Abstract
Hepatocytes, the major epithelial cells of the liver, maintain their morphology in culture dishes coated with extracellular matrix (ECM) components such as collagen and fibronectin or biodegradable polymers (e.g. chitosan, gelatin). In these coated dishes, survival of cells and maintaining of liver-specific functions may increase. The aim of this study was to determine a suitable, cost-effective and simple system for hepatocyte isolation and culture which may be useful for various applications such as in vitro toxicology studies, hepatocyte transplantation and bioartificial liver (BAL) systems. In order to obtain primary cultures, hepatocytes were isolated from liver by an enzymatic method and cultured on plates coated with collagen, chitosan or gelatin. Collagen, gelatin-sandwich and gelatin-cell mixture methods were also evaluated. Morphology and attachment of the cells were observed by inverted microscope and scanning electron microscope (SEM). An MTT assay was used to determine cell viability and mitochondrial activity.
Introduction
Primary hepatocytes have been used as in vitro models to understand biological processes occuring in the liver. Freshly isolated hepatocytes retain their liver-specific functions and also minimize the amounts of components required for understanding of hepatotoxicity (Shen et al. Citation2006). In addition, these cultures offer a model system for drug metabolism and biochemical synthesis.
In order to isolate hepatocytes from the liver, numerous isolation techniques such as collagenase perfusion of the liver (Puviani et al. Citation1998; Guguen-Guillouzo Citation2002; Li et al. Citation2005; Park and Song Citation2006), permeation of the liver with chelator solutions (Battle and Stacey Citation2001; Kravchenko et al. Citation2002), enzymatic digestion or mechanical dissociation of the liver parenchyma have been used in the literature (Puviani et al. Citation1998). When the cell yield is low or selective removal of the non-parenchymal cells is needed, Percoll® (Puviani et al. Citation1998) or Ficoll (Guguen-Guillouzo Citation2002) solutions may be used for the purification of the cells.
It is observed that the functional potencies of the cultured hepatocytes are closely related to the maintenance of the cuboid cell shape, distinct polarity and three-dimensional cellular communication (Fukuda et al. Citation2006). The metabolism and gene expression patterns of primary cells isolated from living organisms are frequently altered during the growth in a 2-D culture, which in turn are influenced by the changes in cellular morphology, intercellular signal transduction, and other extracellular environments (Fukuda et al. Citation2006). For example, from the studies carried out using collagen-based culture systems, it is observed that hepatocytes cultured on single collagen gels indicate a spread phenotype and rapidly dedifferentiate. However, bilayered collagen gels, collagen gel embedded aggregates or floating gel cultures can improve hepatocyte functions and survival. In a sandwich configuration, which is one of the major 3D culture systems used for hepatocytes, restricted cell spreading, enhanced liver-specific polarity and functions have been achieved (Dunn et al. Citation1989; Allen et al. Citation2001). A spheroid technique has been used to re-establish the tissue-like arrangement of the cells (Eschbach et al. Citation2005; Fukuda et al. Citation2006). In addition, hepatocytes were microencapsulated in alginate, alginate-polylysine composites and fibers in order to promote cell aggregation and liver-spesific function as well as provide immuno-isolation (Zavan et al. Citation2005). Several hepatocyte cell culture systems based on the use of synthetic or natural polymers such as chitosan/gelatin composite (Li et al. Citation2004), alginate (Glicklis et al. Citation2000), (PVA)-coated polytetrafluoroethylene (ePTFE) (Tokiwa et al. Citation1997), alginate, chitosan (Chi), collagen (Col), gelatin (Gel) (Li et al. Citation2005) have been reported.
A tissue can be considered as a composite material which consists of cells and extracellular matrix (ECM). ECM is composed of glycoproteins, collagen and gylcosaminoglycan network. Scaffolds used for the regeneration of tissues act as artificial ECMs to promote cell adhesion and maintenance of differentiated function (Mao et al. Citation2004). Collagen, the most abundant structural protein, is an important component of ECM (Kim et al. Citation2000). It has been approved by The United States Food and Drug Administration (FDA) for many types of medical application, including wound dressings and artificial skin (Pachence Citation1996). Collagen contains cell-adhesion domain sequences such as RGD and thus assists the retention of phenotype and functions of various cells.
Gelatin is a natural protein obtained by partial denaturation of collagen. It maintains functional groups suitable for cell adhesion and its biodegradable, biocompatible and non-immunogenic nature makes gelatin a suitable material (Mao et al. Citation2004; Moscato et al. Citation2008).
Chitosan, a copolymer of β-(1→4)-linked N-acetyl-D-glucosamine and D-glucosamine units, is obtained by deacetylation of chitin and found in arthropod exoskeletons. Because chitosan shares some characteristics with glycosaminoglycans (GAG) and hyaluronic acid, it is used in various tissue engineering applications (Suh and Matthew Citation2000). In addition, the biodegradabe, biocompatible, non-antigenic, non-toxic and bifunctional properties of chitosan make this polymer a suitable material for biomedical applications such as wound dressings (Hirano et al. Citation1990) and drug delivery (Aiedeh et al. Citation1997). It is known that chitosan plays a role in cell attachment and proliferation (Zhu et al. Citation2002).
The purpose of this study was to establish a simple and cost-effective method for hepatocyte isolation and culture. Although enzymatic isolation of hepatocytes was achieved in the literature, the low hepatocyte yield has limited its use as the primary isolation method for hepatocytes. The perfusion method is commonly used for hepatocyte isolation; however, it has also some problems such as being expensive and time-consuming. In addition, special equipment is needed to perfuse the liver tissue. In our study, pure populations of primary hepatocytes were obtained using an enzymatic-mechanical method combination with the Percoll gradient procedure. The behavior of freshly isolated hepatocytes was investigated in uncoated, and collagen-, gelatin- and chitosan-coated culture dishes. Behaviors of the cells in culture were determined by microphotography, MTT assay and SEM.
Materials and methods
Materials
All inorganic salts for Krebs solution were of analytical grade. Collagen A (Biochrom AG, Germany) was commercially available. Acetic acid, gelatin and chitosan were purchased from Sigma Co. (Germany). The degree of deacetylation of chitosan was 85%.
Preparation of polymer-coated tissue culture plates
Gelatin was dissolved in ultra pure water at 40–50°C and 1 mg/ml gelatin solution was obtained. Twenty mg of 85% chitosan was dissolved in 20 ml of 1% acetic acid to obtain a chitosan solution with a concentration of 1 mg/ml. Collagen A Solution (1 mg/ml) (Biochrom AG, Germany) was used without modification. Polymer solution (0.5 ml) was added to each well of 24-well tissue culture plates. Plates were placed under UV at room temperature until the gelation occurred (~5–6 hours). Residual polmer solutions were removed by a micropippette.
Isolation and culture of hepatocytes
Hepatocytes were isolated from Wistar rats (weighing 200 + 50 g), for which approval was given by the Ethics Committee for Animal Experiments of Ege University, by an enzymatic method. First, the animal was anesthetized and sacrificed by servical dislocation. Liver was excised and transferred into Krebs solution (154 mM NaCl, 5.4 mM KCl, 1.2 mM MgSO4, 12 mM glucose, 2 mM CaCl2, 10 mM HEPES (Biochrom AG, Germany), 250 µg/ml gentamycin, 100 U/ml penicillin, 100 µg/ml streptomycin, 2.5 µg/ml amphotericin B (Sigma Co., Germany)). After the liver was rinsed with Krebs solution, it was incubated in 0.5 mg/ml collagenase solution (Type CLS II, Biochrom AG, Germany) at 37°C for 15 min. Then the liver was filtered through stainless steel sieves and cell suspension was centrifuged at 1000 rpm for 5 min. After centrifugation, the cell pellet was washed with Krebs solution and Ca2 +, Mg2 +-free PBS (phosphate buffered saline) (250 µg/ml gentamycin, 50 U/ml penicillin, 50 µg/ml streptomycin, 1.25 µg/ml amphotericin B (Sigma Co., Germany)). Cell suspension (12.5 ml) was added to 10.8 ml of Percoll solution (density 1.124 g/ml, Biochrom AG, Germany) and 1.2 ml of 10× concentrated DMEM/F12 medium et al. 1989). The mixture was centrifuged at 1000 rpm for 15 min, and the cell layer was washed with William's E Medium (without insulin or EGF). Cells were re-suspended in fresh William's E Medium (William's E, 5% FCS, 1% L-glutamine, 1% HEPES, 2% NaHCO3, 0.1% insulin, 0.1% EGF, 50 U/ml penicillin, 50 µg/ml streptomycin, 1.25 µg/ml amphotericin B, 200 µg/ml gentamycin) and then seeded at a concentration of 106 cell/ml into polymer-coated and uncoated 24-well plates (1 ml cell suspension to each well). In addition, cells were mixed with gelatin solution (2:1) and seeded into an uncoated 24-well plate.
After 1 day incubation, 0.5 ml of the culture medium was removed and 0.5 ml fresh medium was added. A second layer of collagen and gelatin polymers was added onto the cells in order to obtain a sandwich configuration. Half of the medium was replaced every 2 days. Daily observations of the culture were made with the use of an inverted microscope. SEM and MTT studies were done on the third day of the culture.
Scanning electron microscopy (SEM) examination
The morphology and attachment properties of the hepatocytes were determined on the third day of the culture by SEM. For this purpose, cells were fixed with 2.5% glutaraldehyde in 0.1 M cacodylate buffer (pH 7.2) for 30 min, 7% sucrose in 0.1 M cacodylate buffer and 1% osmium tetraoxide in 0.1 M cacodylate buffer. Samples were dehydrated in graded ethanol series (20, 30, 40, 50, 60, 75%), desiccated for 5 min in hexamethyldisilazane, followed by air drying for 30 min and incubation in a desiccator with phosphorus pentaoxide overnight. Finally, samples were coated with a thin layer of gold by ion sputtering and then examined using a JEOL JSM-5200 scanning electron microscope (Tokyo, Japan).
Cellular activity and viability studies
MTT assay measures the reduction of yellow 3-(4,5-dimethylthiazol-2-yl)-2,5-diphenyltetrazolium bromide to a purple formazan product. Because this reduction occurs only when mitochondrial reductase enzymes are active, convertion is related to the number of viable cells. All experiments were carried out in triplicate for each culture condition. On the third day of culture, cells were centrifuged and cell pellets were homogenized with 0.5 mg/ml of MTT medium. Cell suspension was dispersed into a 96-well plate (200 µl to each well), and incubated in a humidified atmosphere, with 5% CO2 at 37°C during 4 h. After the medium was decanted, 200 µl of dimethylsulphoxide (DMSO) was added to each well in order to dissolve formazan salts. The absorbance was determined at 570 nm using an UV- visible spectrophotometer multiplate reader (VersaMax, Molecular Device, USA).
Statistical analysis
All data are presented as means±standard deviation (SD) for n=3. Statistical comparisons were performed using GraphPad Instant Software. P-values < 0.01 were considered statistically significant.
Results
In this study, hepatocyte isolation was performed using a simple enzymatic method based on the use of collagenase. Percoll solution was used in order to increase cell density and also to obtain a homogeneous hepatocyte suspension. The polymers used in this study were sterilized by UV. When cell culture medium was added onto substrates, a marked pH decrease was noticed in the wells coated with chitosan and a slight one in the wells coated with collagen. In order to increase pH, these wells were washed with ~50 µl of 8.5% NaHCO3. Hepatocyte culture systems were designed as single layers of gelatin, collagen or chitosan polymers. Additionally, a mixture of gelatin-cell suspension and sandwich configurations were examined as hepatocyte culture systems.
Cell culture studies were carried out with the initial concentration of 106 cells/ml. On the second day of the culture, individual cells began to form aggregates. Hepatocytes cultured on chitosan and collagen substrates proliferated quickly and were predominant in terms of aggregate formation in the beginning of the culture. However, damage on the hepatocytes’ membranes was observed in chitosan-coated wells in the following days of the culture. Gelatin showed improved cellular viability for hepatocytes. Especially, after 2 days in culture, cells on gelatin substrates began to lose their membrane borders and formed dense aggregates ().
The adhesion and ECM secretion of the cells were examined by scanning electron microscopy. SEM results indicated that cells on substrates started to form secreta (). Cell viability and mitochondrial activity were determined by MTT assay (). Especially on the third day in culture, gelatin showed a significant stimulation of hepatocyte viability compared to other substrates. MTT values of the cells on chitosan substrates were lower than expected.
Discussion
In our study, aimed at the preparation of primary hepatocyte cultures with high purity and low cost, isolation was achieved by an easy and time-saving method based on the use of collagenase solution. The isolation method is schematized in . Because cell density and viability obtained by this simple enzymatic method are lower than with the perfusion method, Percoll solution was used for the further purification of the cells (Puviani et al. Citation1998). It is possible to separate parenchymal cells from non-parenchymal and dead cells, and obtain pure hepatocyte populations by using Percoll. In order to detect the effect of Percoll on cell yield, two different cell suspensions were used. The first suspension was prepared by taking the layer containing hepatocytes only. In the preparation of the second suspension, Percoll was not used. The results of this experiment are shown in . Eighty to 90% of the whole suspension was composed of hepatocytes with the first technique. However, this ratio decreased to 20–30% with the second one.
In the literature, the mixtures of chitosan/collagen or chitosan/gelatin are reported as suitable substrates for hepatocytic proliferation and function (Li et al. Citation2005). Although chitosan is often used as a scaffold in the tissue engineering field because of its similarities to glycosaminoglycans found in ECM, it is reported that chitosan elicits minimal immunological responses and cytoskelatal actin distribution. It can also restrict cell spreading. It is known that chitosan's ability to stimulate cell proliferation is closely related to its deacetylation degree (Chatelet et al. Citation2001; Amaral et al. Citation2005). Chitosan substrates with high deacetylation degrees can improve cell proliferation compared to those with lower acetylation degrees. Considering this fact, chitosan with a deacetylation degree of 85% was used in this study.
Figure 2. The effect of Percoll solution: (a) Cells purified with Percoll solution, (b) Cell suspension obtained without purification step and contamination with non-parenchymal cells.
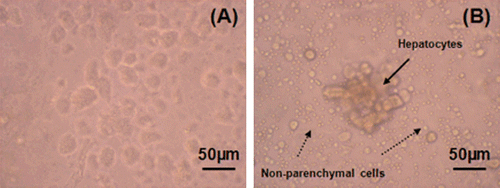
The mechanical properties and biodegradation rates of the collagen and chitosan are not good, especially in aqueous media (Wang et al. Citation2003). Collagen molecules are usually cross-linked by chemical agents such as glutaraldehyde (GE) and formaldehyde. Although GA is an efficient cross-linking agent, because of the cytotoxicity problems physical crosslinking methods such as UV irradition were used for collagen-based materials (Lee et al. Citation2001). In our study, UV, which was mainly used for the sterilization of materials, may also support cross-linking of collagen and also gelatin.
The most suitable substrates for hepatocyte cultures are those which mimic the in vivo environment more closely and thus maintain liver-specific functions and cell viability. Therefore, in our study, substrates were evaluated by aggregate formation, which resembles sinusoid formation in vivo. The individual cells which were observed in the beginning of the culture began to form aggregates on the first day of the culture (). Although smaller cell aggregates (3–20 cells) were seen on chitosan substrates, aggregates consisting of 20–50 or >50 cells were detected on collagen and gelatin polymers. Aggregate formation was similar in the wells coated with gelatin and collagen (C and D). However, aggregate number and size were improved in gelatin-coated wells when compared to those on collagen layers. In addition to aggregates, numerous indiviual cells were observed in chitosan coated wells.
Figure 3. Light microscopy photographs of hepatocytes (first day in culture): (a) In chitosan coated wells, (b) in gelatin coated wells, (c) in gelatin coated wells, (d) in collagen coated wells.
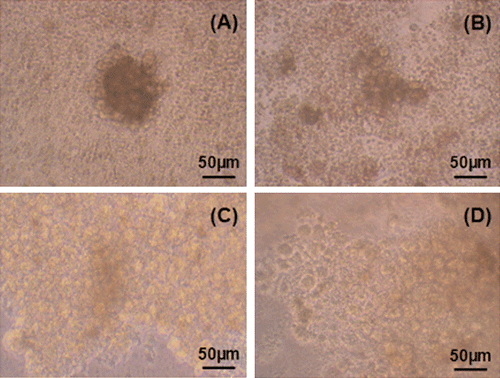
Daily observations made by inverted microscope showed that chitosan stimulated hepatocyte proliferation after the second day of culture. On the third day, the cells on chitosan, collagen and gelatin substrates began to lose their cell borders and dense aggregate formations were observed instead of particular cells ().
Figure 4. Third day in culture: (a) Uncoated well, (b) collagen coated well, (c) chitosan coated well, (d) gelatin coated well.
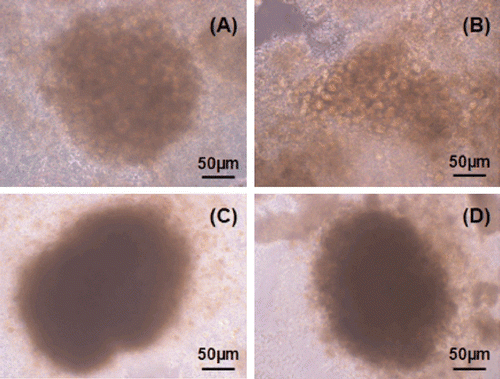
Although chitosan stimulated cell proliferation and aggregate formation in the beginning of the culture, this stimulative effect was lost in the following days and membrane damage was observed in the wells coated with this polymer (). On the fifth day of culture, the cells on collagen, gelatin and chitosan substrates were more rounded compared to uncoated wells (). On this day, newly formed hepatocyte aggregates consisting of 2–10 cells were detected in addition to dense sinusoid-like formations in gelatin-coated wells (data not shown). This result indicated that hepatocytes on gelatin substrates maintained their viability and proliferative capacity.
Figure 5. Fifth day in culture: (a) uncoated well, (b) chitosan coated well, (c) collagen coated well, (d) collogen sandwich method.
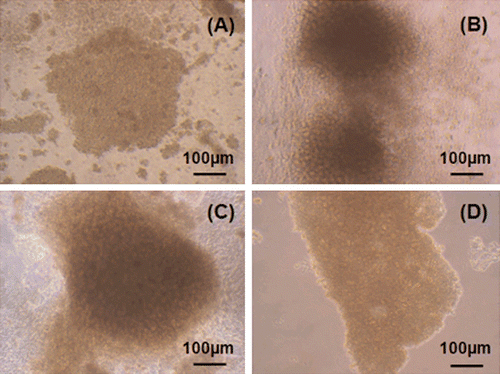
Li et al. (Citation2005) reported that hepatocytes cultured on alginate and chitosan substrates can sustain their functions. Conversely, collagen and gelatin are apt to promote cellular attachment while hepatocytic functions are damaged because of spreading. It is known that functions of the hepatocytes are closely related to their morphology (Fukuda et al. Citation2006). SEM results indicated that the structure of hepatocyte secreta on chitosan and gelatin substrates were similar (a–d). However, pseudopodia attached well to the matrices were found in collagen-coated wells (e and f). This evidence showed that cells on collagen substrates had begun to spread.
Figure 6. SEM images of hepatocytes secreta in the wells coated with: (a) gelatin (×5000), (b) gelatin-cell mixture (×5000), (c) gelatin sandwich (×2000), (d) chitosan (×2000), (e) collagen sandwich (×7500), (f) collagen sandwich (×7500).
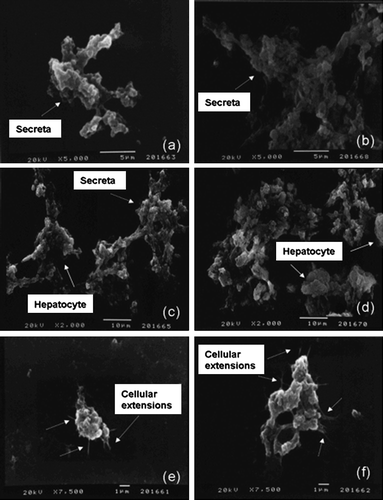
Schmelzer et al. (Citation2007) indicated that human hepatic stem cells have unique features such as expressing NCAM (neural cell adhesion molecule) and EpCAM (epithelial cell adhesion molecule) and having ~9 µm diameter. In our study, small hepatic cells which were 10–15 µm in diameter observed during SEM experiments (c and d). In addition, immunocytochemical staining against NCAM showed that some cells in culture were NCAM+ (data not shown). These findings can be related to the fact that hepatic progenitors were isolated as well as mature hepatocytes using this method. However, more experiments should be done in order to support this theory.
On the sixth day of the culture, aggregate formations on different substrates were counted. Seven aggregates were observed per gelatine-coated well. On collagen and chitosan substrates five formations were counted for each well. In addition, individual cells were seen on the chitosan substrates and in the collagen sandwich method while cells on gelatin and collagen polymers showed aggregate formations.
In different studies, it is reported that hepatocyte morphology, polarity and liver-specific functions are maintained in the collagen sandwich method (Dunn et al. Citation1989). In our study, the method proposed by Dunn et al. was used in order to obtain a sandwich configuration. However, after the addition of a second layer of polymer solution, cells began to float and some of the cells were lost during the removal of residual polymer solutions. Because of these problems encountered, MTT values for sandwich configurations were lower than single-layer methods (). Mitochondrial redox activities of primary hepatocytes on different materials were evaluated by reduction of MTT to formazan by mitochondrial succinate dehydrogenase in complex II (succinate:ubiquinone oxidoreductase complex), which plays a central role in both oxidative phosphorylation and the tricarboxylic acid cycle. When a polymer-cell mixture was used, improved hepatocyte viability was obtained compared to the sandwich configuration. However, values were still lower than single-layer methods. There was no significant difference between chitosan-coated, uncoated wells or the collagen and gelatin sandwich method. Owing to the similarities between gelatin, collagen and ECM of the hepatocytes, these substrates induced mitochondrial activity and viability compared to tissue culture plates (P<0.001). MTT assay results supported microscopic observations and the highest value was obtained in gelatin-coated wells, as expected.
Figure 7. MMT results for different substrates on the third day of culture (*: p < 0.001, **: p < 0.01).
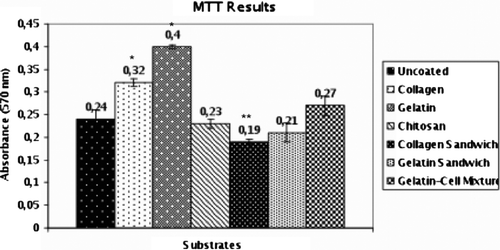
In vitro studies have demonstrated that it is possible to obtain hepatocyte populations using our method. Hepatocyte cultures, which have a high potential for toxicity studies, have been established with low costs and high reproducibility. Gelatin and collagen substrates showed improved cellular viability, mitochondrial activity and desired morphology similar to the results obtained in the literature when the perfusion method was used for cell isolation. Although chitosan stimulated aggregate formation in the beginning of the hepatocyte cultures, unmodified chitosan substrates may not be a suitable substrate for long-term studies of liver functions or applications which require the use of liver cells and they should be further modified.
Acknowledgements
The authors received partial support from Ege University Science and Technology Society (EBILTET) under the Project No. E18/03/06. In addition, we are grateful to Assoc. Prof. Seda Vatansever, Dr. Feyzan Özdal Kurt and Dr. Elgin Türköz from Celal Bayar University for their help in the study.
References
- Aiedeh , K , Gianasi , E , Orienti , I and Zecchi , V. 1997 . Chitosan microcapsules as controlled release systems for insulin . J Microencapsul. , 14 : 567 – 576 .
- Allen , JW , Hassanein , T and Bhatia , S. 2001 . Advances in bioartificial liver devices . Hepatology. , 34 : 447 – 455 .
- Amaral , IF , Lamghari , M , Sousa , SR , Sampaio , P and Barbosa , M. 2005 . Rat bone marrow stromal cell osteogenic differentiation and fibronectin adsorption on chitosan membranes: The effect of the degree of acetylation . J Biomed Mater Res A. , 75A : 387 – 397 .
- Battle , T and Stacey , G. 2001 . Cell culture models for hepatotoxicology . Cell Biol Toxicol. , 17 : 287 – 289 .
- Chatelet , C , Damour , O and Domard , A. 2001 . Influence of the degree of acetylation on some biological properties of chitosan films . Biomaterials. , 22 : 261 – 268 .
- Chupa , JM , Foster , AM , Sumner , SR , Madihally , SV and Matthew , HW. 2000 . Vascular cell responses to polysaccharide materials: in vitro and in vivo evaluations . Biomaterials. , 21 : 2315 – 2322 .
- Dunn , JCY , Yarmush , ML , Koebe , HG and Tompkins , RG. 1989 . Hepatocyte function and extracellular matrix geometry: long-term culture in a sandwich configuration . FASEB J. , 3 : 174 – 177 .
- Eschbach , E , Chatterjee , SS , Nöldner , M , Gottwald , E , Dertinger , H , Weibezahn , KL and Knedlitschek , G. 2005 . Microstructured scaffolds for liver tissue cultures of high cell density: morphological and biochemical characterization of tissue aggregates . J Cell Biochem. , 95 : 243 – 255 .
- Fukuda , J , Sakai , Y and Nakazawa , K. 2006 . Novel hepatocyte culture system developed using microfabrication and collagen/polyethylene glycol microcontact printing . Biomaterials. , 27 : 1061 – 1070 .
- Glicklis , R , Shapiro , L , Agbaria , R and Merchuk , JC. 2000 . Hepatocyte behavior within three-dimensional porous alginate scaffolds . Biotechnol Bioeng. , 67 : 345 – 353 .
- Guguen-Guillouzo , C. 2002 . “ Isolation and culture of animal and human hepatocytes ” . In Culture of epithelial cells , Edited by: Freshney , RI and Freshney , MG . 337 – 379 . New York (NY) : Wiley-Liss .
- Hirano , S , Itakura , C , Seino , H , Akiyama , Y , Nonaka , I , Kanbara , N and Kawakami , T. 1990 . Chitosan as an ingredient for domestic-animal feeds . J Agric Food Chem. , 38 : 1214 – 1217 .
- Huang , Y , Onyeri , S , Siewe , M , Moshfeghian , A and Madihally , SV. 2005 . In vitro characterization of chitosan-gelatin scaffolds for tissue engineering . Biomaterials. , 26 : 7616 – 7627 .
- Kim , BS , Baez , CE and Atala , A. 2000 . Biomaterials for tissue engineering . World J Urol. , 18 : 2 – 9 .
- Kravchenko , L , Petrenko , A , Shanina , I and Fuller , B. 2002 . A simple non-enzymatic method for the isolation of functional rat hepatocytes . Cell Biol Int. , 26 : 1003 – 1006 .
- Lee , JE , Park , JC , Hwang , YS , Kim , JK , Kim , JG and Suh , H. 2001 . Characterization of UV-irradiated dense/porous collagen membranes: morphology, enzymatic degradation, and mechanical properties . Yonsei Med J. , 42 : 172 – 179 .
- Li , K , Qu , X , Wang , Y , Tang , Y , Qin , D , Wang , Y and Feng , M. 2005 . Improved performance of primary rat hepatocytes on blended natural polymers . J Biomed Mater Res A. , 75 : 268 – 274 .
- Li , K , Wang , Y , Miao , Z , Xu , D , Tang , Y and Feng , M. 2004 . Chitosan/gelatin composite microcarrier for hepatocyte culture . Biotechnol Lett. , 26 : 879 – 883 .
- Mao , JS , Zhao , LG , Yin , YJ and Yao , KD. 2003 . Structure and properties of bilayer chitosan-gelatin scaffolds . Biomaterials. , 24 : 1067 – 1074 .
- Mao , JS , Cui , YL , Wang , XH , Sun , Y , Yin , YJ , Zhao , HM and Yao , K. 2004 . A preliminary study on chitosan and gelatin polyelectrolyte complex cytocompatibility by cell cycle and apoptosis analysis . Biomaterials. , 25 : 3973 – 3981 .
- Moscato , S , Mattii , L , D'Alessandro , D , Cascone , MG , Lazzeri , L , Serino , LP , Dolfi , A and Bernardini , N. 2008 . Interaction of human gingival fibroblasts with PVA/gelatine sponges . Micron. , 39 : 569 – 579 .
- Pachence , JM. 1996 . Collagen-based devices for soft tissue repair . J Biomed Mater Res B. , 33 : 35 – 40 .
- Park , KH and Song , SC. 2006 . Morphology of spheroidal hepatocytes within injectable, biodegradable, and thermosensitive poly(organophosphazene) hydrogel as cell delivery vehicle . J Biosci Bioeng. , 101 : 238 – 242 .
- Puviani , AC , Ottolenghi , C , Tassinari , B , Pazzi , P and Morsiani , E. 1998 . An update on high-yield hepatocyte isolation methods and on the potential clinical use of isolated liver cells . Comp Biochem Physiol A. , 121 : 99 – 109 .
- Schmelzer , E , Zhang , L , Bruce , A , Wauthier , E , Ludlow , J , Yao , H , Moss , N , Melhem , A , McClelland , R Turner , W . 2007 . Human hepatic stem cells from fetal and postnatal donors . J Exp Med. , 204 : 1973 – 1987 .
- Shen , C , Zhang , G , Qiu , H and Meng , Q. 2006 . Acetaminophen-induced hepatotoxicity of gel entrapped rat hepatocytes in hollow fibers . Chem Biol Interact. , 162 : 53 – 61 .
- Suh , JKF and Matthew , HWT. 2000 . Application of chitosan-based polysaccharide biomaterials in cartilage tissue engineering: a review . Biomaterials. , 21 : 2589 – 2598 .
- Tokiwa , T , Kano , J , Kodama , M and Matsumura , T. 1997 . Multilayer rat hepatocyte aggregates formed on expanded polytetrafluoroethylene surface . Cytotechnology. , 25 : 137 – 144 .
- Wang , XH , Li , DP , Wang , WJ , Feng , QL , Cui , FZ , Xu , YX , Song , XH and van der Werf , M. 2003 . Crosslinked collagen/chitosan matrix for artificial livers . Biomaterials. , 24 : 3213 – 3220 .
- Zavan , B , Brun , P , Vindigni , V , Amadori , A , Habeler , W , Pontisso , P , Montemurro , D , Abatangelo , G and Cortivo , R. 2005 . Extracellular matrix-enrichted polymeric scaffolds as a substrate for hepatocyte cultures: in vitro and in vivo studies . Biomaterials. , 26 : 7038 – 7045 .
- Zhu , A , Zhang , M , Wu , J and Shen , J. 2002 . Covalent immobilization of chitosan/heparin complex with a photosensitive hetero-bifunctional crosslinking reagent on PLA surface . Biomaterials. , 23 : 4657 – 4665 .