Abstract
Corticotropin-releasing hormone (CRH) is known to be involved in both antinociceptive and nociceptive processing. In the present study, we examined the role of the CRH receptor 1 in the regulation of nociception in mice. First, we found that CRH mRNA level was elevated in the hypothalamus or hippocampus by intraperitoneal (i.p.) injection with 1% acetic acid, intraplantar injection of 5% formalin, or intrathecal (i.t.) injection of substance P (0.7 μg/5 μl). Nociceptive behavior induced by acetic acid, formalin, or substance P were reduced in CRHR1 heterozygous (CRHR1+/−) mice compared to that in C57BL6 (wild type, CRHR1+/+) mice group. Furthermore, administration with CRHR1 antagonists such as CP 154526 (10 mg/kg, i.p.) or α-helical CRF 9-41 (10 μg/5 μl, intracerebroventricular [i.c.v.]) attenuated nociceptive behaviors observed in the substance P and writhing pain models. Our results suggest that CRHR1 might play an important role in the regulation of nociception, especially, toward nociceptive activation rather than producing the antinociception.
Keywords:
Introduction
Corticotropin-releasing hormone (CRH) is a peptide which consisted of 41 amino acids and widely distributed in the brain, including the hypothalamus and hippocampus (Chrousos & Gold Citation1992). CRH is a key mediator of the hypothalamic–pituitary–adrenal (HPA) axis response to stress and is also expressed in the peripheral tissues, such as skin, blood vessels, skeletal muscle, and central nervous system. Previously, several studies have reported that CRH located in the brain exerts a variety of physiological and pharmacological actions, including regulation of nociception. CRH receptors (CRHR) are currently divided into two types; CRHR1 and CRHR2. CRHR1 and CRHR2 are found to be located in the brain regions including the hypothalamus and hippocampus (Chen et al. Citation1993; Dautzenberg & Hauger Citation2002; Hauger et al. Citation2003). CRHR1, a 415-amino acid protein, exhibits high affinity toward CRH. CRHR1 is primarily expressed in the CNS and the anterior pituitary. The CRHR2 receptor shares 70% sequence identity with CRHR1 and is expressed primarily in extra-CNS sites. The CRHR2 receptors exhibit no affinity toward CRH (Bale Citation2005).
Currently, the role of CRH and CRHR in pain and analgesia has a controversy and was not fully evaluated. Earlier studies have shown that CRH has analgesic or anti-hyperalgesic effects on visceral inflammatory pain-like behaviors induced by i.p. injection of irritants (Lariviere & Melzack Citation2000). Supraspinally administered CRF also produces a dose-dependent antinociception in rodent thermal and inflammatory pain models (Vit et al. Citation2006; Lariviere etal. Citation2011). In contrast, CRH consistently has hyperalgesic effects in irritable bowel syndrome models of visceral hypersensitivity (Taché et al. Citation2005). It has been reported that activation of CRHR1 receptor in the amygdala contributes to pain-related synaptic facilitation, increased excitability, and pain behavior (Fu & Neugebauer Citation2008). Bourbia et al. (Citation2010) have demonstrated that microinjection with CRH into central nucleus of the amygdale produces an enhancement of mechanical hypersensitivity in spared nerve ligation model. Although the activation of CRH receptors could induce both analgesia and hyperalgesia, the exact role of CRHR1 in the regulation of nociception has not been well characterized. Thus, in the present study, we investigated to characterize the possible involvement of CRHR1 in the regulation of nociception in both normal and CRHR1 heterozygous (CRHR1+/−) mice.
Materials and methods
These experiments were approved by the Hallym University Animal Care and Use Committee (Registration Number: Hallym 2013-72). All procedures were conducted in accordance with the ‘Guide for Care and Use of Laboratory Animals’ published by the National Institutes of Health and the ethical guidelines of the International Association for the Study of Pain.
Experimental animals
CRHR1-deficient (B6;129-Crhr1tm1Klee/J; CRHR1–/−) and wild type (WT; CRHR1+/+) mice were kindly provided by Dr. P. L. Han at Ewha Womans University. CRHR1 heterozygous knockout mice (CRHR1+/−) were generated by mating male CRHR1+/− mice with female CRHR1+/− mice. The genotypes of mice were examined by polymerase chain reaction (PCR) analysis after extraction of genomic DNA from the tail according to the instruction of the Jackson Laboratory. PCR products 200 bp, 400 bp, and 200 and 400 bp indicate WT, KO, and heterozygote mice, respectively. We note that the viability of CRHR1−/− mice on C57Bl/6 background was very poor; heterozygote crosses produced significantly fewer CRHR1−/− male mice than predicted. Thus, male heterozygote mice (CRHR1+/−) were used in this study. Nociceptive tests were performed at 6 weeks of age. The mice were housed five per cage in a room maintained at 22 ± 1°C with an alternating 12-hour light-dark cycles. Food and water were available ad libitum. The animals were allowed to adapt to the laboratory for at least 2 hr before testing and were only used once. To reduce variation, all experiments were performed during the light phase of the cycle (10:00–17:00).
Nociceptive models and pain behavior measurements
For the writhing test (Choi et al. Citation2003), 1% acetic acid was injected i.p. and then the animals were immediately placed in an acrylic observation chamber (20 cm high, 20 cm diameter). The number of writhes was counted during 30 min after the injection of acetic acid. A writhe was defined as a contraction of the abdominal muscles accompanied by an extension of the forelimbs and elongation of the body. For the formalin test (Hunskaar et al. Citation1985; Choi et al. Citation2003), 10 µl of 5% formalin was injected subcutaneously (s.c.) under the plantar surface of the left hindpaw. Following injection of formalin, the animals were immediately placed in an acrylic observation chamber, and the time spent licking, shaking, and biting the injected paw was measured with a stop-watch timer and considered as indication of nociception. The early phase of the nociceptive response normally peaked 0–5 min, and the last phase 20–40 min after formalin injection, representing the direct effect on nociceptors and inflammatory nociceptive responses, respectively (Hunskaar & Hole Citation1987; Choi et al. Citation2001). For the substance P (0.7 µg) test, immediately after i.t. injection with substance P or glutamate, the mice were placed in an observation chamber (20 cm high, 20 cm diameter) and their nociceptive behavioral responses were recorded during 30 min. The cumulative response time of licking, scratching, and biting episodes directed toward the lumbar and caudal region of spinal cord were measured with a stop-watch timer (Hylden & Wilcox Citation1981; Seo et al. Citation2008). The i.t. administration was performed following the method of Hylden and Wilcox (Citation1981), using a 30-gauge needle connected to a 25 µl Hamilton syringe with polyethylene tubing. The i.t. injection volume was 5 µl, and the injection site was verified by injecting a similar volume of 1% methylene blue solution and determining the distribution of the injected dye in the spinal cord. The dye injected i.t. was distributed both rostrally and caudally but with short distance (about 0.5 cm from the injection site), and no dye was found visually in the brain. The success rate for the injections was consistently found to be over 95%, before the experiments were done.
Intraperitoneal (i.p.) and intracerebroventricular (i.c.v.) injections of CRHR1 antagonists
The i.p. injection with the selective CRHR1 antagonist, CP 154,526 (butyl-[2,5-dimethyl-7-(2,4,6-trimethylphenyl)-7H-pyrrolo[2,3-d]pyrimidin-4-yl]-ethylamine) (Pfizer, Groton, CT) was conducted in conscious mice with a volume of 10 µl/g of body weight, using a 30-gauge needle connected to a 1 ml syringe. The i.c.v. administration with α-helical CRF9–41 (nonselective CRH receptor antagonist; Sigma-Aldrich, Saint Louis, MO) was followed by the method described previously (Choi et al. Citation2002). In brief, each mouse was grasped firmly without anesthesia by the loose skin behind the head. The skin was pulled taut. A 30-guage needle attached to a 25 µl syringe was inserted perpendicularly through the skull into the brain and solution was injected. The injection site was 2 mm from either side of the midline on a line drawn through the anterior base of the ears. The i.c.v. injection volumes were 5 µl, and the injection sites were verified by injecting a similar volume of 1% methylene blue solution and determining the distribution of the injected dye in the ventricular space. The success rate for prior injections with this technique was over 95%.
CP 154,526 and CRF9–41 were dissolved in sterile water and suspended in 0.5% carboxymethylcellulose (Sigma-Aldrich), respectively. CP 154526 (10 mg/kg, i.p.) or α-helical CRF 9-41 (10 µg, i.c.v.) pretreatment time and dose were based on a previous study (Lowery et al. Citation2010).
Isolation of total RNA
The entire process for isolation of total RNA was conducted three times independently. Finally, the animal number used for each group was nine. Three animals of each group were dissected for real-time PCR analysis. Total cellular RNA was extracted from dissected hippocampus tissue using a rapid guanidine thiocyanate-water saturated phenol/chloroform extraction procedure and subsequent precipitation with acidic sodium acetate (Chomczynski & Sacchi Citation1987). Total cellular RNA in the aqueous phase was precipitated with ice-cold isopropyl alcohol. Isolated RNA samples were subjected to spectrophotometric analysis at 260 nm and 280 nm. The separated organic layer was extracted twice with an equal volume of sterilized (Millipore, USA) water and proteins were precipitated by adding two volumes of absolute ethanol to the water-extracted organic phase. The dried pellets were dissolved in a denaturing buffer (6 M guanidium chloride, 20 mM Tris-HCl [pH 8.0], and 1 mM EDTA).
Real-time PCR analysis
Expression of CRH and β-actin mRNA was evaluated by real-time PCR using QuantiTectTM SYBR® Green PCR Kit (Qiagen, Germany). All PCRs were performed in total volume of 20 μl using the QuantiTectTM SYBR® Green PCR Kit (Qiagen, Germany). Each reaction contained 1.5 μl of cDNA, 6.5 μl RNase-Free Water, each 1 μl of sense and antisense primer (20 μM), and 10 μl of 2X SYBR® Green PCR Master Mix (containing QuantiTect SYBR Green PCR buffer, dNTPs, SYBR Green I dye, ROX dye, and HotStarTaq DNA polymerase). After an initial denaturation step at 95°C for 30 s, temperature cycling with a total of 40 cycles was initiated. Each cycle consisted of a denaturation phase at 95°C for 30 s, an annealing phase at 60°C for 30 s, and an elongation phase at 72°C for 30 s. Amplification was followed by melting curve analysis to verify the correctness of the amplification. A negative control with water instead of cDNA was run within every PCR to assess specificity of the reaction. To verify the accuracy of the amplification, PCR products were further analyzed on ethidium bromide-stained 2% agarose gel. For data analysis, Rotor-Gene 6000 Series Software 1.7 (Build 87) was used. Results are given as a ratio of the amount of CRH mRNA to that of β-actin mRNA. The following primers were used: CRH (NM_205769) sense: TTG AAT TTC TTG CAG CCG GAG CAG, antisense: AGC AGC GGG ACT TCT GTT GAG ATT and β-actin (NM_007393) sense: AGA GGG AAA TCG TGC GTG AC, antisense: CAA TAG TGA CCT GGC CGT. The entire process from dissection to RT-PCR was conducted three times independently. Finally, the animal number used for each group was three.
Statistics
Statistical analysis carried out by student t-test GraphPad Prism version 4.0 for Windows (GraphPad Software, San Diego, CA, USA). P-values less than 0.05 were considered to indicate statistical significance. All values were expressed as the mean ± S.E.M.
Results
Changes of CRH mRNA expression in the hypothalamus and hippocampus by various types of pain stimuli
C57BL6 mice were injected with 1% acetic acid (i.p.), 5% formalin into the hindpaw (s.c.), or substance P (0.7 μg, i.t.). CRH mRNA levels in the hypothalamus and hippocampus were measured 0.5, 1, and 2 hr after each drug administration. As shown in and , i.p. administration of 1% acetic acid, intraplantar injection of 5% formalin, and i.t. injection of substance P caused an elevation of CRH mRNA expression in both hypothalamus and hippocampus.
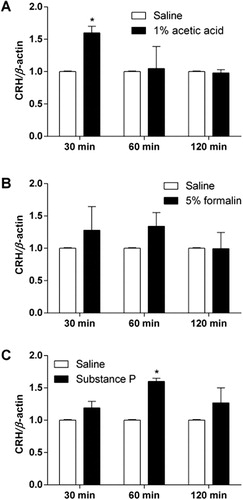
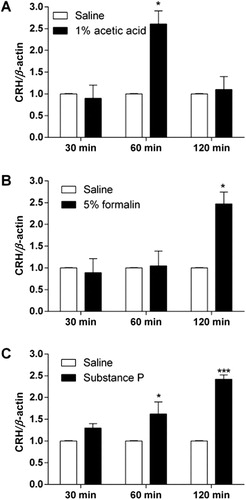
The nociceptive behavioral changes in CRHR1+/− mouse
We have examined the nociceptive behaviors in CRHR1+/− mouse (). Either WT or CRHR1+/− mouse was treated with 1% acetic acid (i.p.), 5% formalin into the hindpaw (s.c.), or substance P (0.7 μg, i.t.). The number of acetic acid-induced writhing response was significantly smaller in CRHR1+/− mouse group compared to that in WT mouse group as revealed in . In the formalin test, nociceptive behaviors observed during the second, but not the first, phase were profoundly attenuated in CRHR1+/− mouse group compared to WT mouse group (). Furthermore, i.t. substance P-induced nociceptive behaviors were greatly reduced in CRHR1+/− mouse group compared to WT mouse group as shown in .
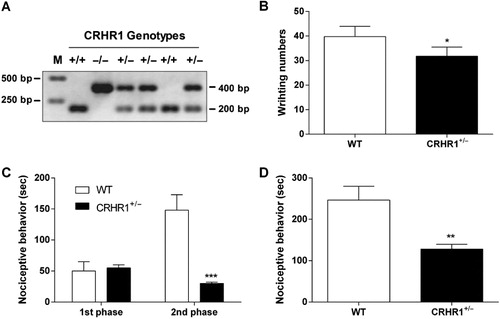
Effect of CRHR1 antagonists administered on nociceptive behaviors in the writhing and substance P pain models
The C57BL6 mouse was pretreated with CP 154526 (10 mg/kg, i.p.) or α-helical CRF 9-41 (10 µg, i.c.v.). After 30 min, substance P (0.7 µg, i.t.) or 1% acetic acid (i.p.) was administered. Nociceptive behaviors induced by substance P or acetic acid administration were observed for 30 min. Pretreatment with CP 154526 (i.p.) or α-helical CRF 9-41 (i.c.v.) attenuated i.t.-administered substance P- or i.p.-administered acetic acid-induced nociceptive behaviors (). Among the antagonists tested, i.c.v. injection of α-helical CRF 9-41 showed more profound inhibitory action in nociceptive behaviors induced by acetic acid or substance P rather than CP 154526 (i.p.).
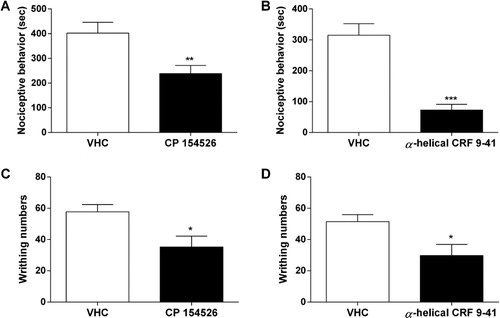
Discussion
The activation of CRHR1 in the hypothalamus is primarily responsible for the regulation of Adrenocorticotropic hormone secretion from the pituitary and a variety of behavioral and autonomic response changes to stress (Timpl et al. Citation1998; Aguilera et al. Citation2004). In addition, the activation of CHRH1 in the hippocampus appears to be responsible for the synaptic formation (Liao et al. Citation2014), memory (Schierloh et al. Citation2007; Thoeringer et al. Citation2011), and behavioral adaption to stress (Refojo et al. Citation2011). However, the role of CRH system in the hypothalamus and the hippocampus has not been well characterized. We observed in the present study that an elevation of CRH mRNA level in the hypothalamus and the hippocampus was observed by various types of nociceptive stimuli such as i.p. administration of acetic acid, intraplantar injection of formalin, and i.t. injection of substance P in C57BL6 mice. Some previous studies have demonstrated that the hypothalamus and the hippocampus play important roles in the regulation of nociception (Duric & McCarson Citation2007; Nishii et al. Citation2007; Kwon et al. Citation2008; del Rey et al. Citation2011). Our findings are in line with those found by others. For example, Nishii et al (Citation2007) have reported that visceral pain stimulation increases hypothalamic CRH mRNA level. Taken together, it suggests that the activation of peripheral or central nociceptive system may activate hypothalamic or hippocampal CRH system.
Numerous studies have previously demonstrated that activation of CRH receptors at supraspinal level produces antinociception (Sherman & Kalin Citation1986; Miguel & Nunes-de-Souza Citation2011). However, Ayesta and Nikolarakis (Citation1989) have previously reported that central administration of CRH does not produce antinociception in the tail-flick test. In addition, hyperalgesic effects, pain-related synaptic facilitation, increased excitability, and increased pain behavior were reported in the several animal pain models (Taché et al. Citation2005; Fu & Neugebauer Citation2008). For example, Bourbia et al. (Citation2010) have demonstrated that microinjection with CRH into central nucleus of the amygdale produces an enhancement of mechanical hypersensitivity in spared nerve ligation model. To examine the role of CRHR1 in the regulation of nociception, we used CRHR1+/− mouse model. Although we did not examine the CRHR1 KO mouse model, we found in the present study that nociceptive behaviors in CRHR1+/− mice are reduced compared to wild-type mouse in all nociceptive tests examined (i.e. writhing, formalin, and substance P tests). There are some limitations to the use of CRHR1+/− mice rather than the use of CRHR1 KO mouse. However, the results of the present study suggest that the CRHR1+/− mouse model can be used for delineating the functional roles of CRHR1. In an attempt to support the role of CRHR1-induced nociceptive activation, we examined the effects of CRHR1 antagonists administered systemically or supraspinally on nociceptive behaviors induced by several types of pain modalities in WT mice. We also demonstrated that administration of CRHR1 antagonists such as CP 154526 (i.p.) and α-helical CRF 9-41 (i.c.v.) reduce nociceptive behaviors induced by acetic acid or substance P administration. Furthermore, we observed that i.c.v. injection of CRHR1 showed more profound inhibition in nociceptive behaviors induced by substance P rather than CP 154526 (i.p.). Interestingly, it has been proposed that CRH has both pro-nociceptive and antinociceptive effects on prolonged inflammatory pain processing in the amygdala (Neugebauer et al. Citation2004; Ji & Neugebauer Citation2008). It was based on the findings that low doses of CRH increased evoked responses in the amygdala via CRHR1 receptors, whereas high doses decreased evoked responses via CRHR2 receptors (Neugebauer et al. Citation2004; Ji & Neugebauer Citation2008). Although the involvement of CRHR2 in the regulation of nociception was not investigated in this study, our findings support that activation of CRHR1 may be responsible for nociceptive producing rather than antinociception.
In conclusion, we demonstrated in the present study that CRH mRNA level in the hypothalamus and the hippocampus is elevated by various types of nociceptive stimuli. However, nociceptive behaviors in CRHR1+/− mice are decreased in various types of nociceptive stimuli. We also demonstrated that administration of CRHR1 antagonists reduce nociceptive behaviors. Our results suggest that CRHR1 may play an important role in the production of nociception.
Acknowledgment
This research was supported by Priority Research Centers [grant numbers NRF-2009-0094071 and NRF-2014R1A1A1006791] through the National Research Foundation of Korea (NRF) funded by the Ministry of Education, Science and Technology and Hallym University Research Fund, 2014 [grant number HRF-201403-014].
References
- Aguilera G, Nikodemova M, Wynn PC, Catt KJ. 2004. Corticotropin releasing hormone receptors: two decades later. Peptides. 25:319–329.10.1016/j.peptides.2004.02.002
- Ayesta FJ, Nikolarakis KE. 1989. Peripheral but not intracerebroventricular corticotropin-releasing hormone (CRH) produces antinociception which is not opioid mediated. Brain Res. 503:219–224.10.1016/0006-8993(89)91667-3
- Bale TL. 2005. Sensitivity to stress: dysregulation of CRF pathways and disease development. Horm Behav. 48:1–10.10.1016/j.yhbeh.2005.01.009
- Bourbia N, Ansah OB, Pertovaara A. 2010. Corticotropin-releasing factor in the rat amygdala differentially influences sensory-discriminative and emotional-like pain response in peripheral neuropathy. J Pain. 11:1461–1471.10.1016/j.jpain.2010.05.004
- Chen RP, Lewis KA, Perrin MH, Vale WW. 1993. Expression cloning of a human corticotropin-releasing factor receptor. Proc Natl Acad Sci USA. 90:8967–8971.10.1073/pnas.90.19.8967
- Choi SS, Han KJ, Lee JK, Lee HK, Han EJ, Kim DH, Suh HW. 2003. Antinociceptive mechanisms of orally administered decursinol in the mouse. Life Sci. 73:471–485.10.1016/S0024-3205(03)00311-4
- Choi SS, Han EJ, Lee TH, Lee JK, Han KJ, Lee HK, Suh HW. 2002. Antinociceptive mechanisms of platycodin D administered intracerebroventricularly in the mouse. Planta Med. 68:794–798.10.1055/s-2002-34396
- Choi SS, Lee JK, Suh HW. 2001. Antinociceptive profiles of aspirin and acetaminophen in formalin, substance P and glutamate pain models. Brain Res. 921:233–239.10.1016/S0006-8993(01)03126-2
- Chomczynski P, Sacchi N. 1987. Single-step method of RNA isolation by acid guanidinium thiocyanate-phenol-chloroform extraction. Anal Biochem. 162:156–159. 10.1016/0003-2697(87)90021-2
- Chrousos GP, Gold PW. 1992. The concepts of stress and stress system disorders overview of physical and behavioral homeostasis. JAMA. 267:1244–1252.10.1001/jama.1992.03480090092034
- Dautzenberg FM, Hauger RL. 2002. The CRF peptide family and their receptors: yet more partners discovered. Trends Pharmacol Sci. 23:71–77.10.1016/S0165-6147(02)01946-6
- del Rey A, Yau HJ, Randolf A, Centeno MV, Wildmann J, Martina M, Besedovsky HO, Apkarian AV. 2011. Chronic neuropathic pain-like behavior correlates with IL-1β expression and disrupts cytokine interactions in the hippocampus. Pain. 152:2827–2835.10.1016/j.pain.2011.09.013
- Duric V, McCarson KE. 2007. Neurokinin-1 (NK-1) receptor and brain-derived neurotrophic factor (BDNF) gene expression is differentially modulated in the rat spinal dorsal horn and hippocampus during inflammatory pain. Mol Pain. 3:32.10.1186/1744-8069-3-32
- Fu Y, Neugebauer V. 2008. Differential mechanisms of CRF1 and CRF2 receptor functions in the amygdala in pain-related synaptic facilitation and behavior. J Neurosci. 28:3861–3876.10.1523/JNEUROSCI.0227-08.2008
- Hauger RL, Grigoriadis DE, Dallman MF, Plotsky PM, Vale WW, Dautzenberg FM. 2003. International union of pharmacology. XXXVI. Current status of the nomenclature for receptors for corticotropinreleasing factor and their ligands. Pharmacol Rev. 55:21–26.10.1124/pr.55.1.3
- Hunskaar S, Fasmer OB. Hole K. 1985. Formalin test in mice, a useful technique for evaluating mild analgesics. J Neurosci Methods. 14:69–76.10.1016/0165-0270(85)90116-5
- Hunskaar S, Hole K. 1987. The formalin test in mice: dissociation between inflammatory and non-inflammatory pain. Pain. 30:103–114.10.1016/0304-3959(87)90088-1
- Hylden JL, Wilcox GL. 1981. Intrathecal substance P elicits a caudally-directed biting and scratching behavior in mice. Brain Res. 217:212–215.10.1016/0006-8993(81)90203-1
- Ji G, Neugebauer V. 2008. Pro- and anti-nociceptive effects of corticotropin-releasing factor (CRF) in central amygdala neurons are mediated through different receptors. J Neurophysiol. 99:1201–1212.10.1152/jn.01148.2007
- Kwon MS, Seo YJ, Shim EJ, Lee JK, Jang JE, Park SH, Jung JS, Suh HW. 2008. The differential effects of emotional or physical stress on pain behaviors or on c-Fos immunoreactivity in paraventricular nucleus or arcuate nucleus. Brain Res. 1190:122–131.10.1016/j.brainres.2007.11.009
- Lariviere WR, Fiorenzani P, Ceccarelli I, Massafra C, Sorda G, Di Canio C, Aloisi AM. 2011. Central CRH administration changes formalin pain responses in male and female rats. Brain Res. 1383:128–134.10.1016/j.brainres.2011.01.106
- Lariviere WR, Melzack R. 2000. The role of corticotropin-releasing factor in pain and analgesia. Pain. 84:1–12.10.1016/S0304-3959(99)00193-1
- Liao XM, Yang XD, Jia J, Li JT, Xie XM, Su YA, Schmidt MV, Si TM, Wang XD. 2014. Blockade of corticotropin-releasing hormone receptor 1 attenuates early-life stress-induced synaptic abnormalities in the neonatal hippocampus. Hippocampus. 24:528–540.10.1002/hipo.22254
- Lowery EG, Spanos M, Navarro M, Lyons AM, Hodge CW, Thiele TE. 2010. CRF-1 antagonist and CRF-2 agonist decrease binge-like ethanol drinking in C57BL/6J mice independent of the HPA axis. Neuropsychopharmacol. 35:1241–1252.10.1038/npp.2009.209
- Miguel TT, Nunes-de-Souza RL. 2011. Anxiogenic and antinociceptive effects induced by corticotropin-releasing factor (CRF) injections into the periaqueductal gray are modulated by CRF1 receptor in mice. Horm Behav. 60:292–300.10.1016/j.yhbeh.2011.06.004
- Neugebauer V, Li W, Bird GC, Han JS. 2004. The amygdala and persistent pain. Neuroscientist. 10:221–234.10.1177/1073858403261077
- Nishii H, Nomura M, Aono H, Fujimoto N, Matsumoto T. 2007. Up-regulation of galanin and corticotropin-releasing hormone mRNAs in the key hypothalamic and amygdaloid nuclei in a mouse model of visceral pain. Regul Pept. 141:105–112.10.1016/j.regpep.2006.12.022
- Refojo D, Schweizer M, Kuehne C, Ehrenberg S, Thoeringer C, Vogl AM, Dedic N, Schumacher M, von Wolff G, Avrabos C, et al. 2011. Glutamatergic and dopaminergic neurons mediate anxiogenic and anxiolytic effects of CRHR1. Science. 333:1903–1907.10.1126/science.1202107
- Schierloh A, Deussing J, Wurst W, Zieglgänsberger W, Rammes G. 2007. Corticotropin-releasing factor (CRF) receptor type 1-dependent modulation of synaptic plasticity. Neurosci Lett. 416:82–86.10.1016/j.neulet.2007.01.047
- Seo YJ, Kwon MS, Choi SS, Han EJ, Jung JS, Choi HW, Park SH, Jang JE, Suh HW. 2008. Characterization of the hypothalamic proopiomelanocortin gene and beta-endorphin expression in the hypothalamic arcuate nucleus of mice elicited by inflammatory pain. Neuroscience. 152:1054–1066.10.1016/j.neuroscience.2007.06.047
- Sherman JE, Kalin NH. 1986. ICV-CRH potently affects behavior without altering antinociceptive responding. Life Sci. 39:433–441.10.1016/0024-3205(86)90523-0
- Taché Y, Million M, Nelson AG, Lamy C, Wang L. 2005. Role of corticotropin-releasing factor pathways in stress-related alterations of colonic motor function and viscerosensibility in female rodents. Gend Med. 2:146–154.10.1016/S1550-8579(05)80043-9
- Thoeringer CK, Henes K, Eder M, Dahlhoff M, Wurst W, Holsboer F, Deussing JM, Moosmang S, Wotjak CT. 2011. Consolidation of remote fear memories involves Corticotropin-Releasing Hormone (CRH) receptor type 1-mediated enhancement of AMPA receptor GluR1 signaling in the dentate gyrus. Neuropsychopharmacol. 37:787–796.10.1038/npp.2011.256
- Timpl P, Spanagel R, Sillaber I, Kresse A, Reul JM, Stalla GK, Blanquet V, Steckler T, Holsboer F, Wurst W. 1998. Impaired stress response and reduced anxiety in mice lacking a functional corticotropin-releasing hormone receptor 1. Nat Genet. 19:162–166.10.1038/520
- Vit JP, Clauw DJ, Moallem T, Boudah A, Ohara PT, Jasmin L. 2006. Analgesia and hyperalgesia from CRF receptor modulation in the central nervous system of Fischer and Lewis rats. Pain. 121:241–260.10.1016/j.pain.2005.12.024