Abstract
Induced pluripotent stem (iPS) cells derive from autologous somatic cells, the application prospect of iPS cells for regenerative medicine and tissue engineering is better than embryonic stem cells (ESCs) to some extent. Alveolar type II (AT II) epithelial cells play key role in the injured lung tissue regeneration and function recovery. The differentiation of iPS cells into AT II cells could provide available source for injured lung treatment. In this study, rat iPS (riPS) cells were resuscitated and proliferated for 14 days before differentiation. A modified three-step induction protocol similar to the reported ESCs inducing procedure was used in this study for the differentiation groups. Routine cell culture was done to the riPS cell control group (riPS-con). At stage 3, cells of day 7 (Diff. 7) and day 14 (Diff. 14) were collected for the real-time polymerase chain reaction tests for gene expressions of Oct4, Nanog, SPA, SPB, SPC, SPD, and CC10. Immunofluorescence staining of SPC and SSEA-1 was conducted. At the end of the differentiation, cell morphology became outstretched and epithelium-like. Cells of the Diff. 14 group positively expressed SPC and negatively expressed SSEA-1, which is contrary to the riPS-con group. In the Diff. 7 and the Diff. 14 groups, the expression of Oct4, Nanog, and SPB decreased (P < 0.05), whereas the expression of SPA, SPC, SPD (P < 0.05), and CC10 (P > 0.05) increased. This study indicated that riPS cells can successfully differentiate into AT II epithelial cells with the three-step induction protocol and may be further applied to implanting in decellularized rat lung scaffolds and building a bio-artificial lung.
Introduction
Lung transplantation is the main treatment of end-stage diseases. However, a scarcity of suitable donor lungs largely restricts the development of lung transplantation, which calls for acute clinical alternatives. Regenerative medicine is a newly developed method that grows healthy cells in vitro and implants these cells into the injured organ to restore its physiological functions. Lung is a vital organ with numerous generations of branching leading to the alveoli, which include various types of cells in each section of the airway (Rippon et al. Citation2006). These complex structure and cell types bring the development of pulmonary regenerative therapy formidable challenges. Alveolar type II (AT II) epithelium cells, which comprise 5% of the alveolar lining, are crucial cells to alveolar homeostasis and repair (Otto Citation1997). These cells secret pulmonary surfactants that are indispensable to lower alveolar surface tension and prevent airway collapse. They also have the ability to undergo proliferation and differentiation to be Alveolar type I (AT I) epithelium cells (Otto Citation1997), that comprise 95% of the alveolar lining. Thus, the growth of normal and functional AT II epithelium cells in vitro shed light on the development of end-stage lung disease therapy.
Induced pluripotent stem (iPS) cells are novel stem cell populations induced from mouse, rat, or human adult somatic cells through reprogramming by transduction of defined transcription factors (Takahashi & Yamanaka Citation2006a; Okita et al. Citation2007; Li et al. Citation2009). These cells demonstrate the same proliferation and differentiation potentials as embryonic stem cells (ESCs) and could avoid the immune rejection reactions after implantation since its autologous cell derivation. Several studies reported that mature AT II epithelium cells could be detected in differentiated murine and human ESC populations (Ali et al. Citation2002; Rippon et al. Citation2004). Murine iPS cell-derived AT II were also successfully achieved lately (Alipio et al. Citation2011; Yang et al. Citation2011; Banerjee & Henderson Citation2012; Schmeckebier et al. Citation2013). However, there is no report about the rat iPS (riPS) cells differentiation into AT II epithelial cells. In this study, we used a three-stage differentiation protocol similar to the inducing methods that reported by Rippon et al. (Citation2006) to study the possibility of development of AT II cells from iPS cells in rat.
Materials and methods
Resuscitation and proliferation of rat-induced pluripotent stem cells
Rat-induced pluripotent stem cell (riPSC) line (Sidansai, Shanghai, China) was used in this study. The freezing tube was taken out from the liquid nitrogen and immersed into 37°C water bath. The melted cell suspension was transferred into riPSCs complete medium (Sidansai, Shanghai, China). The procedure of centrifugation (1000 rpm, 5 minutes) and re-suspension (with 2 ml/4 ml riPSCs complete medium) should be repeated twice in order to remove the residual component of the previous frozen stock solutions. The cell suspension was transferred into T25 culture bottle pre-covered with mouse embryo fibroblast (MEF) cells (Cellapy, Beijing, China) and was added to the 5 ml of total volume of complete medium. Daily culture medium replacement was carried out. riPSCs were passaged by a ratio of 1 to 10 till cells spread to more than 80% of the bottom of the culture bottle. Cell passage was conducted every 2–3 day since the 4th or 5th day of cell resuscitation. After 2 weeks of cell proliferation, differentiation started.
Differentiation of riPSCs
After the proliferation of riPSCs, cells were digested with 0.25% trypsin. Differential adhesion method was used to remove MEF cells in order not to influence the following differentiation. Embryoid bodies (EBs) were formed 24 hours post-passage. The differentiation protocol used in this study for iPSCs included three stages similar to the methods for ESCs (Rippon et al. Citation2006).
Stage 1
EBs were suspension cultured in 9 cm peri-dishes with Dulbecco's Modified Eagle Medium (DMEM) contained 10% fetal bovine serum (FBS) for 2.5 days. Then, culture medium was replaced with DMEM that contained 10% knock out serum replacement (KOSR) and 100 ng/ml Activin A during the next 4.5 days. During the following 4 days, 10% KOSR DMEM was used.
Stage 2
EBs were adherent cultured in 6-well culture plates (pre-covered with gelatin) with 10% FBS DMEM for 10 days. And at the end of the 10th day, cells were digested with 0.25% trypsin and passage to new 6-well culture plates.
Stage 3
Small airway growth medium (Rippon et al. Citation2006) was used for cell culture in the next 4–14 days.
For the control group (riPS-con), routine riPS cell culture medium was used throughout the three steps as a time control to the differentiation groups.
Cells of the 7th (Diff. 7) and 14th (Diff. 14) day of the stage 3 were collected and stored for the follow-up immunofluorescence staining and real-time polymerase chain reaction (RT-PCR) analysis.
Immunofluorescence staining
Immunofluorescence staining was performed on actively dividing cells cultured in 24-well plates. Cells were fixed in 4% paraformaldehyde (PFA) for 30 minutes at room temperature. Cells were removed from the PFA and washed once with phosphate buffer solution and then 0.5% Triton was added for 15 minutes at room temperature. Then cells were washed twice with antibody dilution solution. Then cells were confined for 60 minutes with confining solution. Diluted the primary antibody (SPC and SSEA-1) in the antibody dilution solution (1:500 and 1:100), and put the solution in the cell well, stored the sample at 4 °C overnight. The secondary antibodies used were goat anti-mouse-phycoerythrin (PE) (Sigma, St Louis, MO), goat anti-rabbit-PE, and rabbit anti-goat-PE (both from Southern Biotech, Birmingham, Alabama). Nuclei were counterstained with 4′,6-diamidino-2-phenylindole (DAPI) (sigma) solution (diluted as 1:1000 in the antibody dilution solution) for 5 minutes. Images were captured by OLYMPUS IX71.
Real-time polymerase chain reaction
Total RNA was isolated using Trizol, chloroform, and isopropanal in the proportion of 5:1:2.5. Five micrograms of total RNA were reverse transcribed using cDNA archive kit (Invitrogen). PCR reaction system included primer (), template, SYBR mix (TOYOBO), and double-distilled H2O. The protocol is 95°C for 5 minutes (94°C 15 s, 66°C 10 s, and 72°C 20 s) × 40 cycles. Specific markers of rat-induced pluripotent cells (Oct4 and Nanog) and AT II epithelium cells (SPA, SPB, SPC, SPD, and CC10) were analyzed. The CT values of each group were tested. Internal control gene was G3PD-1. ΔCT represented the CT value of tested gene minus the CT value of G3PD-1. ΔΔCT equaled the ΔCT value of the differentiation group minus the ΔCT value of the control group. The relative gene expressions of each group were calculated as .
Table 1. RT-PCR primers.
Table 2. Abbreviations in this text.
Statistical analysis
Quantitative RT-PCR results () were analyzed by one-way analysis of variance with Tukey post-test.
Results
Cellular morphology variation during the procedure of differentiation
The differentiation procedure included three stages. During stage 1, cells were cultured in suspension medium. EBs formed gradually (as shown in , white arrow). EBs were adherently cultured since stage 2. After the adherence of EBs, cells grew radially (as shown in ). The radial growth indicated the differentiation of EBs. Cell density kept increasing (as shown in ), which represented the proliferation capacity of the differentiation cells. These cells might be the precursor cells. During stage 3, cell morphology became outstretched and epithelium-like. These cells (as shown in , black arrow) could possibly be AT II epithelium cells.
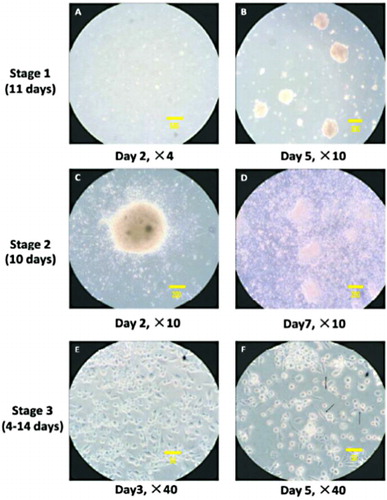
Immunofluorescence staining of SPC and SSEA-1
Immunofluorescence staining for the specific expression markers of AT II epithelial cells (SPC) and riPS cells (SSEA-1) was conducted. Cells of stage 3, day 14 (Diff. 14) were used for staining. SPC and SSEA-1 both existed in cytoplasm. The specific staining was in red color, differentiated from the blue color of DAPI staining for nuclei. Cells of the Diff. 14 group positively expressed SPC and negatively expressed SSEA-1. The percentage of SPC positive cells is about 10%. To the contrary, the cells of the riPS-con group counter-expressed the two markers (SPC, negative; SSEA-1, positive), as shown in and .
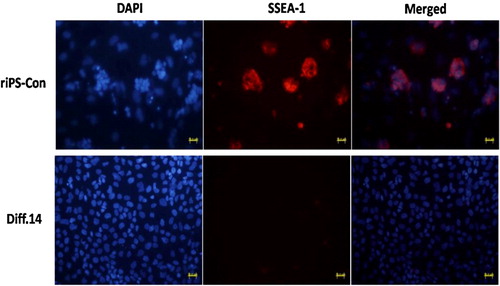
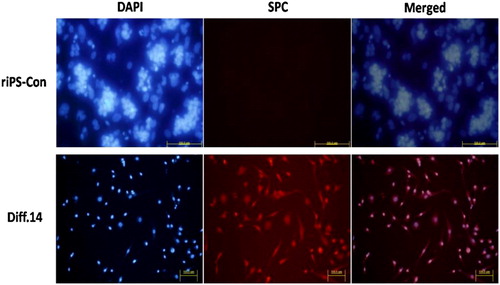
Quantitative RT-PCR
The primer sequence of each gene was shown in . After differentiation, the expression of Oct4 and Nanog decreased significantly (). The expression of SPA, SPC, and SPD increased significantly both in stage 3, day 7 (Diff. 7) and stage 3, day 14 (Diff. 14) groups. However, SPB gene expression decreased in the two groups. The expression of CC10 increased, but no significant differences were found, as shown in . The abbreviations in this text were shown in .
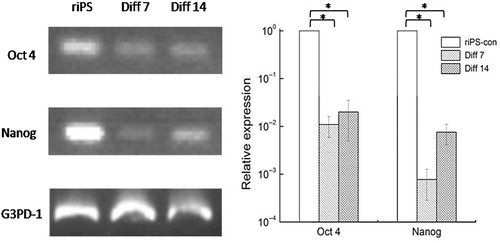
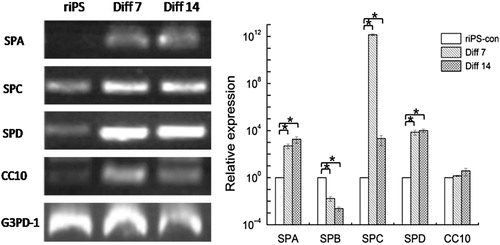
Discussion
Regenerative medicine and tissue engineering are relative newly developed therapeutic technologies for terminal lung diseases. The derivation of seed cells is one of the critical points for the cell replacement therapy and bio-artificial lung system development. AT II epithelial cells comprise 5% of the alveolar lining. These cells secret pulmonary surfactants that are indispensable to lower alveolar surface tension and prevent airway collapse. They also act as progenitor cells for AT I epithelium cells (Otto Citation1997; Reddy et al. Citation2004; Wetsel et al. Citation2011) that comprise 95% of the distal alveolar lining and work for lung air-exchange function.
Directed differentiation of stem cells for AT II epithelial cells opens a promising avenue for the clinical application and basic research of those biomedical technologies. There are quite many investigations focused on the generation of AT II cells from ESCs culture (Ali et al. Citation2002; Denham et al. Citation2006; Rippon et al. Citation2006; Samadikuchaksaraei et al. Citation2006; Yang et al. Citation2011). However, these procedures still have not achieved a level of purity that would be of clinical use and these mixed cell cultures may contain undifferentiated pluripotent cells that could develop into teratomas after transplantation. Mouse iPS cells were first developed by Yamanaka (Takahashi & Yamanaka Citation2006b) by introducing into mouse fibroblast through retroviral transduction. Since the derivation of autologous somatic cells of the iPS cells, it paves the way for the generation of patient-specific pluripotent cells that possess better biocompatibility and less immune reject reaction when used in tissue engineering and cell transplantation therapy.
We used a three-stage culture method, modified on the basis of the similar research with murine ESCs (Ali et al. Citation2002; Rippon et al. Citation2006) and murine iPS cells (Alipio et al. Citation2011), to induce differentiation of riPS cells into AT II epithelial cells in this study. At the end of differentiation, cells positively expressed SPC and negatively expressed SSEA-1. The gene expression of Oct4 and Nanog, the specific gene markers of pluripotent iPS cells, decreased significantly after differentiation. However, the specific gene markers of lung epithelial cells (SPA, SPC, SPD, and CC10) up-regulately expressed. These results indicated the cell characteristic transformation from the riPS cells into the AT II epithelial cells (SPA, SPC, and SPD) and bronchial epithelial cells (CC10 for clara cells) although the CC10 expression showed no significant up-regulation (P > 0.05).
To the contrary, the SPB gene expression did not increase after the differentiation in our study which was different from the characteristics of murine ESC-derived AT II cells reported by Rippon et al. (Citation2006) and Ali et al. (Citation2002). However, the research results of Wetsel (Wang et al. Citation2007; Citation2010) indicated that differentiated human ESCs into AT II epithelial cells were SPB deficiency, which was similar to our research. And the gene-corrected iPS cells derived from patients with SPB deficiency can be used clinically (Wetsel et al. Citation2011). SPA, SPB, and CC10 are the markers of mature distal lung epithelial. In this study, the inconsistent expression of the three genes may attribute to the reason that the cells developed at the period between the immature and mature phrase of AT II cell formation at the end of our differentiation protocol. SPD is also abundantly expressed in mature distal lung epithelium but is also widely expressed in other mucosal epithelia as part of host defense system and is not lung-specific (Rippon et al. Citation2006). The up-regulation of SPD suggested that the existing cells at the end of culture included not only lung epithelial cells but also other types, which need to do further identification evaluation.
We successfully induced the riPS cells into rat AT II epithelial cells with a three-stage induction protocol, provided theoretical basis and feasibility proof for the application of iPS cells-derived AT II cells into the biomedical treatment of terminal lung diseases. However, several limitations exist in this study. First, the culture period of the third stage seemed too short, that we did not observe clear mature characteristics of the AT II cells. Second, we only chose two time points for the cell RT-PCR tests of those specific marker gene expressions, which showed no variation details of those markers during the differentiation procedure.
Acknowledgments
This work was supported by the National Natural Science Foundation of China [Grant No. 31370993].
References
- Ali NN, Edgar AJ, Samadikuchaksaraei A, Timson CM, Romanska HM, Polak JM, Bishop AE. 2002. Derivation of type II alveolar epithelial cells from murine embryonic stem cells. Tissue Eng. 8:541–550. 10.1089/107632702760240463
- Alipio ZA, Jones N, Liao W, Yang J, Kulkarni S, Sree KK, Hauer-Jensen M, Ward, DC, Ma Y, Fink LM. 2011. Epithelial to mesenchymal transition (EMT) induced by bleomycin or TFG(b1)/EGF in murine induced pluripotent stem cell-derived alveolar type II-like cells. Differentiation. 82:89–98. 10.1016/j.diff.2011.05.001
- Banerjee ER, Henderson WJ. 2012. Characterization of lung stem cell niches in a mouse model of bleomycin-induced fibrosis. Stem Cell Res Ther. 3:21. 10.1186/scrt81
- Denham M, Cole TJ, Mollard R. 2006. Embryonic stem cells form glandular structures and express surfactant protein C following culture with dissociated fetal respiratory tissue. Am J Physiol Lung Cell Mol Physiol. 290:L1210–L1215.
- Li W, Wei W, Zhu S, Zhu J, Shi Y, Lin T, Hao E, Hayek A, Deng H, Ding S. 2009. Generation of rat and human induced pluripotent stem cells by combining genetic reprogramming and chemical inhibitors. Cell Stem Cell. 4:16–19. 10.1016/j.stem.2008.11.014
- Okita K, Ichisaka T, Yamanaka S. 2007. Generation of germline-competent induced pluripotent stem cells. Nature. 448:313–317. 10.1038/nature05934
- Otto WR. 1997. Lung stem cells. Int J Exp Pathol. 78:291–310. 10.1046/j.1365-2613.1997.370366.x
- Reddy R, Buckley S, Doerken M, Barsky L, Weinberg K, Anderson KD, Warburton D, Driscoll B. 2004. Isolation of a putative progenitor subpopulation of alveolar epithelial type 2 cells. Am J Physiol Lung Cell Mol Physiol. 286:L658–667. 10.1152/ajplung.00159.2003
- Rippon HJ, Ali NN, Polak JM, Bishop AE. 2004. Initial observations on the effect of medium composition on the differentiation of murine embryonic stem cells to alveolar type II cells. Cloning Stem Cells. 6:49–56. 10.1089/1536230041372328
- Rippon HJ, Polak JM, Qin M, Bishop AE. 2006. Derivation of distal lung epithelial progenitors from murine embryonic stem cells using a novel three-step differentiation protocol. Stem Cells. 24:1389–1398. 10.1634/stemcells.2005-0465
- Samadikuchaksaraei A, Cohen S, Isaac K, Rippon HJ, Polak JM, Bielby RC, Bishop AE. 2006. Derivation of distal airway epithelium from human embryonic stem cells. Tissue Eng. 12:867–875. 10.1089/ten.2006.12.867
- Schmeckebier S, Mauritz C, Katsirntaki K, Sgodda M, Puppe V, Duerr J, Schubert SC, Schmiedl A, Lin Q, Palecek J, et al. 2013. Keratinocyte growth factor and dexamethasone plus elevated cAMP levels synergistically support pluripotent stem cell differentiation into alveolar epithelial type II cells. Tissue Eng Part A. 19:938–951. 10.1089/ten.tea.2012.0066
- Takahashi K, Yamanaka S. 2006a. Induction of pluripotent stem cells from mouse embryonic and adult fibroblast cultures by defined factors. Cell. 126:663–676. 10.1016/j.cell.2006.07.024
- Takahashi K, Yamanaka S. 2006b. Induction of pluripotent stem cells from mouse embryonic and adult fibroblast cultures by defined factors. Cell. 126:663–676. 10.1016/j.cell.2006.07.024
- Wang D, Haviland DL, Burns AR, Zsigmond E, Wetsel RA. 2007. A pure population of lung alveolar epithelial type II cells derived from human embryonic stem cells. Proc Natl Acad Sci USA. 104:4449–4454. 10.1073/pnas.0700052104
- Wang D, Morales JE, Calame DG, Alcorn JL, Wetsel RA. 2010. Transplantation of human embryonic stem cell-derived alveolar epithelial type II cells abrogates acute lung injury in mice. Mol Ther. 18:625–634. 10.1038/mt.2009.317
- Wetsel RA, Wang D, Calame DG. 2011. Therapeutic potential of lung epithelial progenitor cells derived from embryonic and induced pluripotent stem cells. Annu Rev Med .62:95–105. 10.1146/annurev-med-052009-172110
- Yang KY, Shih HC, How CK, Chen CY, Hsu HS, Yang CW, Lee YC, Perng RP, Peng CH, Li HY, et al. 2011. IV Delivery of induced pluripotent stem cells attenuates endotoxin-induced acute lung injury in mice. Chest. 140:1243–1253. 10.1378/chest.11-0539