ABSTRACT
Aging is an inevitable natural process that impacts every individual, and understanding its effect on the gut microbiome and dendritic cell (DC) functionality in elderly subjects is crucial. DCs are vital antigen-presenting cells (APCs) that orchestrate the immune response, maintaining immune tolerance to self-antigens and bridging innate and adaptive immunity. With aging, there is a shift toward nonspecific innate immunity, resulting in a decline in adaptive immune responses. This alteration raises significant concerns about managing the health of an elderly population. However, the precise impact of aging and microbiome changes on DC function and their implications in lung-associated diseases remain relatively understudied. To illuminate this subject, we will discuss recent advancements in understanding the connections between aging, gut dysbiosis, DCs, and lung diseases. Emphasizing the key concepts linking age-related gut microbiome changes and DC functions, we will focus on their relevance to overall health and immune response in elderly individuals. This article aims to improve our understanding of the intricate relationship between aging, gut microbiome, and DCs, potentially benefiting the management of age-associated diseases and promoting healthy aging.
Introduction
The microbiome consists of diverse microorganisms colonizing the skin, mucosal compartments, and the gutCitation1. The microbiome has been showing active involvement in the vital functions of the human body, such as immunity, circadian rhythmicity, metabolism, and nutritional responsesCitation2. The human immune system is a complex network present in all tissues of the human body. The immune system plays a vital role in the host’s defense against harmful exogenous and endogenous molecules for maintaining homeostasis. From an ecological perspective, the commensal microorganisms and mammals co-evolved towards homeostasis and beneficial relationshipsCitation3. It is important to maintain the proper functioning of host immunity to prevent overexploitation of resources by commensals while maintaining immune tolerance against innocuous stimulation for healthy and beneficial relationshipsCitation4. Environmental incursions such as diet, antibiotics, or changes in geography can alter the gut microbiome leading to impairment in the human-microbiome relationship. Diseases like rheumatoid arthritis (RA), metabolic syndrome, celiac diseases, malignancies, inflammatory bowel disease (IBD), and neurodegenerative disorders occur due to alterations in the microbiome populationCitation5. The cross-talk between the immune system and gut microbiome is dynamic, context-dependent, and complexCitation5.
Recent findings have demonstrated that the age-mediated inflamed microenvironment enhances autoimmune and inflammatory responses with decreased protective immune responsesCitation6. Further, there is a decline in immune responses to infection, vaccination, phagocytic activity, antigen capturing capacity, and presentationCitation7. With the advancement of age, it has been suggested that there is the possibility of gaining nonspecific innate immunity with a weakening in adaptive immune responsesCitation8. The professional APCs called dendritic cells (DCs) are vital regulators of immune responses against infectious agentsCitation8. DCs have the property of both the activation and the induction of tolerance to antigens (self/innocuous)Citation8,Citation9. The DC maturation and activation depend on antigen uptake, processing, and the delivery of the pathogen’s danger signalsCitation8. The differentiation and activation of naive T cells can only be done by DCsCitation10. The DCs express the optimum levels of MHCs and costimulatory molecules to activate naive T cellsCitation11. However, the tolerogenic DCs display a low range of costimulatory molecules and proinflammatory cytokines in contrast to immunogenic DCs. Tolerogenic DCs express high amounts of inhibitory molecules like CTLA-4, Lag-3, Tim-3, and PDL-1Citation8. The DCs maintain tolerogenic behavior by inducing anergy and clonal deletion of T-cells and the generation of TregsCitation12. Additionally, they are crucial in maintaining peripheral tolerance against self-antigensCitation13.
Immunosenescence is an age-associated immune system dysfunction characterized by alterations in several aspects of the immunity, such as loss of adaptive immune diversity and thymic involution. The major characteristics of immunosenescence are the loss of the ability to recognize antigens, a decrease in memory T cells, and persistent low-grade inflammation called inflammaging. The other features of immunosenescence are phenotypical alterations in several immune cell types. Several viruses, such as Epstein-Barr and human cytomegalovirus, influence the immune system, resulting in immunosenescenceCitation8. Agingaffects differently various subsets of DCs (plasmacytoid DCs, myeloid DCs, follicular DCs). Plasmacytoid DCs (pDCs) are known for their role in initiating an immune response to viral infection and are reported to be majorly affected by agingCitation14–16. The gut microbiota plays a significant role in inflammation by producing inflammatory mediatorsCitation17. Although there is not much evidence about the role of DCs in inflammaging, different subsets of DCs present in the gut have been reported to express pattern recognition receptors and respond to microbial products to produce inflammatory cytokines and induction of inflammatory cellsCitation18–21.
DCs initiate adaptive immunity and process antigens in the draining lymph nodes (DLNs). In mouse models of respiratory virus infections, the DCs in the lung demonstrated decreased migration to DLNs, resulting in decline in T-cell responses because of the age-dependent increase in prostaglandin D2 in lungsCitation8,Citation22. It is reported that during aging, there is an increased production of auto-antibodies against self-antigens, and the DCs from the elderly revealed increased reactivity toward human DNA, causing the increase in the production of inflammatory markers and T cell proliferationCitation23. The state of chronic inflammation during aging might be an underlying cause of various diseases and deaths that are associated with aging. Although the mechanisms that mediate chronic inflammation associated with aging are not well understoodCitation23. Future investigation should focus on understanding the chronic inflammatory states in elderly subjects.
The gut microbiome has been observed to be increasingly involved in the immune system’s development, maturation, and maintenanceCitation24. The microbiome induces peripheral tolerance by the induction of Tregs, tolerogenic DCs, and IgA-secreting B-cellsCitation5. Advancement in age is associated with alteration in the microbiome, provoking several autoimmune diseasesCitation25. Chronic inflammation and immune dysregulation persist with the loss of Firmicutes and Bacteroides and an increase in the Proteobacteria population in the gutCitation26. It is still unclear how gut dysbiosis and loss of DC tolerance occur with the advancement of age. With a focus on the latest advancements, this article sheds light on the intricate interplay between aging, gut dysbiosis, and DCs, revealing their significant impact on overall health and immune responses.
Alteration in the microbiome with aging
Microbiome alteration occurs throughout human life and plays an important role in health and well-beingCitation27. Microbiome changes with age, antibiotics use and the prevalence of diseases. DCs balance the activation and inhibition of immune responses, a unique feature of their functionality. With aging, a gradual loss of DCs tolerance is demonstrated by low expression of costimulatory molecules and proinflammatory cytokines, the deterioration in phagocytic activity, and the inability to induce Tregs, leading to increased chances of autoimmune and inflammatory disordersCitation28. The main reason behind the DC tolerance is the enhanced proinflammatory responses and activation of NF-κBCitation28. However, the mechanism regarding age-associated DC tolerance is not clear.The studies are required to find out the reasons behind this phenomenon. Contradictory observations have been reported on the differences in the microbiome between young and old subjects, especially the presence of Bifidobacterium, Ruminococcus, and BacteroidesCitation27.
In addition to alterations in the microbiome, dietary factors play a pivotal role in maintaining the homeostasis of the immune system. Research has yielded conflicting findings regarding the impact of specific dietary components, such as carbohydrates, proteins, fatty acids, and phytochemicals, on the aging immune system. These components can exhibit both detrimental and beneficial effects on immune function. When it comes to fatty acids, their influence on pro-inflammatory markers in DCs hinges primarily on the type of fatty acid employedCitation29,Citation30. Some studies have suggested that the elderly residing in Mediterranean (MED) regions often enjoy good health, which is frequently attributed to their adherence to MED diets. Further, it has been reported that a MED diet could potentially enhance immune responses in elderly individuals, particularly by positively affecting DC functionCitation29. The study, involving 120 elderly subjects over the age of 60, revealed that intervention with the MED diet had the potential to mitigate age-associated increase in the secretion of resistin, a marker associated with agingCitation29. These findings emphasize the essential role of diet in immune system function and the importance of identifying suitable dietary choices for the elderly to prevent undesirable immune reactions. Further investigation into the potential beneficial effects of the MED diet in larger cohorts across various regions, especially among the elderly, is warranted.
Reports indicating the significant impact of diet on gut microbiota suggest that elderly individuals (aged >65) often exhibit altered dietary patterns, leading to decreased levels of Firmicutes and Bifidobacterium, as well as an increased abundance of Clostridium and Bacteroides Citation31,Citation32. However, it is important to note that these findings may not universally apply across different regions worldwide due to the substantial heterogeneity in dietary habits. This summary aims to outline the role of diets that promote gut health. For instance, the MED diet, primarily characterized by high consumption of fruits, vegetables, whole grains, and legumes, and reduced intake of meat, fish and lactose-rich products has been associated with beneficial effects on gut microbiota. It enhances gut diversity by increasing Bacteroides and Firmicutes while reducing ClostridiumCitation33. An oriental diet rich in soy protein has also been reported to have a positive impact on the gut flora, particularly by promoting Bacteroides, Proteobacteria, Bifidobacterium and Enterococcus, while reducing the presence of Firmicutes and LactococcusCitation34. Furthermore, recent studies have highlighted the influence of specific dietary components, such as fibers, saturated fats and polyphenols on gut microbiota composition. Fiber-rich foods, for instance, have been found to enrich Bifidobacterium and Lactobacillus while reducing pathogenic coloniesCitation35. On the other hand, saturated fats tend to increase Firmicutes and Proteobacteria but decrease BacteroidesCitation36. Polyphenols, on the other hand, have been associated with a positive impact on Bifidobacterium abundance while decreasing Firmicutes and Clostridium Citation37.
A study in Italy reported that with aging, a different microbiota population of Lachnospiraceae, Bacteroidaceae, and Ruminococcaceae families dominate, which usually decreases as age advancesCitation38. A study from China reports a negative correlation between aging and the persistence of Faecalibacterium, Roseburia, and Coprococcus generaCitation39. The human body is densely populated with Archaea, Eukarya, Bacteria, and viruses, in which four bacterial phyla of Firmicutes, Proteobacteria, Bacteroides, and Actinobacteria contribute to 98% of the microorganismsCitation26.
The microbiota produces short-chain fatty acids (SCFA) from the undigestible fibersCitation40. The secondary bile acids (BAs) modulated by the gut microbiome play an essential role in host metabolism and energy balance, primarily through their interactions with nuclear receptors and G protein-coupled receptors (GPCRs). It has been reported that BAs also exert an influence on the composition of the gut microbiome. Moreover, emerging evidence suggests that BAs are of critical importance in the regulation of immune responses via their interactions with nuclear receptors and GPCRs. Notably, studies have proposed that the restoration of gut BAs can alleviate experimental autoimmune uveitis (EAU) in animal models, primarily through the suppression of NF-κB-associated inflammatory cytokines in DCsCitation41. Furthermore, investigations have demonstrated that DCs treated with BAs exhibit reduced levels of IL-12 and TNF-α in response to bacterial antigens and stimulation. Additionally, BAs induce the differentiation of IL-12 hypo-producing DCs from monocytes through the TGR5/cAMP signaling pathwayCitation42. These findings collectively suggest that the loss of BAs may lead to reduced DC tolerance and contribute to developing autoimmune and inflammatory diseases. Notably, the restoration of BAs has shown promising results, underscoring the requirement for optimal BA levels in maintaining proper immune system functionCitation41.
In addition to SCFAs, the gut microbiota abundantly produces various other metabolites, such as LPS, butyrate, propionate, secondary bile acids (e.g., deoxycholic acid), and protein metabolites (e.g., p-cresol sulfate, spermidine, spermine). These metabolites have been shown to impact the immune compartment, particularly DCs. Butyrate, propionate and deoxycholic acid have been demonstrated to inhibit DC development by downregulating the expression of costimulatory molecules and pro-inflammatory cytokines, reducing chemokine expression, and promoting the induction of Tr1 and TregsCitation41–45. The LPS produced by the gut microbiota exhibits pleiotropic effects on the immune compartment. Gut-derived LPS has been reported to enhance the migratory capability of DCs, while p-cresol sulfate has been found to impede DC migration during airway inflammationCitation46,Citation47. Another metabolite, spermidine, exerts an immune-suppressive effect by enhancing the activation of indoleamine 2,3-dioxygenase (IDO), p-Src, and FOXO3, while inhibiting NF-κB activation when co-cultured with DCsCitation48,Citation49.
Microbiota plays an essential role in food digestion throughout the alimentary canal. In addition, the digestive slurry gets mixed with microbiota for synthesizing, absorbing, and extracting metabolites and nutrientsCitation40. The functions of SCFA are diverse such as regulating immune cells (activation of CD8+ T and differentiation of CD4+ T cells), controlling microbial functions, maintaining intestinal integrity, microbial energy source, and combating pathogensCitation26.
The studies on the microbiome can be classified into two categories i) alterations in gut microbiota composition with age; ii) changes in the microbiome along with aging disorders (). From these two categories, the major finding is literature-based recognition of specific groups of taxa that demonstrate changes with aging, whether healthy or unhealthyCitation50.
Table 1. Changes in the microbiome of aged subjects related to the aging disorder.
Recent studies reported the relative differences in the population of Clostridium cluster XIVa, Escherichia, Shigella, Blautia, Faecalibacterium, Ruminococcaceae, Lachnospiraceae, and Erysipelotrichaceae in aged subjects of more than 100 yearsCitation26. Some investigations suggested that the gut gets enriched with Proteobacteria and Bacteroidetes and drops in Lactobacilli and Bifidobacteria as age advances. Understanding these age-related changes may inspire targeted interventions for healthy aging and disease prevention. It was revealed that the Bifidobacteria adhered more to the intestinal mucosa in infants and young adults than in old subjectsCitation51. Such studies reveal that the aged subjects have less ability to adhere and colonize Bifidobacteria. The intestinal mucosa may lose adhesion property (decay in adhesion proteins), which might be the reason behind the failure in colonization. The Bifidobacteria, which are more sensitive to changes in the intestinal mucosa adhesion property (decreased affinity), may be lost, although the exact mechanism is yet to be identified. Bifidobacteria use lipoteichoic acids (LTA) for adhesion to the intestinal mucosaCitation52. The different Bifidobacteria groups might express LTA to different levels, and other microorganisms may compete with it for adhesion to the intestinal mucosa. Probiotic Bifidobacterium having good adhesive characteristics might help elderly subjects with their colonization in the gut. Akkermansia, Christensenellaceae, Oscillospira, and Bifidobacterium are health-associated bacteriaCitation38.
Christensenellaceae and Oscillospira have been suggested to control leanness and decrease inflammatory disorders in elderly subjectsCitation53. Akkermansia muciniphila has been demonstrated to control metabolic and inflammatory diseases, protect epithelial integrity, and support SCFA-secreting bacteriaCitation54. SCFA and lactate produced by Bifidobacterium help reduce inflammatory microbesCitation26. A study on 371 subjects comprising newborn babies and centenarians revealed an aging-associated increase of Oscillospira compared to middle-aged adults and children. Some beneficial bacteria are lost while advancing with age progressionCitation55. The microbiome alteration occurs in old age despite the influence of external environmental factors such as medications, sedentary lifestyles, diet, exercise, inter-individual variation, and geographical locations.
Influence of gut-dysbiosis on DCs
The DCs play an important role in tolerance by acting as a connecting bridge between adaptive and innate immunityCitation7. The tolerogenic DCs control the Tregs and effector T cell responsesCitation56. The function of DCs, particularly regulatory, gets impaired as age advances and with the loss of beneficial gut microbiotaCitation7. The unique characteristic of DCs is the induction of Th1 response in the presence of infection or Tregs in the absence of any infections. The CD103+ DCs in mice regulate the balance between Th1 cells and Tregs via the p38-MAPK signaling pathway during their differentiation from naïve T cellsCitation57. The DCs may change the T cell phenotype by alteration of danger signals, recruitment of inflammatory cytokines, or changed conditioning. The gut microbes use LTA/polysaccharides or DNA to cross-talk with the intestinal mucosa and produce antibacterial effects against pathogenic microorganisms by conjugated linoleic acid and bacteriocinsCitation58.
Microorganisms can modulate the function of DCs by interacting with the toll-like receptors (TLRs) expressed by DCsCitation59. The different microorganisms network differently with DCs through distinct TLRsCitation60. The DCs (CD10+ CD11b+) expressing TLR5 cross-talk with bacterial flagellin to induce Th17 cellsCitation59. The production and development of the Th17 cell anti-microbial peptide RegIIIγ immune response depend on activating the DCs that produce IL-23 and IL-6Citation18. The DCs can also be activated via TLR7 agonist to generate CD8+ T cell responses in vivoCitation61,Citation62. The gut microbiota co-evolved with the host, which renders the APCs to protect from infectious agents while maintaining self-tolerance with gut microorganisms. The best example of tolerance is that the DCs of the spleen produce low levels of IL-10, whereas the DCs of Peyer’s patches secrete more IL-10 under similar conditionsCitation63. There is a pivotal role of gut microorganisms in regulating the development and maturation of APCs, particularly DCs. Research studies revealed that in the germ-free (GF) mice, the DCs population decreased but not in the systemic circulation. Interestingly, gut colonization with Escherichia coli in the GF mice recruited the DCs to the intestinesCitation64. DCs expressing CXCR1 and CD70 get activated through microbe-derived ATP, which induces Th17 cellsCitation65.
The microbial metabolites influence the DC’s development by altering bone marrow (BM) hematopoiesis, which changes the type/phenotype of DCs in the airways and lungs. The DC number in lymph nodes and spleen remains unchanged in germ-free mice compared to specific pathogen-free mice (SPFM)Citation66. The studies conclude that microbiota dysbiosis does not impact the steady-state generation of DCs. This does not suggest that the gut microbiota does not affect DCsCitation66. The treatment of SCFAs derived from microbiota has demonstrated the generation of DC progenitors and influences their developmentCitation66. From the findings, it is evident that gut dysbiosis has a negative influence on the DCs functions. The maintenance of DC tolerance is crucial for homeostasis. It is important to find microbial species and their metabolites that can recover the loss of DC tolerance. As the microbiome is diverse with many organisms, sophisticated methods should be inculcated to identify the species responsible for DC tolerance and maintenance. In the case of lung disorders such as COPD, infections, and cystic fibrosis, the DCs play a crucial role. Saccharomyces, Lactobacillus, and Bifidobacterium are the most commonly used probiotics as supplements in infectious diseases, IBD, and colon cancer. Probiotics act as immunomodulatory agents that activate the defense pathways in various respiratory disordersCitation67.
The development of probiotic formulations will help in managing old-aged subjects suffering from lung disorders. The involvement of microbial metabolites and their functionality in various lung disorders is paramount such as SCFA metabolites have proven beneficial effects in treating several lung diseases. The susceptibility to lung disorders due to gut dysbiosis leads to a diminished production of SCFAs. With the advancement in research findings, SCFA producers could be formulated as probiotic supplements for managing lung disorders. Future investigations should focus on whether SCFA could be formulated for sustained release in lung disorders. The probiotics have shown promising results in stimulating the DC regulatory functions by targeting specific pathogen recognition receptors (PRR) and signaling pathwaysCitation68. Such results are not only for immune functionality but can be interventional in inflammatory bowel diseaseCitation68. Such studies show promise in potentially restoring the impaired tolerogenic behavior of DCs due to aging and gut dysbiosis. Given that aging and gut dysbiosis can trigger autoimmunity, further investigation into probiotic treatments is warranted, particularly in the context of autoimmune and lung disorders.
DCs dysfunction with age
Aging leads to inflammation, autoimmunity, immunodeficiency, infection susceptibility, and weak/non-response to vaccinesCitation15. This suggests that the immune response against infectious agents decreases during aging, and immune reactivity against endogenous molecules becomes more prominent because of increased inflammatory response. Further, the decrease in immune tolerance and loss of tissue integrity gives rise to new antigens and autoimmune immune reactivity through molecular mimicryCitation69. The continuous impairment in the functions of immune cells in aged subjects compared to young subjects are the major reason for morbidity and mortality.
With significant advancements in molecular and cellular mechanisms in science, there is still much to unravel concerning the changes associated with aging-mediated immune dysfunction and chronic inflammation. A recent study reported that age-related impairment of DC function leads to immune dysfunction, such as a loss of DC tolerance and increased chronic inflammationCitation70. DCs express pathogen-sensing receptors like Toll-like receptors (TLRs), C-type lectin receptors (CLRs), and NOD-like receptors (NLRs). A comparison between young and elderly populations revealed a decrease in the expression of TLR1, TLR2, and TLR8 in classical DCs (cDCs) among the elderly, while TLR2 expression remained unchanged. Additionally, TLR7 was downregulated, with no change observed in TLR9 expression in plasmacytoid DCs (pDCs) in aged individualsCitation71. Another group discovered that both TLR7 and TLR9 expressions were decreased in pDCs, leading to a selective influence on the declining percentage of pDCs during healthy aging, while no association was found with any alteration in myeloid DCs (mDCs)Citation72. Interestingly, a study involving children showed that the number of pDCs decreased by 2.5-fold in the first 10 years of life, while mDCs remained unaffectedCitation73. These findings suggest that specific types of cells undergo alterations during aging, warranting further investigation to understand this phenomenon in greater depthCitation73. However, there have been contrasting results published regarding pDCs and aging. While some studies have demonstrated a decline in the number of pDCs with ageCitation72,Citation74,Citation75, other investigations claim no change in pDC levelsCitation76. It is essential to conduct more comprehensive research to unravel the intricacies of age-mediated immune dysfunction and its impact on DCs. Further studies should focus on understanding the underlying mechanisms responsible for the observed changes in different types of DCs during the aging process, potentially leading to new therapeutic interventions targeting age-related immune dysfunction and inflammation.
The maintenance of self-tolerance is a crucial function of DCs. These cells are consistently exposed to antigens produced from damaged tissues and dead cells. Although the DCs take up these self-antigens, they remain inactive and express low levels of costimulatory markers. Consequently, they fail to present the antigens to T cellsCitation28. Presenting self-antigens to T cells without costimulatory signals results in T cell anergy, a state of unresponsiveness. However, during inflammation or tissue injury, the DCs may become activated and present self-antigens along with costimulatory signals to T cells, leading to autoimmunity. Aging contributes to impaired function, loss of homeostasis, and increased susceptibility to death. Aging reduces DC tolerance, leading to gut dysbiosis and inflammation. This impacts mental well-being and heart health and causes tissue damage. Lung and autoimmune disorders also become more common with aging and gut dysbiosis. Understanding and addressing these changes is vital for healthy aging. The elevated basal level of NF-κB in the DCs of the elderly indicates that these DCs are in an activated stateCitation28. Similarly, we observed an increased level of NF-κB in the DCs of old mice compared to young miceCitation7. After apoptosis, self-DNA is released and phagocytosed by DCs. In the elderly, DCs have a reduced capacity to uptake apoptotic cells, leading to defective clearance and immune responses instead of tolerance against self-antigensCitation28.
During viral pneumonia, DCs fail to migrate into aged lungs and from lungs to lymph nodes, rendering them unable to prime naïve T cells against the influenza virusCitation77. Reports suggest age-related defects in DCs presenting antigens via MHCI to prime CD8+ T cells, with diminished production of IL-1β and inflammasome activationCitation78. The impact of antibiotics on lung microbiota is not well-studied, although it has been established that antibiotics alter the microbiome and lung functions in cystic fibrosisCitation79. Some studies indicate that antibiotics increase the death rate in animals infected with S. pneumoniae and Influenza A virusCitation80. The influence of antibiotics and age-related gut dysbiosis on DC function requires thorough examination, and appropriate probiotic preparations should be investigated in disease models. Supplementation of depleted beneficial bacteria with age through diet is essential for maintaining homeostasis. Recent research has shown that bacteriotherapy holds promise against frailty and unhealthy agingCitation81. External factors such as drug therapy, exercise, and diet play crucial roles in healthy aging and increasing life expectancyCitation81. Moreover, the intestinal microbiota is considered a major factor in the anti-aging processCitation81. Future studies should focus on elucidating the mechanisms involved in the anti-aging process for a better understanding. The elderly population is an important part of society and is more susceptible to various diseases. Therefore, the scientific community needs to investigate better therapies that can improve the quality of life during the later stages of life.
The modulation in the function of DCs in the aged population
Dendritic Cells (DCs) in the infected aged community have been found to weakly induce TregsCitation82. Aged individuals are more susceptible to infections caused by Chlamydia, pneumonia and influenzaCitation83. The TLR function of pDCs and mDCs becomes impaired with age more than 65 years compared to younger individuals of age between 21–30 yearsCitation71. This impairment is associated with a poor Ab response against influenza virusesCitation83. Moreover, aged individuals are more prone to chronic obstructive pulmonary diseases such as bronchitis, emphysema, and asthma. Reduced DC tolerance in the airways allows for the invasion of pathogenic agents and inflammation, increasing susceptibility to lung-associated diseases. Unstimulated DCs from aged subjects exhibit increased expression of cytokines and costimulatory molecules, resulting in heightened epithelial permeabilityCitation28. As a consequence, the functionality of other cells is compromised, leading to the activation of T cells without any infection and similar reactions may occur in the skin and gut. Infections on the airway, gut, and skin surface are more common in aged subjects due to the loss of integrity of cell-to-cell junctionsCitation84.
The gut microbiome synthesizes Short-Chain Fatty Acids (SCFA), such as butyrate, acetate, and propionate, which are crucial metabolites for normal body homeostasis. The percentage of SCFA declines in aged subjects compared to young subjectsCitation85. SCFA induces Tregs, prevents DC activation, and maintains DC tolerance in the gutCitation86. DCs sense SCFA metabolites through special receptors like GPCRs (GPR109A, GPR41, and GPR43), which have different affinities and specificity toward particular metabolitesCitation86. Several immune cells, including Tregs, neutrophils, and macrophages, express GPR43 and GPR109A, which play roles in regulating microbiome homeostasis by binding to butyrate and nicotinic acid, acting as anti-inflammatory moleculesCitation87. Studies also suggest that propionate and GPR41, a receptor for SCFA, play a crucial role in generating DC and macrophage precursorsCitation88.
Infections caused by Clostridium difficile and Helicobacter pylori in the gut are dangerous in aged subjects, leading to hospitalizationCitation89. As people age advances, microbiome alteration occurs in parallel, with an increase in Enterobacteriaceae, Gram-negative, and other pathogenic bacteria in the gutCitation89. The LPS secreted by Gram-negative bacteria activates APCs like DCs and macrophages, leading to inflammationCitation70. The increase in LPS-secreting Gram-negative bacteria is one of the reasons behind inflammation in aged subjects. Therefore, appropriate probiotics should be supplemented in elderly subjects to balance the healthy gut microbiome population and avoid inflammatory reactions.
Infections such as SARS-CoV-2 and influenza impair APCs, TLRs, cytokines, and T cells, resulting in poor immune responsesCitation90. Age-mediated gut dysbiosis weakens the function of DCs and T cells, making old-aged subjects susceptible to lung infections. The supplement of probiotics regulates immune responses via modulation of the TLR signaling pathway, thereby regulating NK cells, DCs, and Th1 and Th2 immune responsesCitation90. Probiotics have demonstrated preventive effects on upper respiratory infections, improved outcomes, and reduced disease duration and severity in children and adultsCitation91.
Numerous probiotics have been investigated in clinical trials in elderly subjects to improve immune responses against influenza vaccination and infections. L. plantarum (CECT7315/7316) has shown an immuno-stimulating effect and might improve immune responses against the influenza virus in old subjectsCitation92. Additionally, two randomized clinical trials have revealed that daily administration of specific probiotic preparations enhances specific Ab responses against influenza vaccination in old-aged subjects (70 years old), suggesting potential beneficial effects for managing lung infectionsCitation93. However, evidence suggests that not all probiotic preparations may improve protection against respiratory infectionsCitation90. Some investigations have shown no significant effect of probiotics in decreasing respiratory infectionsCitation94. For instance, a study focused on 737 healthy old subjects reported that administration of Lactobacillus casei for 176 days did not improve respiratory symptoms or show positive immune responses in vaccinated individuals compared to controlsCitation95. Therefore, it is crucial to identify bacterial species that can reverse regulatory DC functions and prevent the occurrence of diseases in aged subjects.
Molecular mechanisms in DC dysfunction during aging
Molecular mechanisms underlying DC dysfunction during aging are not fully understood, but NF-κB signaling plays a crucial role. NF-κB, the master regulator of inflammation, is implicated in various diseases, including autoimmunity and inflammatory disordersCitation96,Citation97. Overexpression of NF-κB subunit RelA/p65 induces a senescent phenotype in cells, while low expression delays age-associated diseases in in vivo models (Citation98,Citation99. Our group reported that age and gut dysbiosis lead to increased p65 phosphorylation in murine models, and Lactobacillus planetarium supplementation can prevent overt NF-κB activation, which is associated with DC maturationCitation7. Other studies also demonstrate increased basal activation of NF-κB/PI3K in monocyte derived dendritic cells (MODC) and circulatory DCsCitation23,Citation71. The exact cause of DC dysfunction, whether external or intrinsic, remains unclear. Age-associated increases in prostaglandins and pro-inflammatory cytokines in circulation can trigger DC maturation and cytokine secretion even in the absence of diseaseCitation100,Citation101. Additionally, an increase in bone marrow fat levels in old age may contribute to cytokine release, further promoting DC maturation and activationCitation100,Citation101.
Figure 1. (a) Age-related changes in the gut microbiota affect DCs. As individuals progress through the aging process, significant alterations occur within the gut microbiome. These changes are characterized by an augmented presence of Gram-negative bacteria, which, in turn, leads to an increased secretion of LPS. The LPS exerts its influence via TLR4 on DCs, thus initiating a complex signaling cascade. This cascade involves critical components such as MYD88, AKT, PI3K, and MAPK pathways, ultimately culminating in the activation of the transcription factor p65 and a subsequent elevation in the basal activity of NF-κB. The heightened activation of NF-κB within DCs results in a noteworthy upregulation of proinflammatory cytokines and the augmentation of essential costimulatory molecules, including CD80, CD86, MHCII, and CD40. Concurrently, this activation leads to the downregulation of immune checkpoint proteins. These activated DCs then proceed to present antigens bound to MHC molecules and engage in interactions with T cells through the TCR, thus facilitating immune responses. This shift in the equilibrium of immune signaling makes DCs more inclined to mount vigorous inflammatory responses. Consequently, these activated DCs evolve into proficient antigen captors and presenters to T cells, thereby promoting immune responses against potential threats. However, this heightened state of immune activation also brings about a potential loss of DC tolerance, rendering the immune system more susceptible to autoimmune reactions and the development of chronic inflammatory diseases. The maintenance of a harmonious and balanced immune response is paramount for healthy aging, as the dysregulation of DC function can significantly contribute to a spectrum of age-related pathologies. One promising approach to ameliorate the adverse impacts of gut dysbiosis on DCs involves the supplementation of SCFAs. SCFAs are microbial metabolites generated through the fermentation of dietary fibers within the gut. These compounds have been demonstrated to antagonize TLR inflammatory pathways, thereby offering a potential means to safeguard DC tolerance and sustain a more balanced and harmonious immune response. (b) Aging induces various changes in the human body. Throughout the aging process, the human body undergoes a multitude of transformations, encompassing significant shifts within the gut microbiome. These alterations wield a profound influence on the immune system, with a particular emphasis on DCs, pivotal regulators of immune tolerance. DCs play a critical role in the recognition and presentation of antigens to T cells, thus regulating immune responses. The sustained activation of DCs in the elderly populace can precipitate adverse consequences, given that these cells progressively lose their ability to uphold immune tolerance toward self-antigens. Consequently, this loss of tolerance manifests as chronic inflammation, autoimmunity, and tissue damage. The unrestrained expression of costimulatory molecules and the presentation of self-antigens by DCs to autoreactive T cells usher in a disarray of immune responses within aging individuals, fostering inflammation, disruption of neuronal cell architecture, and tissue damage associated autoimmune diseases. It is worth noting that age-related maladies can potentially be ameliorated through the judicious supplementation of probiotics and SCFAs. Probiotics, being beneficial microorganisms, can reinstate equilibrium within the gut microbiome and foster the tolerogenic behavior of DCs. By modulating the functions of DCs, probiotics hold the promise of mitigating inflammation and enhancing immune tolerance, thereby opening a promising avenue for the management of age-related health conditions. The artwork used in this figure was adapted from Servier Medical Art (http://servier.com/Powerpoint-image-bank). Servier Medical Art by Servier is licensed under aCreative Commons Attribution 3.0 Unported License.
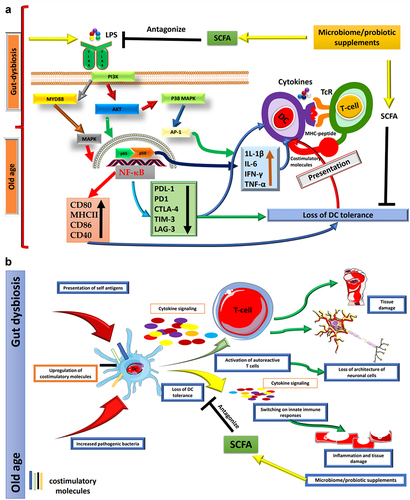
Epigenetic modifications, including DNA methylation and chromatin alterations, can also impair DCs during the aging process. In older individuals, inhibitory H3K9 histone proteins exhibit an increased binding affinity to IFN type I and III promoters in myeloid DCs. This leads to a reduction in IFNs production, particularly in the context of viral infections such as influenzaCitation102. Further investigation is essential to delve into intrinsic alterations within DCs in elderly subjects. This exploration should encompass the examination of factors like miRNAs, epigenetic markers, and various signaling pathways (such as mTOR/PI3K/PTEN/Hedgehog/NrF2/Wnt) to gain a comprehensive understanding of molecular-level changes.
Microbial metabolites influence DCs through various pathways, altering bone marrow hematopoiesis and influencing DC development in different body partsCitation88. Probiotics, like the VSL3 preparation containing specific bacterial strains, have shown promising results in ulcerative colitis patientsCitation103. The protective activity was mediated through inhibition of the PI3K/Akt and NF-κB axis, leading to reduced pro-inflammatory cytokines and increased anti-inflammatory cytokines in colonic tissueCitation104.
Probiotics have demonstrated the potential to restore DC functionality. The VSL3 probiotic preparation showed protective effects against wild-type colitis but not in TLR9 knockout mice, indicating that gut microbiota acts through specific TLR receptors on DCs or other APCsCitation105. Bacterial metabolites and pathogen-associated molecular patterns (PAMPs) often act via TLR pathways, which are interlinked with inflammatory signaling cascades. Dysbiosis in the gut increases inflammatory signaling, resulting in the loss of immune tolerance in DCs. SCFA metabolites, on the other hand, can antagonize TLR4 signaling, offering probable future therapies for various inflammatory conditions. Research should now focus on elucidating the pathways of microbiome metabolites and evaluating their therapeutic potential against lung-associated diseases.
Influence of gut microbiota dysbiosis on the health of aged subjects
Hippocrates (460–370 BCE), the father of modern medicine, proposed that “All Diseases Begin in the Gut,” a concept still relevant todayCitation106. Gut dysbiosis, a natural occurrence in old subjects, can lead to various serious consequences, including loss of DC tolerance, susceptibility to infections, reduced bone mass index, neurodegenerative diseases, autoimmune diseases, and inflammatory diseasesCitation26.
Clinical studies using fecal samples have investigated gut dysbiosis in young (28–46 years) and old (>65 years) subjects. In old subjects, the phylum Firmicutes was dominant at 40%, and phylum Bacteroidetes was at 57%, whereas in young subjects, Firmicutes was 51% dominant, and Bacteroidetes was 41%Citation106. Another investigation reported a decline in the Firmicutes/Bacteroidetes ratio from 10.9 to 0.6 between young (25–45 years) and old (70–90 years) subjectsCitation107. The ratio of Firmicutes and Bacteroidetes was higher (9-fold) in old mice/rats than in young animals, with the old animals demonstrating anxiety behaviors and cognitive impairmentCitation108. The gut-brain axis, linking the gut microbiome to brain functioning, has gained attention, revealing links between the gut microbiome and various body parts in physiological and pathological aspects. Evidence suggests that gut microbiome dysbiosis can influence brain functions and increase the risk of neurological diseases like Alzheimer’s disease, substance use disorders (SUDs), COPD, and Parkinson’s diseaseCitation109,Citation110.
Aging leads to changes in the gut microbiome, increasing Gram-negative bacteria and LPS secretion. This activates DCs through the TLR4 pathway, leading to NF-κB activation and pro-inflammatory cytokine upregulation. DCs become more efficient at presenting antigens to T cells but may lose tolerance, promoting autoimmune reactions and inflammation. SCFA supplementation could help maintain a balanced immune response and mitigate the effects of gut dysbiosis on DCs (). Gut dysbiosis is also being studied in the context of inflammatory bowel disease (IBD). Although pathogenic bacteria were initially proposed as the cause of IBD, current research suggests that gut dysbiosis may play a major role in its pathogenesisCitation111. The rising incidence of cancer in older adults may be linked to gut dysbiosis. By 2035, there is expected to be a 60% increase in cancer cases in older adults, with more than 14 million casesCitation112. Some bacteria, such as Bifidobacterium pseudopodium, Lactobacillus johnsonii, and Olsenella species, are being explored for their potential in treating colorectal cancer (CRC). Probiotics are also under investigation in clinical trials for managing CRCCitation113. Probiotics and immune checkpoint inhibitors are being used to enhance therapeutic potential against various types of cancers.
Aging impacts the gut microbiome and DCs, thereby affecting immune tolerance. Persistent DC activation leads to chronic inflammation and autoimmune diseases. Probiotics and SCFAs offer potential solutions to restore gut balance and promote DC tolerance, managing age-related conditions (). Age-associated lung diseases can be managed with probiotics, exercise, proper diet, and bacterial-associated metabolites. Healthy aging is essential to prevent age-associated diseases, as the elderly population often relies on various therapeutics. Lifestyle factors such as diet, exercise, and water intake play a significant role in disease prevention in old age. Importantly, managing age-associated diseases requires a proactive approach to maintain a healthy lifestyle and prevent the occurrence of these conditions.
Role of aging in the gut-lung axis
During aging, physiological changes in the lung lead to a decline in lung function capacity and homeostasis. Lung-associated diseases such as tuberculosis, cystic fibrosis, pneumonia, chronic obstructive pulmonary disease (COPD), and pulmonary fibrosis become more prevalent with ageCitation114. The occurrence of lung disease during aging is influenced by immunological, cellular, and physiological changes. Understanding age-associated alterations in lung physiology is essential for comprehending the healthy aging processCitation114. Healthy lung status is considered a predictor of aging, with subjects having healthy lungs living longer compared to those with poor lung functionality who are more prone to diseases such as cardiovascular diseases, diabetes, and cognitive declineCitation64.
The nose, gut, and throat microbiota develop coordinately at the young ageCitation115. The gastrointestinal tract (GIT) and respiratory tract share a common entry of microbes in the oral cavity, leading to significant overlap between the lung and gut ecosystemCitation116. The Human Microbiome Project data revealed that 45% of the microbiome population from stools overlaps with the oral cavityCitation117. Aging results in a natural decline in lung functions due to genetic and environmental factors. Several hallmarks contribute to changes in the lung aging axis, including genomic instability, loss of proteostasis, epigenetic changes, telomere attrition, mitochondrial dysfunction, stem cell exhaustion, altered intracellular communication, deregulated nutrient sensing, and dysregulation of the extracellular matrix (ECM)Citation118. These changes lead to physiological and structural alterations in the lung. Major changes during aging include abnormalities in the structure of cilia, mucins, and antioxidants in the lining of the epithelium, as well as reduced lung muscle strength in the diaphragm, resulting in a weakening in lung functionality and increased susceptibility to pulmonary diseasesCitation114. In aged lungs, the size increases due to ECM alterations, leading to increased expiratory lung volume (ELV) and decreased elastic recoilCitation119. Previous studies have indicated that gut dysbiosis is linked to acute and chronic lung-associated diseasesCitation118. Recent research reported that 10–18% of SARS-CoV-2 subjects experienced gastrointestinal symptoms, and older people were at an increased risk of developing severe illnessCitation120. In old-aged subjects, increased epithelium permeability in the lungs and gut suggests impairment in tissue functionsCitation121.
The presence of high bacterial diversity is a hallmark of a healthy gut microbiotaCitation122. Reports indicate that subjects with chronic diseases have an increased abundance of harmful bacteria such as Escherichia and Clostridium species, along with a decrease in commensal bacteriaCitation123. The mechanisms behind these bacterial population changes are not well understood and require further investigation. For asthma subjects, metagenomic analysis of stool samples revealed decreased microbiome diversity compared to healthy subjects in the United KingdomCitation124. Additionally, SCFA-producing bacteria like Coprococcus eutactus and Faecalibacterium prausnitzii were reduced, while Eggerthella lenta and Clostridium species increased in asthma subjectsCitation124.
In lung diseases like cystic fibrosis (CF), gut dysbiosis and intestinal inflammation are frequently reported due to antibiotic use in both young and old subjectsCitation125. Antibiotic use can disrupt the gut microbiome and lead to various ailments. The gut-lung axis has been demonstrated in CF with pathogenic colonization in the lungs and a decrease in the beneficial microbiome population. Metagenomics analysis revealed that in CF subjects, Propionibacterium acnes, Clostridium difficile, and Staphylococcus spp were more abundant in stools than in healthy subjects, while populations of beneficial bacteria like Roseburia, Anaerostipes, Blautia, Pseudobutyrivibrio, Faecalibacterium, Subdoligranulum, Streptococcus, Dorea, and Coprococcus species were depletedCitation125. Considering the microbiota while prescribing antibiotics in clinics is crucial, and certain diets can also impact the lung microbiome, with some spiced foods having negative effects. Major causes of diseases are lack of exercise and improper diet intake. Maintaining a healthy lifestyle is essential to support the body’s natural defenses and promote overall well-being.
Fecal Microbial Transplantation (FMT) has been shown to increase the transcriptional activity of NF-κB in the lungs with lung injury in mouse models, causing an increase in TNF-α, IL-1β, and IL-6 expression, as reported in some studiesCitation126. However, the transplantation of fecal bacteria prevents the transcriptional activity of NF-κB and decreases the secretion of proinflammatory cytokinesCitation127. Some studies have demonstrated that FMT restores the gut microbiome diversity and abundance in the mouse model with pneumonia, prevents tissue damage and inflammation, and maintains the balance between Tregs and Th17 cellsCitation126,Citation127.
The diet and exercise are very important in maintaining homeostasis of body functions. Diet has been found to influence the gut microbiome. The nutrition rich in fibers, like the MED diet, promotes a healthy gut microbiome and releases butyrateCitation128. The benefits of a good diet and a healthy gut microbiome help improve lung functions in diseased conditions such as COPD, asthma, or chronic inflammatory conditionsCitation128. Some clinical studies have shown a significant and independent correlation between low exercise and low adherence to MED diets and changed pulmonary functional patternsCitation129.
Even though probiotics were quite a success, a certain drawback has emerged, e.g., transferring antibiotic-resistant genes to other microorganisms in the gut to produce harmful metabolites. Hence, exploring other alternatives like prebiotics, synbiotics (a mixture of probiotics and prebiotics), and fecal FMT becomes necessary. Applying prebiotics (fructans, oligosaccharides, fructooligosaccharides, galactooligosaccharides, lactose) to enhance gut diversity has recently been explored in altering the immune compartment and their implications for various diseasesCitation130. Using prebiotics, synbiotics, and FMT to enrich gut species (e.g., Bifidobacterium) responsible for DC health may be an interesting approach to overcoming the problem of DCs associated with aging.
Role DCs in age-associated lung diseases
The epithelial lining covering the nasal passages and airways acts as a physical barrier and regulates immune responses against pathogens. DCs and airway epithelial cells (AECs) closely interact, influencing each other’s functionsCitation131. DCs release interferons during viral infections, enhancing MHCI expression on AECs to combat virus-infected cellsCitation132. Immune cells infiltrate infection sites through tight epithelial junctions, facilitated by DC-released pro-inflammatory cytokinesCitation133. AECs also impact DC function, expressing PRR for allergens and initiating pathogen-host interactionsCitation134.
LPS-mediated TLR4 signaling on AECs induces lung DC migration to mediastinal nodesCitation134. House dust mite (HDM) in mouse models triggers AECs to release CCL2 and CCL20, attracting immature DCs/monocytes to the lungsCitation131. The AEC-DC interaction is vital for Th2 immune responses against allergensCitation135. AECs release TSLP and IL-25/IL-33 upon allergen exposure, acting on DCs through OX40-OX40L cross-talk to elicit Th2 immune responsesCitation135. IL-25 induces DCs to release CCL-17, contributing to Th9-mediated immune responses during allergic inflammationCitation136.
Aging increases airway epithelium permeability, heightening susceptibility to environmental triggers and allergens, leading to inflammation and immune responses. AECs detect and respond to allergens by releasing cytokines that activate DCs. In the elderly, activated DCs produce proinflammatory cytokines, contributing to chronic airway inflammation. Dysregulated immune responses in aging airways result in a loss of immune tolerance, increasing susceptibility to lung tissue damage and hyper-responsiveness to antigens, potentially causing asthma or COPD (). Aging affects AEC and DC functions. In healthy-aged subjects, nasal epithelium exhibits reduced ciliary beat frequency and microtubular disarrangementsCitation137. Older adults have difficulty clearing teflon particles from small airways, taking over 21 days, leading to respiratory symptomsCitation138. Age-related changes in the microbiome influence metabolite concentrations that affect immune cells, leading to increased inflammation. Understanding how these metabolites regulate DC function to maintain immune tolerance is crucial. In aged subjects, DCs often exhibit inflammatory responses to microbial metabolitesCitation131. Further research should investigate whether the DCs of aged subjects become immune to gut microbial metabolites or the microbiome, leading to a potential reversal of their immune system functions.
Figure 2. Aging induces significant changes in the structural and functional integrity of the airways. Aging is linked to heightened airway epithelium permeability, rendering it more susceptible to environmental challenges and allergens. This increased vulnerability can induce elevated inflammation and immune reactions within the airways. Airway epithelial cells (AECs) play a pivotal role in sensing and responding to allergens and environmental stimuli. Following allergen exposure, AECs release cytokines, including IL-25, IL-33, and TSLP, which activate DCs. DCs present antigens to T cells, thus initiating and modulating immune responses. In the context of aging, DC activation by AEC-released cytokines can lead to the generation of proinflammatory cytokines, contributing to chronic airway inflammation. Moreover, AECs release chemokines such as CCL2 and CCL20, further activating DCs and promoting the recruitment of inflammatory cells to the airways. This sequence of events can result in the production of CCL17 by DCs, associated with the recruitment and activation of Th2 cells and Th9 cells involved in allergic responses. Dysregulated immune responses in aging airways may lead to diminished immune tolerance, increasing susceptibility to lung tissue damage and heightened antigen hyperresponsiveness, which manifest to asthma or chronic obstructive pulmonary disease (COPD) in older individuals.The artwork used in this figure was adapted from Servier Medical Art (http://servier.com/Powerpoint-image-bank). Servier Medical Art by Servier is licensed under aCreative Commons Attribution 3.0 Unported License.
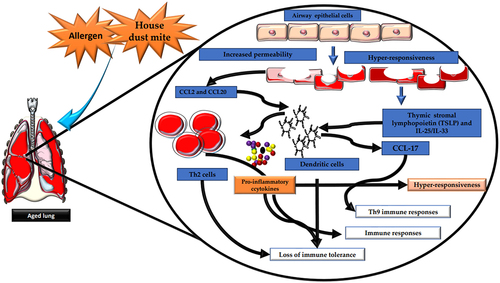
Microbiome interventions in elderly care
Our recent study revealed that DCs from old mice (DCold) mice and DCs from dysbiotic mice (DCdys) exhibited diminished tolerance compared to DC from young mice (DCyoung). Specifically, DCold and DCdys displayed reduced capacity to induce Treg generation and regulate CD4 T cell activation. We observed a notable decrease in the prevalence of the Lactobacillus genus in the gut of aged mice. To explore potential therapeutic approaches, we introduced Lactobacillus plantarum into the gut of elderly mice. This intervention successfully restored the tolerogenic function of DCs by modifying inflammatory and metabolic pathways. This novel finding sheds light on the impact of age-related gut dysbiosis on DC tolerance. Moreover, it offers promising insights into potential therapeutic strategies for addressing age-associated disorders through the use of Lactobacillus plantarumCitation7.
A microbial mixture called IRT5, comprising Bifidobacterium iridium, Streptococcus thermophilus, L. casei, L. reuteri, and L. acidophilus, has shown promising results in experimental models of various diseases. In the context of rheumatoid arthritis, atopic dermatitis, and IBD, the administration of IRT5 increased the levels of IL-10, TGF-β, and IDO in DCs, leading to the induction of TregsCitation139. Additionally, IRT5 was found to be effective in reducing body trembling and weight loss in the myasthenia gravis model by blocking T cell-dependent B cell Ab responses against acetylcholine receptors (AchR)Citation140. Furthermore, IRT5 supplementation resulted in decreased levels of AChR-specific IgG Abs, lymphocyte proliferation, and pro-inflammatory cytokines IL-17, IL-6, TNF-α and IFN-γ. DCs treated with IRT5 showed reduced production of pro-inflammatory cytokines in AChR-specific lymphocytes, accompanied by upregulation of arginase-1, RA-producing gene aldh1a2, IL-10 and TGF-β, indicating enhanced DC tolerance and induction of Tregs, which demonstrated protective effectsCitation60,Citation140. Probiotics are currently under investigation for various diseases and have shown promising outcomes in preclinical and clinical studies. Diets rich in Lactobacillus have been associated with a reduced incidence of colon cancerCitation141. Moreover, our laboratory’s research demonstrated that replenishing Lactobacillus plantarum restored tolerogenic behavior in DCs of aged and dysbiotic miceCitation7. Probiotics are also gaining interest for their potential role in altering tumor apoptosis and proliferation, offering promising alternatives to radiotherapy and chemotherapyCitation142.
Conclusion
DCs play a critical role in maintaining self-antigen tolerance, and their dysfunction significantly impacts the health of elderly individuals. With advancing age, the gut microbiome undergoes changes that profoundly affect the immune system, particularly impairing DC function. This age-mediated dysbiosis creates an inflamed microenvironment, increasing the risk of autoimmune and inflammatory responses. The immune system undergoes a series of changes during aging, shifting the balance toward innate immune responses rather than adaptive ones. Intestinal DCs are particularly affected by these aging-related alterations. Cross-talk between the gut microbiome and intestinal DCs is crucial in maintaining tolerogenic behavior. Studies are exploring probiotic preparations and immune checkpoint inhibitors for cancer management, holding promise for future therapeutic approaches. Further research is needed to address better age-related disorders like COPD, cystic fibrosis, lung infections, Alzheimer’s disease, and Parkinson’s disease.
Although we have highlighted the impact of aging and gut dysbiosis on the immune system, especially focusing on DCs and the gut-lung axis, much remains to be explored. Unraveling the underlying mechanisms responsible for the loss of DC tolerance, both intrinsic and extrinsic, warrants investigation in preclinical and clinical models. Probiotics have emerged as significant players and have demonstrated promising results in preclinical and clinical studies. Future research should concentrate on developing optimal compositions of beneficial microbes that could aid elderly subjects in managing pathophysiological conditions. Strategies for monitoring gut health could prove valuable in detecting and addressing health alterations in elderly individuals at an early stage. Given the vulnerability of older subjects to infections and chronic diseases like age-related cognitive impairments, addressing their healthcare needs becomes a crucial aspect in managing their quality of life.
Future prospects of early diagnosis of diseases and delaying aging by exploiting gut microbiota
Aging exerts a profound influence on the gut microbiota composition, consequently impacting immune system function. To comprehensively understand age-related changes in the gut microbiome, systematic studies are imperative. Unraveling the complicated relationship between specific gut microbes and their association with disease susceptibility or prevention could provide valuable insights for remedial interventions. Of particular interest is exploring the correlation between specific gut microbes and their role in disease susceptibility.
Numerous distinct hallmarks, encompassing immunosenescence, genomic instability, inflammaging, altered intracellular communication, epigenetic modifications, and telomere attrition, collectively contribute to the deterioration of immune cell toleranceCitation143. This complex cascade culminates in the establishment of a chronic inflammatory state, which plays a pivotal role in the process of lung aging (). While the phenomenon of lung aging is multifaceted and characterized by various contributing factors, the underlying mechanism remains enigmatic. Future investigations are imperative to elucidate the precise roles played by DCs and other immune cells in mediating this inflammatory state and their involvement in the overall process of lung aging.
Figure 3. Role of inflammation and other hallmarks in driving lung aging. Numerous factors, including gut dysbiosis, aging, cigarette smoking, exposure to allergens, dietary patterns, and sedentary lifestyles, collectively contribute to a spectrum of immunological phenomena. These encompass immunosenescence, genomic instability, inflammaging, alterations in intracellular communication, epigenetic modifications, and telomere attrition. This intricate web of influences invariably results in the erosion of immune cell tolerance. The culmination of these hallmarks further translates into the loss of tolerance among DCs subsequently giving rise to sustained T cell activation. Consequently, triggers an augmented production of pro-inflammatory cytokines and ROS, ultimately fostering an inflammatory state. The consequences of this chronic inflammation, in the context of the respiratory system, precipitate the phenomenon known as lung aging. The figure is party adaptedCitation143.The artwork used in this figure was adapted from Servier Medical Art (http://servier.com/Powerpoint-image-bank). Servier Medical Art by Servier is licensed under aCreative Commons Attribution 3.0 Unported Licen.
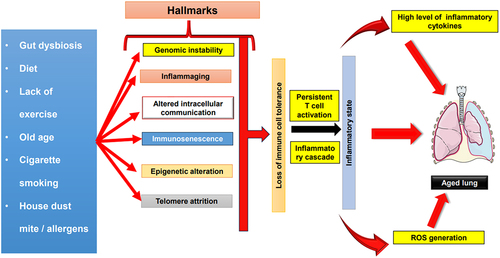
The presence or absence of certain bacteria may serve as indicative markers of disease protection or vulnerability, enabling early disease diagnosis through gut microbiome analysis. However, the cultivation of non-culturable bacteria remains a significant challenge, underscoring the need for research to identify the metabolites secreted by these microbes for potential disease treatment.
Moreover, comparative analysis of the gut microbiota in young and older adults could unveil microbes responsible for maintaining youthfulness. This intriguing concept presents the possibility of using these microbes or their products as therapeutics to delay or reverse aging and promote youthfulness, though unconventional, holding promise for future research and therapeutic interventions. Such investigations have the potential to revolutionize the field of aging and open new avenues for age-related disease management.
Acknowledgments
We are thankful to IIT Ropar for providing facilities and to the Ministry of Human Resources Development, India, for funding the project and fellowship support to JAM for pursuing his PhD.
Disclosure statement
No potential conflict of interest was reported by the authors.
Correction Statement
This article has been corrected with minor changes. These changes do not impact the academic content of the article.
Additional information
Funding
References
- Baquero F, Nombela C. The microbiome as a human organ. Clin Microbiol Infect. 2012;18(Suppl 4):2–23. doi:10.1111/j.1469-0691.2012.03916.x.
- Lynch JB, Hsiao EY. Microbiomes as sources of emergent host phenotypes. Sci. 2019;365(6460):1405–1409. doi:10.1126/science.aay0240.
- Dethlefsen L, McFall-Ngai M, Relman DA. An ecological and evolutionary perspective on human–microbe mutualism and disease. Nature. 2007;449(7164):811–818. doi:10.1038/nature06245.
- Chu H, Mazmanian SK. Innate immune recognition of the microbiota promotes host-microbial symbiosis. Nat Immunol. 2013;14(7):668–675. doi:10.1038/ni.2635.
- Zheng D, Liwinski T, Elinav E. Interaction between microbiota and immunity in health and disease. Cell Res. 2020;30(6):492–506. doi:10.1038/s41422-020-0332-7.
- Kim C, Fang F, Weyand CM, Goronzy JJ. The life cycle of a T cell after vaccination – where does immune ageing strike? Clin Exp Immunol. 2016;187(1):71–81. doi:10.1111/cei.12829.
- Bashir H, Singh S, Singh RP, Agrewala JN, Kumar R. Age-mediated gut microbiota dysbiosis promotes the loss of dendritic cells tolerance. Aging Cell. 2023;22(6): doi:10.1111/ACEL.13838.
- Lee K-A, Flores RR, Jang IH, Saathoff A, Robbins PDIS. Immune Senescence, Immunosenescence and Aging. Front Aging. 2022;3:61. doi:10.3389/fragi.2022.900028.
- Coquerelle C, Moser M. DC subsets in positive and negative regulation of immunity. Immunol Rev. 2010;234(1):317–334. doi:10.1111/j.0105-2896.2009.00887.x.
- Trombetta ES, Mellman I. Cell biology of antigen processing in vitro and in vivo. Annu Rev Immunol. 2004;23(1):975–1028. doi:10.1146/annurev.immunol.22.012703.104538.
- Hackstein H, Thomson AW. Dendritic cells: emerging pharmacological targets of immunosuppressive drugs. Nat Rev Immunol. 2004;4(1):24–35. doi:10.1038/nri1256.
- Horton C, Shanmugarajah K, Fairchild PJ. Harnessing the properties of dendritic cells in the pursuit of immunological tolerance. Biomed J. 2017;40(2):80–93. doi:10.1016/j.bj.2017.01.002.
- Hawiger D, Inaba K, Dorsett Y, Guo M, Mahnke K, Rivera M, Ravetch JV, Steinman RM, Nussenzweig MC. Dendritic cells induce peripheral T cell unresponsiveness under steady state conditions in vivo. J Exp Med. 2001;194(6):769–780. doi:10.1084/jem.194.6.769.
- Lande R, Gilliet M. Plasmacytoid dendritic cells: key players in the initiation and regulation of immune responses. Ann N Y Acad Sci. 2010;1183(1):89–103. doi:10.1111/j.1749-6632.2009.05152.x.
- Agrawal A, Gupta S. Impact of aging on dendritic cell functions in humans. Ageing Res Rev. 2011;10(3):336. doi:10.1016/j.arr.2010.06.004.
- Agrawal A, Agrawal S, Tay J, Gupta S. Biology of dendritic cells in aging. J Clin Immunol. 2008;28(1):14–20. doi:10.1007/s10875-007-9127-6.
- Franceschi C, Garagnani P, Parini P, Giuliani C, Santoro A. Inflammaging: a new immune–metabolic viewpoint for age-related diseases. Nat Rev Endocrinol. 2018;14(10):576–590. doi:10.1038/s41574-018-0059-4.
- Kinnebrew MA, Buffie C, Diehl G, Zenewicz L, Leiner I, Hohl T, Flavell R, Littman D, Pamer E. Interleukin 23 production by intestinal CD103(+)CD11b(+) dendritic cells in response to bacterial flagellin enhances mucosal innate immune defense. Immunity. 2012;36(2):276–287. doi:10.1016/j.immuni.2011.12.011.
- Fujimoto K, Karuppuchamy T, Takemura N, Shimohigoshi M, Machida T, Haseda Y, Aoshi T, Ishii KJ, Akira S, Uematsu S, et al. A new subset of CD103+CD8α+ dendritic cells in the small Intestine Expresses TLR3, TLR7, and TLR9 and induces Th1 response and CTL activity. J Immunol. 2011;186(11):6287–6295. doi:10.4049/jimmunol.1004036.
- Liu H, Chen F, Wu W, Cao AT, Xue X, Yao S, Evans-Marin HL, Li Y-Q, Cong Y. TLR5 mediates CD172α+ intestinal lamina propria dendritic cell induction of Th17 cells. Sci Rep. 2016;6(1): doi:10.1038/srep22040.
- Persson EK, Uronen-Hansson H, Semmrich M, Rivollier A, Hägerbrand K, Marsal J, Gudjonsson S, Håkansson U, Reizis B, Kotarsky K, et al. IRF4 transcription-factor-dependent CD103(+)CD11b(+) dendritic cells drive mucosal T helper 17 cell differentiation. Immunity. 2013;38(5):958–969. doi:10.1016/j.immuni.2013.03.009.
- Zhao J, Zhao J, Legge K, Perlman S. Age-related increases in PGD2 expression impair respiratory DC migration, resulting in diminished T cell responses upon respiratory virus infection in mice. J Clin Invest. 2011;121(12):4921–4930. doi:10.1172/JCI59777.
- Agrawal A, Tay J, Ton S, Agrawal S, Gupta S. Increased reactivity of dendritic cells from aged subjects to self-antigen, the human DNA. J Immunol. 2009;182(2):1138. doi:10.4049/jimmunol.182.2.1138.
- Honda K, Littman DR. The microbiota in adaptive immune homeostasis and disease. Nat. 2016;535(7610):75–84. doi:10.1038/nature18848.
- Bosco N, Noti M. The aging gut microbiome and its impact on host immunity. Genes Immun. 2021;22(5–6):289–303. doi:10.1038/s41435-021-00126-8.
- Ragonnaud E, Biragyn A. Gut microbiota as the key controllers of “healthy” aging of elderly people. Immun Ageing. 2021;18(1):1–11. doi:10.1186/s12979-020-00213-w.
- O’Toole PW, Claesson MJ. Gut microbiota: changes throughout the lifespan from infancy to elderly. Int Dairy J. 2010;20(4):281–291. doi:10.1016/j.idairyj.2009.11.010.
- Agrawal A, Agrawal S, Gupta S. Role of dendritic cells in inflammation and loss of tolerance in the elderly. Front Immunol. 2017;8:896. doi:10.3389/fimmu.2017.00896.
- Clements SJ, Maijo M, Ivory K, Nicoletti C, Carding SR. Age-associated decline in dendritic cell function and the impact of Mediterranean diet intervention in elderly subjects. Front Nutr. 2017;4:294058. doi:10.3389/fnut.2017.00065.
- Calder PC. Lipid-laden dendritic cells fail to function. Cell Res. 2010;20(10):1089–1091. doi:10.1038/cr.2010.124.
- De Clercq NC, Frissen MN, Davids M, Groen AK, Nieuwdorp M. Weight gain after fecal microbiota transplantation in a patient with recurrent underweight following clinical recovery from anorexia nervosa. Psychother Psychosom. 2019;88(1):58–60. doi:10.1159/000495044.
- Claesson MJ, Cusack S, O’Sullivan O, Greene-Diniz R, de Weerd H, Flannery E, Marchesi JR, Falush D, Dinan T, Fitzgerald G, et al. Composition, variability, and temporal stability of the intestinal microbiota of the elderly. Proc Natl Acad Sci U S A. 2011;108 Suppl 1:4586–4591. doi:10.1073/pnas.1000097107.
- Tosti V, Bertozzi B, Fontana L. Health benefits of the Mediterranean diet: metabolic and molecular mechanisms. J Gerontol A Biol Sci Med Sci. 2018;73(3):318–326. doi:10.1093/gerona/glx227.
- Tamura K, Sasaki H, Shiga K, Miyakawa H, Shibata S. The timing effects of soy protein intake on mice gut microbiota. Nutrients. 2019;12(1):87. doi:10.3390/nu12010087.
- Ticinesi A, Tana C, Nouvenne A, Prati B, Lauretani F, Meschi T. Gut microbiota, cognitive frailty and dementia in older individuals: a systematic review. Clin Interv Aging. 2018;13:1497–1511. doi:10.2147/CIA.S139163.
- Everitt AV, Hilmer SN, Brand-Miller JC, Jamieson HA, Truswell AS, Sharma AP, Mason RS, Morris BJ, Couteur DGL. Dietary approaches that delay age-related diseases. Clin Interv Aging. 2006;1(1):11–31. doi:10.2147/ciia.2006.1.1.11.
- Larrosa M, Luceri C, Vivoli E, Pagliuca C, Lodovici M, Moneti G, Dolara P. Polyphenol metabolites from colonic microbiota exert anti-inflammatory activity on different inflammation models. Mol Nutr Food Res. 2009;53(8):1044–1054. doi:10.1002/mnfr.200800446.
- Biagi E, Franceschi C, Rampelli S, Severgnini M, Ostan R, Turroni S, Consolandi C, Quercia S, Scurti M, Monti D, et al. Gut Microbiota and Extreme Longevity. Curr Biol. 2016;26(11):1480–1485. doi:10.1016/j.cub.2016.04.016.
- Wang N, Li R, Lin H, Fu C, Wang X, Zhang Y, Su M, Huang P, Qian J, Jiang F, et al. Enriched taxa were found among the gut microbiota of centenarians in East China. PLoS ONE. 2019;14(10):e0222763. doi:10.1371/journal.pone.0222763.
- Brestoff JR, Artis D. Commensal bacteria at the interface of host metabolism and the immune system. Nat Immunol. 2013;14(7):676. doi:10.1038/ni.2640.
- Hu J, Wang C, Huang X, Yi S, Pan S, Zhang Y, Yuan G, Cao Q, Ye X, Li H, et al. Gut microbiota-mediated secondary bile acids regulate dendritic cells to attenuate autoimmune uveitis through TGR5 signaling. Cell Rep. 2021;36(12):109726. doi:10.1016/j.celrep.2021.109726.
- Ichikawa R, Takayama T, Yoneno K, Kamada N, Kitazume MT, Higuchi H, Matsuoka K, Watanabe M, Itoh H, Kanai T, et al. Bile acids induce monocyte differentiation toward interleukin-12 hypo-producing dendritic cells via a TGR5-dependent pathway. Immunology. 2012;136(2):153. doi:10.1111/j.1365-2567.2012.03554.x.
- Singh N, Thangaraju M, Prasad PD, Martin PM, Lambert NA, Boettger T, Offermanns S, Ganapathy V. Blockade of dendritic cell development by bacterial fermentation products butyrate and propionate through a transporter (Slc5a8)-dependent inhibition of histone deacetylases. J Biol Chem. 2010;285(36):27601–27608. doi:10.1074/jbc.M110.102947.
- Sä MD, Parolini O, Böhmig GA, Kelemen P, Krieger P-M, Neumüller J, Knarr K, Kammlander W, Hörl WH, Diakos C, et al. Bacterial metabolite interference with maturation of human monocyte-derived dendritic cells. J Leukoc Biol. 2002;71(2):238–246. doi:10.1189/jlb.71.2.238.
- Kaisar MMM, Pelgrom LR, van der Ham AJ, Yazdanbakhsh M, Everts B. Butyrate conditions human dendritic cells to prime type 1 regulatory T cells via both histone deacetylase inhibition and G protein-coupled receptor 109A signaling. Front Immunol. 2017;8. doi:10.3389/fimmu.2017.01429.
- Wypych TP, Pattaroni C, Perdijk O, Yap C, Trompette A, Anderson D, Creek DJ, Harris NL, Marsland BJ. Microbial metabolism of L-tyrosine protects against allergic airway inflammation. Nat Immunol. 2021;22(3):279–286. doi:10.1038/s41590-020-00856-3.
- Ichinohe T, Pang IK, Kumamoto Y, Peaper DR, Ho JH, Murray TS, Iwasaki A. Microbiota regulates immune defense against respiratory tract influenza a virus infection. Proc Natl Acad Sci U S A. 2011;108(13):5354–5359. doi:10.1073/pnas.1019378108.
- Li G, Ding H, Yu X, Meng Y, Li J, Guo Q, Zhou H, Shen N. Spermidine suppresses inflammatory DC function by activating the FOXO3 pathway and counteracts autoimmunity. iScience. 2020;23(1):100807. doi:10.1016/j.isci.2019.100807.
- Mondanelli G, Bianchi R, Pallotta MT, Orabona C, Albini E, Iacono A, Belladonna ML, Vacca C, Fallarino F, Macchiarulo A, et al. A relay pathway between arginine and tryptophan metabolism confers immunosuppressive properties on dendritic cells. Immunity. 2017;46(2):233–244. doi:10.1016/j.immuni.2017.01.005.
- Ghosh TS, Shanahan F, O’Toole PW. The gut microbiome as a modulator of healthy ageing. Nat Rev Gastroenterol Hepatol. 2022;19(9):565. doi:10.1038/s41575-022-00605-x.
- Ouwehand AC, Isolauri E, Kirjavainen PV, Salminen SJ. Adhesion of four Bifidobacterium strains to human intestinal mucus from subjects in different age groups. FEMS Microbiol Lett. 1999;172(1):61–64. doi:10.1111/j.1574-6968.1999.tb13450.x.
- Op den Camp HJ, Oosterhof A, Veerkamp JH. Interaction of bifidobacterial lipoteichoic acid with human intestinal epithelial cells. Infect Immun. 1985;47(1):332–334. doi:10.1128/iai.47.1.332-334.1985.
- Waters JL, Ley RE. The human gut bacteria Christensenellaceae are widespread, heritable, and associated with health. BMC Biol. 2019;17(1): doi:10.1186/s12915-019-0699-4.
- Bodogai M, O’Connell J, Kim K, Kim Y, Moritoh K, Chen C, Gusev F, Vaughan K, Shulzhenko N, Mattison JA, et al. Commensal bacteria contribute to insulin resistance in aging by activating innate B1a cells. Sci Transl Med. 2018;10(467). doi:10.1126/scitranslmed.aat4271.
- Xu C, Zhu H, Qiu P. Aging progression of human gut microbiota. BMC Microbiol. 2019;19(1): doi:10.1186/s12866-019-1616-2.
- Hasegawa H, Matsumoto T. Mechanisms of tolerance induction by dendritic cells in vivo. Front Immunol. 2018;9. doi:10.3389/fimmu.2018.00350.
- Huang G, Wang Y, Chi H. Control of T cell fates and immune tolerance by p38α signaling in mucosal CD103+ dendritic cells. J Immunol. 2013;191(2):650–659. doi:10.4049/jimmunol.1300398.
- O’Shea EF, Cotter PD, Stanton C, Ross RP, Hill C. Production of bioactive substances by intestinal bacteria as a basis for explaining probiotic mechanisms: bacteriocins and conjugated linoleic acid. Int J Food Microbiol. 2012;152(3):189–205. doi:10.1016/j.ijfoodmicro.2011.05.025.
- Stagg AJ. Intestinal dendritic cells in health and gut inflammation. Front Immunol. 2018;9:2883. doi:10.3389/fimmu.2018.02883.
- Owen JL, Mohamadzadeh M. Microbial activation of gut dendritic cells and the control of mucosal immunity. J Interf Cytokine Res. 2013;33(11):619. doi:10.1089/jir.2013.0046.
- Malik JA, Kaur G, Agrewala JN. Revolutionizing medicine with toll-like receptors: a path to strengthening cellular immunity. Int J Biol Macromol. 2023;253:127252. doi:10.1016/J.IJBIOMAC.2023.127252.
- Cerovic V, Houston SA, Westlund J, Utriainen L, Davison ES, Scott CL, Bain CC, Joeris T, Agace WW, Kroczek RA, et al. Lymph-borne CD8α+ dendritic cells are uniquely able to cross-prime CD8+ T cells with antigen acquired from intestinal epithelial cells. Mucosal Immunol. 2015;8(1):38–48. doi:10.1038/mi.2014.40.
- Iwasaki A, Kelsall BL. Freshly isolated Peyer’s patch, but not spleen, dendritic cells produce interleukin 10 and induce the differentiation of T helper type 2 cells. The Journal Of Experimental Medicine. 1999;190(2):229–240. doi:10.1084/jem.190.2.229.
- Williams AM, Probert CSJ, Stepankova R, Tlaskalova‐Hogenova H, Phillips A, Bland PW. Effects of microflora on the neonatal development of gut mucosal T cells and myeloid cells in the mouse. Immunology. 2006;119(4):470. doi:10.1111/j.1365-2567.2006.02458.x.
- Atarashi K, Nishimura J, Shima T, Umesaki Y, Yamamoto M, Onoue M, Yagita H, Ishii N, Evans R, Honda K, et al. ATP drives lamina propria TH17 cell differentiation. Nature. 2008;455(7214):808–812. doi:10.1038/nature07240.
- Wilson KR, Gressier E, McConville MJ, Bedoui S. Microbial metabolites in the maturation and activation of dendritic cells and their relevance for respiratory immunity. Front Immunol. 2022;13. doi:10.3389/fimmu.2022.897462.
- Mortaz E, Adcock IM, Folkerts G, Barnes PJ, Paul Vos A, Garssen J. Probiotics in the management of lung diseases. Mediators Inflamm. 2013;2013:1–10. doi:10.1155/2013/751068.
- Foligne B, Zoumpopoulou G, Dewulf J, Ben Younes A, Chareyre F, Sirard J-C, Pot B, Grangette C. A key role of dendritic cells in probiotic functionality. PLoS ONE. 2007;2(3):e313. doi:10.1371/journal.pone.0000313.
- Ginaldi L, De Martinis M, Monti D, Franceschi C. The immune system in the elderly: activation-induced and damage-induced apoptosis. Immunol Res. 2004;30(1):081–094. doi:10.1385/IR:30:1:081.
- Agrawal A, Agrawal S, Cao J-N, Su H, Osann K, Gupta S. Altered innate immune functioning of dendritic cells in elderly humans: a role of phosphoinositide 3-kinase-signaling pathway. J Immunol. 2007;178(11):6912–6922. doi:10.4049/jimmunol.178.11.6912.
- Panda A, Qian F, Mohanty S, van Duin D, Newman FK, Zhang L, Chen S, Towle V, Belshe RB, Fikrig E, et al. Age-associated decrease in TLR function in primary human dendritic cells predicts influenza vaccine response. J Immunol. 2010;184(5):2518–2527. doi:10.4049/jimmunol.0901022.
- Jing Y, Shaheen E, Drake RR, Chen N, Gravenstein S, Deng Y. Aging is associated with a numerical and functional decline in plasmacytoid dendritic cells, whereas myeloid dendritic cells are relatively unaltered in human peripheral blood. Hum Immunol. 2009;70(10):777. doi:10.1016/j.humimm.2009.07.005.
- Teig N, Moses D, Gieseler S, Schauer U. Age-related changes in human blood dendritic cell subpopulations. Scand J Immunol. 2002;55(5):453–457. doi:10.1046/j.1365-3083.2002.01068.x.
- Shodell M, Siegal FP. Circulating, interferon-producing plasmacytoid dendritic cells decline during human ageing. Scand J Immunol. 2002;56(5):518–521. doi:10.1046/j.1365-3083.2002.01148.x.
- Pérez-Cabezas B, Naranjo-Gómez M, Fernández MA, Grífols JR, Pujol-Borrell R, Borràs FE. Reduced numbers of plasmacytoid dendritic cells in aged blood donors. Exp Gerontol. 2007;42(10):1033–1038. doi:10.1016/j.exger.2007.05.010.
- Della Bella S, Bierti L, Presicce P, Arienti R, Valenti M, Saresella M, Vergani C, Villa ML. Peripheral blood dendritic cells and monocytes are differently regulated in the elderly. Clin Immunol. 2007;122(2):220–228. doi:10.1016/j.clim.2006.09.012.
- Valkenburg SA, Venturi V, Dang THY, Bird NL, Doherty PC, Turner SJ, Davenport MP, Kedzierska K. Correction: early priming minimizes the age-related immune Compromise of CD8 + T cell diversity and function. PLoS Pathog. 2012;8(3): doi:10.1371/annotation/e142f9de-7f30-4759-bda1-a651e86d5ba6.
- Stout-Delgado HW, Vaughan SE, Shirali AC, Jaramillo RJ, Harrod KS. Impaired NLRP3 inflammasome function in elderly mice during influenza infection is rescued by treatment with nigericin. J Immunol. 2012;188(6):2815–2824. doi:10.4049/jimmunol.1103051.
- Inam Z, Felton E, Burrell A, Chaney H, Sami I, Koumbourlis AC, Freishtat RJ, Zemanick ET, Crandall KA, Hahn A, et al. Impact of antibiotics on the lung microbiome and lung function in children with cystic fibrosis 1 year after hospitalization for an initial pulmonary exacerbation. Open Forum Infect Dis. 2022;9(9). doi:10.1093/ofid/ofac466.
- Schuijt TJ, Lankelma JM, Scicluna BP, de Sousa e Melo F, Roelofs JJTH, de Boer JD, Hoogendijk AJ, de Beer R, de Vos A, Belzer C, et al. The gut microbiota plays a protective role in the host defence against pneumococcal pneumonia. Gut. 2016;65(4):575–583. doi:10.1136/gutjnl-2015-309728.
- Du Y, Gao Y, Zeng B, Fan X, Yang D, Yang M. Effects of anti-aging interventions on intestinal microbiota. Gut Microbes. 2021;13(1): doi:10.1080/19490976.2021.1994835.
- Agrawal S, Ganguly S, Tran A, Sundaram P, Agrawal A. Retinoic acid treated human dendritic cells induce T regulatory cells via the expression of CD141 and GARP which is impaired with age. Aging (Albany NY). 2016;8(6):1223–1235. doi:10.18632/aging.100973.
- Busse PJ, Mathur SK. Age-related changes in immune function: effect on airway inflammation. J Allergy Clin Immunol. 2010;126(4):690–699. doi:10.1016/j.jaci.2010.08.011.
- MOUTON CP, BAZALDUA OV, PIERCE B, ESPINO DV. Common infections in older adults. Am Fam Physician. 2001;63:257–268.
- Biagi E, Nylund L, Candela M, Ostan R, Bucci L, Pini E, Nikkïla J, Monti D, Satokari R, Franceschi C, et al. Correction: through Ageing, and beyond: gut microbiota and inflammatory status in seniors and centenarians. PLoS ONE. 2010;5(6). doi:10.1371/annotation/df45912f-d15c-44ab-8312-e7ec0607604d.
- Nastasi C, Candela M, Bonefeld CM, Geisler C, Hansen M, Krejsgaard T, Biagi E, Andersen MH, Brigidi P, Ødum N, et al. The effect of short-chain fatty acids on human monocyte-derived dendritic cells. Sci Rep. 2015;5(1). doi:10.1038/srep16148.
- Singh N, Gurav A, Sivaprakasam S, Brady E, Padia R, Shi H, Thangaraju M, Prasad P, Manicassamy S, Munn D, et al. Activation of the receptor (Gpr109a) for niacin and the commensal metabolite butyrate suppresses colonic inflammation and carcinogenesis. Immunity. 2014;40(1):128. doi:10.1016/j.immuni.2013.12.007.
- Trompette A, Gollwitzer ES, Yadava K, Sichelstiel AK, Sprenger N, Ngom-Bru C, Blanchard C, Junt T, Nicod LP, Harris NL, et al. Gut microbiota metabolism of dietary fiber influences allergic airway disease and hematopoiesis. Nat Med. 2014;20(2):159–166. doi:10.1038/nm.3444.
- Kumar M, Babaei P, Ji B, Nielsen J. Human gut microbiota and healthy aging: recent developments and future prospective. Nutr Heal Aging. 2016;4(1):3–16. doi:10.3233/NHA-150002.
- Shahbazi R, Yasavoli-Sharahi H, Alsadi N, Ismail N, Matar C. Probiotics in treatment of viral respiratory infections and neuroinflammatory disorders. Molecules. 2020;25(21):4891. doi:10.3390/molecules25214891.
- Hardy H, Harris J, Lyon E, Beal J, Foey AD. Probiotics, prebiotics and immunomodulation of gut mucosal defences: homeostasis and immunopathology. Nutrients. 2013;5(6):1869–1912. doi:10.3390/nu5061869.
- Bosch M, Méndez M, Pérez M, Farran A, Fuentes MC, Cuñé J. Lactobacillus plantarum CECT7315 and CECT7316 stimulate immunoglobulin production after influenza vaccination in elderly. Nutr Hosp. 2012;27(2):504–509. doi:10.1590/S0212-16112012000200023.
- Boge T, Rémigy M, Vaudaine S, Tanguy J, Bourdet-Sicard R, van der Werf S. A probiotic fermented dairy drink improves antibody response to influenza vaccination in the elderly in two randomised controlled trials. Vaccine. 2009;27(41):5677–5684. doi:10.1016/j.vaccine.2009.06.094.
- Kanauchi O, Andoh A, AbuBakar S, Yamamoto N. Probiotics and paraprobiotics in viral infection: clinical application and effects on the innate and acquired immune systems. Curr Pharm Des. 2018;24(6):710. doi:10.2174/1381612824666180116163411.
- Van Puyenbroeck K, Hens N, Coenen S, Michiels B, Beunckens C, Molenberghs G, Van Royen P, Verhoeven V. Efficacy of daily intake of Lactobacillus casei Shirota on respiratory symptoms and influenza vaccination immune response: a randomized, double-blind, placebo-controlled trial in healthy elderly nursing home residents. Am J Clin Nutr. 2012;95(5):1165–1171. doi:10.3945/ajcn.111.026831.
- Barnabei L, Laplantine E, Mbongo W, Rieux-Laucat F, Weil R. NF-κB: at the borders of autoimmunity and inflammation. Front Immunol. 2021;12. doi:10.3389/fimmu.2021.716469.
- Adler AS, Sinha S, Kawahara TLA, Zhang JY, Segal E, Chang HY. Motif module map reveals enforcement of aging by continual NF-κB activity. Genes Dev. 2007;21(24):000.1–000. doi:10.1101/gad.1588507.
- Flores RR, Clauson CL, Cho J, Lee B-C, McGowan SJ, Baker DJ, Niedernhofer LJ, Robbins PD. Expansion of myeloid-derived suppressor cells with aging in the bone marrow of mice through a NF-κB-dependent mechanism. Aging Cell. 2017;16(3):480–487. doi:10.1111/acel.12571.
- Seitz C, Deng H, Hinata K, Lin Q, Khavari P. Nuclear factor κB subunits induce epithelial cell growth arrest. Cancer Res. 2000;60(15):4085–92.
- Tilg H, Moschen AR. Adipocytokines: mediators linking adipose tissue, inflammation and immunity. Nat Rev Immunol. 2006;6(10):772–783. doi:10.1038/nri1937.
- Tuljapurkar SR, McGuire TR, Brusnahan SK, Jackson JD, Garvin KL, Kessinger MA, Lane JT, O’ Kane BJ, Sharp JG. Changes in human bone marrow fat content associated with changes in hematopoietic stem cell numbers and cytokine levels with aging. J Anat. 2011;219(5):574–581. doi:10.1111/j.1469-7580.2011.01423.x.
- Prakash S, Agrawal S, Cao JN, Gupta S, Agrawal A. Impaired secretion of interferons by dendritic cells from aged subjects to influenza: role of histone modifications. Age (Dordr). 2013;35(5):1785–1797. doi:10.1007/s11357-012-9477-8.
- Bibiloni R, Fedorak RN, Tannock GW, Madsen KL, Gionchetti P, Campieri M, De Simone C, Sartor RB. VSL#3 probiotic-mixture induces remission in patients with active ulcerative colitis. Am J Gastroenterol. 2005;100(7):1539–1546. doi:10.1111/j.1572-0241.2005.41794.x.
- Dai C, Zheng C-Q, Meng F-J, Zhou Z, Sang L-X, Jiang M. VSL#3 probiotics exerts the anti-inflammatory activity via PI3k/Akt and NF-κB pathway in rat model of DSS-induced colitis. Mol Cell Biochem. 2013;374(1–2):1–11. doi:10.1007/s11010-012-1488-3.
- Rachmilewitz D, Katakura K, Karmeli F, Hayashi T, Reinus C, Rudensky B, Akira S, Takeda K, Lee J, Takabayashi K, et al. Toll-like receptor 9 signaling mediates the anti-inflammatory effects of Probiotics in murine experimental colitis. Gastroenterology. 2004;126(2):520–528. doi:10.1053/j.gastro.2003.11.019.
- Alsegiani A, Shah Z. The influence of gut microbiota alteration on age-related neuroinflammation and cognitive decline. Neural Regen Res. 2022;17(11):2407. doi:10.4103/1673-5374.335837.
- Mariat D, Firmesse O, Levenez F, Guimarăes VD, Sokol H, Doré J, Corthier G, Furet J-P. The Firmicutes/Bacteroidetes ratio of the human microbiota changes with age. BMC Microbiol. 2009;9(1):123. doi:10.1186/1471-2180-9-123.
- Li Y, Ning L, Yin Y, Wang R, Zhang Z, Hao L, Wang B, Zhao X, Yang X, Yin L, et al. Age-related shifts in gut microbiota contribute to cognitive decline in aged rats. Aging (Albany NY). 2020;12(9):7801. doi:10.18632/aging.103093.
- Meckel KR, Kiraly DD. A potential role for the gut microbiome in substance use disorders. Psychopharmacol (Berl). 2019;236(5):1513. doi:10.1007/s00213-019-05232-0.
- Wang HX, Wang YPC. Gut microbiota-brain axis. Med J (Engl). 2016;129(19):2373–2380. doi:10.4103/0366-6999.190667.
- Degruttola AK, Low D, Mizoguchi A, Mizoguchi E. Current understanding of dysbiosis in disease in human and animal models. Inflamm Bowel Dis. 2016;22(5):1137. doi:10.1097/MIB.0000000000000750.
- Pilleron S, Sarfati D, Janssen‐Heijnen M, Vignat J, Ferlay J, Bray F, Soerjomataram I. Global cancer incidence in older adults, 2012 and 2035: A population-based study. Int J Cancer. 2019;144(1):49–58. doi:10.1002/ijc.31664.
- Wu J, Wang, S, Zheng, B, Qiu, X, Wang, H, Chen, L. Modulation of gut microbiota to enhance effect of checkpoint inhibitor immunotherapy. Front Immunol. 2021;12:2554. doi:10.3389/fimmu.2021.669150.
- Bowdish DME. The aging lung: is lung health good health for older adults? Chest. 2019;155(2):391–400. doi:10.1016/j.chest.2018.09.003.
- Grier A, McDavid A, Wang B, Qiu X, Java J, Bandyopadhyay S, Yang H, Holden-Wiltse J, Kessler HA, Gill AL, et al. Neonatal gut and respiratory microbiota: coordinated development through time and space. Microbiome. 2018;6(1):1–19. doi:10.1186/s40168-018-0566-5.
- Mathieu E, MacPherson CW, Belvis J, Mathieu O, Robert V, Saint-Criq V, Langella P, Tompkins TA, Thomas M. Oral Primo-Colonizing Bacteria Modulate Inflammation and Gene Expression in Bronchial Epithelial Cells. Microorganisms. 2020;8(8):1–19. doi:10.3390/microorganisms8081094.
- Segata N, Haake S, Mannon P, Lemon KP, Waldron L, Gevers D, Huttenhower C, Izard J. Composition of the adult digestive tract bacterial microbiome based on seven mouth surfaces, tonsils, throat and stool samples. Genome Biol. 2012;13(6):1–18. doi:10.1186/gb-2012-13-6-r42.
- Saint-Criq V, Lugo-Villarino G, Thomas M. Dysbiosis, malnutrition and enhanced gut-lung axis contribute to age-related respiratory diseases. Ageing Res Rev. 2021;66:101235. doi:10.1016/j.arr.2020.101235.
- Cho SJ, Stout-Delgado HW. Aging and lung disease. Annu Rev Physiol. 2020;82(1):433–459. doi:10.1146/annurev-physiol-021119-034610.
- Khan N, Vidyarthi A, Nadeem S, Negi S, Nair G, Agrewala JN. Alteration in the gut microbiota provokes susceptibility to tuberculosis. Front Immunol. 2016;7:218883. doi:10.3389/fimmu.2016.00529.
- Cerqueira César Machado M, Pinheiro da Silva F. Intestinal barrier dysfunction in human pathology and aging. Curr Pharm Des. 2016;22(30):4645–4650. doi:10.2174/1381612822666160510125331.
- Molinero N, Antón-Fernández A, Hernández F, Ávila J, Bartolomé B, Moreno-Arribas M Victoria. (). Gut Microbiota, an Additional Hallmark of Human Aging and Neurodegeneration. Neuroscience. 2023;518:141–161. 10.1016/j.neuroscience.2023.02.014
- Marsland BJ, Trompette A, Gollwitzer ES. The gut-lung axis in respiratory disease. Ann Am Thorac Soc. 2015;12 Suppl 2:S150–S156. doi:10.1513/AnnalsATS.201503-133AW.
- Wang Q, Li F, Liang B, Liang Y, Chen S, Mo X, Ju Y, Zhao H, Jia H, Spector TD, et al. A metagenome-wide association study of gut microbiota in asthma in UK adults. BMC Microbiol. 2018;18(1). doi:10.1186/s12866-018-1257-x.
- Vernocchi P, Del Chierico F, Russo A, Majo F, Rossitto M, Valerio M, Casadei L, La Storia A, De Filippis F, Rizzo C, et al. Gut microbiota signatures in cystic fibrosis: loss of host CFTR function drives the microbiota enterophenotype. PLoS ONE. 2018;13(12):e0208171. doi:10.1371/journal.pone.0208171.
- Song W, Yue Y, Zhang Q. Imbalance of gut microbiota is involved in the development of chronic obstructive pulmonary disease: a review. Biomed Pharmacother. 2023;165:115150. doi:10.1016/j.biopha.2023.115150.
- Wen L, Shi, L, Kong, X-L, Li, K-Y, Li, H, Jiang, D-X, Zhang, F, Zhou, Z-G. Gut microbiota protected against pseudomonas aeruginosa pneumonia via restoring Treg/Th17 balance and metabolism. Front Cell Infect Microbiol. 2022;12:856633. doi:10.3389/fcimb.2022.856633.
- Santo CE, Caseiro C, Martins MJ, Monteiro R, Brandão I. Gut microbiota, in the halfway between nutrition and lung function. Nutrients. 2021;13(5):1716. doi:10.3390/nu13051716.
- Gutiérrez-Carrasquilla L, Sánchez E, Hernández M, Polanco D, Salas-Salvadó J, Betriu À, Gaeta A, Carmona P, Purroy F, Pamplona R, et al. Effects of Mediterranean diet and physical activity on pulmonary function: a cross-Sectional analysis in the ILERVAS project. Nutrients. 2019;11(2):329. doi:10.3390/nu11020329.
- Davani-Davari D, Negahdaripour M, Karimzadeh I, Seifan M, Mohkam M, Masoumi S, Berenjian A, Ghasemi Y. Prebiotics: definition, types, sources, mechanisms, and clinical applications. Foods (Basel, Switzerland). 2019;8(3):92. doi:10.3390/foods8030092.
- Agrawal A. Dendritic cell-airway epithelial cell cross-talk changes with age and contributes to chronic lung inflammatory diseases in the elderly. Int J Mol Sci. 2017;18(6):1206. doi:10.3390/ijms18061206.
- Ank N, Paludan SR. Type III IFNs: new layers of complexity in innate antiviral immunity. Biofactors. 2009;35(1):82–87. doi:10.1002/biof.19.
- Martin LD, Rochelle LG, Fischer BM, Krunkosky TM, Adler KB. Airway epithelium as an effector of inflammation: molecular regulation of secondary mediators. Eur Respir J. 1997;10(9):2139–2146. doi:10.1183/09031936.97.10092139.
- Hammad H, Chieppa M, Perros F, Willart MA, Germain RN, Lambrecht BN. House dust mite allergen induces asthma via Toll-like receptor 4 triggering of airway structural cells. Nat Med. 2009;15(4):410–416. doi:10.1038/nm.1946.
- Lambrecht BN, Hammad H. Allergens and the airway epithelium response: gateway to allergic sensitization. J Allergy Clin Immunol. 2014;134(3):499–507. doi:10.1016/j.jaci.2014.06.036.
- Claudio E, Tassi I, Wang H, Tang W, Ha H-L, Siebenlist U. Cutting edge: IL-25 targets dendritic cells to attract IL-9–producing T cells in acute allergic lung inflammation. J Immunol. 2015;195(8):3525–3529. doi:10.4049/jimmunol.1500436.
- Ho JC, CHAN K, HU W, LAM W, ZHENG L, TIPOE G, SUN J, LEUNG R, TSANG K. The effect of aging on nasal mucociliary clearance, beat frequency, and ultrastructure of respiratory cilia. Am J Respir Crit Care Med. 2012;163(4):983–988. doi:10.1164/ajrccm.163.4.9909121.
- Svartengren M. Long-term clearance from small airways decreases with age. Eur Respir J. 2005;26(4):609–615. doi:10.1183/09031936.05.00002105.
- Kwon HK, Lee C-G, So J-S, Chae C-S, Hwang J-S, Sahoo A, Nam JH, Rhee JH, Hwang K-C, Im S-H, et al. Generation of regulatory dendritic cells and CD4+Foxp3+ T cells by probiotics administration suppresses immune disorders. Proc Natl Acad Sci U S A. 2010;107(5):2159–2164. doi:10.1073/pnas.0904055107.
- Chae CS, Kwon HK, Hwang JS, Kim JE, Im SH, Platten M. Prophylactic effect of Probiotics on the development of experimental autoimmune myasthenia gravis. PLoS ONE. 2012;7(12):52119. doi:10.1371/journal.pone.0052119.
- Goldin BR, Gorbach SL. Effect of Lactobacillus acidophilus dietary supplements on 1,2-dimethylhydrazine dihydrochloride-induced intestinal cancer in rats. J Natl Cancer Inst. 1980;64(2):263–265. doi:10.1093/jnci/64.2.263.
- Górska A, Przystupski D, Niemczura MJ, Kulbacka J. Probiotic bacteria: a promising tool in cancer prevention and therapy. Curr Microbiol. 2019;76(8):939. doi:10.1007/s00284-019-01679-8.
- Guimarães GR, Almeida PP, de Oliveira Santos L, Rodrigues LP, de Carvalho JL, Boroni M. Hallmarks of aging in macrophages: consequences to skin inflammaging. Cells. 2021;10(6):1323. doi:10.3390/cells10061323.