ABSTRACT
The global population is aging and age-related cardiovascular disease is increasing. Even after controlling for cardiovascular risk factors, readmission and mortality rates remain high. In recent years, more and more in-depth studies have found that the composition of the gut microbiota and its metabolites, such as trimethylamine N-oxide (TMAO), bile acids (BAs), and short-chain fatty acids (SCFAs), affect the occurrence and development of age-related cardiovascular diseases through a variety of molecular pathways, providing a new target for therapy. In this review, we discuss the relationship between the gut microbiota and age-related cardiovascular diseases, and propose that the gut microbiota could be a new therapeutic target for preventing and treating cardiovascular diseases.
Introduction
Morbidity and mortality rates from age-related cardiovascular diseases such as atherosclerosis, hypertension, and heart failure remain high worldwide. Despite known risk factors (such as smoking, blood lipids, and blood sugar) and the increasing availability of new targeted drugs, age-related cardiovascular disease continues to pose a threat to global health.
A healthy gut microbiota is essential for human health. The gut microbiota composition and metabolites will change with age, dietary habits, and other factors, and are affected by external factors such as supplementation with probiotics and the use of antibiotics. More recently, fecal microbiota transplantation (FMT), microbial enzyme inhibitors, gut microbiota metabolites, and probiotics supplements have been developed as new precision-medicine approaches to modulating the gut microbiota.Citation1,Citation2 Understanding the relationship between the gut microbiota and age-related cardiovascular disease is crucial for the prevention and treatment of cardiovascular disease, as well as the development of targeted therapies for cardiovascular disease. In this review, we will discuss the pathogenesis of age-related cardiovascular disease and the role of the gut microbiota, with the aim of highlighting new directions for the treatment of age-related cardiovascular disease in the future ().
Figure 1. Gut microbiota and cardiovascular disease. Various gut microbiota in the human body, such as Streptococcus, staphylococcus and Helicobacter pylori, can activate neutrophils in various ways to cause inflammatory reaction, thus causing excessive ROS accumulation in the body and damaging mitochondrial function; on the other hand, SCFAs produced by gut microbiota ingesting dietary components can also trigger autophagy through PI3K/Akt/mTOR pathway. The above mechanisms play a key role in age-related cardiovascular diseases such as atherosclerosis, hypertension, myocardial infarction, myocardial hypertrophy and fibrosis. (ROS: reactive oxygen species. SCFAs: short-chain fatty acids).
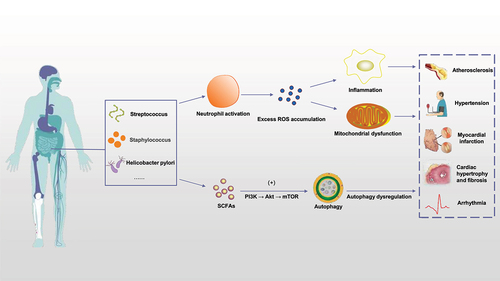
Potential mechanisms of cardiovascular aging
Cardiovascular system aging includes progressive dynamic changes in structure and function that can lead to impaired cardiovascular homeostasis, manifested by a reduction in cardiomyocyte numbers, hypertrophy of aging cardiomyocytes, an increased inflammatory response, progressive development of cardiac fibrosis, decreased elasticity, increased stiffness, and a series of clinical diseases.Citation3 Therefore, exploring the mechanism of cardiovascular aging could help prevent some cardiovascular diseases and improve or even reverse cardiovascular function.
Oxidative stress
Oxidative stress plays an important role in age-related cardiovascular disease. Increased reactive oxygen species (ROS) production or impaired antioxidant production are considered to be key factors in the occurrence and development of age-related cardiovascular diseases.Citation4,Citation5 Aging itself is a risk factor for cardiovascular diseases, and aging is related to oxidative stress, which can also lead to accelerated cell aging and organ dysfunction. Babcock et al. showed that endothelial dysfunction is accelerated by decreased testosterone levels, increased ROS accumulation, and weakened antioxidant defenses in middle-aged and older men, and that supplementation with the antioxidant vitamin C can mitigate these changes.Citation6
Some components of the gut microbiota themselves, or their metabolites, such as TMAO and SCFAs, can regulate exogenous and endogenous ROS levels. Lactobacillus can enhance the permeability of gastrointestinal mucosa or increase the secretion of intestinal mucus, thereby stimulating ROS production.Citation7 Helicobacter pylori activates NADPH oxidase to produce ROS and activates REDOX-sensitive signal transduction via NF-κB, activator protein-1 (AP-1), and mitogen activated protein kinase (MAPK) to induce the release of inflammatory cytokines.Citation8,Citation9 TMAO is a byproduct of gut microbiota metabolism of phosphatidylcholine, carnitine, betaine, and other nutrients.Citation10 Increased circulating TMAO levels caused by increased choline intake may inhibit AMPK and SIRT1 expression levels, increase ROS levels, and activate inflammatory responses.Citation11 Lipopolysaccharide (LPS) is a specific component of the cell wall of Gram-negative bacteria. When the intestinal barrier is compromised, LPS enters the bloodstream, leading to increased production of ROS by NADPH oxidase through activation of the Toll-like receptor 4 (TLR4)-mediated oxidative pathway and resulting in decreased endothelial NO bioavailability due to reduced levels of phosphorylated eNOS, resulting endothelial dysfunction.Citation12 A study performed in aging mice found that treatment with exogenous tetrahydrobiopterin increased endothelium-dependent vasodilation and reduced eNOS-derived superoxide formation in the mesenteric arteries, confirming the important role of eNOS decoupling in age-related endothelial dysfunction.Citation13
In addition to age-related endothelial dysfunction, oxidative stress also induces cardiomyocyte apoptosis and promotes the development of age-related cardiovascular disease. Loss of cardiomyocytes is a hallmark of heart aging, and can lead to replacement of myocardium and, ultimately, cardiac hypertrophy. ROS was found to induce cardiomyocyte apoptosis through the ERK1/2 MAPK, JNK, P38 MAPK, and CAMKII pathways.Citation14,Citation15 Enhanced phosphorylation of JNK and P38 MAPK can promote nuclear translocation of NF-κB, induce cell senescence, and aggravate myocardial damage, while decreased phosphorylation of JNK and P38 MAPK can protect the aging rat heart from oxidative stress.Citation16 Some animal experiments have also confirmed the role of oxidative stress in cardiomyocyte apoptosis. Mice with mitochondrial DNA (mtDNA) mutations exhibit an early aging phenotype. In mtDNA mutant mice, oxidative respiratory chains were inhibited, ATP production decreased, ROS accumulation increased, cardiomyocyte apoptosis increased, premature age-related changes in heart tissue were observed, cardiac hypertrophy decreased, and systolic and diastolic function were inhibited.Citation17 In conclusion, changes in gut microbiota promote oxidative stress and thus affect the pathogenesis of age-related cardiovascular diseases.
Mitochondrial dysfunction
Mitochondrial dysfunction is one of the most important mechanisms of age-related cardiovascular disease. In recent years, the relationship among intestinal flora, mitochondrial dysfunction, and age-related cardiovascular disease has attracted wide attention. Intestinal flora metabolites seem to directly affect mitochondrial oxidative stress and formation of the mitochondrial autophagic lysosome, thus regulating inflammatory body activation and inflammatory cytokine production, which are major components of myocardial cell metabolism disorders.Citation18 A high-fat diet can impair mitochondrial activity in the colonic epithelium and increase choline metabolism in Escherichia coli, thereby increasing circulating TMAO levels and inducing low-grade mucosal inflammation, which plays a key role in age-related cardiovascular disease, especially atherosclerosis.Citation19 The microbiota-derived metabolite trimethyl-5-aminovaleric acid was found to reduce fatty acid oxidation (FAO) and affect mitochondrial optic atrophy protein 1 (OPA1) cleavage and oligomerization, thereby severely impairing mitochondrial function and accelerating cardiomyocyte hypertrophy, resulting in functional impairment.Citation20 Damaged mitochondria not only produce less ATP, but also release more ROS and have a higher propensity to induce apoptosis, all features of age-related cardiovascular disease.Citation21 The mtDNA is located very close to the cellular site of ROS production and lacks protective histones. In addition, the mtDNA repair system is inefficient and prone to mutation.Citation22 Mice with mitochondrial mutations, namely early-aging mouse models expressing the sequencing-defective DNA polymerase gamma, exhibited cumulative mtDNA mutations, a significantly accelerated aging phenotype, and an increased risk of heart disease.Citation23,Citation24 Wang et al. showed that administration of spermidine (a major mammalian polyamine) to older rats preserved myocardial ultrastructure and reduced cardiac aging by activating mitochondrial biogenesis and inhibiting mitochondrial dysfunctionCitation25 ().
Figure 2. Mitochondrial dysfunction affects the underlying mechanisms of age-related cardiovascular disease. TMAO can promote mitochondrial DNA mutation, thus affecting OXPHOS, increasing ROS production, decreasing ATP/ADP ratio, further damaging mitochondrial DNA, and causing impaired calcium ion metabolism, intracellular calcium overload, and promoting cell apoptosis. Increased oxidative stress activates AMPK, which activates autophagy and degrades damaged mitochondria. (TMAO: trimethylamine N-oxide. OXPHOS: oxidative phosphorylation. AMPK: AMP-activated protein kinase).
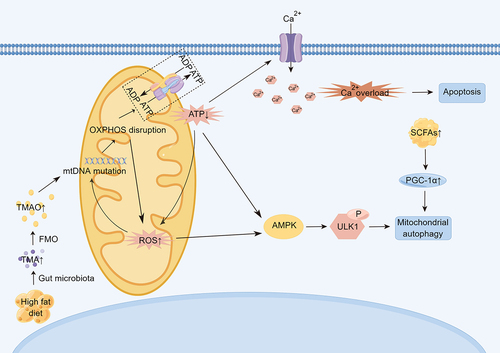
Mitochondrial autophagy, a mitochondrial quality control system, is also believed to be closely related to cardiovascular aging. Mitochondrial autophagy is a selective form of autophagy that degrades old or damaged mitochondria.Citation26 Several specific proteins play key roles in the molecular regulation of mitochondrial autophagy, including PTEN-induced kinase 1 (PINK1).Citation27 Overexpression of PINK1 has been shown to improve mitosis, thereby protecting vascular smooth muscle cells, while PINK1 deficiency leads to mitotic errors and reduced VSMC survival.Citation28 SCFAs produced by intestinal flora can act as signaling molecules to regulate autophagy. When PINK1 was overexpressed in rats treated with rifaximin (an unabsorbable antibiotic that can remodel the intestinal microbiome), the microbial communities producing acetate and propionate and circulating SCFAs levels increased, and PGC-1α expression was activated. PGC-1α acts downstream of SCFAs and is an upstream activator of mitochondrial autophagy and mitochondrial tumorigenesis.Citation29
The negative effects of mitochondrial dysfunction on age-related cardiovascular disease provide insight into the cardiovascular aging process and suggest new avenues for developing treatment strategies.
Inflammation
Inflammation is part of the body’s normal healing and repair response and is essential for protecting the body from bacterial and viral infections, as well as harmful environmental factors.Citation30 However, when complex, acute inflammatory responses are not fully resolved, more defense components are mobilized, resulting in a persistent immune response called chronic inflammation.Citation31 The gut flora may influence the development of age-related cardiovascular disease by participating in inflammation. An imbalance in the intestinal microecology increases the permeability of the intestinal mucosal barrier, allowing intestinal bacteria and their metabolites to enter the blood circulation, leading to chronic inflammation. Disruption of the intestinal microecology is frequently observed in diseases whose prevalence increases with age, such as obesity and type 2 diabetes.Citation32 LPS is thought to be a key factor in this process, as it has a variety of adverse effects on the intestinal tract. For example, it can disrupt intestinal tight junctions via specific signaling pathways, directly causing shedding of intestinal epithelial cells without reclosure of the decompensated tight junction, and may induce oxidative stress, mitochondrial autophagy, and mitochondrial failure in intestinal epithelial cells.Citation33,Citation34 In addition, it binds to toll-like receptor 4 (TLR-4) on immune cells to activate pro-inflammatory cascades in local and distant parts of the intestine.Citation35 Inflammatory factors such as IL-6, IL-1β, TNF-α, and more characterize the senescence-associated secretory phenotype (SASP).Citation36,Citation37 Activation of NF-κB, a key transcription factor involved in the inflammatory response, is thought to play a key role in age-related vascular inflammation, and increased NF-κB expression has been observed in vascular endothelial cells from elderly individuals.Citation38,Citation39 Csisza et al. found that NF-κB activation increases monocyte adhesion to endothelial cells in aging arteries, thus making arteries prone to atherosclerosis.Citation40 Age-dependent NF-κB activation is also associated with systemic inflammation and impairs endothelium-dependent vasodilation.Citation41 Therefore, NF-κB may be a therapeutic target for senile cardiovascular disease in the future. Therefore, it is speculated that TNF-α may play an important role in age-related cardiovascular disease through inflammation mediated endothelial dysfunction.
Autophagy
Autophagy plays a crucial role in the degradation of long-lived proteins and organelles, and is important for maintaining heart tissue homeostasis during aging. Autophagy appears to decrease with age, and studies in C. elegans, rodents, and human cells have shown an age-dependent decline in lysosomal proteolytic function, which impairs autophagy flux, exacerbates cell damage and leads to the development of age-related diseases.Citation42,Citation43 Altered gut microbiota diversity and weakened intestinal barrier integrity can induce abnormal leakage of LPS, and the LPS-activated TLR4 signaling pathway stimulates autophagy in muscle cells, suggesting that LPS can induce excessive autophagy.Citation44 In addition, the rate of colonic epithelium autophagy in germ-free mice is lower than that seen in conventionally-fed mice, suggesting that the gut microbiota affects intestinal autophagy under physiological conditions.Citation45 Bifidobacterium dentium was also found to significantly up-regulate the autophagy signaling pathway and mucin gene expression, enhance intestinal mucous layer and goblet cell function, and net increase mucin production.Citation46 This evidence suggests that the gut microbiota participates in autophagy-related processes. The autophagy-related protein Atg5 regulates autophagy and apoptosis. Eisenberg et al. found that mice lacking Atg5 had increased left ventricular hypertrophy and decreased diastolic function, leading to age-related cardiac deterioration.Citation47 Beclin-1 is a key factor in autophagosome formation. Hamacher-Brady et al. demonstrated that enhancing autophagy through overexpression of Beclin-1 can actually protect myocardial cells from ischemia-reperfusion injury, while decreasing Beclin-1 expression reduces rational cardiac remodeling.Citation48,Citation49 Bcl-2 family proteins can inhibit Beclin-1‘s ability to promote autophagy after binding with Beclin-1. Disruption of the Beclin1-Bcl2 complex has been found to increase autophagy, inhibit age-induced apoptosis, cardiac hypertrophy and fibrosis, and delay cardiac aging.Citation50 It is widely believed that autophagy levels decline with age, and in cardiomyocytes this decline leads to the accumulation of dysfunctional organelles and toxic proteins that mediate general cardiac dysfunction. However, others have suggested that it is not that autophagy levels decline, but rather that the level of autophagy activity cannot compete with increasing levels of ROS and oxidative damage.Citation51 In addition, decreased autophagy leads to protein degradation and misfolded or damaged protein processing imbalances, which are associated with aging and the occurrence and development of heart disease. Overactivation of autophagy can lead to the degradation of contractile proteins and to cardiomyocyte autophagy.Citation52 Therefore, dysregulation of autophagy plays a very important role in age-related cardiovascular diseases.
Gut microbiota and aging
Genetics and environment interact in complex ways to influence aging. The gut microbiome changes dramatically throughout an individual’s life. The composition and function of an infant’s early microbiota are mainly determined by delivery method and early feeding, followed by the maternal microbiota and antibiotic exposure.Citation53 Lactobacillus and Bifidobacterium predominate in breastfed infants, while Enterococcus, Bacteroides, Streptococcus, Clostridium and Enterobacter predominate in formula-fed infants.Citation54 In addition, Dominguez-Bello et al. showed that the skin surface bacteria in infants born by C-section mainly comprises Staphylococcus, Corynebacterium, and Propionibacterium; while babies delivered vaginally have the same skin bacterial community as their mothers, even though their mothers’ vaginal microbiomes largely consist of Lactobacillus, Prevotella, or Cilium.Citation55 The diversity of the human microbiome increases with age and plateaus in adulthood. Roswall et al. showed that the microbiota of infants at 4 and 12 months is highly heterogeneous and very different from that of adults, but that the microbiota of children at 3 and 5 years is more similar to that of adults.Citation56 As people age, their gut microbiota is influenced by a variety of factors, such as genetics, diet, mood, and life events. However, in general Bacteroides dominates the gut microbiota in children and decreases significantly with age, while Firmicutes increases.Citation57 Middle-aged adults and elderly individuals exhibit similar gut microbiota structure, i.e., Bacteroides and Firmicutes are dominant (about 95% of the microbiota), and Actinobacteria and Proteus are present in smaller proportions.Citation58 However, centenarians stand out as a separate population with a microbiome that displays distinctive traits and has low diversity in terms of species composition. Bacteroides and Firmicutes remain dominant in the gut microbiota of centenarians (accounting for more than 93% of the total bacteria), but a decreased proportion of Firmicutes and an increased proportion of Bacteroides were observed compared with younger adults, and the gut was rich in Proteus.Citation58,Citation59 This change that is thought to be likely to lead to a compromised immune system, which in turn is associated with more inflammationCitation58 ().
Figure 3. Gut microbiota and aging. The composition of intestinal flora and the level of derived metabolites changed with age. The composition and function of an infant’s early microbiota are influenced by factors such as birth mode and early feeding pattern, and the gut microbiota is influenced by more factors such as genetics, diet, mood, and life events as the infant ages. However, the general trend was that with the increase of age, the diversity of intestinal flora increased, the number of Bacteroides decreased significantly, and the number of Firmicutes increased, the level of TMAO increased, and the abundance of SCFAs producing bacteria decreased. However, due to the existence of higher inflammation levels in centenarians, the diversity of intestinal flora decreased, the proportion of Firmicutes decreased, and Bacteroides increased. (TMAO: trimethylamine N-oxide. SCFAs: short-chain fatty acids).
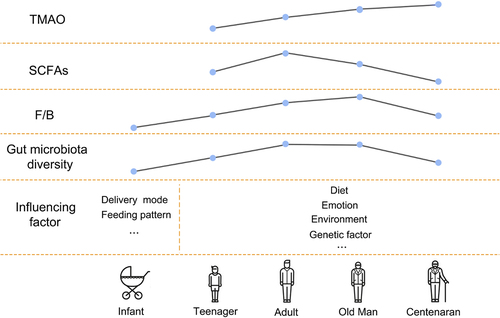
Age and renal function have significant effects on intestinal microbial composition and the levels of metabolites derived from intestinal flora. The intestinal flora of the elderly is characterized by a decrease in glycolysis genes and an increase in proteolytic genes, thus promoting the excessive growth of pathogenic bacteria and aggravating the inflammatory response. Inflammation is accompanied by massive ROS production, which inactivates strict anaerobic bacteria (i.e., Firmicutes) and promotes facultative aerobe,Citation60 The increased expression of inflammatory cytokines reduces the expression of tight junction proteins. This further increases intestinal permeability and may perpetuate inflammation.Citation61,Citation62 With age and deterioration of renal function, TMAO level increases,Citation63 the abundance of SCFA-producing bacteria decreases,Citation64 and bile acid composition becomes unbalanced.Citation65 Studies have shown that patients with chronic kidney disease (CKD) have reduced intestinal bacterial α diversity, altered community composition, and significantly higher TMAO levels than patients with other diseases.Citation66 Exogenous choline supplementation or increased dietary intake of TMAO can lead to the development of renal tubulointerstitial fibrosis and early renal dysfunction.Citation67 Exogenous butyrate supplementation improved AMPK phosphorylation in a rat model of CKD, increased glucagon-like peptide-1 secretion, and promoted colonic mucin and tight junction protein expression levels, thereby strengthening the intestinal barrier and reducing LPS leakage and inflammation.Citation68 Treating diabetic mice with a selective agonist of TGR5, a membrane Ba-activated G-protein – coupled receptor whose activation can inhibit inflammation and reduce atherosclerosis, increased mitochondrial biogenesis and fatty acid β oxidation in the kidney and reduced oxidative stress, as well as having certain anti – renal fibrosis effects.Citation69 In addition, intestinal homeostasis imbalance increases with age, which leads to increased intestinal microbiota LPS production, Toll-like receptor activation, and mucous layer degradation, further increasing intestinal permeability and accelerating systemic endotoxemia and inflammation.Citation70,Citation71 Yamamoto et al. showed that the induction amplitude of CD14 and TLR4 mRNA in the liver and kidney of older mice treated with LPS was greater than that seen in young mice, and that significantly increasing CD14 and TLR4 gene expression enhanced LPS binding and intracellular signaling, mediating many inflammatory responses involved in systemic damage.Citation72
Gut microbiota in age-related cardiovascular diseases
Atherosclerosis
Atherosclerosis (AS) is a chronic metabolic disease caused by abnormal accumulation of lipids and complex carbohydrates in the inner wall of the arteries that leads to artery hardening and the formation of secondary lesions. AS is the pathological basis of coronary heart disease, cerebral infarction, and other serious cardiovascular diseases. It is generally believed that the atherosclerotic plaque itself is a microbial environment. Haraszthy et al. showed that atherosclerotic plaques in human endarterectomy specimens contain DNA from a variety of bacteria, such as Prevotella intermedia, Porphyromonas gingivalis, Actinobacillus actinomycetemcomitans, and Bacteroides forsythus.Citation73 However, the intestinal microbiota of patients with atherosclerotic cardiovascular disease is different from that of healthy individuals. The intestinal microbiota of patients with atherosclerotic cardiovascular disease has higher levels of Streptococcus and Enterobacteriaceae,Citation74 indicating that alterations in the intestinal microbiota are indeed associated with atherosclerosis.
An imbalance in the intestinal flora affects the development of atherosclerosis, mainly through oxidative stress and inflammation. H. pylori has been found to activate NADPH oxidase, which produces ROS.Citation8 REDOX-sensitive signal transduction is activated by NF-κB, activating protein-1 (AP-1), and mitogen activating protein kinase (MAPK), which induces the release of inflammatory cytokines and ultimately vascular inflammation through upregulation of adhesion molecules.Citation75,Citation76 Intestinal flora metabolites are also involved in promoting atherosclerosis. In the study by Vienna E. Brunt et al., plasma TMAO levels in mice were found to increase with age and lead to endothelial dysfunction through oxidative stress and inflammation.Citation77 As an endogenous ligand, TMAO can bind to TLR to increase the secretion of pro-inflammatory cytokines IL-1β and IL-18 as well as ROS.Citation78,Citation79 ROS can damage cells, leading to lipid peroxidation and the oxidation of low-density lipoprotein (LDL). Oxidized low-density lipoprotein (ox-LDL) remains in the intima of blood vessels and induces endothelial cell (EC) dysfunction.Citation80 Scavenger receptors expressed on macrophages (mainly scavenger receptor type A (SR-A) and some CD36 type B family members) are key to the ingestion of ox-LDL molecules by macrophages, which induces their transformation into foam cells.Citation81 Microbial TMA-producing enzyme inhibitors have been found to inhibit platelet reactivity and the rate of thrombosis in animals, as well as inhibiting foam cell formation and atherosclerosis development and reducing the number of bacteria associated with TMAO production.Citation82,Citation83 In addition, LPS and other bacterial components (such as peptidoglycan) can leak into the circulation when intestinal permeability increases, triggering an inflammatory response while stimulating LDL uptake; this reduces cholesterol production by foam cells, promoting monocyte recruitment and macrophage foam cell formation, ultimately leading to atherosclerosis.Citation84 SCFAs, which are intestinal microbe metabolites, have been found to be less abundant in the intestinal flora of patients with atherosclerosis than in healthy individuals.Citation85 SCFAs have anti-inflammatory effects and can inhibit the NF-κB activity of immune cells and reduce the production of pro-inflammatory cytokines.Citation86,Citation87 A study performed in human mononuclear macrophages showed that exogenous SCFA supplementation significantly inhibited NF-κB p65 activation and ROS production, thereby inhibiting activation of the NLRP3 inflammasome and slowing progression of atherosclerotic plaque formation.Citation88 Exogenous propionic acid supplementation modulates intestinal Niemann-Pick C1-like protein-1, a major transmembrane transporter that mediates intestinal cholesterol absorption, thereby reducing cholesterol absorption and inhibiting the development of atherosclerosis.Citation89 In addition, sodium butyrate can inhibit PI3K/Akt/mTOR signaling pathway mediated autophagy, enhance Atg5-mediated autophagy, reduce α-synaptic nucleoprotein expression, increase human microtubule-associated protein light chain 3II (LC3II) expression, reduce p62 expression, and play a protective role in arteriosclerosis.Citation90 Surprisingly, BAs regulate metabolites derived from the gut microbiota, such as TMAO, and play a role in atherosclerosis. BAs are the end product of cholesterol catabolism in the liver. Conversion of cholesterol into BAs is the primary means of eliminating excess cholesterol and maintaining systemic cholesterol homeostasis. In vivo, the farnesol X receptor (FXR) upregulates flavin-containing monooxygenase-3 (FMO3) and increases the plasma TMAO level, thus promoting atherogenesis.Citation91,Citation92 TMAO also activates FXR and nuclear receptor small heterodimer chaperone (SHP) by altering the bile acid profile, downregulates cholesterol 7α-hydroxylase (CYP7A1) expression, and restricts BA synthesis in the liver, thus accelerating the formation of aortic lesions in Apolipoprotein E knockout (ApoE−/−) mice.Citation93 Finasteride, a specific inhibitor of intracellular enzyme-type II 5A-reductase, has been shown to improve gut microbiota richness and diversity, thereby downregulating FMO3 expression, which reduces circulating TMAO levels and inhibits the development of atherosclerosis.Citation94 Little is known about how the gut microbiome mediates mitochondrial dysfunction and thus plays a role in atherosclerosis. However, there is some evidence that changes in intestinal microecology after Canagliflozin treatment can improve cardiac mitochondrial balance, relieve oxidative stress, reduce lipid accumulation, and reduce plasma arteriosclerotic index and atherogenic index.Citation95 This has led to the hypothesis that gut flora is mitochondrial dysfunction – mediated atherosclerosis. In addition to TMAO, LPS and SCFAs, BAs, indole sulfate, a tryptophan metabolite derived from the gut microbiota, has been shown to play a role in AS by promoting pro-inflammatory macrophage activation and thus vascular inflammation,Citation96 while indole-3-propionic acid (IPA) has a protective effect. IPA promotes the reverse transport of cholesterol by macrophages and alleviates the development of atherosclerotic plaques in ApoE−/− mice.Citation97 Gut microbiota – derived phenylalanine metabolite phenylacetylglutamine (PAGln) accelerates platelet clot formation and enhances thrombosis potential.Citation98 For example, greater numbers of microbes associated with PAGln synthesis, such as Roseburia, Blautia, and Ruminococcus, as well as elevated plasma levels of intestinal microbiota – derived metabolite PAGln, were detected in CAD patients with in-stent restenosis.Citation99
Hypertension
Due to a combination of genetic and environmental variables, hypertension is one of the most common chronic diseases and a significant risk factor for cardiovascular and kidney disease. Intestinal ecological imbalance may be a potentially modifiable risk factor for hypertension.Citation100 The microbial-gut-brain axis refers to bidirectional communication between the gut microbiota and the brain mediated by humoral factors such as TMAO and other metabolites (). Changes in this axis are believed to be related to blood pressure regulation.Citation101 It is thought that dysfunction of the gut-brain axis can lead to elevated levels of inflammatory mediators, intestinal flora metabolites, circulating bacteria, and other factors in the circulation that cause neuroinflammation and disrupt autonomic nervous system activity, thus increasing blood pressure, which in turn has a negative impact on intestinal function, resulting in a vicious cycle.Citation102 Neuroinflammation can promote hypertension by enhancing sympathetic nervous system activity.Citation102 Long-term high-salt diets have been shown to increase TMAO levels in the blood and brain, promote neuroinflammation and oxidative stress in the paravicular nucleus of the hypothalamus, and increase the production of pro-inflammatory cytokines such as IL-1β, TNF-α, and NF-κB in the brain, leading to increased sympathetic activity and the development of hypertension.Citation103 Treating rats with DMB (an inhibitor of TMAO formation) reduces neuroinflammation and oxidative stress in the hypothalamic paraventricular nucleus, thereby improving high-fat diet – induced sympathetic excitation and hypertension.Citation103 As mentioned above, TAMO has a significant effect on kidney function. Circulating TMAO not only causes kidney damage by increasing blood pressure, but also directly promotes renal interstitial fibrosis and dysfunction by mediating TGF-β/Smad3 phosphorylation.Citation104 In patients with end-stage renal disease due to the accumulation of circulating metabolic waste, intestinal cell damage is increased, intestinal barrier function is impaired, and local inflammation and cytokine production are triggered, thus exacerbating adverse cardiovascular outcomes and mortality.Citation104,Citation105 In addition, SCFAs have been found to regulate blood pressure through Olfr78,Gpr41,and Gpr43. SCFAs act on renal afferent arteries through Olfr78 to increase renin release and promote basal renin levels, thereby increasing blood pressure.Citation106 SCFAs also act on Gpr41 and Gpr43 to promote vasodilation and thus reduce blood pressure.Citation107
Figure 4. The relationship between hypertension and SCFAs and neuroinflammation. SCFAs increases blood pressure through Olfr78, and also acts on Gpr41 and Gpr43, promoting vasodilation and thus lowering blood pressure. At the same time, neuroinflammation and activity imbalance of autonomic nervous system caused by gut-brain axis dysfunction can also lead to elevated blood pressure. (SCFAs: short-chain fatty acids).
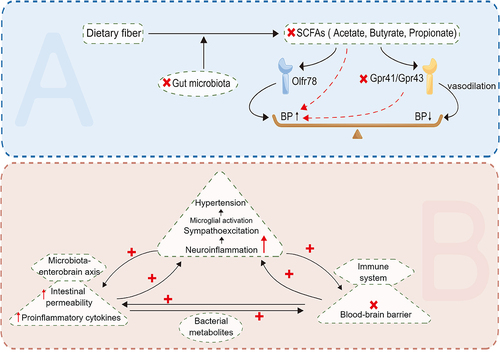
Neuroinflammation can also disrupt the blood-brain barrier and affect the development of hypertension. Disruption of the blood-brain barrier may allow circulating inflammatory factors or intestinal metabolites to enter the brain, thereby affecting microglia function. LPS can be recognized by pattern recognition receptors (PRRS) on microglia cell membranes, activate local immune responses and increase levels of neuroinflammation, thus having a hypertensive effect.Citation101 In addition, microglia are activated by hypertensive stimuli (such as high-salt diet, Ang II, etc.) and release pro-inflammatory cytokines such as TNF-α, IL-1β, and IL-6 and anti-inflammatory cytokines such as IL-10, while inhibition of Ang II signaling reduces neuroinflammation and restores blood pressure.Citation108,Citation109 In a study of 60 diabetic patients, Roshanravan et al. found that exogenous sodium butyrate and inulin supplementation can significantly reduce TNF-α mRNA levels, which may affect the Ang II signaling pathway and thus significantly reduce diastolic blood pressure.Citation110 At the same time, minocycline attenuates microglia activation, inhibits neuroinflammatory processes and rebalances the gut microbiome to play an antihypertensive role.Citation111 In summary, an imbalance in the intestinal flora can cause neuroinflammation, thus promoting hypertension. Therefore, it seems likely that addressing intestinal flora imbalance with targeted drugs could inhibit neuroinflammation and thus control blood pressure.
Myocardial infarction and heart failure
Myocardial infarction is one of the most common causes of heart failure and cardiogenic death worldwide. While percutaneous coronary intervention (PCI), also known as mechanical reperfusion, has reduced acute mortality from myocardial infarction, the incidence of cardiovascular events after myocardial infarction remains high.
Increased intestinal permeability and bloodstream infection are typical complications associated with myocardial infarction and heart failure.Citation112 Lactobacillus, Bacteroides, and Streptococcus, possibly from the gut, have been detected in the blood microbiome of patients with acute ST-segment elevation myocardial infarction.Citation113 Reduced intestinal blood flow, increased gastrointestinal symptoms, increased serum LPS and elevated IgA-anti-LPS levels were also found in patients with chronic cardiac dysfunction.Citation114,Citation115 It has been suggested that the accumulation of intestinal bacteria and intestinal LPS in the systemic circulation triggers the recruitment of monocytes, which activate systemic inflammation and ultimately lead to cardiovascular events after myocardial infarction.Citation113 LPS plays an important role in the progression of coronary heart disease by initiating the transcription of inflammatory factors, inducing the release of inflammatory factors, and inducing chronic low-grade inflammation through the TLR4/MyD88/NF-κB pathway.Citation116 Polymyxin B was used in mice for three consecutive days to inhibit intestinal bacterial transfer and eliminate microbial products from systemic circulation, and significant reductions in plasma LPS levels, myocardial infarction size, local inflammation, and myocardial fibrosis were observed.Citation113
SCFAs secreted by intestinal flora are usually absorbed by epithelial cells. They act on mitochondria and stabilize hypoxia-inducing factors. Intestinal hypoxia caused by visceral under perfusion leads to intestinal acidification and a decrease in the number of butyrate-producing bacteria.Citation117 In addition, one study found that dietary supplementation with SCFAs or monocyte transplantation after myocardial infarction can significantly reverse the adverse effects of antibiotics on mortality and ventricular rupture rate,Citation118 suggesting that SCFAs is beneficial for cardiac repair and improves prognosis after myocardial infarction.
Some studies have found that primary BA levels are increased and secondary BA levels are decreased in patients with chronic heart failure.Citation119 Given that secondary BAs are generated from primary BAs by intestinal microbes,Citation120 this suggests that intestinal microbes are related to heart failure. As mentioned earlier, PAGln plays a role in the formation of blood clots, and it has also been found to play an important role in heart failure. An important manifestation of heart failure is sympathetic overactivation. Although sympathetic overactivation can maintain ejection fraction in the short term, decompensated heart failure will still occur in the long term. PAGln can aggravate heart failure by activating G-protein – coupled receptors such as the α2A, α2B, and β2 adrenergic receptors,Citation98 to a certain extent causing overactivation of the sympathetic nervous system.Citation121 Furthermore, it has been demonstrated that increasing PAGln concentrations correlate with increasing heart failure severity.Citation122 This suggests that PAGln may be a potential predictor of heart failure severity. In addition, the gut microbiota that produce imidazole propionate (ImP) such as Streptococcus mutans, Clostridium symbiotic and Eubacterium eligen have also been found to be enriched in patients with heart failure.Citation123 ImP impacts insulin signaling at the insulin receptor substrate level by activating p38γ MAPK, thereby promoting p62 phosphorylation and subsequently inducing rapamycin complex 1 (mTORC1) signaling; these mechanisms are associated with cardiac fibrosis, hypertrophy, and heart failure.Citation124
Myocardial hypertrophy and fibrosis
Pathological cardiac remodeling, characterized by heart hypertrophy and fibrosis, is a pathological feature of many heart diseases and can lead to heart failure and cardiac arrest. Although few studies have been published on the relationship between the intestinal flora and myocardial hypertrophy and fibrosis, there is some evidence that there is a relationship between the two. One study showed that administering probiotics, prebiotics, and biotin to improve the composition and function of the intestinal flora in rats significantly reduced the cardiac hypertrophy caused by long-term exposure to hypobaric hypoxia.Citation125 This suggests that a normal gut flora is essential to prevent cardiac hypertrophy and fibrosis. Asgharzadeh et al. showed that LPS reduces cardiac antioxidant levels, increases cardiac oxidative stress, and induces myocardial and perivascular fibrosis in rats.Citation126 Singh’s experiments in mice confirmed that LPS also activated TLR-4, thereby inducing the activation of CaMKII in cardiomyocytes. As a signaling molecule for myocardial infarction and ROS activation, overexpression of heart-specific transgenic CaMKII has also been found to cause myocardial hypertrophy, heart failure, and premature death.Citation127 In addition, it has been found that elevated circulatory TMAO levels increase the likelihood of developing heart failure, as they are associated with pathological dilatation of the left ventricle, reduced left ventricular ejection fraction, increased circulating BNP levels, and increased pulmonary edema and myocardial fibrosisCitation128. Li et al. demonstrated that TMAO at least partially aggravates doxorubicin-induced myocardial fibrosis in mice by activating NLRP3 inflammasomes.Citation129 As previously mentioned, NF-κB activates the inflammatory response, and NF-κB and Smad3 play important roles in myocardial fibrosis. TMAO promotes cardiac and renal interstitial fibrosis by activating NF-κB and Smad3. TMAO inhibition reduces NF-κB and Smad3 activation to prevent cardiac remodeling and renal interstitial fibrosis in a variety of heart and kidney diseases.Citation130 For example, Organ et al. found that the microbial enzyme choline TMA lyase blocked the conversion of choline to TMAO at the gut microbiota level, significantly improved left ventricular remodeling, and significantly reduced left ventricular pro-fibrotic gene expression.Citation131 The intestinal flora has been shown to regulate key transcription factors, coactivators, and enzymes related to mitochondrial biogenesis and metabolism, and can directly affect mitochondrial oxidative stress and the formation of mitochondrial autophagic lysosomes, thereby regulating the activation of inflammatory bodies and the production of inflammatory cytokines, which are major factors in myocardial or cardiomyocyte metabolic disorders.Citation18 Pyridine is an acetylcholinesterase inhibitor that inhibits intestinal inflammation, restores intestinal microbiome homeostasis, reduces the abundance of microorganisms that produce branched amino acids, and improves intestinal barrier integrity to decrease circulating levels of branched amino acid.Citation132,Citation133 Yang et al. showed that pyridostigmine alleviated mitochondrial structural abnormalities in mice with diabetic cardiomyopathy induced by a high-fat diet plus streptomycin, increased ATP production, reduced reactive oxygen generation, reduced mitochondria-related cell apoptosis, and alleviated cardiac insufficiency, hypertrophy, and fibrosis.Citation133
Arrhythmia
Arrhythmias are caused by abnormal excitation of the SA node or by excitation generated outside the SA node. Excitation conduction is slow, blocked, or conducted through abnormal channels, with the origin of heart activity and/or conduction disorders resulting in abnormal heart beat frequency and/or rhythm. Recent studies have found that several risk factors for atrial fibrillation, such as diabetes mellitus, obesity, hypertension, obstructive sleep apnea, and coronary artery disease, are independently associated with the progression of atrial fibrillation, as well as with intestinal ecological disorders.Citation134 In other words, arrhythmias and intestinal disorders may share some of the same risk factors and are closely related. Similar to other cardiovascular diseases, butyrate-producing bacteria such as Faecalibacterium and Oscillibacter have decreased abundance in the gut of patients with atrial fibrillation, while Ruminococcus, Streptococcus, and Enterococcus are significantly increased in the gut of patients with atrial fibrillation.Citation135 One study found that the levels of Bacteroides and Prevotella in a high-risk CHA2DS2-VASc group were higher and lower, respectively, than those in a low-risk CHA2DS2-VASc group, suggesting that the risk of thromboembolism in patients with atrial fibrillation is associated with intestinal flora.Citation136
The relationship between atrial fibrillation and inflammation, especially the NLRP3 inflammasome, which is involved in the pathogenesis of atrial fibrillation, has recently attracted increasing attention. Right atrial cardiomyocytes showed higher NLRP3 inflammasome activity in patients with paroxysmal and chronic atrial fibrillation compared with the control group (which comprised individuals with a normal sinus rhythm).Citation137 A selective inflammasome inhibitor (MCC950) blocked AF induction by preventing NLRP3 inflammatory complex formation. Furthermore, SCFAs attenuate NLRP3 inflammasome activation through the GPR43 signaling pathway and ultimately induce NLRP3 degradation through the autophagy pathway, thereby reducing the occurrence of atrial fibrillation.Citation138 Mice with low SCFAs levels have an increased vulnerability to atrial fibrillation, which can be alleviated by SCFAs supplementation.Citation139 In addition, high TMAO levels promote atrial fibrillation by inducing left atrial inflammation and fibrosis;Citation140 indeed, injection of TMAO into the atrial autonomic ganglion cluster in a canine model increased local expression of pro-inflammatory cytokines. While elevated TMAO levels appear to be associated with increased nerve plexus activity, shortening of the atrial effective refractory period due to atrial tachycardia, and increased susceptibility to atrial fibrillation,Citation141 more research is needed to confirm these findings. TMAO also induces a significant upregulation in the expression of pro-inflammatory markers such as IL-1β, IL-6, and TNF-α in tyrosine hydroxylase – positive neurons, which may lead to sympathetic overactivation in the left stellate ganglion and contribute to the occurrence of ventricular arrhythmias.Citation142 LPS is also associated with arrhythmia, and administration of LPS in dogs leads to elevated levels of TNF-α and IL-6 in the blood and right atrium, which may play a role in LPS-induced NF-κB activation and increase susceptibility to atrial fibrillation in the context of systemic inflammation through the adrenergic receptor dependent pathway.Citation143 In addition to inflammation, oxidative stress also plays an important role in arrhythmia. PAGln has been found to increase apoptosis, ROS production, and CaMKII and ryanodine receptor activation, as well as reduce cell viability. In short, PAGln can promote oxidative stress and atrial cell apoptosis to promote atrial fibrillation.Citation144
Gut microbiome as a potential therapeutic direction
The association of gut microbiota composition and gut microbiota – derived metabolites with age-related cardiovascular disease suggests that the gut microbiota may be a potential therapeutic target for age-related cardiovascular disease. Improving gut microbial diversity and depleting or supplementing gut microbiota – derived metabolites with synthetic analogs are two broad categories of potential intervention that are currently under investigation.Citation145 FMT, which involves the transfer of stool from a healthy donor to the gut of another patient, can reverse the recipient’s intestinal microbiome imbalance to a certain extent.Citation1 Studies have found that the relative abundance of Bacteroides in the stool of patients receiving FMT increases, while the abundance of Proteus decreases; in addition, the fecal microbiome of recipients is more diverse after transplantation compared with before transplantation.Citation146 A clinical study of 18 treatment-naive male patients with cardiometabolic syndrome who received a single duodenal infusion of allogeneic FMT or autologous FMT from a lean, healthy donor showed improvement in peripheral blood insulin sensitivity 6 weeks after the infusion. In addition, bacterial diversity and the number of butyrate-producing bacteria increased.Citation147 At present, there are few clinical reports on the effect of FMT on age-related cardiovascular disease, but intestinal flora diversity changes with age, so targeted FMT treatment for different age groups is a potential treatment. In addition, the beneficial effects of FMT on intestinal flora diversity only last for a few weeks,Citation148 so further research is needed to determine how to maintain the effects of this treatment.
As described above, FXR plays a key role in TMAO production and cholesterol metabolism. FXR is distributed in the liver, small intestine, pancreas, kidney, adrenal gland, and other parts of the human body. It plays a role in regulating bile acid metabolism, lipid metabolism, and glucose metabolism, and has been shown to play a protective role in nonalcoholic fatty liver diseaseCitation149 and acute kidney injury.Citation150 Therefore, specific inhibition of FXR expression in the intestine could reduce TMAO production without affecting cholesterol metabolism, and as such is a promising therapeutic approach.
Conclusion
Despite the many clinical treatments available for cardiovascular disease, cardiovascular disease remains the leading cause of death worldwide. The gut microbiota is strongly associated with cardiovascular diseases, which provides potential new therapeutic targets for treating age-related cardiovascular disease. Maintaining a healthy gut flora is essential, not only for proper food digestion and absorption, but also for proper intra-organ metabolism. Especially for patients with cardiovascular disease, dietary optimization is important for maintaining good health. Prebiotics can have beneficial effects on host health by selectively stimulating the growth and/or activity of a healthy gut microbiota. In particular, targeted supplementation with probiotics reduces the abundance of harmful components of the gut microbiota. Regarding FMT, it remains unclear whether infectious diseases can be transmitted to patients through donated stool, whether adverse reactions occur after transplantation, how long the beneficial effects of the transplantation last, and whether additional transplantations are needed to maintain the effects, and more research is needed to address these issues in the future. The gut microbiome may an important target of precision medicine, and increasing our understanding of the underlying mechanisms by which the gut microbiota affects cardiovascular health could help improve the health of patients with age-related cardiovascular disease in the future.
Disclosure statement
No potential conflict of interest was reported by the author(s).
Data availability statement
The data used to support the findings of this study are included within the article.
Additional information
Funding
References
- Zhang PP, Li LL, Han X, Li QW, Zhang XH, Liu JJ, Wang Y. Fecal microbiota transplantation improves metabolism and gut microbiome composition in db/db mice. Acta Pharmacol Sin. 2020;41(5):678–20. doi:10.1038/s41401-019-0330-9.
- Oniszczuk A, Oniszczuk T, Gancarz M, Szymanska J. Role of gut microbiota, probiotics and prebiotics in the cardiovascular diseases. Molecules. 2021;26(4):1172. doi:10.3390/molecules26041172.
- Oh YJ, Pau VC, Steppan J, Sikka G, Bead VR, Nyhan D, Levine BD, Berkowitz DE, Santhanam L. Role of tissue transglutaminase in age-associated ventricular stiffness. Amino Acids. 2017;49(3):695–704. doi:10.1007/s00726-016-2295-z.
- Wenzel P, Schuhmacher S, Kienhofer J, Muller J, Hortmann M, Oelze M, Schulz E, Treiber N, Kawamoto T, Scharffetter-Kochanek K, et al. Manganese superoxide dismutase and aldehyde dehydrogenase deficiency increase mitochondrial oxidative stress and aggravate age-dependent vascular dysfunction. Cardiovasc Res. 2008;80(2):280–289. doi:10.1093/cvr/cvn182.
- Didion SP, Kinzenbaw DA, Schrader LI, Faraci FM. Heterozygous CuZn superoxide dismutase deficiency produces a vascular phenotype with aging. Hypertension. 2006;48(6):1072–1079. doi:10.1161/01.HYP.0000247302.20559.3a.
- Babcock MC, DuBose LE, Witten TL, Stauffer BL, Hildreth KL, Schwartz RS, Kohrt WM, Moreau KL. Oxidative stress and inflammation are associated with age-related endothelial dysfunction in men with Low Testosterone. J Clin Endocrinol Metab. 2022;107(2):e500–e514. doi:10.1210/clinem/dgab715.
- Chen SY, Wang TY, Zhao C, Wang HJ. Oxidative stress bridges the gut microbiota and the occurrence of frailty syndrome. World J Gastroenterol. 2022;28(38):5547–5556. doi:10.3748/wjg.v28.i38.5547.
- Cha B, Lim JW, Kim KH, Kim H. d15-deoxy-Δ12,14-prostaglandin J2 suppresses RANTES expression by inhibiting NADPH oxidase activation in Helicobacter pylori-infected gastric epithelial cells. J Physiol Pharmacol. 2011;62:167–174.
- Choi JH, Cho SO, Kim H. α-Lipoic acid inhibits expression of IL-8 by suppressing activation of MAPK, Jak/Stat, and NF-κB in H. pylori-infected gastric epithelial AGS cells. Yonsei Med J. 2016;57(1):260–264. doi:10.3349/ymj.2016.57.1.260.
- Gatarek P, Kaluzna-Czaplinska J. Trimethylamine N-oxide (TMAO) in human health. Excli J. 2021;20:301–319. doi:10.17179/excli2020-3239.
- Zhou S, Xue J, Shan J, Hong Y, Zhu W, Nie Z, Zhang Y, Ji N, Luo X, Zhang T, et al. Gut-flora-dependent metabolite trimethylamine-N-Oxide promotes atherosclerosis-associated inflammation responses by indirect ROS stimulation and signaling involving AMPK and SIRT1. Nutrients. 2022;14(16):3338. doi:10.3390/nu14163338.
- Choy KW, Lau YS, Murugan D, Vanhoutte PM, Mustafa MR. Paeonol attenuates LPS-Induced endothelial dysfunction and apoptosis by inhibiting BMP4 and TLR4 signaling simultaneously but independently. J Pharmacol Exp Ther. 2018;364(3):420–432. doi:10.1124/jpet.117.245217.
- West SA. Medical education and the role of computers–as seen through the eyes of a medical student. J Med Syst. 1989;13(5):237–241. doi:10.1007/BF00996457.
- Dubois-Deruy E, Peugnet V, Turkieh A, Pinet F. Oxidative Stress in Cardiovascular Diseases. Antioxid (Basel). 2020;9(9):9. doi:10.3390/antiox9090864.
- Moris D, Spartalis M, Tzatzaki E, Spartalis E, Karachaliou GS, Triantafyllis AS, Karaolanis GI, Tsilimigras DI, Theocharis S. The role of reactive oxygen species in myocardial redox signaling and regulation. Ann Transl Med. 2017;5(16):324. doi:10.21037/atm.2017.06.17.
- Feng W, Xu X, Zhao G, Zhao J, Dong R, Ma B, Zhang Y, Long G, Wang DW, Tu L, et al. Increased age-related cardiac dysfunction in bradykinin B2 receptor–deficient mice. J Gerontol A Biol Sci Med Sci. 2016;71(2):178–187. doi:10.1093/gerona/glu210.
- Dai DF, Santana LF, Vermulst M, Tomazela DM, Emond MJ, MacCoss MJ, Gollahon K, Martin GM, Loeb LA, Ladiges WC, et al. Overexpression of catalase targeted to mitochondria attenuates murine cardiac aging. Circulation. 2009;119(21):2789–2797. doi:10.1161/CIRCULATIONAHA.108.822403.
- Wang J, Chen P, Cao Q, Wang W, Chang X. Traditional Chinese medicine ginseng dingzhi decoction ameliorates myocardial fibrosis and high glucose-induced cardiomyocyte injury by regulating intestinal flora and mitochondrial dysfunction. Oxid Med Cell Longev. 2022;2022:1–33. doi:10.1155/2022/9205908.
- Yoo W, Zieba JK, Foegeding NJ, Torres TP, Shelton CD, Shealy NG, Byndloss AJ, Cevallos SA, Gertz E, Tiffany CR, et al. High-fat diet–induced colonocyte dysfunction escalates microbiota-derived trimethylamine N-oxide. Sci. 2021;373(6556):813–818. doi:10.1126/science.aba3683.
- Zhao M, Wei H, Li C, Zhan R, Liu C, Gao J, Yi Y, Cui X, Shan W, Ji L, et al. Gut microbiota production of trimethyl-5-aminovaleric acid reduces fatty acid oxidation and accelerates cardiac hypertrophy. Nat Commun. 2022;13(1):1757. doi:10.1038/s41467-022-29060-7.
- Dutta D, Calvani R, Bernabei R, Leeuwenburgh C, Marzetti E, Sinclair D, North B. Contribution of impaired mitochondrial autophagy to cardiac aging: mechanisms and therapeutic opportunities. Circ Res. 2012;110(8):1125–1138. doi:10.1161/CIRCRESAHA.111.246108.
- Yakes FM, Van Houten B. Mitochondrial DNA damage is more extensive and persists longer than nuclear DNA damage in human cells following oxidative stress. Proc Natl Acad Sci U S A. 1997;94(2):514–519. doi:10.1073/pnas.94.2.514.
- Trifunovic A, Wredenberg A, Falkenberg M, Spelbrink JN, Rovio AT, Bruder CE, Bohlooly-Y M, Gidlöf S, Oldfors A, Wibom R, et al. Premature ageing in mice expressing defective mitochondrial DNA polymerase. Nature. 2004;429(6990):417–423. doi:10.1038/nature02517.
- Dai DF, Chen T, Wanagat J, Laflamme M, Marcinek DJ, Emond MJ, Ngo CP, Prolla TA, Rabinovitch PS. Age-dependent cardiomyopathy in mitochondrial mutator mice is attenuated by overexpression of catalase targeted to mitochondria. Aging Cell. 2010;9(4):536–544. doi:10.1111/j.1474-9726.2010.00581.x.
- Wang J, Li S, Wang J, Wu F, Chen Y, Zhang H, Guo Y, Lin Y, Li L, Yu X, et al. Spermidine alleviates cardiac aging by improving mitochondrial biogenesis and function. Aging (Albany NY). 2020;12(1):650–671. doi:10.18632/aging.102647.
- Wu NN, Zhang Y, Ren J. Mitophagy, Mitochondrial Dynamics, and Homeostasis in Cardiovascular Aging. Oxid Med Cell Longev. 2019;2019:1–15. doi:10.1155/2019/9825061.
- Youle RJ, Narendra DP. Mechanisms of mitophagy. Nat Rev Mol Cell Biol. 2011;12(1):9–14. doi:10.1038/nrm3028.
- Doblado L, Lueck C, Rey C, Samhan-Arias AK, Prieto I, Stacchiotti A, Monsalve M. Mitophagy in human diseases. Int J Mol Sci. 2021;22(8):3903. doi:10.3390/ijms22083903.
- Omar NN, Mosbah RA, Sarawi WS, Rashed MM, Badr AM. Rifaximin protects against malathion-induced rat testicular toxicity: a possible clue on modulating gut microbiome and inhibition of oxidative stress by Mitophagy. Molecules. 2022;27(13):4069. doi:10.3390/molecules27134069.
- Rea IM, Gibson DS, McGilligan V, McNerlan SE, Alexander HD, Ross OA. Age and age-related diseases: role of inflammation triggers and cytokines. Front Immunol. 2018;9:586. doi:10.3389/fimmu.2018.00586.
- Chung HY, Kim DH, Lee EK, Chung KW, Chung S, Lee B, Seo AY, Chung JH, Jung YS, Im E, et al. Redefining chronic inflammation in aging and age-related diseases: proposal of the senoinflammation concept. Aging Dis. 2019;10(2):367–382. doi:10.14336/AD.2018.0324.
- Ferrucci L, Fabbri E. Inflammageing: chronic inflammation in ageing, cardiovascular disease, and frailty. Nat Rev Cardiol. 2018;15(9):505–522. doi:10.1038/s41569-018-0064-2.
- Cao S, Zhang Q, Wang C, Wu H, Jiao L, Hong Q, Hu C. LPS challenge increased intestinal permeability, disrupted mitochondrial function and triggered mitophagy of piglets. Innate Immun. 2018;24(4):221–230. doi:10.1177/1753425918769372.
- Guo S, Al-Sadi R, Said HM, Ma TY. Lipopolysaccharide causes an increase in intestinal tight junction permeability in vitro and in vivo by inducing enterocyte membrane expression and localization of TLR-4 and CD14. Am J Pathol. 2013;182(2):375–387. doi:10.1016/j.ajpath.2012.10.014.
- Al BZ, Nitert MD, Mousa A, Naderpoor N. The Gut Microbiota and Inflammation: An Overview. Int J Environ Res Public Health. 2020;17(20):7618. doi:10.3390/ijerph17207618.
- Jia G, Aroor AR, Jia C, Sowers JR. Endothelial cell senescence in aging-related vascular dysfunction. Biochim Biophys Acta Mol Basis Dis. 2019;1865(7):1802–1809. doi:10.1016/j.bbadis.2018.08.008.
- Barbe-Tuana F, Funchal G, Schmitz C, Maurmann RM, Bauer ME. The interplay between immunosenescence and age-related diseases. Semin Immunopathol. 2020;42(5):545–557. doi:10.1007/s00281-020-00806-z.
- Donato AJ, Eskurza I, Silver AE, Levy AS, Pierce GL, Gates PE, Seals DR. Direct evidence of endothelial oxidative stress with aging in humans: relation to impaired endothelium-dependent dilation and upregulation of nuclear factor-κB. Circ Res. 2007;100(11):1659–1666. doi:10.1161/01.RES.0000269183.13937.e8.
- Donato AJ, Black AD, Jablonski KL, Gano LB, Seals DR. Aging is associated with greater nuclear NFκB, reduced IκBα, and increased expression of proinflammatory cytokines in vascular endothelial cells of healthy humans. Aging Cell. 2008;7(6):805–812. doi:10.1111/j.1474-9726.2008.00438.x.
- Ungvari Z, Orosz Z, Labinskyy N, Rivera A, Xiangmin Z, Smith K, Csiszar A. Increased mitochondrial H2O2 production promotes endothelial NF-κB activation in aged rat arteries. Am J Physiol Heart Circ Physiol. 2007;293(1):H37–H47. doi:10.1152/ajpheart.01346.2006.
- Donato AJ, Pierce GL, Lesniewski LA, Seals DR. Role of NFκB in age-related vascular endothelial dysfunction in humans. Aging (Albany NY). 2009;1(8):678–680. doi:10.18632/aging.100080.
- Sun Y, Li M, Zhao D, Li X, Yang C, Wang X. Lysosome activity is modulated by multiple longevity pathways and is important for lifespan extension in C. elegans. Elife. 2020;9:e55745. doi:10.7554/eLife.55745.
- Hughes AL, Gottschling DE. An early age increase in vacuolar pH limits mitochondrial function and lifespan in yeast. Nature. 2012;492(7428):261–265. doi:10.1038/nature11654.
- Ryu JY, Choi HM, Yang HI, Kim KS. Dysregulated autophagy mediates Sarcopenic obesity and its complications via AMPK and PGC1α signaling pathways: potential involvement of gut dysbiosis as a pathological link. Int J Mol Sci. 2020;21(18):6887. doi:10.3390/ijms21186887.
- Donohoe DR, Garge N, Zhang X, Sun W, O’Connell TM, Bunger MK, Bultman S. The microbiome and butyrate regulate energy metabolism and autophagy in the mammalian colon. Cell Metab. 2011;13(5):517–526. doi:10.1016/j.cmet.2011.02.018.
- Engevik MA, Luk B, Chang-Graham AL, Hall A, Herrmann B, Ruan W, Endres BT, Shi Z, Garey KW, Hyser JM, et al. Bifidobacterium dentium fortifies the intestinal mucus layer via autophagy and calcium signaling pathways. Mbio. 2019;10(3):e01087–19. doi:10.1128/mBio.01087-19.
- Eisenberg T, Abdellatif M, Schroeder S, Primessnig U, Stekovic S, Pendl T, Harger A, Schipke J, Zimmermann A, Schmidt A, et al. Cardioprotection and lifespan extension by the natural polyamine spermidine. Nat Med. 2016;22(12):1428–1438. doi:10.1038/nm.4222.
- He C, Zhu H, Li H, Zou MH, Xie Z. Dissociation of bcl-2–Beclin1 complex by activated AMPK enhances cardiac autophagy and protects against cardiomyocyte apoptosis in diabetes. Diabetes. 2013;62(4):1270–1281. doi:10.2337/db12-0533.
- Wang L, Ma H, Huang P, Xie Y, Near D, Wang H, Xu J, Yang Y, Xu Y, Garbutt T, et al. Down-regulation of Beclin1 promotes direct cardiac reprogramming. Sci Transl Med. 2020;12(566):12. doi:10.1126/scitranslmed.aay7856.
- Fernandez AF, Sebti S, Wei Y, Zou Z, Shi M, McMillan KL, He C, Ting T, Liu Y, Chiang W-C, et al. Disruption of the beclin 1/Bcl-2 autophagy regulatory complex promotes longevity in mice. Nature. 2018;558(7708):136–140. doi:10.1038/s41586-018-0162-7.
- Woodall BP, Gustafsson AB. Autophagy—A key pathway for cardiac health and longevity. Acta Physiol (Oxf). 2018;223(4):e13074. doi:10.1111/apha.13074.
- Li J, Zhang D, Wiersma M, Brundel B. Role of autophagy in Proteostasis: friend and foe in cardiac diseases. Cells. 2018;7(12):279. doi:10.3390/cells7120279.
- Robertson RC, Manges AR, Finlay BB, Prendergast AJ. The human microbiome and child growth – first 1000 days and beyond. Trends Microbiol. 2019;27(2):131–147. doi:10.1016/j.tim.2018.09.008.
- Anwar H, Iftikhar A, Muzaffar H, Almatroudi A, Allemailem KS, Navaid S, Saleem S, Khurshid M. Biodiversity of gut microbiota: impact of various Host and environmental factors. Biomed Res Int. 2021;2021:1–9. doi:10.1155/2021/5575245.
- Dominguez-Bello MG, Costello EK, Contreras M, Magris M, Hidalgo G, Fierer N, Knight R. Delivery mode shapes the acquisition and structure of the initial microbiota across multiple body habitats in newborns. Proc Natl Acad Sci U S A. 2010;107(26):11971–11975. doi:10.1073/pnas.1002601107.
- Roswall J, Olsson LM, Kovatcheva-Datchary P, Nilsson S, Tremaroli V, Simon MC, Kiilerich P, Akrami R, Krämer M, Uhlén M, et al. Developmental trajectory of the healthy human gut microbiota during the first 5 years of life. Cell Host & Microbe. 2021;29(5):765–776.e3. doi:10.1016/j.chom.2021.02.021.
- Nicholson JK, Holmes E, Kinross J, Burcelin R, Gibson G, Jia W, Pettersson S. Host-gut microbiota metabolic interactions. Sci. 2012;336(6086):1262–1267. doi:10.1126/science.1223813.
- Biagi E, Nylund L, Candela M, Ostan R, Bucci L, Pini E, Nikkïla J, Monti D, Satokari R, Franceschi C, et al. Through ageing, and beyond: gut microbiota and inflammatory status in seniors and centenarians. PLoS ONE. 2010;5(5):e10667. doi:10.1371/journal.pone.0010667.
- Salazar N, Arboleya S, Fernandez-Navarro T, de Los CG, Gonzalez S, Gueimonde M. Age-associated changes in gut microbiota and dietary components related with the immune system in Adulthood and old age: a cross-sectional study. Nutrients. 2019;11(8):11. doi:10.3390/nu11081765.
- Rampelli S, Candela M, Turroni S, Biagi E, Collino S, Franceschi C, O’Toole PW, Brigidi P. Functional metagenomic profiling of intestinal microbiome in extreme ageing. Aging (Albany NY). 2013;5(12):902–912. doi:10.18632/aging.100623.
- Fransen F, van Beek AA, Borghuis T, Aidy SE, Hugenholtz F, van der Gaast-de JC, Savelkoul HFJ, De Jonge MI, Boekschoten MV, Smidt H, et al. Aged gut microbiota contributes to systemical inflammaging after transfer to germ-free mice. Front Immunol. 2017;8:1385. doi:10.3389/fimmu.2017.01385.
- DeJong EN, Surette MG, Bowdish D. The gut microbiota and unhealthy aging: disentangling cause from consequence. Cell Host & Microbe. 2020;28(2):180–189. doi:10.1016/j.chom.2020.07.013.
- Rath S, Rox K, Kleine BS, Schminke U, Dorr M, Mayerle J, Frost F, Lerch MM, Karch A, Brönstrup M, et al. Higher trimethylamine-N-Oxide plasma levels with increasing age are mediated by diet and trimethylamine-forming bacteria. mSystems. 2021;6(5):e94521. doi:10.1128/mSystems.00945-21.
- Wong J, Piceno YM, DeSantis TZ, Pahl M, Andersen GL, Vaziri ND. Expansion of urease- and uricase-containing, indole- and p-cresol-forming and contraction of short-chain fatty acid-producing intestinal microbiota in ESRD. Am J Nephrol. 2014;39(3):230–237. doi:10.1159/000360010.
- Wang X, Yang S, Li S, Zhao L, Hao Y, Qin J, Zhang L, Zhang C, Bian W, Zuo L, et al. Aberrant gut microbiota alters host metabolome and impacts renal failure in humans and rodents. Gut. 2020;69(12):2131–2142. doi:10.1136/gutjnl-2019-319766.
- Xu KY, Xia GH, Lu JQ, Chen MX, Zhen X, Wang S, You C, Nie J, Zhou H-W, Yin J, et al. Impaired renal function and dysbiosis of gut microbiota contribute to increased trimethylamine-N-oxide in chronic kidney disease patients. Sci Rep. 2017;7(1):1445. doi:10.1038/s41598-017-01387-y.
- Tang WH, Wang Z, Kennedy DJ, Wu Y, Buffa JA, Agatisa-Boyle B, Li XS, Levison BS, Hazen SL. Gut microbiota-dependent trimethylamine N-oxide (TMAO) pathway contributes to both development of renal insufficiency and mortality risk in chronic kidney disease. Circ Res. 2015;116(3):448–455. doi:10.1161/CIRCRESAHA.116.305360.
- Gonzalez A, Krieg R, Massey HD, Carl D, Ghosh S, Gehr T, Ghosh SS. Sodium butyrate ameliorates insulin resistance and renal failure in CKD rats by modulating intestinal permeability and mucin expression. Nephrol Dial Transplant. 2019;34(5):783–794. doi:10.1093/ndt/gfy238.
- Wang XX, Edelstein MH, Gafter U, Qiu L, Luo Y, Dobrinskikh E, Lucia S, Adorini L, D’Agati VD, Levi J, et al. G protein-coupled bile acid receptor TGR5 activation inhibits kidney disease in obesity and diabetes. J Am Soc Nephrol. 2016;27(5):1362–1378. doi:10.1681/ASN.2014121271.
- Kim KA, Jeong JJ, Yoo SY, Kim DH. Gut microbiota lipopolysaccharide accelerates inflamm-aging in mice. BMC Microbiol. 2016;16(1):9. doi:10.1186/s12866-016-0625-7.
- Xu Y, Liu X, Liu X, Chen D, Wang M, Jiang X, Xiong Z. The roles of the gut microbiota and chronic low-grade inflammation in older adults with frailty. Front Cell Infect Microbiol. 2021;11:675414. doi:10.3389/fcimb.2021.675414.
- Yamamoto K, Shimokawa T, Yi H, Isobe K, Kojima T, Loskutoff DJ, Saito H. Aging accelerates endotoxin-induced thrombosis: increased responses of plasminogen activator inhibitor-1 and lipopolysaccharide signaling with aging. Am J Pathol. 2002;161(5):1805–1814. doi:10.1016/S0002-9440(10)64457-4.
- Haraszthy VI, Zambon JJ, Trevisan M, Zeid M, Genco RJ. Identification of periodontal pathogens in atheromatous plaques. J Periodontol. 2000;71(10):1554–1560. doi:10.1902/jop.2000.71.10.1554.
- Jie Z, Xia H, Zhong SL, Feng Q, Li S, Liang S, Zhong H, Liu Z, Gao Y, Zhao H, et al. The gut microbiome in atherosclerotic cardiovascular disease. Nat Commun. 2017;8(1):845. doi:10.1038/s41467-017-00900-1.
- Cho SO, Lim JW, Kim KH, Kim H. Diphenyleneiodonium inhibits the activation of mitogen-activated protein kinases and the expression of monocyte chemoattractant protein-1 in Helicobacter pylori-infected gastric epithelial AGS cells. Inflamm Res. 2011;60(5):501–507. doi:10.1007/s00011-010-0297-y.
- Chu SH, Kim H, Seo JY, Lim JW, Mukaida N, Kim KH. Role of NF-kappaB and AP-1 on Helicobater pylori-induced IL-8 expression in AGS cells. Dig Dis Sci. 2003;48(2):257–265. doi:10.1023/A:1021963007225.
- Brunt VE, Gioscia-Ryan RA, Richey JJ, Zigler MC, Cuevas LM, Gonzalez A, Vázquez‐Baeza Y, Battson ML, Smithson AT, Gilley AD, et al. Suppression of the gut microbiome ameliorates age-related arterial dysfunction and oxidative stress in mice. J Physiol. 2019;597(9):2361–2378. doi:10.1113/JP277336.
- Hakhamaneshi MS, Abdolahi A, Vahabzadeh Z, Abdi M, Andalibi P. Toll-like receptor 4: a macrophage cell surface receptor is activated by trimethylamine-N-Oxide. Cell J. 2021;23(5):516–522. doi:10.22074/cellj.2021.7849.
- Chen ML, Zhu XH, Ran L, Lang HD, Yi L, Mi MT. Trimethylamine-N-Oxide induces vascular inflammation by activating the NLRP3 inflammasome through the SIRT3-SOD2-mtROS signaling pathway. J Am Heart Assoc. 2017;6(9):e006347. doi:10.1161/JAHA.117.006347.
- Malekmohammad K, Bezsonov EE, Rafieian-Kopaei M. Role of Lipid Accumulation and Inflammation in Atherosclerosis: Focus on Molecular and Cellular Mechanisms. Front Cardiovasc Med. 2021;8:707529. doi:10.3389/fcvm.2021.707529.
- Poznyak AV, Nikiforov NG, Starodubova AV, Popkova TV, Orekhov AN. Macrophages and foam cells: brief Overview of their role, linkage, and targeting potential in atherosclerosis. Biomedicines. 2021;9(9):1221. doi:10.3390/biomedicines9091221.
- Wang Z, Roberts AB, Buffa JA, Levison BS, Zhu W, Org E, Gu X, Huang Y, Zamanian-Daryoush M, Culley M, et al. Non-lethal inhibition of gut microbial trimethylamine production for the treatment of atherosclerosis. Cell. 2015;163(7):1585–1595. doi:10.1016/j.cell.2015.11.055.
- Roberts AB, Gu X, Buffa JA, Hurd AG, Wang Z, Zhu W, Gupta N, Skye SM, Cody DB, Levison BS, et al. Development of a gut microbe–targeted nonlethal therapeutic to inhibit thrombosis potential. Nat Med. 2018;24(9):1407–1417. doi:10.1038/s41591-018-0128-1.
- Lau K, Srivatsav V, Rizwan A, Nashed A, Liu R, Shen R, Akhtar M. Bridging the Gap between gut microbial dysbiosis and cardiovascular diseases. Nutrients. 2017;9(8):859. doi:10.3390/nu9080859.
- Kwun JS, Kang SH, Lee HJ, Park HK, Lee WJ, Yoon CH, Suh J-W, Cho Y-S, Youn T-J, Chae I-H, et al. Comparison of thrombus, gut, and oral microbiomes in Korean patients with ST-elevation myocardial infarction: a case–control study. Experimental & Molecular Medicine. 2020;52(12):2069–2079. doi:10.1038/s12276-020-00543-1.
- Brandsma E, Kloosterhuis NJ, Koster M, Dekker DC, Gijbels M, van der Velden S, Ríos-Morales M, van Faassen MJR, Loreti MG, de Bruin A, et al. A proinflammatory gut microbiota increases systemic inflammation and accelerates atherosclerosis. Circ Res. 2019;124(1):94–100. doi:10.1161/CIRCRESAHA.118.313234.
- Bartolomaeus H, Balogh A, Yakoub M, Homann S, Marko L, Hoges S, Tsvetkov D, Krannich A, Wundersitz S, Avery EG, et al. Short-chain fatty acid propionate protects from hypertensive cardiovascular damage. Circulation. 2019;139(11):1407–1421. doi:10.1161/CIRCULATIONAHA.118.036652.
- Yi C, Sun W, Ding L, Yan M, Sun C, Qiu C, Wang D, Wu L. Short-chain fatty acids Weaken ox-LDL-Induced cell inflammatory injury by inhibiting the NLRP3/Caspase-1 pathway and affecting cellular metabolism in THP-1 cells. Molecules. 2022;27(24):8801. doi:10.3390/molecules27248801.
- Haghikia A, Zimmermann F, Schumann P, Jasina A, Roessler J, Schmidt D, Heinze P, Kaisler J, Nageswaran V, Aigner A, et al. Propionate attenuates atherosclerosis by immune-dependent regulation of intestinal cholesterol metabolism. Eur Heart J. 2022;43(6):518–533. doi:10.1093/eurheartj/ehab644.
- Qiao CM, Sun MF, Jia XB, Shi Y, Zhang BP, Zhou ZL, Zhao L-P, Cui C, Shen Y-Q. Sodium butyrate causes α-synuclein degradation by an Atg5-dependent and PI3K/Akt/mTOR-related autophagy pathway. Exp Cell Res. 2020;387(1):111772. doi:10.1016/j.yexcr.2019.111772.
- Bennett BJ, de Aguiar VT, Wang Z, Shih DM, Meng Y, Gregory J, Allayee H, Lee R, Graham M, Crooke R, et al. Trimethylamine-N-oxide, a metabolite associated with atherosclerosis, exhibits complex genetic and dietary regulation. Cell Metab. 2013;17(1):49–60. doi:10.1016/j.cmet.2012.12.011.
- Guan B, Tong J, Hao H, Yang Z, Chen K, Xu H, Wang A. Bile acid coordinates microbiota homeostasis and systemic immunometabolism in cardiometabolic diseases. Acta Pharm Sin B. 2022;12(5):2129–2149. doi:10.1016/j.apsb.2021.12.011.
- Ding L, Chang M, Guo Y, Zhang L, Xue C, Yanagita T, Zhang T, Wang Y. Trimethylamine-N-oxide (TMAO)-induced atherosclerosis is associated with bile acid metabolism. Lipids Health Dis. 2018;17(1):286. doi:10.1186/s12944-018-0939-6.
- Wang Z, You L, Ren Y, Zhu X, Mao X, Liang X, Wang T, Guo Y, Liu T, Xue J, et al. Finasteride alleviates high fat associated protein-overload nephropathy by inhibiting trimethylamine N-Oxide synthesis and regulating gut microbiota. Front Physiol. 2022;13:900961. doi:10.3389/fphys.2022.900961.
- Wang X, Wang Z, Liu D, Jiang H, Cai C, Li G, Yu G. Canagliflozin Prevents Lipid Accumulation, Mitochondrial Dysfunction, and Gut Microbiota Dysbiosis in Mice With Diabetic Cardiovascular Disease. Front Pharmacol. 2022;13:839640. doi:10.3389/fphar.2022.839640.
- Nakano T, Katsuki S, Chen M, Decano JL, Halu A, Lee LH, Pestana DVS, Kum AST, Kuromoto RK, Golden WS, et al. Uremic toxin indoxyl sulfate promotes proinflammatory macrophage activation via the interplay of OATP2B1 and Dll4-notch signaling. Circulation. 2019;139(1):78–96. doi:10.1161/CIRCULATIONAHA.118.034588.
- Xue H, Chen X, Yu C, Deng Y, Zhang Y, Chen S, Chen X, Chen K, Yang Y, Ling W, et al. Gut Microbially produced indole-3-propionic acid inhibits atherosclerosis by promoting reverse cholesterol Transport and its deficiency is causally related to atherosclerotic cardiovascular disease. Circ Res. 2022;131(5):404–420. doi:10.1161/CIRCRESAHA.122.321253.
- Nemet I, Saha PP, Gupta N, Zhu W, Romano KA, Skye SM, Cajka T, Mohan ML, Li L, Wu Y, et al. A Cardiovascular Disease-Linked Gut Microbial Metabolite Acts via Adrenergic Receptors. Cell. 2020;180(5):862–877.e22. doi:10.1016/j.cell.2020.02.016.
- Fang C, Zuo K, Fu Y, Li J, Wang H, Xu L, Yang X. Dysbiosis of gut microbiota and metabolite phenylacetylglutamine in coronary artery disease patients with stent stenosis. Front Cardiovasc Med. 2022;9:832092. doi:10.3389/fcvm.2022.832092.
- Qin Y, Zhao J, Wang Y, Bai M, Sun S. Specific alterations of gut microbiota in Chinese patients with hypertension: a systematic review and meta-analysis. Kidney Blood Press Res. 2022;47(7):433–447. doi:10.1159/000524282.
- Wang X, Chen Z, Geng B, Cai J, D Elia L. The Bidirectional signal Communication of microbiota-gut-brain axis in hypertension. Int J Hypertens. 2021;2021:1–9. doi:10.1155/2021/8174789.
- Richards EM, Li J, Stevens BR, Pepine CJ, Raizada MK. Gut Microbiome and Neuroinflammation in Hypertension. Circ Res. 2022;130(3):401–417. doi:10.1161/CIRCRESAHA.121.319816.
- Liu G, Cheng J, Zhang T, Shao Y, Chen X, Han L, Zhou R, Wu B. Inhibition of microbiota-dependent trimethylamine N-Oxide production ameliorates high salt diet-induced sympathetic excitation and hypertension in rats by attenuating central neuroinflammation and oxidative stress. Front Pharmacol. 2022;13:856914. doi:10.3389/fphar.2022.856914.
- Zhou J, Wang D, Li B, Li X, Lai X, Lei S, Li N, Zhang X. Relationship between plasma trimethylamine N-Oxide levels and renal dysfunction in patients with hypertension. Kidney Blood Press Res. 2021;46(4):421–432. doi:10.1159/000513033.
- Chaves LD, McSkimming DI, Bryniarski MA, Honan AM, Abyad S, Thomas SA, Wells S, Buck M, Sun Y, Genco RJ, et al. Chronic kidney disease, uremic milieu, and its effects on gut bacterial microbiota dysbiosis. Am J Physiol Renal Physiol. 2018;315(3):F487–F502. doi:10.1152/ajprenal.00092.2018.
- Pluznick JL, Protzko RJ, Gevorgyan H, Peterlin Z, Sipos A, Han J, Brunet I, Wan L-X, Rey F, Wang T, et al. Olfactory receptor responding to gut microbiota-derived signals plays a role in renin secretion and blood pressure regulation. Proc Natl Acad Sci U S A. 2013;110(11):4410–4415. doi:10.1073/pnas.1215927110.
- Lu Y, Fan C, Li P, Lu Y, Chang X, Qi K. Short chain fatty acids prevent high-fat-diet-induced obesity in mice by regulating G protein-coupled receptors and gut microbiota. Sci Rep. 2016;6(1):37589. doi:10.1038/srep37589.
- Krishnan SM, Sobey CG, Latz E, Mansell A, Drummond GR. IL-1β and IL-18: inflammatory markers or mediators of hypertension? Br J Pharmacol. 2014;171(24):5589–5602. doi:10.1111/bph.12876.
- Yu Y, Wei SG, Weiss RM, Felder RB. Angiotensin II Type 1a Receptors in the Subfornical Organ Modulate Neuroinflammation in the Hypothalamic Paraventricular Nucleus in Heart Failure Rats. Neuroscience. 2018;381:46–58. doi:10.1016/j.neuroscience.2018.04.012.
- Roshanravan N, Mahdavi R, Alizadeh E, Ghavami A, Rahbar SY, Mesri AN, Alipour S, Dastouri MR, Ostadrahimi A. The effects of sodium butyrate and inulin supplementation on angiotensin signaling pathway via promotion of akkermansia muciniphila abundance in type 2 diabetes; a randomized, double-blind, placebo-controlled trial. J Cardiovasc Thorac Res. 2017;9(4):183–190. doi:10.15171/jcvtr.2017.32.
- Sharma RK, Yang T, Oliveira AC, Lobaton GO, Aquino V, Kim S, Richards EM, Pepine CJ, Sumners C, Raizada MK, et al. Microglial cells impact gut microbiota and gut pathology in angiotensin II-Induced hypertension. Circ Res. 2019;124(5):727–736. doi:10.1161/CIRCRESAHA.118.313882.
- Khan I, Khan I, Kakakhel MA, Xiaowei Z, Ting M, Ali I, Fei Y, Jianye Z, Zhiqiang L, Lizhe A, et al. Comparison of microbial populations in the blood of patients with myocardial infarction and healthy individuals. Front Microbiol. 2022;13:845038. doi:10.3389/fmicb.2022.845038.
- Zhou X, Li J, Guo J, Geng B, Ji W, Zhao Q, Li J, Liu X, Liu J, Guo Z, et al. Gut-dependent microbial translocation induces inflammation and cardiovascular events after ST-elevation myocardial infarction. Microbiome. 2018;6(1):66. doi:10.1186/s40168-018-0441-4.
- Sandek A, Bjarnason I, Volk HD, Crane R, Meddings JB, Niebauer J, Kalra PR, Buhner S, Herrmann R, Springer J, et al. Studies on bacterial endotoxin and intestinal absorption function in patients with chronic heart failure. Int J Cardiol. 2012;157(1):80–85. doi:10.1016/j.ijcard.2010.12.016.
- Sandek A, Swidsinski A, Schroedl W, Watson A, Valentova M, Herrmann R, Scherbakov N, Cramer L, Rauchhaus M, Grosse-Herrenthey A, et al. Intestinal blood flow in patients with chronic heart failure: a link with bacterial growth, gastrointestinal symptoms, and cachexia. J Am Coll Cardiol. 2014;64(11):1092–1102. doi:10.1016/j.jacc.2014.06.1179.
- Chistiakov DA, Bobryshev YV, Kozarov E, Sobenin IA, Orekhov AN. Role of gut microbiota in the modulation of atherosclerosis-associated immune response. Front Microbiol. 2015;6:671. doi:10.3389/fmicb.2015.00671.
- Gallo A, Macerola N, Favuzzi AM, Nicolazzi MA, Gasbarrini A, Montalto M. The gut in heart failure: Current knowledge and novel frontiers. Med Princ Pract. 2022;31(3):203–214. doi:10.1159/000522284.
- Tang T, Chen HC, Chen CY, Yen C, Lin CJ, Prajnamitra RP, Chen L-L, Ruan S-C, Lin J-H, Lin P-J, et al. Loss of gut microbiota alters immune system composition and cripples postinfarction cardiac repair. Circulation. 2019;139(5):647–659. doi:10.1161/CIRCULATIONAHA.118.035235.
- Mayerhofer C, Ueland T, Broch K, Vincent RP, Cross GF, Dahl CP, Aukrust P, Gullestad L, Hov JR, Trøseid M, et al. Increased secondary/primary bile acid ratio in chronic heart failure. J Card Fail. 2017;23(9):666–671. doi:10.1016/j.cardfail.2017.06.007.
- Zhang R, Ma WQ, Fu MJ, Li J, Hu CH, Chen Y, Zhou M-M, Gao Z-J, He Y-L. Overview of bile acid signaling in the cardiovascular system. World J Clin Cases. 2021;9(2):308–320. doi:10.12998/wjcc.v9.i2.308.
- Zong X, Fan Q, Yang Q, Pan R, Zhuang L, Tao R. Phenylacetylglutamine as a risk factor and prognostic indicator of heart failure. ESC Heart Fail. 2022;9(4):2645–2653. doi:10.1002/ehf2.13989.
- Zhang Z, Cai B, Sun Y, Deng H, Wang H, Qiao Z. Alteration of the gut microbiota and metabolite phenylacetylglutamine in patients with severe chronic heart failure. Front Cardiovasc Med. 2022;9:1076806. doi:10.3389/fcvm.2022.1076806.
- Molinaro A, Nemet I, Bel LP, Chakaroun R, Nielsen T, Aron-Wisnewsky J, Bergh P-O, Li L, Henricsson M, Køber L, et al. Microbially produced imidazole propionate is associated with heart failure and mortality. JACC Heart Fail. 2023;11(7):810–821. doi:10.1016/j.jchf.2023.03.008.
- Koh A, Molinaro A, Stahlman M, Khan MT, Schmidt C, Manneras-Holm L, Wu H, Carreras A, Jeong H, Olofsson LE, et al. Microbially produced imidazole propionate impairs insulin signaling through mTORC1. Cell. 2018;175(4):947–961.e17. doi:10.1016/j.cell.2018.09.055.
- Hu Y, Pan Z, Huang Z, Li Y, Han N, Zhuang X, Peng H, Gao Q, Wang Q, Yang Lee BJ, et al. Gut microbiome-targeted modulations regulate metabolic profiles and alleviate altitude-related cardiac hypertrophy in rats. Microbiol Spectr. 2022;10(1):e105321. doi:10.1128/spectrum.01053-21.
- Asgharzadeh F, Bargi R, Beheshti F, Hosseini M, Farzadnia M, Khazaei M. Thymoquinone prevents myocardial and perivascular fibrosis induced by chronic lipopolysaccharide exposure in male rats: - thymoquinone and cardiac fibrosis. J Pharmacopuncture. 2018;21(4):284–293. doi:10.3831/KPI.2018.21.032.
- Singh MV, Swaminathan PD, Luczak ED, Kutschke W, Weiss RM, Anderson ME. MyD88 mediated inflammatory signaling leads to CaMKII oxidation, cardiac hypertrophy and death after myocardial infarction. J Mol Cell Cardiol. 2012;52(5):1135–1144. doi:10.1016/j.yjmcc.2012.01.021.
- Organ CL, Otsuka H, Bhushan S, Wang Z, Bradley J, Trivedi R, Polhemus DJ, Tang WHW, Wu Y, Hazen SL, et al. Choline diet and its gut microbe–derived metabolite, trimethylamine N-Oxide, exacerbate pressure overload–induced heart failure. Circ Heart Fail. 2016;9(1):e2314. doi:10.1161/CIRCHEARTFAILURE.115.002314.
- Li X, Geng J, Zhao J, Ni Q, Zhao C, Zheng Y, Chen X, Wang L. Trimethylamine N-Oxide exacerbates cardiac fibrosis via activating the NLRP3 inflammasome. Front Physiol. 2019;10:866. doi:10.3389/fphys.2019.00866.
- Zou D, Li Y, Sun G. Attenuation of circulating trimethylamine N-Oxide prevents the progression of cardiac and renal dysfunction in a rat model of chronic cardiorenal syndrome. Front Pharmacol. 2021;12:751380. doi:10.3389/fphar.2021.751380.
- Organ CL, Li Z, Sharp TR, Polhemus DJ, Gupta N, Goodchild TT, Tang WHW, Hazen SL, Lefer DJ. Nonlethal inhibition of gut microbial trimethylamine N-oxide production improves cardiac function and remodeling in a murine model of heart failure. J Am Heart Assoc. 2020;9(10):e16223. doi:10.1161/JAHA.119.016223.
- Singh SP, Chand HS, Banerjee S, Agarwal H, Raizada V, Roy S, Sopori M. Acetylcholinesterase inhibitor pyridostigmine bromide attenuates gut pathology and bacterial dysbiosis in a murine model of ulcerative colitis. Dig Dis Sci. 2020;65(1):141–149. doi:10.1007/s10620-019-05838-6.
- Yang Y, Zhao M, He X, Wu Q, Li DL, Zang WJ. Pyridostigmine Protects Against diabetic cardiomyopathy by regulating vagal activity, gut microbiota, and branched-chain amino acid catabolism in diabetic mice. Front Pharmacol. 2021;12:647481. doi:10.3389/fphar.2021.647481.
- Gawalko M, Agbaedeng TA, Saljic A, Muller DN, Wilck N, Schnabel R, Penders J, Rienstra M, van Gelder I, Jespersen T, et al. Gut microbiota, dysbiosis and atrial fibrillation. Arrhythmogenic mechanisms and potential clinical implications. Cardiovasc Res. 2022;118(11):2415–2427. doi:10.1093/cvr/cvab292.
- Zuo K, Li J, Li K, Hu C, Gao Y, Chen M, Hu R, Liu Y, Chi H, Wang H, et al. Disordered gut microbiota and alterations in metabolic patterns are associated with atrial fibrillation. Gigascience. 2019;8(6):giz058. doi:10.1093/gigascience/giz058.
- Fang C, Zuo K, Zhang W, Zhong J, Li J, Xu L, Yang X. Association between gut microbiota dysbiosis and the CHA2DS2-VASc score in atrial fibrillation patients. Int J Clin Pract. 2022;2022:1–10. doi:10.1155/2022/7942605.
- Scott LJ, Li N, Dobrev D. Role of inflammatory signaling in atrial fibrillation. Int J Cardiol. 2019;287:195–200. doi:10.1016/j.ijcard.2018.10.020.
- Zhang J, Zuo K, Fang C, Yin X, Liu X, Zhong J, Li K, Li J, Xu L, Yang X, et al. Altered synthesis of genes associated with short-chain fatty acids in the gut of patients with atrial fibrillation. Bmc Genom. 2021;22(1):634. doi:10.1186/s12864-021-07944-0.
- Zuo K, Fang C, Liu Z, Fu Y, Liu Y, Liu L, Wang Y, Yin X, Liu X, Li J, et al. Commensal microbe-derived SCFA alleviates atrial fibrillation via GPR43/NLRP3 signaling. Int J Biol Sci. 2022;18(10):4219–4232. doi:10.7150/ijbs.70644.
- Yang WT, Yang R, Zhao Q, Li XD, Wang YT. A systematic review and meta-analysis of the gut microbiota-dependent metabolite trimethylamine N-oxide with the incidence of atrial fibrillation. Ann Palliat Med. 2021;10(11):11512–11523. doi:10.21037/apm-21-2763.
- Linz D, Gawalko M, Sanders P, Penders J, Li N, Nattel S, Dobrev D. Does gut microbiota affect atrial rhythm? Causalities and speculations. Eur Heart J. 2021;42(35):3521–3525. doi:10.1093/eurheartj/ehab467.
- Meng G, Zhou X, Wang M, Zhou L, Wang Z, Wang M, Deng J, Wang Y, Zhou Z, Zhang Y, et al. Gut microbe-derived metabolite trimethylamine N-oxide activates the cardiac autonomic nervous system and facilitates ischemia-induced ventricular arrhythmia via two different pathways. EBioMedicine. 2019;44:656–664. doi:10.1016/j.ebiom.2019.03.066.
- Chen YY, Sun ZW, Jiang JP, Kang XD, Wang LL, Shen YL, Xie X-D, Zheng L-R. α-adrenoceptor-mediated enhanced inducibility of atrial fibrillation in a canine system inflammation model. Mol Med Rep. 2017;15(6):3767–3774. doi:10.3892/mmr.2017.6477.
- Fang C, Zuo K, Jiao K, Zhu X, Fu Y, Zhong J, Xu L, Yang X. Pagln, an atrial fibrillation-Linked gut microbial metabolite, acts as a promoter of atrial myocyte injury. Biomolecules. 2022;12(8):1120. doi:10.3390/biom12081120.
- Yuan S, Cai Z, Luan X, Wang H, Zhong Y, Deng L, Feng J. Gut microbiota: a new therapeutic target for diabetic cardiomyopathy. Front Pharmacol. 2022;13:963672. doi:10.3389/fphar.2022.963672.
- Seekatz AM, Aas J, Gessert CE, Rubin TA, Saman DM, Bakken JS, Young VB. Recovery of the gut microbiome following fecal microbiota transplantation. Mbio. 2014;5(3):e814–e893. doi:10.1128/mBio.00893-14.
- Vrieze A, Van Nood E, Holleman F, Salojarvi J, Kootte RS, Bartelsman JF, Dallinga–Thie GM, Ackermans MT, Serlie MJ, Oozeer R, et al. Transfer of intestinal microbiota from lean donors increases insulin sensitivity in individuals with metabolic syndrome. Gastroenterology. 2012;143(4):913–916.e7. doi:10.1053/j.gastro.2012.06.031.
- Manrique P, Zhu Y, van der Oost J, Herrema H, Nieuwdorp M, de Vos WM, Young M. Gut bacteriophage dynamics during fecal microbial transplantation in subjects with metabolic syndrome. Gut Microbes. 2021;13(1):1–15. doi:10.1080/19490976.2021.1897217.
- Gonzalez FJ, Jiang C, Xie C, Patterson AD. Intestinal Farnesoid X Receptor Signaling Modulates Metabolic Disease. Dig Dis. 2017;35(3):178–184. doi:10.1159/000450908.
- Xu S, Jia P, Fang Y, Jin J, Sun Z, Zhou W, Li J, Zhang Y, Wang X, Ren T, et al. Nuclear farnesoid X receptor attenuates acute kidney injury through fatty acid oxidation. Kidney Int. 2022;101(5):987–1002. doi:10.1016/j.kint.2022.01.029.