Abstract
In this study, we investigated changes in the standing crop, species composition, and ecological index of phytoplankton and zooplankton caused by the installation of an artificial upwelling structure in the sea area. The standing crop of phytoplankton and zooplankton in 2009, when the artificial upwelling structure was completed, increased 50-fold and 2.3-fold, respectively, from 2005 when the artificial upwelling structure was installed. During the investigation period, however, the correlation between the standing crop of zooplankton and the standing crop of phytoplankton did not show significant difference (SPSS, p >0.05). Phytoplankton and zooplankton species showed a 2.47-fold increase in a total of 94 species and a 1.68-fold increase in a total of 27 species, respectively, from 2006 to 2009. During the study period, the dominant species of phytoplankton were Chaetoceros didymus (16.5%), Chaetoceros pseudocrinitus (8.5%), Copelatus anastomosans (7.6%), and Skeletonema costatum (6.0%), and the dominant species of zooplankton were Paracalanus parvus (21.6%), Evadne tergestina (6.6%), Paracalanus aculeatus (6.1%), Lucifer intermedius (5.7%), and Sinocalanus tenellus (5.4%), and copepods accounted for the majority of zooplankton. Phytoplankton species diversity index and richness index increased significantly from 1.68 to 2.45 and from 1.61 to 3.65, respectively, in the period from 2006 to 2009, but dominant species index decreased from 0.55 to 0.40 in the same period. It was known that bigger artificial upwelling structures caused a gradually rising upwelling effect and that in the study area, from 2006 to 2009, the ecosystem was changing to a more stable one where a variety of species habitat gradually.
Introduction
As Korea's neighbors, including Japan and China, declare exclusive economic zones and strengthen fishing regulations, and Korea's offshore fishing grounds diminish, the coastal regions are becoming very important. Korean coastal areas are experiencing a reduction in fisheries due to the expansion of large-scale land reclamation projects and a deteriorating coastal fishery environment as a result of industrial and domestic wastewater inflows.
In order to cope with these changes in domestic and foreign fishing conditions and foster competitiveness in the coastal fishing industry, the importance of the construction of fishery resources must be recognized.
The term ‘artificial reefs’ refers to installations established undersea to protect and nurture the aquatic organisms. ‘Artificial reef fisheries’ refers to systematically arranged fisheries resource shelters designed to promote the growth, protection, and efficient operation of aquatic resources.
In order to increase production of these fishery resources and create artificial spawning sites and habitats, a variety of studies on artificial reef have been attempted at home and abroad (Ohno et al. Citation1990; Lee & Kang Citation1994; Serisawa & Ohno Citation1995; Pickering & Whitmarsh Citation1997; Park & Shin Citation2003). It was found that artificial reefs caused increased catch due to an increase in fish resources, a natural increase in production due to increased biological resources, and had fishing effects of 1.2–12.0 times more than normal fishery ground and of 1.3–2.2 times more than natural reef fishery ground (Choi et al. Citation2002; Choi et al. Citation2006; Cho et al. Citation2007).
The ocean upwelling phenomenon means that when surface-layer water is moved by external forces such as wind, seawater from the bottom layer rises into the upper layer to fill its place (Beer Citation1996). It is known that when upwelling occurs, bottom-layer water rich in nutrients and minerals reaches the euphotic zone of the surface layer, increases the primary productivity of the coastal region and helps to create rich fishing grounds by promoting fish communities in the food chain (Mitchell-Innes & Walker Citation1991; Choi et al. Citation2002; Nicholas et al. Citation2009).
Also Ryther (Citation1969) reported that upwelling seawaters occupying about 0.1% of the entire ocean area yielded fish productivity equivalent to that of half the entire ocean, with very high primary productivity. It has been reported that beneath the Dokdo Islands, a 2000-meter sea mount is formed, currents that pass through this area cause upwelling and eddy currents, where phytoplankton communities are rich, and fishery ground is formed which harbors pollock and squid (KORDI Citation2000).
A new concept has been highlighted in which an attempt is made to increase the primary productivity of a coastal region and create a good fishery ground, by using an artificial reef and upwelling water together through means of installing artificial marine structures to cause artificial upwelling currents. In one case, researchers installed artificial upwelling structures in the eastern region of the Bongo Channel in Japan. The results showed a two- to three-fold increase in chlorophyll-a concentrations and a two-fold increase in the abundance of zooplankton. Two years after the installation of the artificial upwelling structures, there was a decrease in benthic biomass, and three years after the installation the benthic biomass increased (Yanagi & Nakajima Citation1991). In addition, the effect was reported to increase proportionally as the size of the structure increased (Imamura et al. Citation1995; Okaichi & Yanagi Citation1997).
In this study, we investigated changes in standing crop, species composition, and ecological index of phytoplankton and zooplankton, caused by an artificial upwelling structure installed in the sea between the Maemuldo and Gukdo Islands in Korea.
Materials and methods
In order to investigate changes in phytoplankton and zooplankton in the area where the artificial upwelling structures were installed (vertex 1: artificial structure installed site, E: 128°30′, N: 34°36′); we investigated four times a year, at nine stations, for a total of 20 times from 2005 to 2009 ().
We collected surface-layer water (0.5 m below the surface) and middle-layer water (20 m below the surface) with a Van Dorn sampler at the station of each investigation, put it in 1 L polyethylene sample bottles, blocked the light by fixing with Lugol solution in the field, transported it to the laboratory, and then precipitated it for more than 24 hours until it concentrated to 100 ml. We collected sample from the middle layer vertically with a zooplankton net (net mouth diameter: 30 cm, net mesh aperture: 300 µm), put samples in 250-ml polyethylene sample bottles, blocked the light by fixing with 10% neutral formalin solution in the field, and then transported to the laboratory.
After counting and identifying the species of zooplankton and phytoplankton samples with a Nikon TS-100 microscope, we converted them to the standing crop of the original seawater. In order to analyze phytoplankton communities and understand the function of ecosystems, we calculated Species Diversity Index, Evenness Index, Richness Index (R) and Dominance Index for the ecological index. Richness Index was obtained according to Margalef's formula (Citation1958) and Species Diversity Index (H) followed the formula of Shannon and Wiener (Citation1963). Evenness Index (E) was calculated by using Pielou's index (Citation1966), and dominance index was expressed as the value of sum of the most dominant species and the second most dominant species divided by the total population. Classification and identification of the samples were conducted by using references including that of Yoo (Citation1995), Smith and Johnson (Citation1996), Chihara and Murano (Citation1997), and Young (Citation2002). Statistical analyses were performed with SPSS 12.0 software (Statistical Program for Social Sciences). The correlation results were considered significant at p<0.05.
From June 2005 to August 2009, the artificial upwelling structures were installed by dropping hexagonal blocks (1 m×1 m×1 m) from marine crane ship. By dropping 11,015 blocks in 2005, conical structures with a radius of 35–42 m were created, and then by dropping 8100 blocks in 2006, 8100 blocks in 2007, 5200 blocks in 2008 and 4400 blocks in 2009, when the structures were completed, a sea mount with a width of 42 m, height of 17 m, and length of 129 m was finally created.
Results and discussion
Standing crop of phytoplankton
As the annual mean standing crop of phytoplankton in the research region was investigated, it was shown that in 2005, the period of the initial installation of the artificial upwelling structures, the average value in surface-layer water was 8700 cells/L, and the average value in middle-layer water was 9700 cells/L, while in 2006, the average value in surface-layer water increased to 68,500 cells/L and the average value in middle-layer water increased to 51,800 cells/L. In 2009, the final year of the investigation, the average value in surface-layer water was 471,900 cells/L, and the average value in middle-layer water was 456,800 cells/L, which were 54 times and 47 times higher than the values in 2005, respectively ().
Figure 2. Seasonal fluctuations of standing crop of phytoplankton during the study period (2005–2009).
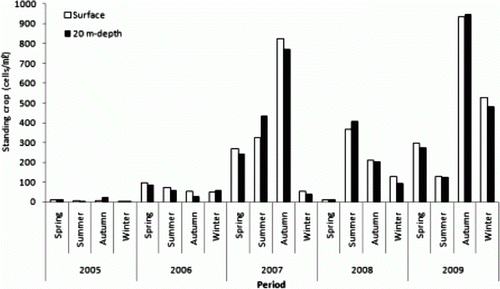
After dropping the structures, the standing crop of phytoplankton was found to increase continually except in 2008. Kim and Hwang (Citation2006) reported that nutrients in summer increased more significantly in 2005, after the structures were dropped, than in 2002, before they were dropped. As Nakashima (Citation2005) and Kim (Citation2008) reported that upwelling of bottom-layer water due to the installation of the structures and rich nutrients contained in the bottom-layer water contributed to increases in phytoplankton number; we suggest that since the structures were dropped, the increase in the standing crop of phytoplankton resulted from nutrients contained in the bottom-layer water.
Also as Okaichi and Yanagi (Citation1997) reported that effect of the structure was proportional to the size of the structure, we suggest that the continuous increase in phytoplankton's standing crop resulted from the gradually increasing size of the artificial structures from 2005 to 2009. Annual mean biomass of phytoplankton in 2008 was relatively small compared to 2007 and 2009. We assume that since the biomass of zooplanktons in 2008 was the highest during the study period, the decrease in phytoplankton biomass resulted from these predation pressures.
The average of the seasonal phytoplankton standing crop was highest in the autumn of 2005, the time of the initial installation of the artificial upwelling structures, followed by 11,800 cells/L in spring, 6700 cells/L in summer, and 3100 cells/L in winter. In the survey in 2006, the highest value was 83,900 cells/L in spring, while the lowest was 38,800 cells/L in autumn, and in the survey in 2007, the highest value was 798,300 cells/L in autumn, while the lowest was 45,400 cells/L in winter. In the survey in 2008, the highest was 386,900 cells/L in summer, while the lowest was 11,100 cells/L in spring, and in the final survey in 2009, the highest was 941,900 cells/L in autumn, while the lowest was 126,600 cells/L in summer.
It can be seen that during the survey period, the results did not show distinct seasonal features; however, it was shown that the highest mean standing crop appeared to be 400,100 cells/L in autumn, followed by 193,100cells/L in summer, 143,600cells/L in winter and 129,700cells/L in autumn (). As Jung et al. (Citation2007) reported that the phytoplankton standing crop of the Tongyeong marine ranching waters was highest in autumn (436,000 cells/L) and was similar throughout the other seasons (averaging 113,000 cells/L), these results were similar to their report. Average standing crop of phytoplankton in spring 2007 and winter 2008 appeared to be very small during the period of seasonal survey since 2007, and the standing crop of zooplankton was relatively high during the period.
As Frost (Citation1980) reported that zooplankton feeding on phytoplankton can reduce the standing crop of phytoplankton and change its species composition, it was suggested that feeding pressure of zooplankton caused the decrease in phytoplankton's standing crop. During the investigation period, however, the correlation between zooplankton and phytoplankton was not significantly different between the two groups (SPSS, p>0.05).
Standing crop of zooplankton
Annual mean standing crop of zooplankton in this study region was 517.9 inds./m3 in 2005, 576.7 inds./m3 in 2006, 1419.9 inds./m3 in 2007, 1619.1 inds./m3 in 2008, and 1197.7 inds./m3 in 2009. Compared to the survey in 2005, the time of the initial installation of the artificial upwelling structures, a 2.3-fold increase in the standing crop, was shown in 2009, the time when the structures were completed (). These results are consistent with the report by Lee et al. (Citation2004) who noted that upwelling supplied the surface layer with rich nutrients, resulting in increased phytoplankton and zooplankton to feed on them.
Figure 3. Seasonal fluctuations of standing crop of zooplankton during the study period (2005–2009).
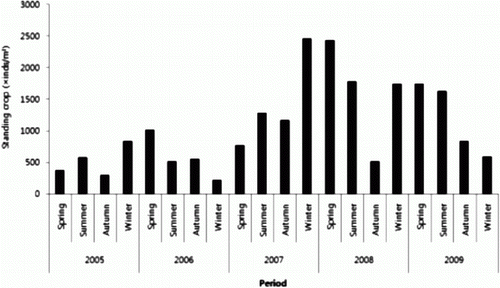
Seasonal standing crop of zooplankton in 2005 was 371.0 inds./m3 in spring, 573.9 inds./m3 in summer, 294.0 inds./m3 (the lowest) in autumn, and 832.8 inds./m3 (the highest) in winter. Seasonal standing crop of zooplankton in 2006 was 1014.4 inds./m3 (the highest) in spring and 222.8 inds./m3 (the lowest) in winter. Seasonal standing crop of zooplankton in 2007 was 2465.4 inds./m3 (the highest) in winter and 764.5 inds./m3 (the lowest) in spring. Seasonal standing crop of zooplankton in 2008 was 2434.5 inds./m3 (the highest) in spring and 515.0 inds./m3 (the lowest) in autumn. Seasonal standing crop of zooplankton in 2009, the time of the last survey, was 1747.8 inds./m3 (the highest) in spring and 593.0 inds./m3 (the lowest) in winter ().
Kang (Citation2008) analyzed the seasonal variation of the waters of the entire Korean Peninsula through the average standing crop of zooplankton from 1965 to 2000 and reported that the southern coast of Korea showed the characteristics of typical temperate waters, showing the spring peak in April and a small peak in October. In this study, however, we found different patterns each year without distinct seasonal characteristics.
Species composition and dominant species of phytoplankton
During the study period, the species composition of phytoplankton in these regions was all four families, 43 genuses, and 129 species, and taxonomically, Bacillariophyceae was 30 genuses and 100 species, Dinophyceae was 11 genuses and 26 species, Dictyochophyceae was 1 genus and 2 species, and Raphidophyceae was 1 genus and 1 species (). The recorded species in 2006, the time of the initial installation of the structures, showed monotonic species composition, with a total of 38 species, and the recorded taxa were 29 Bacillariophyceaes (76.3%), 7 Dinophyceas (18.4%), and 2 Dictyochophyceaes (5.3%).
Table 1. Classification of phytoplankton communities from 2006 to 2009.
In 2009, at the time of completion of structure installation, they showed a diverse species composition with a total of 94 species, while the emergence of species increased 2.47-fold compared to 2006, and a new taxon, Raphidophyceae, emerged. Recorded taxa were 66 Bacillariophyceaes (70.2%), 25 Dinophyceae (26.6%), 2 Dictyochophyceae (2.1%), and a Raphidophyceae (1.0%). Nutrient-rich upwelling of seawater in the bottom layer by the artificial structure seemed to contribute to the increase in recorded species as well as the standing crop of phytoplankton.
Compared to the total number of annual recorded species in the coastal regions of the South Sea – 35 genuses and 84 species in the Yeoja Bay (Lee & Yoon Citation2000), 51 genuses and 95 species in the Jinju Bay (Oh & Yoon Citation2008), and 50 genuses and 101 species in the Deukryang Bay (Lee & Lee Citation1999), the total number recorded in 2006, the time of the initial installation of the structure, was less than the number recorded in coastal regions of the South Sea. However, the number recorded in 2009, the last year of survey, was similar to the number recorded in the coastal regions of the South Sea.
During the period of investigation, the dominant species in the study area were Chaetoceros didymus (16.5%), followed by Chaetoceros pseudocrinitus (8.5%), Copelatus anastomosans (7.6%), and Skeletonema costatum (6.0%). The dominant species between 2006 and 2009 appeared as shown in . During the survey in 2006, Pseudo-nitzschia spp. was the most dominant species with a standing crop of 10,300 cells/L, constituting 17.1% of the total, and followed by Chaetoceros pseudocurvisetum (8100 cells/L, 13.5%), Thalassionema nitzschioides (6500 cells/L, 10.8%), S. costatum (4900 cells/L, 8.2%), and Eucampia zoodiacus (4600 cells/L, 7.7%). During the survey in 2007, C. didymus was the most dominant species with a standing crop of 70,800 cells/L, constituting 19.1% of the total, followed by C. anastomosans (34,000 cells/L, 9.2%), Leptocylindrus danicus (26,500 cells/L, 7.1%), C. pseudocrinitus (24,400 cells/L, 6.5%), and Pseudo nitzschia spp. (22,200 cells/L, 6.0%).
During the survey in 2008, C. didymus was the most dominant species with a standing crop of 34,700 cells/L, constituting 19.5% of the total, followed by Pseudo-nitzschia seriata (21,600 cells/L, 12.1%), T. nitzschioides (9300 cells/L, 5.2%), C. anastomosans (9300 cells/L, 5.2%), and S. costatum (8900 cells/L, 5.0%). During the survey in 2009, C. didymus was the most dominant species with a total biomass of 69,100 cells/L (14.9%), followed by C. pseudocrinitus (58,400 cells/L, 12.6%), C. anastomosans (37,700 cells/L, 8.1%), S. costatum (35,600 cells/L, 7.7%), and E. zoodiacus (26,300 cells/L, 5.7%).
Species composition and dominant species of zooplankton
During the study period, the species composition of zooplankton recorded in these regions was all 20 genuses and 35 species, and taxonomically, Copepoda was 11 genuses and 22 species, Chaetognatha was three genuses and four species, and Cladocera was three genuses and four species, Depapoda was a genus and two species, Ostracoda was a genus and two species, and Protozoa was a genus and a species (). The recorded species in 2006, the time of the initial installation of the structure, was a total of 38 species, and recorded taxa were 29 Copepoda (75.0%), a protozoa, a Cladocera, a Depapoda, and a Chaetognatha (6.25%). In 2009, the time of completion of structure installation, zooplankton showed a total of 27 species, with the emergence of species increasing 1.68-fold compared to 2006, and a new taxon, Ostracoda, emerged. Recorded taxa were 18 Copepoda (66.7%), 3 Cladocera (11.1%), 3 Chaetognatha (11.1%), 2 Ostracoda (7.4%) and a Depapoda (3.7%).
Table 2. Classification of zooplankton communities from 2006 to 2009.
During the period of investigation, the dominant species in the study area was Paracalanus parvus (21.6%), followed by Evadne tergestina (6.6%), Paracalanus aculeatus (6.1%), Lucifer intermedius (5.7%), and Sinocalanus tenellus (5.4%) (). P. parvus has been reported to be the dominant species in Ulsan-Gampo waters where upwelling occurs frequently (Lee et al. Citation2004). It is distributed in a relatively wide range of temperatures (Park et al. Citation1998) and has been reported to be the dominant species emerging in the upwelling zones of areas including Peru, Portugal, North Africa, and Chile (Peterson Citation1998).
During the survey in 2006, P. aculeatus was the most dominant species with 123.0 inds./m3 (21.3%), followed by P. parvus (108.6 inds./m3, 18.8%), S. tenellus (52.3 inds./m3, 9.1%), Acartia hudsonica (55.7 inds./m3, 9.7%), and Temora discaudata (44.2 inds./m3, 7.7%). In 2007, P. parvus was the most dominant species with 277.0 inds./m3 (19.5%), followed by Labeobarbus intermedius (one of the Depapoda, 238.0 inds./m3, 16.8%). In 2008, P. parvus was also the most dominant species with 526.7 inds./m3 (32.5%), followed by P. aculeatus (8.2%), S. tenellus (7.2%), A.hudsonica (6.0%), and Oithona similis (5.5%). In 2009, at the time when the structures were completed, E. tergestina (one of the Cladocera) showed the highest standing crop with 312.1 inds./m3 (26.1%), followed by P. parvus (132.6 inds./m3, 11.1%), Cirriped nauplius (89.7 inds./m3, 7.5%), Podon sp. (74.1 inds./m3, 6.2%), and T. discaudata (74.1 inds./m3, 6.2%). The characteristics of communities in specific localities reflect climatic and environmental status and change (Choi & Na Citation2010). Since the increase of nutrients in waters, where upwelling had occurred, raised the level of particulate organic carbon (POC) and particulate organic nitrogen (PON) as well as phytoplankton (Furuya et al. Citation1986; Yang et al. Citation1998; Kim Citation2002), we suggest that it would also affect the community structure of zooplankton to feed on them.
Ecological index
Species diversity exhibits a very close relationship with the stability of ecosystems (Pimm Citation1984; Tilman Citation2000). The species diversity index indicates the relative emergence between the populations of each species and the populations of the total emergence. A higher index value means that a variety of species inhabit an ecosystem stably. It is the most popular index among the ecologists and has been used extensively in the aquatic environment (Boyle et al. Citation1990). Shannon's index has been used also for diversity in microecosystems (Reed Citation1978) and marine phytoplankton (Ignatiades et al. Citation1985).
Richness Index measures the abundance of emerging species' diversity by considering the total number of recorded organisms and the total population; the higher the index, the higher the ecological stability.
Evenness index shows the extent of evenness of species composition and appears closer to 1 in a stable ecosystem. If dominance index gets closer to 1, it means that communities within ecosystems are much simpler, but if it gets closer to 0, it means that the ecosystems have much higher biodiversity and stability.
Comparing the phytoplankton ecology indexes between 2006, the time of the initial installation of the artificial upwelling structures, and 2009, the time of completion of the structure installation, species diversity index in 2009 was 2.45, which had increased gradually from 1.68 in 2006, showing that the ecosystem had become the site inhabited by a variety of species. Lately, assessment method of community stability and environmental condition using species diversity index has been utilized by several researchers (Lee et al. Citation2007; Kang et al. Citation2008; Choi & Rho Citation2010). If species diversity index is from 2 to 3, it is evaluated that community stability is unstable and environmental condition is quite well. Also, if species diversity index is from 1 to 2, it is evaluated that community stability is very unstable and environmental condition is bad, too (Kim et al. Citation2011).
Richness Index in 2009 was 3.65, which had increased significantly from 1.61 in 2006, showing that composition of species was also getting richer ().
Figure 6. Seasonal fluctuations of species diversity index and richness index of phytoplankton during the study period (2006–2009).
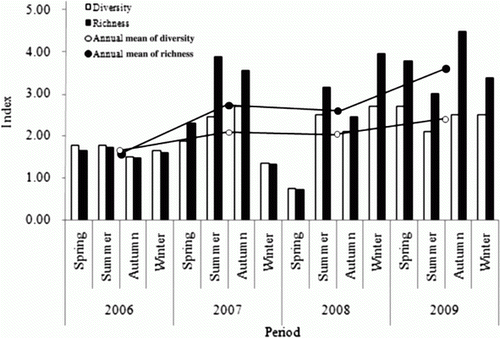
Also the Dominance Index in 2009 was 0.40, which had decreased gradually from 0.55 in 2006, showing that a simple ecosystem was gradually transformed into a stable ecosystem. The evenness index was found to show little change (). Very favorable evaluated waters by aquatic environment assessment using ESB (ecological score of benthic macroinvertebrate community) has high value in Species Diversity Index and Richness Index and low value in Dominance Index, relatively (Lee et al. Citation2012). Since the bigger the artificial upwelling structures, the stronger the upwelling effect, we could realize that the studied area was gradually transformed into a stable ecosystem where various species could exist.
References
- Beer , T. 1996 . Environmental oceanography , 2nd ed , 367 Boca Raton : CRC Press .
- Boyle , TP , Smillie , GM , Anderson , JD and Beeson , DR. 1990 . A sensitivity analysis of nine diversity and seven similarity indices . Res J WPCF , 62 : 749 – 762 .
- Chihara , M and Murano , M. 1997 . An illustrated guide to marine plankton in Japan , Tokyo : Tokai University Press .
- Cho , SH , Choi , CG and Choa , JH. 2007 . Restoration of the seaweed forest and algae succession on a Porous type (shaped half sea teeth) artificial reef . J Korean Fish Soc , 40 : 220 – 225 .
- Choi , CG and Rho , HS. 2010 . Marine algal community of Ulsan on the eastern coast of Korea . Kor J Fish Aquat Sci , 43 : 246 – 253 .
- Choi , CG , Ohno , M and Sohn , CH. 2006 . Algal succession on different substrata covering the artificial iron reef at Ikata in Shikoku, Japan . Algae , 21 : 305 – 310 . doi: 10.4490/ALGAE.2006.21.3.305
- Choi , CG , Takeuchi , Y , Terawaki , T , Serisawa , Y , Ohno , M and Shon , CH. 2002 . Ecology of seaweed beds on two types of artificial reef . J Appl Phycol , 14 : 343 – 349 . doi: 10.1023/A:1022126007684
- Choi , SE and Na , SD. 2010 . Review of the description pattern of newly recorded insect species from 1999 to 2009 in Korea . Anim Cells Syst , 14 ( 3 ) : 207 – 212 . doi: 10.1080/19768354.2010.504335
- Furuya , K , Takahshi , M and Nemoto , N. 1986 . Summer phytoplankton community structure and growth in a regional upwelling area off Hachijo Island, Japan . J Exp Mar Biol Ecol , 96 : 43 – 55 . doi: 10.1016/0022-0981(86)90012-2
- Frost , BW. 1980 . “ Grazing ” . In The physiological ecology of phytoplankton , Edited by: Morris , I . 465 – 491 . Oxford , , UK : Blackwell .
- Ignatiades , L , Vassiliou , A and Karydis , M. 1985 . A comparison of phytoplankton biomass parameters and their interrelation with nutrients in Saronikos Gulf . Hydrobiologia , 128 : 201 – 206 . doi: 10.1007/BF00006815
- Imamura , H , Tomoda , K , Suzuki , T and Hosono , S. 1995 . Research on construction of artificial upwelling current generating fishing ground . Ann J Coast Eng , 42 : 1131 – 1136 .
- Jung , SW , Kwon , OY , Joo , HM and Lee , JH. 2007 . Variation of phytoplankton standing crop affecting by environmental factors in the marine ranching ground of Tongyeong coastal water from 2000 to 2007 . Kor J Environ Biol , 25 ( 4 ) : 303 – 312 .
- Kang , PJ , Kim , YS and Nam , KW. 2008 . Flora and community structure of benthic marine algae in Ilkwang Bay, Korea . Algae , 23 : 1 – 10 . doi: 10.4490/ALGAE.2008.23.1.001
- Kang , YS. 2008 . Seasonal variation in zooplankton related to North Pacific regime shift in Korea Sea . J Korea Fish Soc , 41 : 493 – 504 .
- Kim , DS and Hwang , SB. 2006 . Charateristics of oceanographic environment in a sea area for the building of artificial upwelling structure . J Kor Soc Mar Environ Saf , 12 : 1 – 8 .
- Kim , JH , Ko , YD , Kim , YS and Nam , KW. 2011 . Marine algal flora and community structure of Gogunsan Islands outside the Saemangeum dike . Kor J Env Eco , 25 ( 2 ) : 156 – 165 .
- Kim KW. 2008 . Cultivation of marine microalgae from east sea and production of bioactive compounds by them using deep seawater . MSc, thesis . Gangwon , , Korea : National University of Kangnung .
- Kim YN. 2002 . Size fractionation and flow cytometric analysis of phytoplankton with changes in upwelling event off Gampo . MSc, thesis . Busan , , Korea : National University of Pukyong .
- KORDI . 2000 . Dokdo ecosystems and basic research BSPM 99045–00-1282-6 . 1033 .
- Lee , CR , Park , C and Moon , CH. 2004 . Appearance of cold water and distribution of zooplankton off Ulsan-Gampo area, eastern coastal area of Korea . The Sea , 9 : 51 – 63 .
- Lee , GH , Yoo , HI and Cho , HG. 2007 . Seasonal communitystructure and vertical distribution of medicinal seaweeds at Kkotji in Taean peninsula, Korea . Algae , 22 : 209 – 219 . doi: 10.4490/ALGAE.2007.22.3.209
- Lee , HG , Jung , SW and Choi , JK. 2012 . Spatial analysis of ecological characteristics for benthic macroinvertebrate community structure in Lake Hoengseong Region . Kor J Env Eco , 26 ( 1 ) : 45 – 56 .
- Lee , JH and Yoon , SM. 2000 . Water quality and phytoplankton communities in Yeoja Bay of Korea . Algae , 15 : 89 – 98 .
- Lee , JH and Lee , EH. 1999 . Water quality and phytoplankton red tide in Deukryang Bay of Korea . Kor Soc Environ Biol , 17 : 271 – 278 .
- Lee , JW and Kang , YS. 1994 . Variations of fish community and density on artificial reefs . Fish Aquat Sci , 27 : 535 – 548 .
- Margalef , R. 1958 . Information theory in ecology . Gen Syste , 3 : 36 – 71 .
- Mitchell-Innes , BA and Walker , DR. 1991 . Short-term variability during an actor station study in the southern Benguela upwelling system: phytoplankton production and biomass in relation to specie changes . Prog Oceanog , 28 : 65 – 89 . doi: 10.1016/0079-6611(91)90021-D
- Nakashima T. 2005 . What is deep ocean water; The utility of deep ocean water . In: Kim HJ , Kaiyoushinsousui no riyou [Development and utility of deep ocean water] . Seoul : Shingisul Press . 53 – 67 .
- Nicholas , W , Atsuki , K , Shigenao , M , Masud , B and Steve , WA. 2009 . Nutrient transport from an artificial upwelling of deep sea water . J Oceanog , 65 : 349 – 359 . doi: 10.1007/s10872-009-0032-x
- Ohno , M , Arai , S and Watanabe , M. 1990 . Seaweed succession on artificial reefs on different bottom substrata . J Appl Phycol , 2 : 327 – 332 . doi: 10.1007/BF02180922
- Oh , SJ and Yoon , YH. 2008 . Environmental factor on the succession of phytoplankton community in Jinju Bay, Korea . J Kor Soc Mar Environ Eng , 11 : 98 – 104 .
- Okaichi T , Yanagi T . 1997 . Sustainable development in the Seto Inland Sea Japan – from the viewpoint of fisheries . TERRAPUB ; 329
- Park , C , Lee , PG and Lee , CR. 1998 . Seasonal variation in abundance, species occurrence and species diversity of zooplankton in Asan Bay, the Yellow Sea over the last eight years . Yellow Sea , 4 : 40 – 48 .
- Park , HH and Shin , JK. 2003 . An effect on fisheries resources enhancement of hollow jumbo structure and a search for artificial reefs by side scan sonar in the western Sea of Kor Soc . Fish Technol , 39 : 230 – 238 .
- Peterson , W. 1998 . Life cycle strategies of copepods in coastal upwelling zones . J Mar Syst , 15 : 313 – 326 . doi: 10.1016/S0924-7963(97)00082-1
- Pickering , H and Whitmarsh , D. 1997 . Artificial reefs and fisheries exploitation: a review of the ‘attraction versus production’ debate, the influence of design and its significance for policy . Fish Res , 31 : 39 – 59 . doi: 10.1016/S0165-7836(97)00019-2
- Pielou , EC. 1966 . The measurement of diversity in different types of biological collections . J Theor Biol , 13 : 131 – 144 . doi: 10.1016/0022-5193(66)90013-0
- Pimm SL. 1984 . The complexity and stability of ecosystem . Nature 307 : 321 – 326 . doi: 10.1038/307321a0
- Reed , C. 1978 . Species diversity in aquatic microecosystems . Ecology , 59 : 481 – 488 . doi: 10.2307/1936578
- Ryther , JH. 1969 . Photosynthesis and fish production in the sea, the production of organic matter and its conversation to higher forms of life vary throughout the world ocean . Science , 166 : 72 – 76 . doi: 10.1126/science.166.3901.72
- Serisawa , Y and Ohno , M. 1995 . Succession of seaweed communities on artificial reefs in the inlet of Tosa bay, Japan . Suisan zoshoku , 43 : 437 – 443 .
- Shannon , CE and Wiener , W. 1963 . The mathematical theory to communication , 125 Urban , IL : University of Illinois Press .
- Smith , DL and Johnson , K. 1996 . A guide to marine coastal plankton and marine invertebrate larvea , 221 Dubuque , IA : Kendall/Hunt Publishing Company .
- Tilman D. 2000 . Causes, consequences and ethics of biodiversity . Nature 405 : 208 – 211 . doi: 10.1038/35012217
- Yanagi , T and Nakajima , M. 1991 . Change of oceanic condition by the man-made structure for upwelling . Mar Pollut Bull , 23 : 131 – 135 . doi: 10.1016/0025-326X(91)90662-C
- Yang , HS , Oh , SJ , Lee , HP , Moon , CH , Han , MS and Kim , BK. 1998 . Distribution of particulate organic matter in the Gampo upwelling area of the southwestern East Sea . J Oceanol Soc Kor , 33 : 157 – 167 .
- Yoo KI. 1995 . Illustrated encyclopedia of fauna and flora of Korea, marine zooplankton . 35 . Seoul : The Ministry of Education ; 415 .
- Young , CM. 2002 . Atlas of marine invertebrate larvae , 626 London : Academic press .