but also provides an important insight into setting specific guidelines in designing clinical studies addressing the therapeutic efficacy of treatments in a sex-dependent manner.
Finally, whether other study design parameters, such as probiotic dose and treatment duration, would influence the clinical outcomes of probiotic application should be systematically investigated.
Clinical evidence
Akbari et al. (2016) conducted a randomized, double-blind, and controlled clinical trial (n = 60) to evaluate the effects of a multispecies probiotic milk (containing Lactobacillus acidophilus, Lactobacillus casei, Bifidobacterium bifidum, and Lactobacillus fermentum) and found that a 12-week probiotic consumption could improve the cognitive function (measured by mini-mental state examination score) and affect the metabolic statuses of patients with AD.Citation98 This early study has provided a rationale for the clinical practice of probiotic-assisted therapy in AD management, and, shortly thereafter, a number of other probiotic intervention studies were published, in which the beneficial effects of administering a variety of probiotic strains in AD were tested (), including Lactobacillus plantarum (n = 100),Citation101 Bifidobacterium breve (n = 27, 121, 80, 80, 130),Citation102,Citation103,Citation105,Citation106,Citation108 Bifidobacterium longum (n = 90),Citation107 Lactobacillus rhamnosus (n = 90),Citation107 and some probiotic consortia (n = 60, 60, 20, 90).Citation98–100,Citation104 As in the probiotic intervention trials conducted in animals, the therapeutic effects of probiotics in AD seen in human subjects are promising. Although these studies applied a considerably wide range of probiotic doses (from as low as 3 × 109 to as much as 1 × 1015 colony-forming units) and treatment durations (lasting between 1 and 6 months), most studies (nine out of 10) consistently reported significant clinical effects on improving cognitive impairment after probiotic administration. However, it is worth noting that the randomized, double-blind, and placebo-controlled clinical trial (n = 60) conducted by Agahi et al. (2018) found that the cognitive (Test Your Memory score) and biochemical (oxidant/antioxidant and inflammatory/anti-inflammatory biomarkers) functions in patients with severe AD were insensitive to the probiotic supplementation.Citation99 Thus, the disease stage of AD should be taken in consideration when designing treatment regimen.
Table 2. Comprehensive summary of human probiotic intervention studies of Alzheimer’s disease (AD).
Despite the excitement around health benefits from probiotics in AD clinical trials, these studies have several bottlenecks. First, the sample size of some studies was small, decreasing the statistical power and effect size.Citation100,Citation102 Second, the design of some of the clinical trials did not adhere to the general principles of control, randomization, or blinding.Citation98,Citation100,Citation102 Third, it would be more conclusive to include key clinical indicators of diagnostic and therapeutic implication, fulling the primary neuropathologic criteria for AD, such as the presence/level of extracellular Aβ deposition and intracellular accumulation of hyperphosphorylated tau in the brain.Citation109 However, only a paucity of studies have focused on these definitive pathological parameters.
In short, in both human and animal intervention trials, probiotic administration has shown consistent and promising symptom alleviation effects in AD, opening a new paradigm for probiotic-assisted therapy in managing AD. Yet, a lot more well-planned and large-scale intervention studies would be needed to layout guidelines for testing the therapeutic effects and optimal clinical use of probiotics in AD.
Prebiotics
Prebiotics are “substrates that are selectively utilized by host microorganisms conferring a health benefit”.Citation16 Initially, the concept of prebiotics mainly refers to carbohydrate substances such as oligosaccharides and human milk oligosaccharides, which is later extended to non-carbohydrate substances, mainly including polyunsaturated fatty acids (PUFAs), phenolics, and phytochemicals. Among these prebiotics, PUFAs are of particular relevance to AD. The study of Kalmijn et al. (1997) found an inversely relationship between PUFA-rich fish consumption and risk of AD,Citation110 and such finding has catalyzed investigations into the effects of prebiotics on AD in both animals () and humans ().
Table 3. Summary of common prebiotic intervention studies of Alzheimer’s disease (AD) using transgenic rodent models.
Table 4. Comprehensive summary of human prebiotic intervention studies of Alzheimer’s disease (AD).
Preclinical evidence
Docosahexaenoic acid (DHA) is a PUFA that has been explored extensively as a potential therapeutic option for AD in preclinical studies,Citation111–127,Citation132,Citation134,Citation136–138 but its clinical efficacy varied largely between studies. The AD alleviation effect of carbohydrate-based prebiotics, including but not limited to inulin,Citation128,Citation130 fructooligosaccharide,Citation129,Citation133 xylooligosaccharide,Citation131 and mannanoligosaccharide,Citation135 have also been tested, showing satisfactory results.
A closer examination of many of the studies has revealed pitfalls in the experimental design, rendering their conclusions ineffective. For example, a common experimental design is to compare the therapeutic effects of the control and intervention groups, in which a standard diet and a so-called prebiotic-enriched diet are provided, respectively.Citation114–116,Citation121 Such experimental design assumes that prebiotics are only given to the intervention but not the control group; however, in many studies, the diet of both groups contain carbohydrate- and/or lipid-based prebiotics as part of the basic feed components.Citation114–116,Citation121 Thus, the observed clinical outcome differences between groups do not really reflect the effects of prebiotic intake on AD development or improvement of the subjects. To obviate such limitation, additional supplement with and without prebiotics between treatment and control groups on the basis of a normal equivalent diet would be a more effective approach. Finally, phenolics and phytochemicals have been shown to reduce the risk of AD in both animal and human studies, reviewed by EI Gaamouch et al. (2022).Citation167
Clinical evidence
A number of clinical studies have evaluated the effects of prebiotics on mitigating AD, particularly the functional effect of PUFAs.
The first effort to interrogate the function of prebiotics in AD patients came from the OmegAD trial conducted in 2006, which is a randomized, double-blind, placebo-controlled trial (n = 204). The study preliminarily observed that dietary consumption of omega-3 fatty acids was linked to improved cognitive functions in very mild but not mild to moderate AD patients.Citation139 Subsequently, the OmegAD trial data were reassessed based on patient stratification, focusing on neuropsychiatric symptoms (n = 204),Citation142 release of cytokines (n = 25),Citation143 inflammatory markers (n = 35),Citation144 weight and appetite (n = 204),Citation145 release of prostaglandin F2alpha (n = 25),Citation147 expression of genes (n = 20),Citation149 transthyretin levels (n = 204),Citation150 PUFA levels (n = 40),Citation152 oxidative stress and inflammation (n = 41),Citation153 plasma fatty acid profiles in relation to cognition and gender (n = 174),Citation156 release of specialized pro-resolving mediators (n = 17),Citation159 DNA methylation (n = 63),Citation160 plasma total homocysteine level (n = 171),Citation164 and cerebrospinal fluid biomarkers (n = 33).Citation165 Aside from the well-known OmegAD study conducted in Sweden, other interventional studies involving prebiotics were conducted in eight countries, including Japan (n = 29),Citation140 China (n = 46, 240, 240, 163),Citation141,Citation161,Citation163,Citation166 USA (n = 402, 39, 29),Citation146,Citation155,Citation157 Australia (n = 50),Citation148 Malaysia (n = 36),Citation151 Iran (n = 199),Citation154 UK (n = 76),Citation158 and Germany (n = 49).Citation162 While most of these studies found significant improvement in patient clinical outcomes after prebiotic use, a few studies reported no apparent beneficial effects on AD, in the aspects of neuropsychiatric symptoms,Citation142 inflammatory markers,Citation144 cognitive dysfunction,Citation146,Citation154,Citation158,Citation166 and oxidative stress and inflammation.Citation153
An interesting Cardiovascular Health Cognition Study compared the effects of lean fish versus fatty fish (tuna or other fish) intake on patients with AD, vascular dementia, and other types of dementia (Parkinson’s disease and Lewy body dementia), and in relation to APOE4 status, they found that consumption of fatty fish reduced the risk of AD, Parkinson’s disease and Lewy body dementia, especially in patients without APOE4 allele.Citation168 Transgenic mice expressing the human APOE4 isoform had a lower brain uptake of DHA than those expressing the APOE2 isoform due to limited biodistribution and transport of DHA in cerebral tissues.Citation169 The inter-individual distribution of the APOE4 allele is thus a possible factor contributing the heterogeneous response of PUFA intervention in AD, and such genetic risk factor should be considered when implementing prebiotic therapy, especially PUFAs, in future studies and in patients with AD.
Finally, while most prebiotic intervention studies investigated the beneficial effects of PUFAs on AD, few of them explored the functions of carbohydrate-based prebiotics, such as inulin, fructooligosaccharide, xylooligosaccharide, and mannanoligosaccharide. Given the gut microbiota modulation capacity of carbohydrate-based prebiotics, particularly enriching the SCFA-producers and gut microbes synthesizing beneficial bioactive molecules, it merits further attention.
Synbiotics
While an enormous amount of knowledge has been generated from experimental and clinical studies of probiotic and prebiotic intervention in AD, fewer studies have looked into the beneficial effects of synbiotics. By definition, synbiotics are classified into two subsets: a complementary synbiotic that is a mixture of a probiotic plus a prebiotic, and a synergistic synbiotic that is a synbiotic in which the substrate is designed to be selectively utilized by the co-administered microorganism(s).Citation17
Data on the use of synbiotics in Tg AD rodent models are limited to few preclinical studies. Deng et al. (2022) found that the application of a complementary synbiotic, comprising inulin and a multispecies probiotic preparation (containing Bacillus natto, Bacillus coagulans, Lactobacillus casei, Lactobacillus acidophilus, Bifidobacterium longum, and Bifidobacterium breve), but not inulin alone, could ameliorate cognitive impairment, decrease Aβ42 and induce neurogenesis, and reduce the inflammatory response, suggesting that synbiotic but not prebiotic supplementation could delay AD progression via regulating the gut-brain axis.Citation170 Another interesting study conducted in transgenic humanized Drosophila melanogaster of AD demonstrated that administering the synbiotic formulation (containing three metabolically active probiotics, including Lactobacillus plantarum NCIMB 8826, Lactobacillus fermentum NCIMB 5221, and Bifidobacteria longum spp. infantis, and a novel polyphenol-rich prebiotic, namely triphala powder) could increase the survivability, motility, and rescued Aβ deposition and acetylcholinesterase activity of the fruit flies. The observed beneficial effects were likely achieved through gut-brain-axis signaling and regulation of metabolic stability, immune signaling, oxidative, and mitochondrial stress.Citation171
Collectively, limited available evidence is available to demonstrate the beneficial effects of synbiotics in curbing AD progression. It merits more effort to uncover the beneficial synergistic AD remission effect unleashed by synbiotics than administering probiotics or prebiotics alone, particularly by animal and human intervention trials focusing on elucidating the specific synergistic mechanisms of action.
Postbiotics
Albeit extremely rare, probiotic therapy has some safety concerns, such as the spread of antibiotic resistance genes, the presence of potential virulence factors, and the development of bacteremia or fungemia. Thus, functional research of postbiotics has emerged.Citation172 Some of the biggest advantages of postbiotics are their good stability and ease of storage.Citation18 Moreover, increasing evidence shows that postbiotics offer comparable health effects to probiotics, although the available studies are mostly limited to the health-promoting effects of postbiotics in gastrointestinal disorders.Citation172,Citation173 The safety-stability-health claims triangle from postbiotic intervention has unsurprisingly attracted considerable attention.Citation174 Thus, high-quality, well-designed animal models and human studies are still needed to fill the gaps in the realm of AD.
FMT
Historical accounts linking FMT and disease date back to the 4th century BC in China when human fecal suspension was used to treat patients with food poisoning, diarrhea, and those with fever and imminent dying.Citation175 In 1958, the application of FMT in treating Clostridioides difficile infection (CDI)-associated pseudomembranous enterocolitis has opened a new era of modern medical research of FMT.Citation176 The resurgence of FMT in the past decade was due to the efficacy of up to 90% in treating recurrent CDI in a landmark clinical study published in 2013.Citation177 Contemporaneously, a plethora of FMT-related studies have been conducted to explore other potential therapeutic indications associated with an altered gut microbiome, such as inflammatory bowel disease, with the goals of restoring of the gut microbiota to a premorbid state.Citation178 In 2022, the first fecal microbiota product, Rebyota, was approved by the United States Food and Drug Administration for the prevention of recurrence of CDI in individuals 18 y of age and older who have completed antibiotic treatment for recurrent CDI.Citation179 This optimism heralds the emergence of FMT therapy as part of clinical practice. The great progress of FMT in managing gastrointestinal diseases has invigorated its application extending beyond the intestine, including AD ().Citation22,Citation27,Citation45,Citation180–193 However, as seen in , most current FMT-related studies pertaining to AD are animal trials with only very few human clinical studies, and most of these studies demonstrated promising results of FMT therapy in AD, which warrants further evaluation of FMT in both preclinical and clinical research.
Table 5. Comprehensive summary of studies of fecal microbiota transplantation (FMT) in Alzheimer’s disease (AD) transgenic rodent models and patients with AD.
A few problems have been identified in some of the current FMT intervention trials, and, in future interventional research, robust experimental designs are of paramount importance for conducting reliable, valid, and safe studies. For instance, the eradication of indigenous microbial communities of recipient animals via broad-spectrum antibiotics prior to FMT appears to be incomplete, and it is not easy to assess the effect of residual microbiota in the experimental process; thus, it would be vital to use GF animals to conduct studies aiming to elucidate the clinical efficacy of FMT. Furthermore, there is a lack of consensual protocol for FMT implementation in the preclinical research included in this review, ranging from 1 time to 24 times of application. Experimental parameters like the method and frequency of FMT application is particularly important for transitioning from preclinical to clinical translation research, ensure reproducibility between and validity of studies. Finally, despite patients’ safety is the prime concern for implementing any medical treatment, particularly novel therapies like FMT, this is often omitted. Short-term and long-term safety assessment of FMT should be carefully conducted, recorded, and reported.
Future directions
Despite in its infancy, there is a remarkable leap in our understanding of the gut microbiome in AD in the past 5 y. Mounting information has been generated from both descriptive studies that detail the gut microbiome in AD and explanatory studies aiming to interrogate the causal relationship between the gut microbiome and AD. While increasing evidence supports that gut dysbiosis is a driver for AD, it merits more effort to further verify the causal relationship between the two and to dissect the definitive mechanisms for driving the progress of AD. Future development of the field will require more well-designed, large-scale, and precise observational and mechanistic studies. Multi-omics, such as metagenomics, metabolomics, metaproteomics, and metatranscriptomics, are powerful tools that can help answer some fundamental questions regarding the role of gut microbiome in the initiation, maintenance, and progression of AD.
Successful implementation of the described gut microbiota-targeting strategies in AD improvement remains a formidable challenge. One important reason is that the beneficial effects on AD do not solely depend on the regimen but also on the native host gut microbiota. Our previous study found that the inter-individual endogenous gut microbiota composition was an important factor relating to the heterogenous beneficial effects seen across study subjects.Citation194 Similarly, the success of FMT in treating recurrent CDI was principally associated with the gut microbiota composition in the recipients.Citation195 Thus, to maximize treatment benefits, both the specificity of the gut microbiota-modifying products and the host indigenous intestinal microbiome should be taken into account in future studies. Another obvious challenge in applying preclinical data in translational research is the tremendous variability between the rodent and human gut microbiome. Thus, attempts to establish animal models that mimic the characteristics of human intestinal microbiome, e.g., by developing humanized gnotobiotic animal models, will greatly facilitate cross-validation between animal and human studies and, ultimately, promote clinical practice.
Considering that diet and lifestyle are closely related to us and that they are the most influential in shaping gut microbial communities,Citation24,Citation196 they play a pivotal role in AD management.Citation197 For example, the Mediterranean, Dietary Approaches to Stop Hypertension (DASH), and Mediterranean-DASH Intervention for Neurodegenerative Delay (MIND) diets were linked to a reduced risk of AD,Citation198 while the Western diet showed an opposite trend.Citation199 A prospective cohort study conducted in USA and published in 2022 analyzed the impact of lifestyle factors on life expectancy lived with and without Alzheimer’s dementia; it was found that they lived a larger proportion of their remaining years without Alzheimer’s dementia.Citation200 However, a very recent study suggested that the MIND diet could not improve brain health, cognitive function, and brain imaging outcomes compared with those who followed a control diet with mild caloric restriction.Citation201 Despite some unexpected research results, optimism is growing that multidimensional interventions, including dietary patterns and lifestyle, might prevent, or even halt AD development.
Conclusions
A raft of recent observational and mechanistic studies consistently demonstrated that the gut microbiome is a key player in modulating the disease state of AD, opening a new paradigm for intervening or even curbing the progress of AD. Gut microbiome-targeting strategies, such as probiotics, prebiotics, synbiotics, postbiotics, and FMT, hold great promise in the treatment and prophylaxis of AD in the years to come. Yet, concerted effort is still needed to provide answers to unresolved fundamental questions in regards to the role and mechanism of gut microbiota in AD, establish standard protocols for gut microbiome research, and generate high-quality data from preclinical and clinical studies, ultimately translating gut microbiome research into clinical practice.
Authors’ contributions
T.Z. and G.G. designed the outline of the review. T.Z. wrote the manuscript. L.K. and Z.S. critically revised the manuscript and provided advice. All authors read and approved the final manuscript.
Supplemental Material
Download ()Disclosure statement
No potential conflict of interest was reported by the author(s).
Supplementary material
Supplemental data for this article can be accessed online at https://doi.org/10.1080/19490976.2023.2271613
Additional information
Funding
References
- Knopman DS, Amieva H, Petersen RC, Chételat G, Holtzman DM, Hyman BT, Nixon RA, Jones DT. Alzheimer disease. Nat Rev Dis Primers. 2021;7(1):7. doi:10.1038/s41572-021-00269-y.
- Rajan KB, Weuve J, Barnes LL, McAninch EA, Wilson RS, Evans DA. Population estimate of people with clinical Alzheimer’s disease and mild cognitive impairment in the United States (2020-2060). Alzheimers Dement. 2021;17:1966–35. PMID: 34043283. doi:10.1002/alz.12362.
- 2021 Alzheimer’s disease facts and figures. Alzheimers Dement. 2021;17(3):327–406. PMID: 33756057. doi:10.1002/alz.12328.
- Khan S, Barve KH, Kumar MS. Recent advancements in pathogenesis, diagnostics and treatment of Alzheimer’s disease. Curr Neuropharmacol. 2020;18:1106–1125. PMID: 32484110. doi:10.2174/1570159x18666200528142429.
- Scheltens P, De Strooper B, Kivipelto M, Holstege H, Chételat G, Teunissen CE, Cummings J, van der Flier WM. Alzheimer’s disease. Lancet. 2021;397:1577–1590. doi:10.1016/s0140-6736(20)32205-4.
- Wightman DP, Jansen IE, Savage JE, Shadrin AA, Bahrami S, Holland D, Rongve A, Børte S, Winsvold BS, Drange OK, et al. A genome-wide association study with 1,126,563 individuals identifies new risk loci for Alzheimer’s disease. Nat Genet. 2021;53(9):1276–1282. PMID: 34493870. doi:10.1038/s41588-021-00921-z.
- Yamazaki Y, Zhao N, Caulfield TR, Liu CC, Bu G. Apolipoprotein E and Alzheimer disease: pathobiology and targeting strategies. Nat Rev Neurol. 2019;15(9):501–518. PMID: 31367008. doi: 10.1038/s41582-019-0228-7.
- Wolters FJ, van der Lee S J, Koudstaal PJ, van Duijn CM, Hofman A, Ikram MK, Vernooij MW, Ikram MA. Parental family history of dementia in relation to subclinical brain disease and dementia risk. Neurology. 2017;88(17):1642–1649. PMID: 28356461. doi: 10.1212/wnl.0000000000003871.
- Livingston G, Huntley J, Sommerlad A, Ames D, Ballard C, Banerjee S, Brayne C, Burns A, Cohen-Mansfield J, Cooper C, et al. Dementia prevention, intervention, and care: 2020 report of the Lancet commission. Lancet. 2020;396(10248):413–446. PMID: 32738937. doi:10.1016/s0140-6736(20)30367-6.
- FDA. Drugs@FDA: FDA-approved drugs. Aduhelm. https://www.accessdata.fda.gov/scripts/cder/daf/index.cfm?event=overview.process&ApplNo=761178.
- Lythgoe MP, Jenei K, Prasad V. Regulatory decisions diverge over aducanumab for Alzheimer’s disease. Bmj. 2022;e069780. doi:10.1136/bmj-2021-069780.
- Travagli RA, Browning KN, Camilleri M. Parkinson disease and the gut: new insights into pathogenesis and clinical relevance. Nat Rev Gastroenterol Hepatol. 2020;17(11):673–685. PMID: 32737460. doi: 10.1038/s41575-020-0339-z.
- Thursby E, Juge N. Introduction to the human gut microbiota. Biochem J. 2017;474:1823–1836. PMID: 28512250. doi:10.1042/bcj20160510.
- Berg G, Rybakova D, Fischer D, Cernava T, Vergès MC, Charles T, Chen X, Cocolin L, Eversole K, Corral GH, et al. Microbiome definition re-visited: old concepts and new challenges. Microbiome. 2020;8(1):103. PMID: 32605663. doi:10.1186/s40168-020-00875-0.
- Hill C, Guarner F, Reid G, Gibson GR, Merenstein DJ, Pot B, Morelli L, Canani RB, Flint HJ, Salminen S, et al. Expert consensus document. The international scientific association for probiotics and prebiotics consensus statement on the scope and appropriate use of the term probiotic. Nat Rev Gastroenterol Hepatol. 2014;11(8):506–514. PMID: 24912386. doi:10.1038/nrgastro.2014.66.
- Gibson GR, Hutkins R, Sanders ME, Prescott SL, Reimer RA, Salminen SJ, Scott K, Stanton C, Swanson KS, Cani PD, et al. Expert consensus document: The international scientific association for probiotics and prebiotics (ISAPP) consensus statement on the definition and scope of prebiotics. Nat Rev Gastroenterol Hepatol. 2017;14(8):491–502. PMID: 28611480. doi:10.1038/nrgastro.2017.75.
- Swanson KS, Gibson GR, Hutkins R, Reimer RA, Reid G, Verbeke K, Scott KP, Holscher HD, Azad MB, Delzenne NM, et al. The International Scientific association for probiotics and prebiotics (ISAPP) consensus statement on the definition and scope of synbiotics. Nat Rev Gastroenterol Hepatol. 2020;17(11):687–701. PMID: 32826966. doi:10.1038/s41575-020-0344-2.
- Salminen S, Collado MC, Endo A, Hill C, Lebeer S, Quigley EMM, Sanders ME, Shamir R, Swann JR, Szajewska H, et al. The International Scientific association of probiotics and prebiotics (ISAPP) consensus statement on the definition and scope of postbiotics. Nat Rev Gastroenterol Hepatol. 2021;18(9):649–667. PMID: 33948025. doi:10.1038/s41575-021-00440-6.
- Cammarota G, Ianiro G, Tilg H, Rajilić-Stojanović M, Kump P, Satokari R, Sokol H, Arkkila P, Pintus C, Hart A, et al. European consensus conference on faecal microbiota transplantation in clinical practice. Gut. 2017;66(4):569–580. PMID: 28087657. doi:10.1136/gutjnl-2016-313017.
- Cryan JF, O’Riordan KJ, Sandhu K, Peterson V, Dinan TG. The gut microbiome in neurological disorders. Lancet Neurol. 2020;19:179–194. PMID: 31753762. doi:10.1016/S1474-4422(19)30356-4.
- Chandra S, Sisodia SS, Vassar RJ. The gut microbiome in Alzheimer’s disease: what we know and what remains to be explored. Mol Neurodegener. 2023;18:9. PMID: 36721148. doi:10.1186/s13024-023-00595-7.
- Kim MS, Kim Y, Choi H, Kim W, Park S, Lee D, Kim DK, Kim HJ, Choi H, Hyun DW, et al. Transfer of a healthy microbiota reduces amyloid and tau pathology in an Alzheimer’s disease animal model. Gut. 2020;69(2):283–294. PMID: 31471351. doi:10.1136/gutjnl-2018-317431.
- Xiao L, Feng Q, Liang S, Sonne SB, Xia Z, Qiu X, Li X, Long H, Zhang J, Zhang D, et al. A catalog of the mouse gut metagenome. Nat Biotechnol. 2015;33(10):1103–1108. PMID: 26414350. doi:10.1038/nbt.3353.
- Parizadeh M, Arrieta MC. The global human gut microbiome: genes, lifestyles, and diet. Trends Mol Med. 2023;29:789–801. PMID: 37516570. doi:10.1016/j.molmed.2023.07.002.
- Minter MR, Hinterleitner R, Meisel M, Zhang C, Leone V, Zhang X, Oyler-Castrillo P, Zhang X, Musch MW, Shen X, et al. Antibiotic-induced perturbations in microbial diversity during post-natal development alters amyloid pathology in an aged APPSWE/PS1ΔE9 murine model of Alzheimer’s disease. Sci Rep. 2017;7(1):10411. PMID: 28874832. doi:10.1038/s41598-017-11047-w.
- Minter MR, Zhang C, Leone V, Ringus DL, Zhang X, Oyler-Castrillo P, Musch MW, Liao F, Ward JF, Holtzman DM, et al. Antibiotic-induced perturbations in gut microbial diversity influences neuro-inflammation and amyloidosis in a murine model of Alzheimer’s disease. Sci Rep. 2016;6(1):30028. PMID: 27443609. doi:10.1038/srep30028.
- Dodiya HB, Kuntz T, Shaik SM, Baufeld C, Leibowitz J, Zhang X, Gottel N, Zhang X, Butovsky O, Gilbert JA, et al. Sex-specific effects of microbiome perturbations on cerebral Aβ amyloidosis and microglia phenotypes. J Exp Med. 2019;216:1542–1560. PMID: 31097468. doi:10.1084/jem.20182386.
- Dodiya HB, Frith M, Sidebottom A, Cao Y, Koval J, Chang E, Sisodia SS. Synergistic depletion of gut microbial consortia, but not individual antibiotics, reduces amyloidosis in APPPS1-21 Alzheimer’s transgenic mice. Sci Rep. 2020;10(1):8183. PMID: 32424118. doi: 10.1038/s41598-020-64797-5.
- Agirman G, Hsiao EY. SnapShot: The microbiota-gut-brain axis. Cell. 2021;184(9):2524–2524.e2521. PMID: 33930299. doi: 10.1016/j.cell.2021.03.022.
- Perry VH, Nicoll JA, Holmes C. Microglia in neurodegenerative disease. Nat Rev Neurol. 2010;6(4):193–201. PMID: 20234358. doi: 10.1038/nrneurol.2010.17.
- Hansen DV, Hanson JE, Sheng M. Microglia in Alzheimer’s disease. J Cell Biol. 2018;217:459–472. PMID: 29196460. doi:10.1083/jcb.201709069.
- Leng F, Edison P. Neuroinflammation and microglial activation in Alzheimer disease: where do we go from here? Nat Rev Neurol. 2021;17(3):157–172. PMID: 33318676. doi: 10.1038/s41582-020-00435-y.
- Erny D, Hrabe de Angelis AL, Jaitin D, Wieghofer P, Staszewski O, David E, Keren-Shaul H, Mahlakoiv T, Jakobshagen K, Buch T, et al. Host microbiota constantly control maturation and function of microglia in the CNS. Nat Neurosci. 2015;18(7):965–977. PMID: 26030851. doi:10.1038/nn.4030.
- Matcovitch-Natan O, Winter DR, Giladi A, Vargas Aguilar S, Spinrad A, Sarrazin S, Ben-Yehuda H, David E, Zelada Gonzalez F, Perrin P, et al. Microglia development follows a stepwise program to regulate brain homeostasis. Sci. 2016;353(6301):aad8670. PMID: 27338705. doi:10.1126/science.aad8670.
- Thion MS, Low D, Silvin A, Chen J, Grisel P, Schulte-Schrepping J, Blecher R, Ulas T, Squarzoni P, Hoeffel G, et al. Microbiome influences prenatal and adult microglia in a sex-specific manner. Cell. 2018;172(3):500–516 e516. PMID: 29275859. doi:10.1016/j.cell.2017.11.042.
- Mossad O, Batut B, Yilmaz B, Dokalis N, Mezo C, Nent E, Nabavi LS, Mayer M, Maron FJM, Buescher JM, et al. Gut microbiota drives age-related oxidative stress and mitochondrial damage in microglia via the metabolite N(6)-carboxymethyllysine. Nat Neurosci. 2022;25(3):295–305. PMID: 35241804. doi:10.1038/s41593-022-01027-3.
- Dodiya HB, Lutz HL, Weigle IQ, Patel P, Michalkiewicz J, Roman-Santiago CJ, Zhang CM, Liang Y, Srinath A, Zhang X, et al. Gut microbiota–driven brain Aβ amyloidosis in mice requires microglia. J Exp Med. 2022;219(1). PMID: 34854884. doi: 10.1084/jem.20200895.
- Mezo C, Dokalis N, Mossad O, Staszewski O, Neuber J, Yilmaz B, Schnepf D, de Aguero MG, Ganal-Vonarburg SC, Macpherson AJ, et al. Different effects of constitutive and induced microbiota modulation on microglia in a mouse model of Alzheimer’s disease. Acta Neuropathol Commun. 2020;8(1):119. PMID: 32727612. doi:10.1186/s40478-020-00988-5.
- Huang Y, Wu J, Zhang H, Li Y, Wen L, Tan X, Cheng K, Liu Y, Pu J, Liu L, et al. The gut microbiome modulates the transformation of microglial subtypes. Mol Psychiatry. 2023;28(4):1611–1621. PMID: 36914812. doi:10.1038/s41380-023-02017-y.
- Seo DO, O’Donnell D, Jain N, Ulrich JD, Herz J, Li Y, Lemieux M, Cheng J, Hu H, Serrano JR, et al. ApoE isoform– and microbiota-dependent progression of neurodegeneration in a mouse model of tauopathy. Sci. 2023;379(6628):eadd1236. PMID: 36634180. doi:10.1126/science.add1236.
- De Strooper B, Karran E. The cellular phase of Alzheimer’s disease. Cell. 2016;164(4):603–615. PMID: 26871627. doi: 10.1016/j.cell.2015.12.056.
- Endo F, Kasai A, Soto JS, Yu X, Qu Z, Hashimoto H, Gradinaru V, Kawaguchi R, Khakh BS. Molecular basis of astrocyte diversity and morphology across the CNS in health and disease. Sci. 2022;378(6619):eadc9020. PMID: 36378959. doi: 10.1126/science.adc9020.
- Ferrari-Souza JP, Ferreira PCL, Bellaver B, Tissot C, Wang YT, Leffa DT, Brum WS, Benedet AL, Ashton NJ, De Bastiani MA, et al. Astrocyte biomarker signatures of amyloid-β and tau pathologies in Alzheimer’s disease. Mol Psychiatry. 2022;27(11):4781–4789. PMID: 35948658. doi:10.1038/s41380-022-01716-2.
- Mann CN, Devi SS, Kersting CT, Bleem AV, Karch CM, Holtzman DM, Gallardo G. Astrocytic α2-Na + /K + ATPase inhibition suppresses astrocyte reactivity and reduces neurodegeneration in a tauopathy mouse model. Sci Transl Med. 2022;14(632):eabm4107. PMID: 35171651. doi: 10.1126/scitranslmed.abm4107.
- Wang M, Cao J, Gong C, Amakye WK, Yao M, Ren J. Exploring the microbiota-Alzheimer’s disease linkage using short-term antibiotic treatment followed by fecal microbiota transplantation. Brain Behav Immun. 2021;96:227–238. PMID: 34111528. doi:10.1016/j.bbi.2021.06.003.
- Rothhammer V, Mascanfroni ID, Bunse L, Takenaka MC, Kenison JE, Mayo L, Chao CC, Patel B, Yan R, Blain M, et al. Type I interferons and microbial metabolites of tryptophan modulate astrocyte activity and central nervous system inflammation via the aryl hydrocarbon receptor. Nat Med. 2016;22(6):586–597. PMID: 27158906. doi:10.1038/nm.4106.
- Sanmarco LM, Wheeler MA, Gutierrez-Vazquez C, Polonio CM, Linnerbauer M, Pinho-Ribeiro FA, Li Z, Giovannoni F, Batterman KV, Scalisi G, et al. Gut-licensed IFNγ+ NK cells drive LAMP1+TRAIL+ anti-inflammatory astrocytes. Nature. 2021;590(7846):473–479. PMID: 33408417. doi:10.1038/s41586-020-03116-4.
- Benakis C, Brea D, Caballero S, Faraco G, Moore J, Murphy M, Sita G, Racchumi G, Ling L, Pamer EG, et al. Commensal microbiota affects ischemic stroke outcome by regulating intestinal γδ T cells. Nat Med. 2016;22(5):516–523. PMID: 27019327. doi:10.1038/nm.4068.
- Baruch K, Rosenzweig N, Kertser A, Deczkowska A, Sharif AM, Spinrad A, Tsitsou-Kampeli A, Sarel A, Cahalon L, Schwartz M. Breaking immune tolerance by targeting Foxp3+ regulatory T cells mitigates Alzheimer’s disease pathology. Nat Commun. 2015;6(1):7967. PMID: 26284939. doi: 10.1038/ncomms8967.
- Keren-Shaul H, Spinrad A, Weiner A, Matcovitch-Natan O, Dvir-Szternfeld R, Ulland TK, David E, Baruch K, Lara-Astaiso D, Toth B, et al. A unique microglia type associated with restricting development of Alzheimer’s disease. Cell. 2017;169(7):1276–1290.e1217. PMID: 28602351. doi:10.1016/j.cell.2017.05.018.
- Kim K, Wang X, Ragonnaud E, Bodogai M, Illouz T, DeLuca M, McDevitt RA, Gusev F, Okun E, Rogaev E, et al. Therapeutic B-cell depletion reverses progression of Alzheimer’s disease. Nat Commun. 2021;12(1):2185. PMID: 33846335. doi:10.1038/s41467-021-22479-4.
- Chen X, Firulyova M, Manis M, Herz J, Smirnov I, Aladyeva E, Wang C, Bao X, Finn MB, Hu H, et al. Microglia-mediated T cell infiltration drives neurodegeneration in tauopathy. Nature. 2023;615(7953):668–677. PMID: 36890231. doi:10.1038/s41586-023-05788-0.
- Wang X, Sun G, Feng T, Zhang J, Huang X, Wang T, Xie Z, Chu X, Yang J, Wang H, et al. Sodium oligomannate therapeutically remodels gut microbiota and suppresses gut bacterial amino acids-shaped neuroinflammation to inhibit Alzheimer’s disease progression. Cell Res. 2019;29(10):787–803. PMID: 31488882. doi:10.1038/s41422-019-0216-x.
- Fung TC, Olson CA, Hsiao EY. Interactions between the microbiota, immune and nervous systems in health and disease. Nat Neurosci. 2017;20(2):145–155. PMID: 28092661. doi: 10.1038/nn.4476.
- Ahmed H, Leyrolle Q, Koistinen V, Karkkainen O, Laye S, Delzenne N, Hanhineva K. Microbiota-derived metabolites as drivers of gut–brain communication. Gut Microbes. 2022;14(1):2102878. PMID: 35903003. doi: 10.1080/19490976.2022.2102878.
- Marizzoni M, Cattaneo A, Mirabelli P, Festari C, Lopizzo N, Nicolosi V, Mombelli E, Mazzelli M, Luongo D, Naviglio D, et al. Short-chain fatty acids and lipopolysaccharide as mediators between gut dysbiosis and amyloid pathology in Alzheimer’s disease. J Alzheimers Dis. 2020;78(2):683–697. PMID: 33074224. doi:10.3233/JAD-200306.
- MahmoudianDehkordi S, Arnold M, Nho K, Ahmad S, Jia W, Xie G, Louie G, Kueider-Paisley A, Moseley MA, Thompson JW, et al. Altered bile acid profile associates with cognitive impairment in Alzheimer’s disease—an emerging role for gut microbiome. Alzheimers Dement. 2019;15(1):76–92. PMID: 30337151. doi:10.1016/j.jalz.2018.07.217.
- Vogt NM, Romano KA, Darst BF, Engelman CD, Johnson SC, Carlsson CM, Asthana S, Blennow K, Zetterberg H, Bendlin BB, et al. The gut microbiota-derived metabolite trimethylamine N-oxide is elevated in Alzheimer’s disease. Alzheimers Res Ther. 2018;10:124. PMID: 30579367. doi:10.1186/s13195-018-0451-2.
- Madeira C, Vargas-Lopes C, Brandao CO, Reis T, Laks J, Panizzutti R, Ferreira ST. Elevated glutamate and glutamine levels in the cerebrospinal fluid of patients with probable Alzheimer’s disease and depression. Front Psychiatry. 2018;9:561. PMID: 30459657. doi:10.3389/fpsyt.2018.00561.
- Colombo AV, Sadler RK, Llovera G, Singh V, Roth S, Heindl S, Sebastian Monasor L, Verhoeven A, Peters F, Parhizkar S, et al. Microbiota-derived short chain fatty acids modulate microglia and promote Abeta plaque deposition. Elife. 2021;10. PMID: 33845942. doi:10.7554/eLife.59826.
- Erny D, Dokalis N, Mezo C, Castoldi A, Mossad O, Staszewski O, Frosch M, Villa M, Fuchs V, Mayer A, et al. Microbiota-derived acetate enables the metabolic fitness of the brain innate immune system during health and disease. Cell Metab. 2021;33(11):2260–2276 e2267. PMID: 34731656. doi:10.1016/j.cmet.2021.10.010.
- Sampson TR, Debelius JW, Thron T, Janssen S, Shastri GG, Ilhan ZE, Challis C, Schretter CE, Rocha S, Gradinaru V, et al. Gut microbiota regulate motor deficits and neuroinflammation in a model of Parkinson’s disease. Cell. 2016;167(6):1469–1480 e1412. PMID: 27912057. doi:10.1016/j.cell.2016.11.018.
- Ho L, Ono K, Tsuji M, Mazzola P, Singh R, Pasinetti GM. Protective roles of intestinal microbiota derived short chain fatty acids in Alzheimer’s disease-type beta-amyloid neuropathological mechanisms. Expert Rev Neurother. 2018;18:83–90. PMID: 29095058. doi:10.1080/14737175.2018.1400909.
- Fernando W, Martins IJ, Morici M, Bharadwaj P, Rainey-Smith SR, Lim WLF, Martins RN. Sodium butyrate reduces brain amyloid-β levels and improves cognitive memory performance in an Alzheimer’s disease transgenic mouse model at an early disease stage. J Alzheimers Dis. 2020;74(1):91–99. PMID: 31958090. doi: 10.3233/JAD-190120.
- Jiang Y, Li K, Li X, Xu L, Yang Z. Sodium butyrate ameliorates the impairment of synaptic plasticity by inhibiting the neuroinflammation in 5XFAD mice. Chem Biol Interact. 2021;341:109452. PMID: 33785315. doi:10.1016/j.cbi.2021.109452.
- Sheng JG, Bora SH, Xu G, Borchelt DR, Price DL, Koliatsos VE. Lipopolysaccharide-induced-neuroinflammation increases intracellular accumulation of amyloid precursor protein and amyloid β peptide in APPswe transgenic mice. Neurobiol Dis. 2003;14(1):133–145. PMID: 13678674. doi: 10.1016/s0969-9961(03)00069-x.
- Kitazawa M, Oddo S, Yamasaki TR, Green KN, LaFerla FM. Lipopolysaccharide-induced inflammation exacerbates tau pathology by a cyclin-dependent kinase 5-mediated pathway in a transgenic model of Alzheimer’s disease. J Neurosci. 2005;25:8843–8853. PMID: 16192374. doi:10.1523/JNEUROSCI.2868-05.2005.
- Drummond E, Wisniewski T. Alzheimer’s disease: experimental models and reality. Acta Neuropathol. 2017;133(2):155–175. PMID: 28025715. doi: 10.1007/s00401-016-1662-x.
- Bonfili L, Cecarini V, Berardi S, Scarpona S, Suchodolski JS, Nasuti C, Fiorini D, Boarelli MC, Rossi G, Eleuteri AM. Microbiota modulation counteracts Alzheimer’s disease progression influencing neuronal proteolysis and gut hormones plasma levels. Sci Rep. 2017;7(1):2426. PMID: 28546539. doi: 10.1038/s41598-017-02587-2.
- Bonfili L, Cecarini V, Cuccioloni M, Angeletti M, Berardi S, Scarpona S, Rossi G, Eleuteri AM. SLAB51 probiotic formulation activates SIRT1 pathway promoting antioxidant and neuroprotective effects in an AD mouse model. Mol Neurobiol. 2018;55(10):7987–8000. PMID: 29492848. doi: 10.1007/s12035-018-0973-4.
- Lee HJ, Hwang YH, Kim DH. Lactobacillus plantarum C29-Fermented soybean (DW2009) alleviates memory impairment in 5XFAD transgenic mice by regulating microglia activation and gut microbiota composition. Mol Nutr Food Res. 2018;62(20):e1800359. PMID: 30152045. doi: 10.1002/mnfr.201800359.
- Lee HJ, Lee KE, Kim JK, Kim DH. Suppression of gut dysbiosis by Bifidobacterium longum alleviates cognitive decline in 5XFAD transgenic and aged mice. Sci Rep. 2019;9(1):11814. PMID: 31413350. doi: 10.1038/s41598-019-48342-7.
- Bonfili L, Cecarini V, Gogoi O, Berardi S, Scarpona S, Angeletti M, Rossi G, Eleuteri AM. Gut microbiota manipulation through probiotics oral administration restores glucose homeostasis in a mouse model of Alzheimer’s disease. Neurobiol Aging. 2020;87:35–43. PMID: 31813629. doi:10.1016/j.neurobiolaging.2019.11.004.
- Cecarini V, Bonfili L, Gogoi O, Lawrence S, Venanzi FM, Azevedo V, Mancha-Agresti P, Drumond MM, Rossi G, Berardi S, et al. Neuroprotective effects of p62(SQSTM1)-engineered lactic acid bacteria in Alzheimer’s disease: a pre-clinical study. Aging (Albany NY). 2020;12(16):15995–16020. PMID: 32855357. doi:10.18632/aging.103900.
- Kaur H, Golovko S, Golovko MY, Singh S, Darland DC, Combs CK. Effects of probiotic supplementation on short chain fatty acids in the AppNL-G-F mouse model of Alzheimer’s disease. J Alzheimers Dis. 2020;76:1083–1102. PMID: 32623399. doi:10.3233/jad-200436.
- Kaur H, Nagamoto-Combs K, Golovko S, Golovko MY, Klug MG, Combs CK. Probiotics ameliorate intestinal pathophysiology in a mouse model of Alzheimer’s disease. Neurobiol Aging. 2020;92:114–134. PMID: 32417748. doi:10.1016/j.neurobiolaging.2020.04.009.
- Ou Z, Deng L, Lu Z, Wu F, Liu W, Huang D, Peng Y. Protective effects of Akkermansia muciniphila on cognitive deficits and amyloid pathology in a mouse model of Alzheimer’s disease. Nutr Diabetes. 2020;10(1):12. PMID: 32321934. doi: 10.1038/s41387-020-0115-8.
- Sun J, Xu J, Yang B, Chen K, Kong Y, Fang N, Gong T, Wang F, Ling Z, Liu J. Effect of Clostridium butyricum against microglia-mediated neuroinflammation in Alzheimer’s disease via regulating gut microbiota and metabolites butyrate. Molecular Nutrition Food Res. 2020;64(2):e1900636. PMID: 31835282. doi: 10.1002/mnfr.201900636.
- Wang F, Xu T, Zhang Y, Zheng T, He Y, He F, Jiang Y. Long-term combined administration of Bifidobacterium bifidum TMC3115 and Lactobacillus plantarum 45 alleviates spatial memory impairment and gut dysbiosis in APP/PS1 mice. FEMS Microbiol Lett. 2020;367. PMID: 32239209. doi:10.1093/femsle/fnaa048.
- Wang QJ, Shen YE, Wang X, Fu S, Zhang X, Zhang YN, Wang RT. Concomitant memantine and Lactobacillus plantarum treatment attenuates cognitive impairments in APP/PS1 mice. Aging (Albany NY). 2020;12(1):628–649. PMID: 31907339. doi: 10.18632/aging.102645.
- Wu Q, Li Q, Zhang X, Ntim M, Wu X, Li M, Wang L, Zhao J, Li S. Treatment with Bifidobacteria can suppress Aβ accumulation and neuroinflammation in APP/PS1 mice. PeerJ. 2020;8:e10262. PMID: 33194428. doi:10.7717/peerj.10262.
- Cao J, Amakye WK, Qi C, Liu X, Ma J, Ren J. Bifidobacterium lactis Probio-M8 regulates gut microbiota to alleviate Alzheimer’s disease in the APP/PS1 mouse model. Eur J Nutr. 2021;60(7):3757–3769. PMID: 33796919. doi: 10.1007/s00394-021-02543-x.
- Go J, Chang DH, Ryu YK, Park HY, Lee IB, Noh JR, Hwang DY, Kim BC, Kim KS, Lee CH. Human gut microbiota Agathobaculum butyriciproducens improves cognitive impairment in LPS-induced and APP/PS1 mouse models of Alzheimer’s disease. Nutr Res. 2021;86:96–108. PMID: 33551257. doi:10.1016/j.nutres.2020.12.010.
- Guilherme MDS, Nguyen VTT, Reinhardt C, Endres K. Impact of gut microbiome manipulation in 5xFAD mice on Alzheimer’s disease-like pathology. Microorganisms. 2021;9(4):815. PMID: 33924322. doi: 10.3390/microorganisms9040815.
- Huang HJ, Chen JL, Liao JF, Chen YH, Chieu MW, Ke YY, Hsu CC, Tsai YC, Hsieh-Li HM. Lactobacillus plantarum PS128 prevents cognitive dysfunction in Alzheimer’s disease mice by modulating propionic acid levels, glycogen synthase kinase 3 beta activity, and gliosis. BMC Complement Med Ther. 2021;21(1):259. PMID: 34627204. doi: 10.1186/s12906-021-03426-8.
- Kaur H, Nookala S, Singh S, Mukundan S, Nagamoto-Combs K, Combs CK. Sex-dependent effects of intestinal microbiome Manipulation in a mouse model of Alzheimer’s disease. Cells. 2021;10(9):2370. PMID: 34572019. doi: 10.3390/cells10092370.
- Kim H, Kim S, Park SJ, Park G, Shin H, Park MS, Kim J. Administration of Bifidobacterium bifidum BGN4 and Bifidobacterium longum BORI improves cognitive and memory function in the mouse model of Alzheimer’s disease. Front Aging Neurosci. 2021;13:709091. PMID: 34421576. doi:10.3389/fnagi.2021.709091.
- Sun M, Bao W, Huang C, Xia Z, Zhang C, Wang G, Wang R, Li J, Roux S, Li Q, et al. A novel probiotic Formula, BIOCG, Protects against Alzheimer’s-related cognitive deficits via regulation of dendritic spine dynamics. Curr Alzheimer Res. 2021;18:558–572. PMID: 34674621. doi:10.2174/1567205018666211022091110.
- Abdelhamid M, Zhou C, Ohno K, Kuhara T, Taslima F, Abdullah M, Jung CG, Michikawa M. Probiotic Bifidobacterium breve prevents memory impairment through the Reduction of both amyloid-β production and microglia activation in APP Knock-in mouse. J Alzheimers Dis. 2022;85:1555–1571. PMID: 34958017. doi:10.3233/jad-215025.
- Bonfili L, Cuccioloni M, Gong C, Cecarini V, Spina M, Zheng Y, Angeletti M, Eleuteri AM. Gut microbiota modulation in Alzheimer’s disease: Focus on lipid metabolism. Clin Nutr. 2022;41:698–708. PMID: 35158177. doi:10.1016/j.clnu.2022.01.025.
- Ohno K, Abdelhamid M, Zhou C, Jung CG, Michikawa M. Bifidobacterium breve MCC1274 supplementation Increased the plasma levels of metabolites with potential anti-oxidative activity in APP Knock-in mice. J Alzheimers Dis. 2022;89(4):1413–1425. PMID: 36057824. doi: 10.3233/jad-220479.
- Webberley TS, Masetti G, Bevan RJ, Kerry-Smith J, Jack AA, Michael DR, Thomas S, Glymenaki M, Li J, McDonald JAK, et al. The impact of probiotic supplementation on cognitive, Pathological and metabolic markers in a transgenic mouse model of Alzheimer’s disease. Front Neurosci. 2022;16:843105. PMID: 35685773. doi:10.3389/fnins.2022.843105.
- Ye T, Yuan S, Kong Y, Yang H, Wei H, Zhang Y, Jin H, Yu Q, Liu J, Chen S, et al. Effect of probiotic fungi against cognitive impairment in mice via regulation of the fungal microbiota–gut–brain axis. J Agric Food Chem. 2022;70(29):9026–9038. PMID: 35833673. doi:10.1021/acs.jafc.2c03142.
- Webberley TS, Bevan RJ, Kerry-Smith J, Dally J, Michael DR, Thomas S, Rees M, Morgan JE, Marchesi JR, Good MA, et al. Assessment of Lab4P probiotic effects on cognition in 3xTg-AD Alzheimer’s disease model mice and the SH-SY5Y neuronal cell line. Int J Mol Sci. 2023;24(5):4683. PMID: 36902113. doi:10.3390/ijms24054683.
- Zhu G, Zhao J, Wang G, Chen W. Bifidobacterium breve HNXY26M4 attenuates cognitive deficits and neuroinflammation by regulating the gut–brain axis in APP/PS1 mice. J Agric Food Chem. 2023;71(11):4646–4655. PMID: 36888896. doi: 10.1021/acs.jafc.3c00652.
- Liu Y, Sheng Y, Pan Q, Xue Y, Yu L, Tian F, Zhao J, Zhang H, Zhai Q, Chen W. Identification of the key physiological characteristics of Lactobacillus plantarum strains for ulcerative colitis alleviation. Food Funct. 2020;11(2):1279–1291. PMID: 31984399. doi: 10.1039/c9fo02935d.
- Beck LC, Masi AC, Young GR, Vatanen T, Lamb CA, Smith R, Coxhead J, Butler A, Marsland BJ, Embleton ND, et al. Strain-specific impacts of probiotics are a significant driver of gut microbiome development in very preterm infants. Nature Microbiology. 2022;7(10):1525–1535. PMID: 36163498. doi:10.1038/s41564-022-01213-w.
- Akbari E, Asemi Z, Daneshvar Kakhaki R, Bahmani F, Kouchaki E, Tamtaji OR, Hamidi GA, Salami M. Effect of probiotic supplementation on cognitive function and metabolic status in Alzheimer’s disease: A randomized, double-blind and controlled trial. Front Aging Neurosci. 2016;8:256. PMID: 27891089. doi:10.3389/fnagi.2016.00256.
- Agahi A, Hamidi GA, Daneshvar R, Hamdieh M, Soheili M, Alinaghipour A, Esmaeili Taba SM, Salami M. Does severity of Alzheimer’s disease contribute to its responsiveness to modifying gut microbiota? A double blind clinical trial. Front Neurol. 2018;9:662. PMID: 30158897. doi:10.3389/fneur.2018.00662.
- Leblhuber F, Steiner K, Schuetz B, Fuchs D, Gostner JM. Probiotic supplementation in patients with Alzheimer’s dementia - an Explorative intervention study. Curr Alzheimer Res. 2018;15:1106–1113. PMID: 30101706. doi:10.2174/1389200219666180813144834.
- Hwang YH, Park S, Paik JW, Chae SW, Kim DH, Jeong DG, Ha E, Kim M, Hong G, Park SH, et al. Efficacy and safety of Lactobacillus plantarum C29-Fermented soybean (DW2009) in individuals with Mild cognitive impairment: A 12-week, multi-center, randomized, double-blind, placebo-controlled clinical trial. Nutrients. 2019;11(2):305. PMID: 30717153. doi:10.3390/nu11020305.
- Kobayashi Y, Kinoshita T, Matsumoto A, Yoshino K, Saito I, Xiao JZ. Bifidobacterium Breve A1 supplementation Improved cognitive decline in older adults with Mild cognitive impairment: An Open-label, single-arm study. J Prev Alzheimers Dis. 2019;6:70–75. PMID: 30569089. doi:10.14283/jpad.2018.32.
- Kobayashi Y, Kuhara T, Oki M, Xiao JZ. Effects of Bifidobacterium breve A1 on the cognitive function of older adults with memory complaints: a randomised, double-blind, placebo-controlled trial. Benef Microbes. 2019;10(5):511–520. PMID: 31090457. doi: 10.3920/bm2018.0170.
- Tamtaji OR, Heidari-Soureshjani R, Mirhosseini N, Kouchaki E, Bahmani F, Aghadavod E, Tajabadi-Ebrahimi M, Asemi Z. Probiotic and selenium co-supplementation, and the effects on clinical, metabolic and genetic status in Alzheimer’s disease: A randomized, double-blind, controlled trial. Clin Nutr. 2019;38:2569–2575. PMID: 30642737. doi:10.1016/j.clnu.2018.11.034.
- Xiao J, Katsumata N, Bernier F, Ohno K, Yamauchi Y, Odamaki T, Yoshikawa K, Ito K, Kaneko T. Probiotic Bifidobacterium breve in improving cognitive functions of older adults with Suspected Mild cognitive impairment: A randomized, double-blind, placebo-controlled trial. J Alzheimers Dis. 2020;77:139–147. PMID: 32623402. doi:10.3233/jad-200488.
- Bernier F, Ohno K, Katsumata N, Shimizu T, Xiao J. Association of plasma hemoglobin A1c with improvement of cognitive functions by probiotic Bifidobacterium breve supplementation in healthy adults with Mild cognitive impairment. J Alzheimers Dis. 2021;81:493–497. PMID: 33814441. doi:10.3233/jad-201488.
- Akhgarjand C, Vahabi Z, Shab-Bidar S, Etesam F, Djafarian K. Effects of probiotic supplements on cognition, anxiety, and physical activity in subjects with mild and moderate Alzheimer’s disease: A randomized, double-blind, and placebo-controlled study. Front Aging Neurosci. 2022;14:1032494. PMID: 36389063. doi:10.3389/fnagi.2022.1032494.
- Asaoka D, Xiao J, Takeda T, Yanagisawa N, Yamazaki T, Matsubara Y, Sugiyama H, Endo N, Higa M, Kasanuki K, et al. Effect of probiotic Bifidobacterium breve in improving cognitive function and preventing brain atrophy in older patients with Suspected Mild cognitive impairment: Results of a 24-week randomized, double-blind, placebo-controlled trial. J Alzheimers Dis. 2022;88:75–95. PMID: 35570493. doi:10.3233/jad-220148.
- Long JM, Holtzman DM. Alzheimer disease: An update on pathobiology and treatment strategies. Cell. 2019;179(2):312–339. PMID: 31564456. doi: 10.1016/j.cell.2019.09.001.
- Kalmijn S, Launer LJ, Ott A, Witteman JC, Hofman A, Breteler MM. Dietary fat intake and the risk of incident dementia in the Rotterdam study. Ann Neurol. 1997;42(5):776–782. PMID: 9392577. doi: 10.1002/ana.410420514.
- Calon F, Lim GP, Yang F, Morihara T, Teter B, Ubeda O, Rostaing P, Triller A, Salem N Jr., Ashe KH, et al. Docosahexaenoic acid protects from dendritic pathology in an Alzheimer’s disease mouse model. Neuron. 2004;43:633–645. PMID: 15339646. doi:10.1016/j.neuron.2004.08.013.
- Calon F, Lim GP, Morihara T, Yang F, Ubeda O, Salem N Jr., Frautschy SA, Cole GM. Dietary n-3 polyunsaturated fatty acid depletion activates caspases and decreases NMDA receptors in the brain of a transgenic mouse model of Alzheimer’s disease. Eur J Of Neuroscience. 2005;22(3):617–626. PMID: 16101743. doi: 10.1111/j.1460-9568.2005.04253.x.
- Lim GP, Calon F, Morihara T, Yang F, Teter B, Ubeda O, Salem N Jr., Frautschy SA, Cole GM. A diet enriched with the omega-3 fatty acid docosahexaenoic acid reduces amyloid burden in an aged Alzheimer mouse model. J Neurosci. 2005;25(12):3032–3040. PMID: 15788759. doi: 10.1523/jneurosci.4225-04.2005.
- Oksman M, Iivonen H, Hogyes E, Amtul Z, Penke B, Leenders I, Broersen L, Lütjohann D, Hartmann T, Tanila H. Impact of different saturated fatty acid, polyunsaturated fatty acid and cholesterol containing diets on beta-amyloid accumulation in APP/PS1 transgenic mice. Neurobiol Dis. 2006;23:563–572. PMID: 16765602. doi:10.1016/j.nbd.2006.04.013.
- Arendash GW, Jensen MT, Salem N Jr., Hussein N, Cracchiolo J, Dickson A, Leighty R, Potter H. A diet high in omega-3 fatty acids does not improve or protect cognitive performance in Alzheimer’s transgenic mice. Neuroscience. 2007;149(2):286–302. PMID: 17904756. doi: 10.1016/j.neuroscience.2007.08.018.
- Green KN, Martinez-Coria H, Khashwji H, Hall EB, Yurko-Mauro KA, Ellis L, LaFerla FM. Dietary docosahexaenoic acid and docosapentaenoic acid ameliorate amyloid-β and tau pathology via a mechanism involving presenilin 1 levels. J Neurosci. 2007;27(16):4385–4395. PMID: 17442823. doi: 10.1523/jneurosci.0055-07.2007.
- Hooijmans CR, Rutters F, Dederen PJ, Gambarota G, Veltien A, van Groen T, Broersen LM, Lütjohann D, Heerschap A, Tanila H, et al. Changes in cerebral blood volume and amyloid pathology in aged Alzheimer APP/PS1 mice on a docosahexaenoic acid (DHA) diet or cholesterol enriched Typical Western diet (TWD). Neurobiol Dis. 2007;28:16–29. PMID: 17720508. doi:10.1016/j.nbd.2007.06.007.
- Ma QL, Teter B, Ubeda OJ, Morihara T, Dhoot D, Nyby MD, Tuck ML, Frautschy SA, Cole GM. Omega-3 fatty acid docosahexaenoic acid increases SorLA/LR11, a sorting protein with reduced expression in sporadic Alzheimer’s disease (AD): relevance to AD prevention. J Neurosci. 2007;27(52):14299–14307. PMID: 18160637. doi: 10.1523/jneurosci.3593-07.2007.
- Hooijmans CR, der Zee CE V, Dederen PJ, Brouwer KM, Reijmer YD, van Groen T, Broersen LM, Lütjohann D, Heerschap A, Kiliaan AJ. DHA and cholesterol containing diets influence Alzheimer-like pathology, cognition and cerebral vasculature in APPswe/PS1dE9 mice. Neurobiol Dis. 2009;33(3):482–498. PMID: 19130883. doi: 10.1016/j.nbd.2008.12.002.
- Perez SE, Berg BM, Moore KA, He B, Counts SE, Fritz JJ, Hu YS, Lazarov O, Lah JJ, Mufson EJ. DHA diet reduces AD pathology in young APPswe/PS1 Delta E9 transgenic mice: possible gender effects. J Neurosci Res. 2010;88:1026–1040. PMID: 19859965. doi:10.1002/jnr.22266.
- Arsenault D, Julien C, Tremblay C, Calon F. DHA improves cognition and prevents dysfunction of entorhinal cortex neurons in 3xTg-AD mice. PloS One. 2011;6(2):e17397. PMID: 21383850. doi: 10.1371/journal.pone.0017397.
- Fiol-DeRoque MA, Gutierrez-Lanza R, Terés S, Torres M, Barceló P, Rial RV, Verkhratsky A, Escribá PV, Busquets X, Rodríguez JJ. Cognitive recovery and restoration of cell proliferation in the dentate gyrus in the 5XFAD transgenic mice model of Alzheimer’s disease following 2-hydroxy-DHA treatment. Biogerontology. 2013;14(6):763–775. PMID: 24114505. doi: 10.1007/s10522-013-9461-4.
- Ma QL, Zuo X, Yang F, Ubeda OJ, Gant DJ, Alaverdyan M, Kiosea NC, Nazari S, Chen PP, Nothias F, et al. Loss of MAP function leads to hippocampal synapse loss and deficits in the Morris water maze with aging. J Neurosci. 2014;34(21):7124–7136. PMID: 24849348. doi:10.1523/jneurosci.3439-13.2014.
- Torres M, Price SL, Fiol-Deroque MA, Marcilla-Etxenike A, Ahyayauch H, Barceló-Coblijn G, Terés S, Katsouri L, Ordinas M, López DJ, et al. Membrane lipid modifications and therapeutic effects mediated by hydroxydocosahexaenoic acid on Alzheimer’s disease. Biochimica et Biophysica Acta (BBA) - Biomembranes. 2014;1838(6):1680–1692. PMID: 24374316. doi:10.1016/j.bbamem.2013.12.016.
- Hosono T, Mouri A, Nishitsuji K, Jung CG, Kontani M, Tokuda H, Kawashima H, Shibata H, Suzuki T, Nabehsima T, et al. Arachidonic or docosahexaenoic acid diet prevents memory impairment in Tg2576 mice. J Alzheimers Dis. 2015;48:149–162. PMID: 26401936. doi:10.3233/jad-150341.
- Teng E, Taylor K, Bilousova T, Weiland D, Pham T, Zuo X, Yang F, Chen PP, Glabe CG, Takacs A, et al. Dietary DHA supplementation in an APP/PS1 transgenic rat model of AD reduces behavioral and Aβ pathology and modulates Aβ oligomerization. Neurobiol Dis. 2015;82:552–560. PMID: 26369878. doi:10.1016/j.nbd.2015.09.002.
- Raefsky SM, Furman R, Milne G, Pollock E, Axelsen P, Mattson MP, Shchepinov MS. Deuterated polyunsaturated fatty acids reduce brain lipid peroxidation and hippocampal amyloid beta-peptide levels, without discernable behavioral effects in an APP/PS1 mutant transgenic mouse model of Alzheimer’s disease. Neurobiol Aging. 2018;66:165–176. PMID: 29579687. doi:10.1016/j.neurobiolaging.2018.02.024.
- Hoffman JD, Yanckello LM, Chlipala G, Hammond TC, McCulloch SD, Parikh I, Sun S, Morganti JM, Green SJ, Lin AL. Dietary inulin alters the gut microbiome, enhances systemic metabolism and reduces neuroinflammation in an APOE4 mouse model. PloS One. 2019;14(8):e0221828. PMID: 31461505. doi: 10.1371/journal.pone.0221828.
- Sun J, Liu S, Ling Z, Wang F, Ling Y, Gong T, Fang N, Ye S, Si J, Liu J. Fructooligosaccharides Ameliorating cognitive deficits and neurodegeneration in APP/PS1 transgenic mice through modulating gut microbiota. J Agric Food Chem. 2019;67(10):3006–3017. PMID: 30816709. doi: 10.1021/acs.jafc.8b07313.
- Chang Y-H, Hoffman J, Yanckello L, McCulloch S, Lin P, Lane A, Chlipala G, Green S, Lin A-L. Apolipoprotein E Genotype-dependent nutrigenetic effects to prebiotic inulin for reducing risk for Alzheimer’s disease in a mouse model. Curr Dev Nutr. 2020;4:1197–1197. doi:10.1093/cdn/nzaa057_013.
- Han D, Li Z, Liu T, Yang N, Li Y, He J, Qian M, Kuang Z, Zhang W, Ni C, et al. Prebiotics regulation of intestinal microbiota attenuates cognitive dysfunction induced by surgery stimulation in APP/PS1 mice. Aging Dis. 2020;11:1029–1045. PMID: 33014520. doi:10.14336/ad.2020.0106.
- Park YH, Shin SJ, Kim HS, Hong SB, Kim S, Nam Y, Kim JJ, Lim K, Kim JS, Kim JI, et al. Omega-3 fatty acid-type docosahexaenoic acid protects against Aβ-mediated mitochondrial deficits and pathomechanisms in Alzheimer’s disease-related animal model. Int J Mol Sci. 2020;21(11):3879. PMID: 32486013. doi:10.3390/ijms21113879.
- Wu S, Zhang J, Jiang C, Wang S, Que R, An L. Up-regulation of neprilysin mediates the protection of fructo-oligosaccharides against Alzheimer’s disease. Food Funct. 2020;11(7):6565–6572. PMID: 32644062. doi: 10.1039/d0fo00161a.
- Bie N, Li J, Li C, Lian R, Qin L, Wang C. Protective effect and mechanism of docosahexaenoic acid on the cognitive function in female APP/PS1 mice. Food Funct. 2021;12(22):11435–11448. PMID: 34676845. doi: 10.1039/d1fo01922h.
- Liu Q, Xi Y, Wang Q, Liu J, Li P, Meng X, Liu K, Chen W, Liu X, Liu Z. Mannan oligosaccharide attenuates cognitive and behavioral disorders in the 5xFAD Alzheimer’s disease mouse model via regulating the gut microbiota-brain axis. Brain Behav Immun. 2021;95:330–343. PMID: 33839232. doi:10.1016/j.bbi.2021.04.005.
- Badesso S, Cartas-Cejudo P, Espelosin M, Santamaria E, Cuadrado-Tejedor M, Garcia-Osta A. Docosahexaenoic acid ameliorates contextual fear memory deficits in the Tg2576 Alzheimer’s disease mouse model: Cellular and molecular correlates. Pharmaceutics. 2022;15(1):82. PMID: 36678710. doi: 10.3390/pharmaceutics15010082.
- Xiao M, Xiang W, Chen Y, Peng N, Du X, Lu S, Zuo Y, Li B, Hu Y, Li X. DHA ameliorates cognitive ability, reduces amyloid deposition, and nerve fiber production in Alzheimer’s disease. Front Nutr. 2022;9:852433. PMID: 35782939. doi:10.3389/fnut.2022.852433.
- Zussy C, John R, Urgin T, Otaegui L, Vigor C, Acar N, Canet G, Vitalis M, Morin F, Planel E, et al. Intranasal administration of Nanovectorized docosahexaenoic acid (DHA) improves cognitive function in two complementary mouse models of Alzheimer’s disease. Antioxid (Basel). 2022;11(5):838. PMID: 35624701. doi:10.3390/antiox11050838.
- Freund-Levi Y, Eriksdotter-Jönhagen M, Cederholm T, Basun H, Faxén-Irving G, Garlind A, Vedin I, Vessby B, Wahlund LO, Palmblad J. ω-3 fatty acid treatment in 174 patients with Mild to moderate Alzheimer disease: OmegAD study. Arch Neurol. 2006;63(10):1402–1408. PMID: 17030655. doi: 10.1001/archneur.63.10.1402.
- Kotani S, Sakaguchi E, Warashina S, Matsukawa N, Ishikura Y, Kiso Y, Sakakibara M, Yoshimoto T, Guo J, Yamashima T. Dietary supplementation of arachidonic and docosahexaenoic acids improves cognitive dysfunction. Neurosci Res (N Y). 2006;56:159–164. PMID: 16905216. doi:10.1016/j.neures.2006.06.010.
- Chiu CC, Su KP, Cheng TC, Liu HC, Chang CJ, Dewey ME, Stewart R, Huang SY. The effects of omega-3 fatty acids monotherapy in Alzheimer’s disease and mild cognitive impairment: a preliminary randomized double-blind placebo-controlled study. Progress In Neuro-Psychopharmacology And Biological Psychiatry. 2008;32(6):1538–1544. PMID: 18573585. doi: 10.1016/j.pnpbp.2008.05.015.
- Freund-Levi Y, Basun H, Cederholm T, Faxén-Irving G, Garlind A, Grut M, Vedin I, Palmblad J, Wahlund LO, Eriksdotter-Jönhagen M. Omega-3 supplementation in mild to moderate Alzheimer’s disease: effects on neuropsychiatric symptoms. Int J Geriat Psychiatry. 2008;23(2):161–169. PMID: 17582225. doi: 10.1002/gps.1857.
- Vedin I, Cederholm T, Freund Levi Y, Basun H, Garlind A, Faxén Irving G, Jönhagen ME, Vessby B, Wahlund LO, Palmblad J. Effects of docosahexaenoic acid–rich n−3 fatty acid supplementation on cytokine release from blood mononuclear leukocytes: the OmegAD study. Am J Clin Nutr. 2008;87(6):1616–1622. PMID: 18541548. doi: 10.1093/ajcn/87.6.1616.
- Freund-Levi Y, Hjorth E, Lindberg C, Cederholm T, Faxen-Irving G, Vedin I, Palmblad J, Wahlund LO, Schultzberg M, Basun H, et al. Effects of omega-3 fatty acids on inflammatory markers in cerebrospinal fluid and plasma in Alzheimer’s disease: the OmegAD study. Dement Geriatr Cogn Disord. 2009;27(5):481–490. PMID: 19439966. doi:10.1159/000218081.
- Irving GF, Freund-Levi Y, Eriksdotter-Jönhagen M, Basun H, Brismar K, Hjorth E, Palmblad J, Vessby B, Vedin I, Wahlund LO, et al. Omega-3 fatty acid supplementation effects on weight and appetite in patients with Alzheimer’s disease: the omega-3 Alzheimer’s disease study. J American Geriatrics Society. 2009;57(1):11–17. PMID: 19054188. doi:10.1111/j.1532-5415.2008.02055.x.
- Quinn JF, Raman R, Thomas RG, Yurko-Mauro K, Nelson EB, Van Dyck C, Galvin JE, Emond J, Jack CR Jr., Weiner M, et al. Docosahexaenoic acid supplementation and cognitive decline in Alzheimer disease: a randomized trial. Jama. 2010;304(17):1903–1911. PMID: 21045096. doi:10.1001/jama.2010.1510.
- Vedin I, Cederholm T, Freund-Levi Y, Basun H, Hjorth E, Irving GF, Eriksdotter-Jönhagen M, Schultzberg M, Wahlund LO, Palmblad J. Reduced prostaglandin F2α release from blood mononuclear leukocytes after oral supplementation of ω3 fatty acids: the OmegAD study. J Lipid Res. 2010;51(5):1179–1185. PMID: 19965584. doi: 10.1194/jlr.M002667.
- Sinn N, Milte CM, Street SJ, Buckley JD, Coates AM, Petkov J, Howe PR. Effects of n-3 fatty acids, EPA v. DHA, on depressive symptoms, quality of life, memory and executive function in older adults with mild cognitive impairment: a 6-month randomised controlled trial. Br J Nutr. 2012;107(11):1682–1693. PMID: 21929835. doi: 10.1017/s0007114511004788.
- Vedin I, Cederholm T, Freund-Levi Y, Basun H, Garlind A, Irving GF, Eriksdotter-Jönhagen M, Wahlund LO, Dahlman I, Palmblad J, et al. Effects of DHA-rich n-3 fatty acid supplementation on gene expression in blood mononuclear leukocytes: the OmegAD study. PloS One. 2012;7(4):e35425. PMID: 22545106. doi: 10.1371/journal.pone.0035425.
- Faxén-Irving G, Freund-Levi Y, Eriksdotter-Jönhagen M, Basun H, Hjorth E, Palmblad J, Vedin I, Cederholm T, Wahlund LO. Effects on transthyretin in plasma and cerebrospinal fluid by DHA-Rich n – 3 fatty acid supplementation in patients with Alzheimer’s disease: The OmegAD study. J Alzheimers Dis. 2013;36(1):1–6. PMID: 23563245. doi: 10.3233/jad-121828.
- Lee LK, Shahar S, Chin AV, Yusoff NA. Docosahexaenoic acid-concentrated fish oil supplementation in subjects with mild cognitive impairment (MCI): a 12-month randomised, double-blind, placebo-controlled trial. Psychopharmacol (Berl). 2013;225(3):605–612. PMID: 22932777. doi: 10.1007/s00213-012-2848-0.
- Freund Levi Y, Vedin I, Cederholm T, Basun H, Faxén Irving G, Eriksdotter M, Hjorth E, Schultzberg M, Vessby B, Wahlund LO, et al. Transfer of omega-3 fatty acids across the blood–brain barrier after dietary supplementation with a docosahexaenoic acid-rich omega-3 fatty acid preparation in patients with a lzheimer’s disease: the O meg AD study. J Intern Med. 2014;275(4):428–436. PMID: 24410954. doi:10.1111/joim.12166.
- Freund-Levi Y, Vedin I, Hjorth E, Basun H, Faxén Irving G, Schultzberg M, Eriksdotter M, Palmblad J, Vessby B, Wahlund LO, et al. Effects of supplementation with omega-3 fatty acids on oxidative stress and inflammation in patients with Alzheimer’s disease: the OmegAD study. JAD. 2014;42(3):823–831. PMID: 24934544. doi:10.3233/jad-132042.
- Mahmoudi MJ, Hedayat M, Sharifi F, Mirarefin M, Nazari N, Mehrdad N, Ghaderpanahi M, Tajalizadekhoob Y, Badamchizade Z, Larijani B, et al. Effect of low dose ω-3 poly unsaturated fatty acids on cognitive status among older people: a double-blind randomized placebo-controlled study. J Diabetes Metab Disord. 2014;13(1):34. PMID: 24507770. doi:10.1186/2251-6581-13-34.
- Shinto L, Quinn J, Montine T, Dodge HH, Woodward W, Baldauf-Wagner S, Waichunas D, Bumgarner L, Bourdette D, Silbert L, et al. A randomized placebo-controlled pilot trial of omega-3 fatty acids and alpha lipoic acid in Alzheimer’s disease. JAD. 2014;38(1):111–120. PMID: 24077434. doi:10.3233/jad-130722.
- Eriksdotter M, Vedin I, Falahati F, Freund-Levi Y, Hjorth E, Faxen-Irving G, Wahlund LO, Schultzberg M, Basun H, Cederholm T, et al. Plasma fatty acid profiles in relation to cognition and gender in Alzheimer’s disease patients during Oral Omega-3 fatty acid supplementation: The OmegAD study. J Alzheimers Dis. 2015;48:805–812. PMID: 26402079. doi:10.3233/jad-150102.
- Fiala M, Halder RC, Sagong B, Ross O, Sayre J, Porter V, Bredesen DE. ω-3 supplementation increases amyloid-β phagocytosis and resolvin D1 in patients with minor cognitive impairment. FASEB J. 2015;29(7):2681–2689. PMID: 25805829. doi: 10.1096/fj.14-264218.
- Phillips MA, Childs CE, Calder PC, Rogers PJ. No effect of Omega-3 fatty acid supplementation on cognition and mood in individuals with cognitive impairment and probable Alzheimer’s disease: A Randomised controlled trial. Int J Mol Sci. 2015;16:24600–24613. PMID: 26501267. doi:10.3390/ijms161024600.
- Wang X, Hjorth E, Vedin I, Eriksdotter M, Freund-Levi Y, Wahlund LO, Cederholm T, Palmblad J, Schultzberg M. Effects of n-3 FA supplementation on the release of proresolving lipid mediators by blood mononuclear cells: the OmegAD study. J Lipid Res. 2015;56:674–681. PMID: 25616438. doi:10.1194/jlr.P055418.
- Karimi M, Vedin I, Freund Levi Y, Basun H, Faxén Irving G, Eriksdotter M, Wahlund LO, Schultzberg M, Hjorth E, Cederholm T, et al. DHA-rich n–3 fatty acid supplementation decreases DNA methylation in blood leukocytes: the OmegAD study. Am J Clin Nutr. 2017;106(4):1157–1165. PMID: 28855224. doi:10.3945/ajcn.117.155648.
- Zhang YP, Miao R, Li Q, Wu T, Ma F. Effects of DHA supplementation on hippocampal volume and cognitive function in older adults with Mild cognitive impairment: A 12-month randomized, double-blind, placebo-controlled trial. J Alzheimers Dis. 2017;55(2):497–507. PMID: 27716665. doi: 10.3233/jad-160439.
- Schwarz C, Wirth M, Gerischer L, Grittner U, Witte AV, Köbe T, Flöel A. Effects of Omega-3 fatty acids on resting cerebral perfusion in patients with Mild cognitive impairment: A randomized controlled trial. J Prev Alzheimers Dis. 2018;5:26–30. PMID: 29405229. doi:10.14283/jpad.2017.23.
- Zhang YP, Lou Y, Hu J, Miao R, Ma F. DHA supplementation improves cognitive function via enhancing Aβ-mediated autophagy in Chinese elderly with mild cognitive impairment: a randomised placebo-controlled trial. J Neurol Neurosurg Psychiatry. 2018;89(4):382–388. PMID: 29142143. doi: 10.1136/jnnp-2017-316176.
- Jernerén F, Cederholm T, Refsum H, Smith AD, Turner C, Palmblad J, Eriksdotter M, Hjorth E, Faxen-Irving G, Wahlund LO, et al. Homocysteine status modifies the treatment effect of Omega-3 fatty acids on cognition in a randomized clinical trial in Mild to moderate Alzheimer’s disease: The OmegAD study. J Alzheimers Dis. 2019;69(1):189–197. PMID: 30958356. doi:10.3233/jad-181148.
- Tofiq A, Zetterberg H, Blennow K, Basun H, Cederholm T, Eriksdotter M, Faxén-Irving G, Hjorth E, Jernerén F, Schultzberg M, et al. Effects of peroral Omega-3 fatty acid supplementation on cerebrospinal fluid biomarkers in patients with Alzheimer’s disease: A randomized controlled trial—the OmegAD study. J Alzheimers Dis. 2021;83(3):1291–1301. PMID: 34420949. doi:10.3233/jad-210007.
- Lin PY, Cheng C, Satyanarayanan SK, Chiu LT, Chien YC, Chuu CP, Lan TH, Su KP. Omega-3 fatty acids and blood-based biomarkers in Alzheimer’s disease and mild cognitive impairment: A randomized placebo-controlled trial. Brain Behav Immun. 2022;99:289–298. PMID: 34755655. doi:10.1016/j.bbi.2021.10.014.
- El Gaamouch F, Chen F, Ho L, Lin HY, Yuan C, Wong J, Wang J. Benefits of dietary polyphenols in Alzheimer’s disease. Front Aging Neurosci. 2022;14:1019942. PMID: 36583187. doi:10.3389/fnagi.2022.1019942.
- Huang TL, Zandi PP, Tucker KL, Fitzpatrick AL, Kuller LH, Fried LP, Burke GL, Carlson MC. Benefits of fatty fish on dementia risk are stronger for those without APOE ε4. Neurology. 2005;65(9):1409–1414. PMID: 16275829. doi: 10.1212/01.wnl.0000183148.34197.2e.
- Vandal M, Alata W, Tremblay C, Rioux-Perreault C, Salem N Jr., Calon F, Plourde M. Reduction in DHA transport to the brain of mice expressing human APOE4 compared to APOE2. J Neurochem. 2014;129:516–526. PMID: 24345162. doi:10.1111/jnc.12640.
- Deng SM, Chen CJ, Lin HL, Cheng IH. The beneficial effect of synbiotics consumption on Alzheimer’s disease mouse model via reducing local and systemic inflammation. Iubmb Life. 2022;74:748–753. PMID: 34962691. doi:10.1002/iub.2589.
- Westfall S, Lomis N, Prakash S. A novel synbiotic delays Alzheimer’s disease onset via combinatorial gut-brain-axis signaling in Drosophila melanogaster. PloS One. 2019;14:e0214985. PMID: 31009489. doi:10.1371/journal.pone.0214985.
- Abbasi A, Rad AH, Ghasempour Z, Sabahi S, Kafil HS, Hasannezhad P, Rahbar Saadat Y, Shahbazi N. The biological activities of postbiotics in gastrointestinal disorders. Crit Rev Food Sci Nutr. 2022;62(22):1–22. PMID: 33715539. doi: 10.1080/10408398.2021.1895061.
- Zhang T, Zhang W, Feng C, Kwok LY, He Q, Sun Z. Stronger gut microbiome modulatory effects by postbiotics than probiotics in a mouse colitis model. NPJ Sci Food. 2022;6(1):53. PMID: 36379940. doi: 10.1038/s41538-022-00169-9.
- Sabahi S, Homayouni Rad A, Aghebati-Maleki L, Sangtarash N, Ozma MA, Karimi A, Hosseini H, Abbasi A. Postbiotics as the new frontier in food and pharmaceutical research. Crit Rev Food Sci Nutr. 2022;1–28. PMID: 35348016. doi:10.1080/10408398.2022.2056727.
- Zhang F, Luo W, Shi Y, Fan Z, Ji G. Should we standardize the 1,700-year-old fecal microbiota transplantation? Am J Gastroenterol. 2012;107:1755. author reply 1755-1756 PMID: 23160295. doi:10.1038/ajg.2012.251.
- Eiseman B, Silen W, Bascom GS, Kauvar AJ. Fecal enema as an adjunct in the treatment of pseudomembranous enterocolitis. Surgery. 1958;44(5):854–859. PMID: 13592638.
- van Nood E, Vrieze A, Nieuwdorp M, Fuentes S, Zoetendal EG, de Vos WM, Visser CE, Kuijper EJ, Bartelsman JF, Tijssen JG, et al. Duodenal infusion of donor feces for recurrent Clostridium difficile. N Engl J Med. 2013;368:407–415. PMID: 23323867. doi:10.1056/NEJMoa1205037.
- Allegretti JR, Mullish BH, Kelly C, Fischer M. The evolution of the use of faecal microbiota transplantation and emerging therapeutic indications. Lancet. 2019;394(10196):420–431. PMID: 31379333. doi: 10.1016/s0140-6736(19)31266-8.
- WHO. https://www.fda.gov/news-events/press-announcements/fda-approves-first-fecal-microbiota-product. 2022.
- Harach T, Marungruang N, Duthilleul N, Cheatham V, Mc Coy KD, Frisoni G, Neher JJ, Fåk F, Jucker M, Lasser T, et al. Reduction of Abeta amyloid pathology in APPPS1 transgenic mice in the absence of gut microbiota. Sci Rep. 2017;7(1):41802. PMID: 28176819. doi:10.1038/srep41802.
- Sun J, Xu J, Ling Y, Wang F, Gong T, Yang C, Ye S, Ye K, Wei D, Song Z, et al. Fecal microbiota transplantation alleviated Alzheimer’s disease-like pathogenesis in APP/PS1 transgenic mice. Transl Psychiatry. 2019;9(1):189. PMID: 31383855. doi:10.1038/s41398-019-0525-3.
- Shen H, Guan Q, Zhang X, Yuan C, Tan Z, Zhai L, Hao Y, Gu Y, Han C. New mechanism of neuroinflammation in Alzheimer’s disease: The activation of NLRP3 inflammasome mediated by gut microbiota. Prog Neuropsychopharmacol Biol Psychiatry. 2020;100:109884. PMID: 32032696. doi:10.1016/j.pnpbp.2020.109884.
- Kim N, Jeon SH, Ju IG, Gee MS, Do J, Oh MS, Lee JK. Transplantation of gut microbiota derived from Alzheimer’s disease mouse model impairs memory function and neurogenesis in C57BL/6 mice. Brain Behav Immun. 2021;98:357–365. PMID: 34500036. doi:10.1016/j.bbi.2021.09.002.
- Valeri F, Dos Santos Guilherme M, He F, Stoye NM, Schwiertz A, Endres K. Impact of the age of cecal material transfer donors on Alzheimer’s disease pathology in 5xFAD mice. Microorganisms. 2021;9(12):2548. PMID: 34946148. doi: 10.3390/microorganisms9122548.
- Bi W, Cai S, Hang Z, Lei T, Wang D, Wang L, Du H. Transplantation of feces from mice with Alzheimer’s disease promoted lung cancer growth. Biochem Biophys Res Commun. 2022;600:67–74. PMID: 35196629. doi:10.1016/j.bbrc.2022.01.078.
- Elangovan S, Borody TJ, Holsinger RMD. Fecal microbiota transplantation reduces pathology and improves cognition in a mouse model of Alzheimer’s disease. Cells. 2022;12(1):119. PMID: 36611911. doi: 10.3390/cells12010119.
- Hang Z, Cai S, Lei T, Zhang X, Xiao Z, Wang D, Li Y, Bi W, Yang Y, Deng S, et al. Transfer of tumor-bearing mice intestinal flora can ameliorate cognition in Alzheimer’s disease mice. J Alzheimers Dis. 2022;86(3):1287–1300. PMID: 35180124. doi:10.3233/jad-215495.
- Kim JE, Roh YJ, Choi YJ, Lee SJ, Jin YJ, Song HJ, Seol AY, Son HJ, Hong JT, Hwang DY. Dysbiosis of fecal microbiota in Tg2576 mice for Alzheimer’s disease during pathological constipation. Int J Mol Sci. 2022;23(23):14928. PMID: 36499254. doi: 10.3390/ijms232314928.
- Soriano S, Curry K, Wang Q, Chow E, Treangen TJ, Villapol S. Fecal microbiota transplantation derived from Alzheimer’s disease mice worsens brain trauma outcomes in wild-type controls. Int J Mol Sci. 2022;23(9):4476. PMID: 35562867. doi: 10.3390/ijms23094476.
- Wang F, Gu Y, Xu C, Du K, Zhao C, Zhao Y, Liu X. Transplantation of fecal microbiota from APP/PS1 mice and Alzheimer’s disease patients enhanced endoplasmic reticulum stress in the cerebral cortex of wild-type mice. Front Aging Neurosci. 2022;14:858130. PMID: 35966768. doi:10.3389/fnagi.2022.858130.
- Fujii Y, Nguyen TTT, Fujimura Y, Kameya N, Nakamura S, Arakawa K, Morita H. Fecal metabolite of a gnotobiotic mouse transplanted with gut microbiota from a patient with Alzheimer’s disease. Biosci Biotechnol Biochem. 2019;83:2144–2152. PMID: 31327302. doi:10.1080/09168451.2019.1644149.
- Hazan S. Rapid improvement in Alzheimer’s disease symptoms following fecal microbiota transplantation: a case report. J Int Med Res. 2020;48(6):300060520925930. PMID: 32600151. doi: 10.1177/0300060520925930.
- Park SH, Lee JH, Shin J, Kim JS, Cha B, Lee S, Kwon KS, Shin YW, Choi SH. Cognitive function improvement after fecal microbiota transplantation in Alzheimer’s dementia patient: a case report. Curr Med Res Opin. 2021;37:1739–1744. PMID: 34289768. doi:10.1080/03007995.2021.1957807.
- Hou Q, Zhao F, Liu W, Lv R, Khine WWT, Han J, Sun Z, Lee YK, Zhang H. Probiotic-directed modulation of gut microbiota is basal microbiome dependent. Gut Microbes. 2020;12(1):1736974. PMID: 32200683. doi: 10.1080/19490976.2020.1736974.
- Smillie CS, Sauk J, Gevers D, Friedman J, Sung J, Youngster I, Hohmann EL, Staley C, Khoruts A, Sadowsky MJ, et al. Strain tracking reveals the determinants of bacterial engraftment in the human gut following fecal microbiota transplantation. Cell Host & Microbe. 2018;23(2):229–240 e225. PMID: 29447696. doi:10.1016/j.chom.2018.01.003.
- Zmora N, Suez J, Elinav E. You are what you eat: diet, health and the gut microbiota. Nat Rev Gastroenterol Hepatol. 2019;16(1):35–56. PMID: 30262901. doi: 10.1038/s41575-018-0061-2.
- Arora S, Santiago JA, Bernstein M, Potashkin JA. Diet and lifestyle impact the development and progression of Alzheimer’s dementia. Front Nutr. 2023;10:1213223. PMID: 37457976. doi:10.3389/fnut.2023.1213223.
- van den Brink AC, Brouwer-Brolsma EM, Berendsen AAM, van de Rest O. The Mediterranean, Dietary approaches to stop hypertension (DASH), and Mediterranean-DASH intervention for neurodegenerative delay (MIND) diets are associated with less cognitive decline and a lower risk of Alzheimer’s disease—A review. Adv Nutr. 2019;10(6):1040–1065. PMID: 31209456. doi: 10.1093/advances/nmz054.
- Wieckowska-Gacek A, Mietelska-Porowska A, Wydrych M, Wojda U. Western diet as a trigger of Alzheimer’s disease: From metabolic syndrome and systemic inflammation to neuroinflammation and neurodegeneration. Ageing Res Rev. 2021;70:101397. PMID: 34214643. doi:10.1016/j.arr.2021.101397.
- Dhana K, Franco OH, Ritz EM, Ford CN, Desai P, Krueger KR, Holland TM, Dhana A, Liu X, Aggarwal NT, et al. Healthy lifestyle and life expectancy with and without Alzheimer’s dementia: population based cohort study. BMJ. 2022;377:e068390. PMID: 35418416. doi:10.1136/bmj-2021-068390.
- Barnes LL, Dhana K, Liu X, Carey VJ, Ventrelle J, Johnson K, Hollings CS, Bishop L, Laranjo N, Stubbs BJ, et al. Trial of the MIND diet for prevention of cognitive decline in older persons. N Engl J Med. 2023;389(7):602–611. PMID: 37466280. doi:10.1056/NEJMoa2302368.
- Pellegrini C, Fornai M, D’Antongiovanni V, Antonioli L, Bernardini N, Derkinderen P. The intestinal barrier in disorders of the central nervous system. Lancet Gastroenterol Hepatol. 2023;8:66–80. PMID: 36334596. doi:10.1016/S2468-1253(22)00241-2.
- Carrasco E, de Las Heras MM G, Gabande-Rodriguez E, Desdin-Mico G, Aranda JF, Mittelbrunn M. The role of T cells in age-related diseases. Nat Rev Immunol. 2022;22(2):97–111. PMID: 34099898. doi: 10.1038/s41577-021-00557-4.