Abstract
One neuron receives thousands of inputs through synapses, which contain distinct molecular components and display different properties. It has been a major challenge in neuroscience to understand the development and function of specific synapses. One of the critical molecules involved in shaping synapse-specific properties is the cell adhesion molecule (CAM). Remarkable numbers of studies have shown the importance of cell-cell interaction mediated by various types of CAMs in defining synapse-specific function. Here, we summarize current understanding of CAMs playing a pivotal role in constructing neural circuits and guiding synapse-specific plasticity. Different CAMs localized at specific synapses are discussed in this review.
Introduction
In the neural circuits, synaptic contacts mediate the information flow between pre- and postsynaptic neurons. Each synapse is composed of distinct molecules with a specific chemical nature of neurotransmitters. The terminal of the presynaptic neuron contains active zone where synaptic vesicles are ready to be released (Südhof, Citation2012). Postsynaptic membrane contacting the presynaptic terminal contains receptors for certain neurotransmitters and proteins constructing postsynaptic density (Sheng, Citation2001). Precise control of the synaptic contact is important for the accurate information processing within a given neural circuit and not to cause any errors of animal perception or behavior.
How are the neural circuits built in such a precise and accurate way? Early studies in the visual cortex have discovered that activity of neurons (either spontaneous or experience-dependent) is critical for establishing functional neural circuits in vivo (Wiesel & Hubel, Citation1963; Katz & Shatz, Citation1996). However, Roger Sperry suggested that the cytochemical molecules guide the development of neural circuits, and neurons and fibers contain individual identification tags, which distinguish one from another in the level of single neurons (Sperry, Citation1963). Supporting this idea, many lines of studies have identified guidance molecules, which are released from the neuron or tagged on the extracellular side of the neuron. One of the critical molecules that are exposed from each neuron is the cell-adhesion molecule (CAM) (Washbourne et al., Citation2004). The adhesive property of the extracellular domain of CAMs is crucial to provide stable interactions between pre- and postsynaptic neurons in building specific synaptic contacts. Molecular mechanisms of homophilic and heterophilic interactions between various CAM molecules have been suggested to be necessary for establishing billions of synaptic contacts between neurons in the brain.
CAMs not only work as a guiding molecule to construct neural circuits, but they are also important for adjusting synaptic properties in adult neural circuits. As a core component of active synapses, CAMs are involved in signal transduction mediated by activity between neurons. Therefore, understanding the roles of CAMs in a specific spatiotemporal window of neuronal development and plasticity is required for us to draw a big picture of their function. Here, we summarize roles of different types of CAMs in defining synapse specificity in various nervous systems (). This review further suggests insights into molecular mechanisms by which CAMs establish synapse-specific contacts and control synaptic plasticity.
Figure 1. A schematic illustration of cell adhesion molecules. Dscam, down syndrome cell adhesion molecule; L1, L1 cell adhesion molecule; NCAM, neuronal cell adhesion molecule; Fasciclin-II, the Drosophila orthologue of neuronal cell adhesion molecule; TM-apCAM, transmembrane form of Aplysia cell adhesion molecule; GPI-linked apCAM, glycophosphatidylinositol-linked Aplysia cell adhesion molecule; SYG-1, cell adhesion molecule in C. elegans; SynCAM, vertebrate synaptic cell adhesion molecule; NLGN, neuroligin.
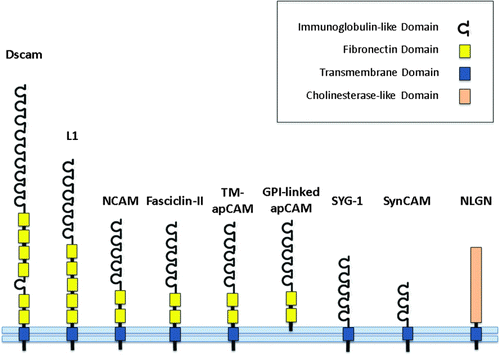
Role of CAMs during development and synaptogenesis
In the developing neural circuits, CAMs are active players in constructing specific axonal projections and synaptic contacts. By membrane-membrane adhesion, CAMs adjust the level of rigidity of the axon fascicles, the growth of the axon terminals, and the synaptic protrusion. One of the well-known CAMs in these processes is the CAM of the immunoglobulin (Ig) superfamily (IgCAM). IgCAM contains the homologous extracellular Ig domain that is particularly famous for the critical function in developing neurons (Walsh & Doherty, Citation1997). For example, two major IgCAM proteins, NCAM and L1, promote axonal growth and guidance through their extracellular Ig domains, which work as a co-receptor of growth hormones (Schmid & Maness, Citation2008). The Ig domains mediate not only homophilic interactions but also heterophilic interaction with other transmembrane proteins such as integrins. Recruitment of transmembrane proteins further initiates the signaling cascade through their intracellular domains by activating Src, Fyn, FAK, ERK, and the transcription factor cAMP response element binding protein. Major effect of this signaling pathway is the reorganization of actin cytoskeleton and neurite outgrowth. Therefore, as a guidance molecule, IgCAMs recruit other membrane proteins and intracellular signaling molecules to the stimulated area to enhance the guided growth of the neurites.
Physical growth of neurites is not the only function of CAMs; CAMs promote the formation of functional synapses. In the early study, it has been shown that the cell-cell interaction induces the redistribution of the cell surface receptors (Chow & Poo, Citation1982). More recent studies have shown that CAM-mediated contact between pre- and postsynaptic neurons triggers rapid redistribution of both pre- and postsynaptic compartments and promotes synapse formation. Although the exact determinants and the mediators during rapid synaptogenesis are still unclear, two major CAMs are known to be sufficient to drive the formation of functional synapses: synaptic CAM (SynCAM) and neuroligin (NLGN). SynCAM is one of IgCAMs and shows homophilic binding properties. It is homologous to the invertebrate IgCAM molecules such as fasciclin II and Aplysia CAM (apCAM) but expresses only in the mammalian brain. Interestingly, when the SynCAM-expressing non-neuronal cells contacted the axon terminal of neurons, the presynaptic terminal is rapidly organized at the contacting site (Biederer et al., Citation2002). Similarly, neuroligin promotes synapse formation at the contacting sites of the presynaptic terminal (Scheiffele et al., Citation2000; Fu et al., Citation2003). Five different neuroligin genes have been identified in different species including humans, and they all act as postsynaptic CAMs that bind to the specific alternative splicing isoforms of β-neurexin at the presynaptic terminals (Ichtchenko et al., Citation1995). Indeed, the neuroligin-neurexin interaction is the most well-known mechanism for setting up the asymmetry of the synaptic structures between neurons (Lisé & El-Husseini, Citation2006).
Neuroligins clearly show synaptogenic function in vitro (Scheiffele et al., Citation2000; Fu et al., Citation2003). However, genetic deletion of neuroligins did not alter the normal synapse density and synaptic ultrastructure in vivo (Varoqueaux et al., Citation2006; Kim et al., Citation2008; Blundell et al., Citation2010). These contradicting results have suggested that neuroligins are not required for the initial synaptic formation but for the maturation of the pre-existing synapses or normal synaptic function. Compromising two different stories, recent study has shown that the relative amount of neuroligin-1 in each neuron is critical for the synapse formation in vivo (Kwon et al., Citation2012). This study demonstrates that transcellular competition between neurons expressing different amount of neuroligins for binding fixed amount of neurexins governs the mechanism of the neuroligin-mediated synapse formation. Therefore, complete knockdown of neuroligin-1 diminishes the differences in the expression level of neuroligin-1 between neurons and compensates overall defects in synapse formation in vivo.
Both SynCAM and neuroligin are localized at postsynaptic membranes. However, their presynaptic partners might also be important for initiating the functional recruitment of postsynaptic molecules upon the binding, as they showed important roles in modulating the postsynaptic receptor properties (Ichtchenko et al., Citation1995; Graf et al., Citation2004; Kattenstroth et al., Citation2004; Heine et al., Citation2008). Future studies are required to determine the detailed mechanism of synapse formation in vivo and the role of different types of pre- and postsynaptic CAMs in forming asymmetric structure of synapses.
Determining synaptic properties: neuroligins
Distinct feature of the synapses is determined by the chemical property of the neurotransmitter in the presynaptic terminal and the receptors in the postsynaptic membrane. Each synapse belongs to either excitatory or inhibitory synapse based on the effect of its neurotransmitter on the postsynaptic neurons. In adult brain circuits, glutamate is the major excitatory transmitter while GABA is inhibitory. Although the combination of the neuroligin-neurexin interaction is not fully understood yet, a body of experimental evidence has suggested that neuroligin subtypes at the postsynaptic side of the neurons determine GABAergic and glutamatergic synaptogenesis. Endogenous neuroligins 1 and 3 are mainly observed at the excitatory synapses whereas neuroligin-2 localizes primarily to GABAergic inhibitory synapses (Song et al., Citation1999; Varoqueaux et al., Citation2004; Budreck & Scheiffele, Citation2007). Furthermore, knockdown or overexpression of neuroligins 1, 2, or 3 in cultured hippocampal neurons decreased or increased the number of excitatory and inhibitory synapses in a subtype-specific manner by disrupting the excitatory-inhibitory balance (Chih et al., Citation2005). These findings support the idea that neuroligins specify chemical nature of synapses where they are selectively localized.
Neuroligins 1 and 3 show further distinctive properties in regulating synaptic function, even though both of them are localized at the excitatory synapses. In adult mice, knock-out of neuroligin-1 causes deficits in memory and learning-related synaptic plasticity (Kim et al., Citation2008; Blundell et al., Citation2010), whereas knock-out of neuroligin-3 induces autism and impairment in social behavior without memory deficit (Radyushkin et al., Citation2009). Supporting this, neuroligin-1 but not neuroligin-3 is required for the induction of long-term potentiation, and this subtype-specific synaptic role is dependent on its extracellular domain of neuroligin-1 (Shipman & Nicoll, Citation2012). Therefore, molecular properties of neuroligins residing postsynaptically at each synapse determine the physiological properties of that synapse: not only the chemical transmission but also the synaptic plasticity. Neuroligin subtypes might act as a synaptic tag to define primary properties of synapses in one neuron receiving inputs from many other presynaptic neurons. Molecular mechanisms of synapse-specific localization of neuroligin subtypes within a single neuron, which is intermingled with many other neurons in a specific brain circuit in vivo, will be interesting to study in the future.
Dynamic adjustment of synaptic transmission requires activity-dependent modification of the synaptic molecules, which can lead to functional changes of the synapse. Interestingly, recent studies have shown that enhanced neuronal activity increases the cleavage of neuroligin-1 both in vitro and in vivo (Peixoto et al., Citation2012; Suzuki et al., Citation2012). This activity-dependent cleavage of neuroligin-1 reduces the net synaptic transmission in the local area, which explains a homeostatic plasticity in neuronal networks. Therefore, neuroligin-1 selectively adjusts synaptic transmission in highly active synapses amongst the abundant excitatory synaptic contacts present in neural circuits.
Synapse-specific function of invertebrate CAMs
Invertebrate CAMs have been identified as a critical molecule-regulating neuronal development and synaptic plasticity. Earlier studies in Aplysia have found that CAMs are critically involved in learning-related synaptic growth (Bailey & Kandel, Citation1993). In particular, the transmembrane isoform of Aplysia CAM (TM-apCAM) is known to play an important role in regulating synaptic strength in pre-existing synapses (Han et al., 2004) and during learning-related synaptic growth in Aplysia (Bailey et al., Citation1992). More recently, CAMAP, the intracellular binding partner of TM-apCAM, has been identified as a retrograde signaling molecule initiating gene transcription during synapse-specific form of long-term facilitation (Lee et al., Citation2007). Thus, TM-apCAM holds the ready-to-release intracellular messenger (CAMAP) in each synapse and transmits synapse-specific information to the nucleus upon receiving the facilitating signal at the synaptic terminal. TM-apCAM is internalized after dissociation of CAMAP, and this may lead to the synapse-specific growth (Martin et al., Citation1997; Lee et al., Citation2012). Future studies will be required to understand the role of TM-apCAM in synapse-specific growth in Aplysia.
Another important CAM that is identified in Caenorhabditis elegans is the SYG-1 (Shen & Bargmann, Citation2003). SYG-1 is the IgCAM expressed in HSNL motor neuron and binds to SYG-2 that is transiently expressed in the epithelial neurons. A heterophilic interaction between SYG-1 and SYG-2 is important for the target-specific synapse formation (Shen et al., Citation2004). Both apCAM and SYG-1 are the presynaptic CAMs, suggesting that the presynaptic CAMs are critical in both developing specific synapses onto the right targets and mediating synaptic plasticity and growth.
Beyond molecular identity of different CAMs, alternative splicing variants in each CAM gene provide further diversity of CAM populations. Large numbers of CAM isoforms create specific identity of axonal branches and synapses in neurons through selective expression and localization of distinct isoforms. Dscam, a well-known Drosophila CAM molecule, potentially generates 38,016 different messenger RNA isoforms through alternative splicing (Schmucker et al., Citation2000). These variants are required for developing specific connectivity between large numbers of axonal branches in Drosophila (Chen et al., Citation2006). Overall, besides the mammalian CAMs, invertebrate CAMs show functional diversity and specificity at the level of single axonal branch and synapse, supporting the idea that CAM is a genuine molecular identity defining synapse-specific function throughout different animal species.
CAM dysfunction and psychiatric disorders
As described above, CAMs are important for the development of neural circuits as well as adult synaptic plasticity. Therefore, dysfunction of CAMs can cause severe deficits in normal brain functions. Some CAMs are known to be related to psychiatric disorders. For example, the level of neural CAM (NCAM) is increased in patients with schizophrenia (Lyons et al., Citation1988), while it decreases in patients with autism (Plioplys et al., Citation1989). Although direct causal link of the NCAM function and these psychiatric disorders are not yet known, it has been suggested that abnormal function of NCAM may underlie dysfunction of synapses related with neuropsychiatric disorders (Vawter, Citation2000).
More recently, it has been revealed that the specific mutation of neuroligin-3 causes autism-related behaviors and increases inhibitory synaptic transmission in mice (Tabuchi et al., Citation2007). Interestingly, the mutation but not the deletion of neuroligin-3 caused specific behavioral changes, such as decreasing social behavior and increasing learning, indicating mere functional defects in neuroligin-3 does not account for the autism-like behavior. Furthermore, this mutation selectively increases inhibitory synaptic transmission at both hippocampus and cortex. This clearly suggests that disruption in normal function of neuroligin-3 induces autism-like behavior by altering synaptic function at specific synapses. Beyond the general increase in the inhibitory synaptic conductance, circuits or cell types involved in this change need to be disentangled in future experiments.
Conclusion
CAMs are critical in shaping normal brain circuits and function throughout the animal kingdom. At specific branches, axons, and synapses within a single neuron, CAMs establish precise synaptic structure and mediate activity-dependent synaptic signals. Future studies are required to understand the molecular mechanism on how different CAM molecules are localized at certain synaptic contacts and the physiological impact of synapse-specific CAMs on shaping activity patterns of neural circuits in vivo.
References
- Bailey , CH , Chen , M , Keller , F and Kandel , ER. 1992 . Serotonin-mediated endocytosis of apCAM: An early step of learning-related synaptic growth in Aplysia . Science , 256 : 645 – 649 . doi: 10.1126/science.1585177
- Bailey , CH and Kandel , ER. 1993 . Structural changes accompanying memory storage . Ann Rev Physiol , 55 : 397 – 426 . doi: 10.1146/annurev.ph.55.030193.002145
- Biederer , T , Sara , Y , Mozhayeva , M , Atasoy , D , Liu , X , Kavalali , ET and Sudhof , TC. 2002 . SynCAM, a synaptic adhesion molecule that drives synapse assembly . Science , 297 : 1525 – 1531 . doi: 10.1126/science.1072356
- Blundell , J , Blaiss , CA , Etherton , MR , Espinosa , F , Tabuchi , K , Walz , C , Bolliger , MF , Sudhof , TC and Powell , CM. 2010 . Neuroligin-1 deletion results in impaired spatial memory and increased repetitive behavior . J Neurosci , 30 : 2115 – 2129 . doi: 10.1523/JNEUROSCI.4517-09.2010
- Budreck , EC and Scheiffele , P. 2007 . Neuroligin-3 is a neuronal adhesion protein at GABAergic and glutamatergic synapses . Eur J Neurosci , 26 : 1738 – 1748 . doi: 10.1111/j.1460-9568.2007.05842.x
- Chen , BE , Kondo , M , Garnier , AL , Watson , FL , Puettmann-Holgado , R , Lamar , DR and Schmucker , D. 2006 . The molecular diversity of Dscam is functionally required for neuronal wiring specificity in Drosophila . Cell , 125 : 607 – 620 . doi: 10.1016/j.cell.2006.03.034
- Chih , B , Engelman , H and Scheiffele , P. 2005 . Control of excitatory and inhibitory synapse formation by neuroligins . Science , 307 : 1324 – 1328 . doi: 10.1126/science.1107470
- Chow , I and Poo , MM. 1982 . Redistribution of cell surface receptors induced by cell-cell contact . J Cell Biol , 95 : 510 – 518 . doi: 10.1083/jcb.95.2.510
- Fu , Z , Washbourne , P , Ortinski , P and Vicini , S. 2003 . Functional excitatory synapses in HEK293 cells expressing neuroligin and glutamate receptors . J Neurophysiol , 90 : 3950 – 3957 . doi: 10.1152/jn.00647.2003
- Graf , ER , Zhang , XZ , Jin , SX , Linhoff , MW and Craig , AM. 2004 . Neurexins induce differentiation of GABA and glutamate postsynaptic specializations via neuroligins . Cell , 119 : 1013 – 1026 . doi: 10.1016/j.cell.2004.11.035
- Han , JH , Lim , CS , Lee , YS , Kandel , ER and Kaang , BK. 2004 . Role of Aplysia cell adhesion molecules during 5-HT-induced long-term functional and structural changes . Learn Memory , 11 : 421 – 435 . doi: 10.1101/lm.61104
- Heine , M , Thoumine , O , Mondin , M , Tessier , BA , Giannone , GG and Choquet , D. 2008 . Activity-independent and subunit-specific recruitment of functional AMPA receptors at neurexin/neuroligin contacts . Proc Natl Acad Sci , 105 : 20947 – 20952 . doi: 10.1073/pnas.0804007106
- Ichtchenko , K , Hata , Y , Nguyen , T , Ullrich , B , Missler , M , Moomaw , C and Sudhof , TC. 1995 . Neuroligin 1: A splice site-specific ligand for β-neurexins . Cell , 81 : 435 – 443 . doi: 10.1016/0092-8674(95)90396-8
- Kattenstroth , G , Tantalaki , E , Sudhof , TC , Gottmann , K and Missler , M. 2004 . Postsynaptic N-methyl-d-aspartate receptor function requires β-neurexins . Proc Natl Acad Sci , 101 : 2607 – 2612 . doi: 10.1073/pnas.0308626100
- Katz , LC and Shatz , CJ. 1996 . Synaptic activity and the construction of cortical circuits . Science , 274 : 1133 – 1138 . doi: 10.1126/science.274.5290.1133
- Kim , J , Jung , S-Y , Lee , YK , Park , S , Choi , J-S , Lee , CJ , Kim , H-S , Choi , Y-B , Scheiffele , P Bailey , CH . 2008 . Neuroligin-1 is required for normal expression of LTP and associative fear memory in the amygdala of adult animals . Proc Natl Acad Sci , 105 : 9087 – 9092 . doi: 10.1073/pnas.0803448105
- Kwon , H-B , Kozorovitskiy , Y , Oh , W-J , Peixoto , RT , Akhtar , N , Saulnier , JL , Gu , C and Sabatini , BL. 2012 . Neuroligin-1-dependent competition regulates cortical synaptogenesis and synapse number . Nat Neurosci , 15 : 1667 – 1674 . doi: 10.1038/nn.3256
- Lee , S-H , Lim , C-S , Park , H , Lee , J-A , Han , J-H , Kim , H , Cheang , Y-H , Lee , S-H , Lee , Y-S Ko , H-G . 2007 . Nuclear translocation of CAM-associated protein activates transcription for long-term facilitation in aplysia . Cell , 129 : 801 – 812 . doi: 10.1016/j.cell.2007.03.041
- Lee , S-H , Shim , J , Choi , S-L , Lee , N , Lee , C-H , Bailey , CH , Kandel , ER , Jang , D-J and Kaang , B-K. 2012 . Learning-related synaptic growth mediated by internalization of Aplysia cell adhesion molecule is controlled by membrane phosphatidylinositol 4,5-bisphosphate synthetic pathway . J Neurosci , 32 : 16296 – 16305 . doi: 10.1523/JNEUROSCI.1872-12.2012
- Lisé , M-F and El-Husseini , A. 2006 . The neuroligin and neurexin families: from structure to function at the synapse . Cell Mol Life Sci , 63 : 1833 – 1849 .
- Lyons , F , Martin , ML , Maguire , C , Jackson , A , Regan , CM and Shelley , RK. 1988 . The expression of an N-CAM serum fragment is positively correlated with severity of negative features in type II schizophrenia . Biol Psychiat , 23 : 769 – 775 . doi: 10.1016/0006-3223(88)90065-0
- Martin , KC , Casadio , A , Zhu , HEY , Rose , JC , Chen , M , Bailey , CH and Kandel , ER. 1997 . Synapse-specific, long-term facilitation of Aplysia sensory to motor synapses: A function for local protein synthesis in memory storage . Cell , 91 : 927 – 938 . doi: 10.1016/S0092-8674(00)80484-5
- Peixoto , RT , Kunz , PA , Kwon , H , Mabb , AM , Sabatini , BL , Philpot , BD and Ehlers , MD. 2012 . Transsynaptic signaling by activity-dependent cleavage of neuroligin-1 . Neuron , 76 : 396 – 409 . doi: 10.1016/j.neuron.2012.07.006
- Plioplys , AV , Hemmens , SE and Regan , CM. 1989 . Expression of a neural expression of a neural cell-adhesion molecule serum fragment is depressed in autism . Annal Neurol , 26 : 483–483
- Radyushkin , K , Hammerschmidt , K , Boretius , S , Varoqueaux , F , El-Kordi , A , Ronnenberg , A , Winter , D , Frahm , J , Fischer , J , Brose , N and Ehrenreich , H. 2009 . Neuroligin-3-deficient mice: Model of a monogenic heritable form of autism with an olfactory deficit . Genes Brain Behav , 8 : 416 – 425 . doi: 10.1111/j.1601-183X.2009.00487.x
- Scheiffele , P , Fan , J , Choih , J , Fetter , R and Serafini , T. 2000 . Neuroligin expressed in non-neuronal cells triggers presynaptic development in contacting axons . Cell , 101 : 657 – 669 . doi: 10.1016/S0092-8674(00)80877-6
- Schmid , RS and Maness , PF. 2008 . L1 and NCAM adhesion molecules as signaling co-receptors in neuronal migration and process outgrowth . Cur Opin Neurobiol , 18 : 245 – 250 . doi: 10.1016/j.conb.2008.07.015
- Schmucker , D , Clemens , JC , Shu , H , Worby , CA , Xiao , J , Muda , M , Dixon , JE and Zipursky , SL. 2000 . Drosophila Dscam is an axon guidance receptor exhibiting extraordinary molecular diversity . Cell , 101 : 671 – 684 . doi: 10.1016/S0092-8674(00)80878-8
- Shen , K and Bargmann , CI. 2003 . The immunoglobulin superfamily protein SYG-1 determines the location of specific synapses in C. elegans . Cell , 112 : 619 – 630 . doi: 10.1016/S0092-8674(03)00113-2
- Shen , K , Fetter , RD and Bargmann , CI. 2004 . Synaptic specificity is generated by the synaptic guidepost protein SYG-2 and its receptor, SYG-1 . Cell , 116 : 869 – 881 . doi: 10.1016/S0092-8674(04)00251-X
- Sheng , M. 2001 . Molecular organization of the postsynaptic specialization . Proc Natl Acad Sci , 98 : 7058 – 7061 . doi: 10.1073/pnas.111146298
- Shipman , SL and Nicoll , RA. 2012 . A subtype-specific function for the extracellular domain of neuroligin 1 in hippocampal LTP . Neuron , 76 : 309 – 316 . doi: 10.1016/j.neuron.2012.07.024
- Song , J-Y , Ichtchenko , K , Sudhof , TC and Brose , N. 1999 . Neuroligin 1 is a postsynaptic cell-adhesion molecule of excitatory synapses . Proc Natl Acad Sci , 96 : 1100 – 1105 . doi: 10.1073/pnas.96.3.1100
- Sperry , RW. 1963 . Chemoaffinity in the orderly growth of nerve fiber patterns and connections . Proc Natl Acad Sci , 50 : 703 – 710 . doi: 10.1073/pnas.50.4.703
- Südhof , TC. 2012 . The presynaptic active zone . Neuron , 75 : 11 – 25 . doi: 10.1016/j.neuron.2012.06.012
- Suzuki , K , Hayashi , Y , Nakahara , S , Kumazaki , H , Prox , J , Horiuchi , K , Zeng , M , Tanimura , S , Nishiyama , Y Osawa , S. 2012 . Activity-dependent proteolytic cleavage of neuroligin-1 . Neuron , 76 : 410 – 422 . doi: 10.1016/j.neuron.2012.10.003
- Tabuchi , K , Blundell , J , Etherton , MR , Hammer , RE , Liu , X , Powell , CM and Suedhof , TC. 2007 . Neuroligin-3 mutation implicated in autism increases inhibitory synaptic transmission in mice . Science , 318 : 71 – 76 . doi: 10.1126/science.1146221
- Varoqueaux , FDR , Aramuni , G , Rawson , RL , Mohrmann , R , Missler , M , Gottmann , K , Zhang , W , Sudhof , TC and Brose , N. 2006 . Neuroligins determine synapse maturation and function . Neuron , 51 : 741 – 754 . doi: 10.1016/j.neuron.2006.09.003
- Varoqueaux , FDR , Jamain , SP and Brose , N. 2004 . Neuroligin 2 is exclusively localized to inhibitory synapses . Eur J Cell Biol , 83 : 449 – 456 . doi: 10.1078/0171-9335-00410
- Vawter , MP. 2000 . Dysregulation of the neural cell adhesion molecule and neuropsychiatric disorders . Eur J Pharmacol , 405 : 385 – 395 . doi: 10.1016/S0014-2999(00)00568-9
- Walsh , FS and Doherty , P. 1997 . Neural cell adhesion molecules of the immunoglobulin superfamily: role in axon growth and guidance . Annual Rev Cell Develop Biol , 13 : 425 – 456 . doi: 10.1146/annurev.cellbio.13.1.425
- Washbourne , P , Dityatev , A , Scheiffele , P , Biederer , T , Weiner , JA , Christopherson , KS and El-Husseini , A. 2004 . Cell adhesion molecules in synapse formation . J Neurosci , 24 : 9244 – 9249 . doi: 10.1523/JNEUROSCI.3339-04.2004
- Wiesel , TN and Hubel , DH. 1963 . Single-cell responses in striate cortex of kittens deprived of vision in one eye . J Neurophysiol , 26 : 1003 – 1017 .