ABSTRACT
Airborne particulate matter (PM) is a major health hazard worldwide and is a key factor in lung cancer, which remains the most common type of malignancy and the leading cause of cancer-related deaths. DNA methylation is a critical mechanism underlying the detrimental effects of PM, however, the molecular link between PM exposure and lung cancer remains to be elucidated. N-α-acetyltransferase 10 (NAA10) is involved in the cell cycle, migration, apoptosis, differentiation, and proliferation. In order to investigate the role of NAA10 in PM-induced pathogenesis processes leading to lung cancer, we determined the expression and methylation of NAA10 in normal human bronchial epithelial (NHBE) cells treated with PM10, PM10-polycyclic aromatic hydrocarbons (PAH), and PM2.5 and evaluated the prognostic value of the NAA10 methylation status in lung cancer patients. Exposure to all PM types significantly increased the expression of NAA10 mRNA and decreased the methylation of the NAA10 promoter in NHBE cells compared with the mock-treated control. NAA10 hypomethylation was observed in 9.3% (13/140) of lung cancer tissue samples and correlated with NAA10 transcriptional upregulation. Univariate and multivariate analyses revealed that NAA10 hypomethylation was associated with decreased survival of patients with lung cancer. Therefore, these results suggest that PM-induced hypomethylation of the NAA10 may play an important role in the pathogenesis of lung cancer and may be used as a potential prognostic biomarker for lung cancer progression. Further studies with large numbers of patients are warranted to confirm our findings.
Introduction
Air pollution is a worldwide environmental threat as a major cause of premature death and disease (Schraufnagel et al. Citation2019; Fuller et al. Citation2022). Its drivers, its dispersion, and its effects on health transcend local boundaries and demand a global response. Particulate matter (PM), one of the major air pollutants, is a heterogeneous mixture of solid particles and liquid droplets that can be inhaled to cause serious health problems like heart and lung diseases (Samet and Krewski Citation2007; Ruckerl et al. Citation2011). Its particle size and chemical composition vary (Valavanidis et al. Citation2008). Coarse (thoracic) PM10 (particles diameter ≤10 μm) consisting of dust, pollen, and mold can penetrate the upper respiratory tract, whereas fine (respirable) PM2.5 (particles diameter ≤2.5 μm) comprised of organic carbon compounds, nitrates, and sulfates can deposit into the lower respiratory tract. Moreover, particle-borne polycyclic aromatic hydrocarbons (PAH) are known to be the main etiological factors that can cause adverse health outcomes of PM (Yang et al. Citation2021). However, there is still a fundamental lack of understanding of the toxicological mechanisms of PM and its possible triggers.
Lung cancer is the leading cause of cancer-related deaths because of its high incidence and low survival rate, despite the rapid progress in treatment methods for advanced lung cancer (Yang et al. Citation2020; Siegel et al. Citation2021). Although cigarette smoking is a well-known risk factor for lung cancer, emerging evidence suggests that outdoor air pollution, especially PM exposure, is associated with increased lung cancer risk and mortality independent of cigarette smoking (Hamra et al. Citation2014; Turner et al. Citation2020). However, the carcinogenic effects of PM in lung cancer and potential underlying mechanisms remain unclear. Accumulating data indicate that PM exposure modulates DNA methylation, a major epigenetic pathway affecting gene expression, which might contribute to the development and maintenance of inflammation and chronic conditions (Sun et al. Citation2018; Rider and Carlsten Citation2019). As aberrant DNA methylation is also an important epigenetic regulator of lung tumorigenesis (Hoang and Landi Citation2022), analysis of DNA methylation patterns should provide a foundation for improving the prevention, diagnosis, and treatment strategies for lung cancer.
N-α-acetyltransferase A (NatA) catalyzes N-terminal acetylation of eukaryotic proteins at both N-terminal amino acid and internal lysine residues and is, therefore, involved in the regulation of protein stability, function, and targeting to specific cellular compartments as well as in protein–protein interaction (Dorfel and Lyon Citation2015). NatA consists of the catalytic subunit NAA10 and auxiliary subunit NAA15, and accumulating evidence demonstrates that the NAA10 is strongly overexpressed in various types of cancer, including lung cancer, suggesting its involvement in cancer progression (Kim et al. Citation2020). However, little is known about the correlation of these upregulation with NAA10 methylation and the effect of PM on NAA10 expression, which contributes to the pathological consequences of lung cancer. In the present study, we determined the methylation status of the NAA10 promoter in normal human bronchial epithelial (NHBE) cells and normal human epidermal keratinocytes (NHEKs) exposed to PM (PM2.5, PM10, and PM10-PAH) and in surgically resected tissues of non-small cell lung cancer (NSCLC) patients, and analyzed the correlation between NAA10 methylation and clinicopathological characteristics.
Materials and methods
Cell culture, PM preparation and treatment
NHBE (ATCC PCS-300-011) and NHEK (ATCC PCS-200-011) cells were obtained from American Type Culture Collection (ATCC, Manassas, VA, USA) and cultured as described previously (Lee et al. Citation2022). NSCLC cells A549 (CCL-185) and H2009 (CRL-5911) were also purchased from ATCC and maintained in RPMI-1640 medium (Thermo Fisher Scientific Inc, Waltham, MA, USA) supplemented with 10% heat-inactivated fetal bovine serum. PM10-PAH (ERM-CZ100), PM10 (ERM-CZ120), and PM2.5 (SRM1650b) were purchased from Sigma-Aldrich (St. Louis, MO, USA) and prepared as described previously (Lee et al. Citation2022). NHBE and NHEK cells were grown to 50% confluency and treated with PM2.5, PM10, and PM10-PAH at the final concentration of 50μg/ml for three days without medium change. Cells maintained in culture media with vehicle (0.1% DMSO or 1% PBS) were used as untreated control groups. A549 and H2009 cells were treated with 20 μM 5-AzadC for three days with daily change of culture medium.
NSCLC patients and tissues sample
Tumor and corresponding non-malignant lung tissue specimens (n = 140) were provided by the National Biobank of Korea – Kyungpook National University Hospital (KNUH), which is supported by the Ministry of Health, Welfare, and Family Affairs. All materials from the National Biobank of Korea – KNUH were obtained after approval of the institutional review board (approval no. 2014-04-210). The clinicopathological characteristics of the patients are summarized in . Histological sections of NSCLC tissues were manually microdissected to obtain 80% neoplastic cellularity.
Table 1. Correlation between NAA10 hypomethylation and clinicopathological characteristics of NSCLC patients.
RNA isolation and reverse transcription (RT)-PCR
Total RNA was extracted from cultured cells and tissue specimens, using TRIzol reagent (Invitrogen, Waltham, MA, USA). After removing residual DNA, first-strand cDNA was synthesized from total RNA, using SuperScript preamplification kit (Invitrogen) according to the manufacturer’s instructions. The resulting cDNA was amplified with NAA10- and NAA15-specific primers. All primer sequences were described in Supplementary Table 1. Amplified products were separated on 2% agarose gel, stained with ethidium bromide, visualized under UV light, and photographed by Syngene DigiGenius gel documentation system (Syngene, Frederick, MD, USA). Band intensity values were semi-quantitatively analyzed with ImageJ 1.53t program (National Institute of Health, Bethesda, MD, USA) and the relative amount of NAA10 mRNA, normalized to glyceraldehyde 3-phosphate dehydrogenase (GAPDH), was expressed as gray values.
Genomic DNA isolation and pyrosequencing
Genomic DNA was extracted from cultured cells and primary tumor tissues by the QIAamp DNA Mini kit (Qiagen, Valencia, CA, USA) and subjected to bisulfite treatment with the EZ DNA Methylation-Gold kit (Zymo Research, Orange, CA, USA) according to the manufacturer’s instruction. Bisulfite-modified DNA was amplified with PCR primers, and the purified products were assayed on the PyroMark Q96MD System (Qiagen), as previously described (Kim et al. Citation2018). The bisulfite PCR primer and sequencing primer sequences are shown in Supplementary Table 1. The degree of methylation was expressed for each DNA locus as the percentage of methylated cytosines among all cytosine residues (methylated and unmethylated), and the methylation level was calculated from the mean methylation percentage of the six CpG sites evaluated. Non-CpG cytosine residues were used as built-in controls to verify bisulfite conversion. Three controls were included for each pyrosequencing run. One well was filled with water to ensure no contamination, and two wells were filled with CpGenome universal methylated and unmethylated DNA (Chemicon, Temecula, CA, USA) as positive and negative controls, respectively, to assess the repeatability of the assay.
The Cancer Genome Atlas (TCGA) data collection
We collected clinical information and gene expression profiles in TCGA databases (updated to April 7, 2020) which included 540 lung adenocarcinoma (ADC), 503 squamous cell carcinoma (SQC) tissues, and 59 and 51 corresponding normal tissues, respectively. Additionally, a box plot was drawn to show discrete variable distribution visually.
Statistical analysis
Methylation levels of NHEK and NHBE cells were presented as the means ± standard error of three independent experiments. The independent t-test was used to compare the means between two groups. One way ANOVA was used for analyzing the mean difference test between three or more groups, and the Dunnett’s method was used for post-hoc comparison when ANOVA indicates statistical significance. The relationship between the methylation status and clinicopathological characteristics was analyzed using the chi-square test for categorical variables. The survival probability of NSCLC patients according to the NAA10 methylation status was calculated using the Kaplan-Meier product limit estimator and log-rank test. Hazard ratios (HRs) and 95% confidence intervals (CIs) were estimated using a multiple Cox proportional hazard model. The significance level (P) for all statistical tests was set at 5%. Statistical analyses were conducted using SAS 9.4 (SAS Inc., Cary, NC, USA), and plots were constructed using R version 4.1.1 (The R Foundation for Statistical Computing, Vienna, Austria).
Results
Upregulation of NAA10 and promoter hypomethylation in PM-exposed NHBE cells
RT–PCR analysis of NAA10 and NAA15 transcription in PM-exposed cells showed that NAA10 mRNA expression was increased in NHBE cells, but not in NHEKs after treatments with all PM types, whereas NAA15 mRNA expression level was not affected (). The NAA10 methylation status was assessed by pyrosequencing, which revealed that the average methylation level was significantly decreased in NHBE cells after exposure to PM10-PAH, PM10, and PM2.5, but was unchanged in NHEKs ().
Figure 1. Expression of NAA10 mRNA in NHBE cells and NHEKs exposed to PM. Cells were treated with PM10-PAH (lanes 2 and 6), PM10 (lanes 3 and 7), and PM2.5 (lanes 4 and 8) at the final concentration of 50-μg/ml for 3 days. Cells exposed to vehicle (1% PBS and 0.1% DMSO) were used as the untreated control group (lanes 1 and 5, respectively). Expression of NAA10 and NAA15 mRNAs was analyzed by semi-quantitative RT-PCR and electrophoresis using 2% agarose gel, where bands appeared at positions corresponding to the expected lengths. GAPDH expression was used as an internal loading control. Band intensity values were semi-quantitatively analyzed with ImageJ 1.53t program and the relative amount of NAA10 and NAA15 mRNAs, normalized to housekeeping gene GAPDH levels, were expressed as gray values. SM, 100-bp ladder.
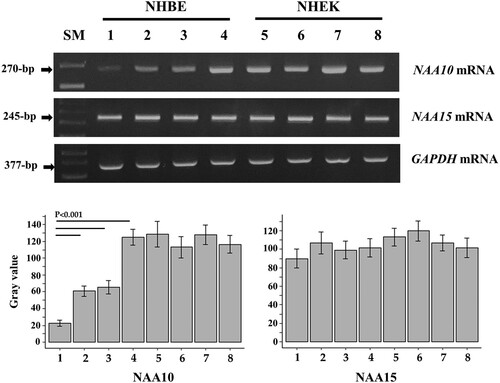
Figure 2. NAA10 methylation in PM-exposed NHBE cells (A) and NHEKs (B). The methylation status was expressed as the percentage of 5-methylcytosine among all (methylated and unmethylated) cytosine residues. Mean methylation levels were compared in PM-exposed and control cells treated with vehicle (CTLa, 1% PBS; CTLb, 0.1% DMSO) using ANOVA.
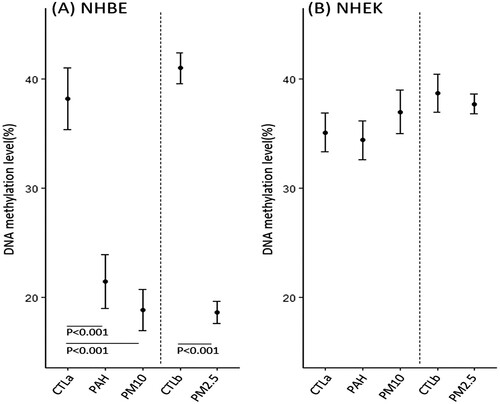
NAA10 methylation status and expression in NSCLC samples
We analyzed the NAA10 methylation status in 140 primary NSCLC samples and corresponding non-malignant lung tissue controls using pyrosequencing. The pyrosequencing primers were designed to encompass six CpGs from 638 to 708-bp upstream of the transcription start site (NG031987). Reproducible estimates of the methylated cytosine content were obtained for all tested specimens; representative pyrograms are shown in . The results indicated that all cytosine residues at non-CpG sites were converted to thymines, ruling out the possibility of incomplete bisulfite conversion. More than two-fold reduction in NAA10 methylation of NSCLC tissues compared to non-malignant tissues was considered as ‘hypomethylation’. NAA10 was hypomethylated in the tumor tissue compared with matching normal tissue (251N 66.8% vs 251 T 17.5%), whereas obvious hypomethylation was undetectable in other lung tissues (200N 43.7% vs 200 T 31.8%) (A-D). NAA10 hypomethylation was detected exclusively in malignant tissues, at a frequency of 9.3% (13/140), suggesting that the hypomethylation of NAA10 promoter may be a tumor-associated event during NSCLC tumorigenesis.
Figure 3. Representative result of pyrosequencing and RT-PCR for the NAA10 gene in NSCLC patients. (A–D) The methylation status of the NAA10 promoter in tumors and normal tissues of NSCLC patients was analyzed by pyrosequencing. The letters on the X-axis represent the dispensation order: E, enzyme; S, substrate; A, G, C, and T, nucleotides. Shaded boxes encompassing T/C pairs indicate six interrogated CpG sites. The methylation level of each site was calculated as the percentage of C incorporation. (E) NAA10 mRNAs expression in primary tissues of NSCLC patients was assessed using semi-quantitative RT-PCR. Band intensities were quantified with ImageJ 1.53t program and the relative amount of NAA10 mRNAs, normalized to GAPDH levels, were expressed as gray values. N, normal tissue; T, tumor tissue.
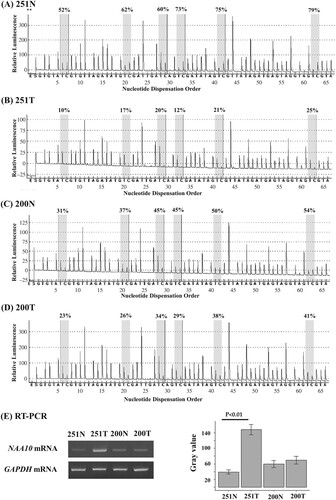
Next, we analyzed TCGA data, and found that NAA10 expression was significantly increased in tumor tissues compared to normal pulmonary tissues of lung cancer patients (Supplementary Figure 1). To determine whether CpG methylation was involved in the regulation of NAA10 expression, we analyzed the NAA10 mRNA levels and combined with methylation status in representative tissue samples. NAA10 transcript levels were increased in hypomethylated tumor tissue compared to methylated normal counterpart (251N/T), whereas no change was detected in tumor and normal lung tissues with methylated allele (200N/T) (E). We have further confirmed these results in A549 and H2009 NSCLC cell lines treated with demethylating agent 5-aza-2’-deoxycytidine (5-AzadC). 5-AzadC treatment induced re-expression of NAA10 mRNA in the A549 cells with methylated allele, but not in the H2009 cells with unmethylated allele (). Although RT–PCR analysis has been checked in limited clinical samples, due to the availability of actual samples, these results suggest that NAA10 upregulation in lung cancer might be associated with CpG hypomethylation.
Figure 4. Pyrosequencing and RT-PCR analysis of NAA10 in NSCLC cell lines. (A–D) The methylation status of the NAA10 promoter was analyzed by pyrosequencing in A549 cells (A), 5-AzadC treated A549 cells (B), H2009 (C) cell (C), and 5-AzadC treated H2009 cells (D). The letters on the X-axis represent the dispensation order: E, enzyme; S, substrate; A, G, C, and T, nucleotides. Shaded boxes encompassing T/C pairs indicate six interrogated CpG sites. The methylation level of each site was calculated as the percentage of C incorporation. (E) NAA10 mRNAs expression in the same cell lines, as determined by semi-quantitative RT-PCR. Band intensities were measured with ImageJ 1.53t program and the relative amount of NAA10 mRNAs, normalized to GAPDH levels, were expressed as gray values. (−), vehicle alone; (+), 20 μM 5-AzadC for 3 days treatment.
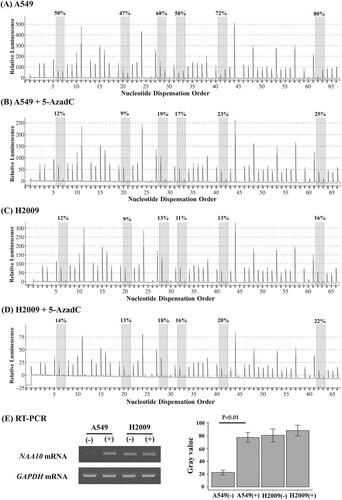
Association of NAA10 hypomethylation with unfavorable prognosis of NSCLC patients
NAA10 hypomethylation was more frequent in SQC than in ADC with a borderline significance (P = 0.06) (). However, no significant correlation was observed between the methylation status and other patient characteristics, including age, sex, smoking status, and pathological stage (). The prognostic potential of NAA10 hypomethylation was determined by Kaplan-Meier survival analyses, which revealed that patients with hypomethylated NAA10 had reduced overall survival (OS) and disease-free survival (DFS) rates compared to those with methylated NAA10 (log-rank PLR = 0.01 and 0.05, respectively) ( and ). Furthermore, Cox proportional hazards regression models adjusted for possible confounders of survival showed that NAA10 hypomethylation was significantly associated with worse OS of NSCLC patients (adjusted HR = 1.93, 95% CI = 0.97–3.89, P = 0.05] ().
Figure 5. Association of NAA10 hypomethylation with unfavorable prognosis of NSCLC patients. Kaplan-Meier plots of overall survival (A) and disease-free survival (B) according to the NAA10 methylation status in NSCLC tissues.
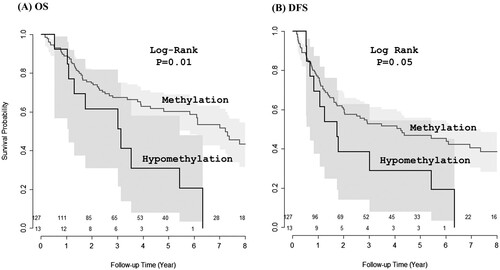
Table 2. Overall survival (OS) and disease-free survival (DFS) according to NAA10 hypomethylation in NSCLC patients.
Discussion
Despite accumulating epidemiological data indicating that air pollution-derived PM exposure is linked to epigenetic alterations (Rider and Carlsten Citation2019), a detailed mechanistic explanation of the adverse health effects exerted by PM is lacking. The use of in vitro systems such as primary bronchial epithelial cells to model human exposure to PM in vivo should help in elucidating PM-induced molecular mechanisms and provide support for clinical and epidemiological observations (Hebelbach et al. Citation2017; Shi et al. Citation2019; Wang et al. Citation2020; Cambronero-Urena et al. Citation2021). On their way to alveoli, ambient PM can trigger pathological events leading to respiratory system diseases, including lung cancer (Falcon-Rodriguez et al. Citation2016). Bronchial epithelium, which is the first line of defense against inhaled pollutants, plays a key role in initiating and orchestrating inflammatory responses by recognizing pathogenic patterns and secreting inflammatory mediators (Holtzman et al. Citation2014).
The main finding of this study was that the exposure of NHBE cells to all types of PM (PM10-PAH, PM10, and PM2.5) significantly upregulated NAA10 expression and reduced its promoter methylation. This finding suggests that NAA10 hypomethylation might be a critical mechanism underlying the harmful effects of airborne PM and that monitoring NAA10 methylation status could serve as a sensor of PM-induced DNA methylation. Recently, we demonstrated that PM exposure promoted hypomethylation of Alu and LINE1 repetitive elements in NHBE cells (Lee et al. Citation2022). Although the molecular mechanisms contributing to the NAA10 promoter hypomethylation remain elusive, there is in vitro evidence that oxidative DNA damage by PM2.5 downregulates the expression of DNA methyltransferase (DNMT) in BEAS-2B cells, resulting in DNA hypomethylation (Leclercq et al. Citation2017). In bronchial epithelial cells exposed to PM2.5, hypomethylated DNA sequences are prone to transcriptional activation (Huang et al. Citation2021), which is consistent with our results. Although many differentially hypomethylated CpG sites have been identified in PM2.5-treated BEAS-2B cells (Hebelbach et al. Citation2017; Shi et al. Citation2019; Wang et al. Citation2020), the methylation status after PM10-PAH and PM10 exposure has not been studied. Thus, our observations provide novel experimental evidence that NAA10 hypomethylation may be a common target of PM10-PAH, PM10, and PM2.5 exposure.
The second novel finding of the present study was the significant association of NAA10 hypomethylation with reduced survival of NSCLC patients, which suggests that the NAA10 methylation status could be a candidate prognostic factor in NSCLC. Analysis of TCGA data showed that NAA10 expression was significantly increased in lung tumors compared to normal tissues. Because NAA10 is ubiquitously expressed in almost all tissues and has broad substrate specificity, it is involved in many cellular processes, including cell proliferation, migration, differentiation, apoptosis, and autophagy (Dorfel and Lyon Citation2015). NAA10 is also implicated in the susceptibility to or protection against oxidative stress, which is one of the major factors linked to the adverse effects of PM on the pulmonary system (Li et al. Citation2018; Vo et al. Citation2018), suggesting a potential mechanism underlying PM toxicity in the lungs. Notably, NAA10 directly interacts with DNMT1 in H1299 lung cancer cells, resulting in silencing of the tumor suppressor gene coding for E-cadherin (Lee et al. Citation2010), and it would be interesting to determine the functional association between NAA10 hypomethylation and E-cadherin expression. Moreover, accumulating evidence indicates that NAA10 is overexpressed in various types of malignancies, including liver, bone, lung, breast, colon, and prostate cancers (Kim et al. Citation2020); however, analysis of the methylation status in these cancers remains a challenge.
Lung and skin are two of the mainly involved systems due to the primary route of exposure for ambient PM. Recently, we have demonstrated that PM treatment could induce global DNA hypomethylation in NHBE as well as NHEK cells (Lee et al. Citation2022). However, the current study showed NAA10 hypomethylation in NHBE, but not in NHEK cells following PM exposure. Similarly, PM2.5 treatment induced a p16INK4a gene promoter hypermethylation in NHBE (Leclercq et al. Citation2017), whereas PM2.5-treated NHEK cells showed lower methylation of p16INK4a promoter region (Ryu et al. Citation2019). Moreover, a recent finding has demonstrated that read-level CpG methylation patterns in tissue whole genome bisulfite sequencing libraries associate with cell type (Scott et al. Citation2020). Although the reason why NHBE and NHEK react to PM treatment differently is unclear, it is likely that the susceptibility to gene-specific methylation change may follow cell type-specific manners which associate with cell type-specific biological processes. The molecular mechanisms underlying the cell-type variability of NAA10 hypomethylation should be further explored.
It is noteworthy that NAA10 hypomethylation was more prominent in SQC. Despite significant advances in knowledge surrounding associations of PM in outdoor air and lung cancer, a mechanistic basis for a link of air pollution to particular histological types is uncertain though some studies have suggested more substantial findings with SQC (Raaschou-Nielsen et al. Citation2010; Lamichhane et al. Citation2017; Consonni et al. Citation2018). SQC tends to be found in the central part of the lungs, near the main airway. Also, it is more common in men than in women, and is closely correlated with cigarette smoking, a kind of air pollutants. In this regard, SQC preference could be attributable to anatomical and pathological differences.
Although the present study could not fully characterize the role of NAA10 in NSCLC because of the small sample size and lack of information on protein expression, it is the first to demonstrate aberrant methylation of the NAA10 promoter in NSCLC. Taken together, our findings suggest that NAA10 is a potential prognostic biomarker of NSCLC. However, further large-scale studies are needed to confirm the clinical significance, including the prognosis of NAA10 hypomethylation/expression in NSCLC.
The present study showed that PM induced NAA10 hypomethylation and transcriptional upregulation in NHBE cells, and that NAA10 hypomethylation in NSCLC patients was significantly associated with unfavorable prognosis, suggesting methylation-dependent regulation of NAA10 expression as a mechanism underlying lung cancer-promoting effects of PM. Our findings provide a new direction for investigating carcinogenic biomarkers related to PM exposure and may help identify specific interventions to prevent PM-induced lung cancer., Further studies on the molecular basis of NAA10 function are needed to elucidate pathogenic mechanisms leading to NSCLC development.
Institutional review board statement
The presented study was conducted in accordance with the Declaration of Helsinki, and approved by the Ethics Committee of Kyungpook National University Hospital (2014-04-210).
Supplemental Material
Download Zip (280.8 KB)Acknowledgements
DSK designed the study and drafted the manuscript. JYP collected and curated the clinical data and samples. JYL performed the experiments and analyzed the results. SML evaluated TCGA data and WKL performed statistical analyses.
Disclosure statement
No potential conflict of interest was reported by the author(s).
Data availability statement
The datasets generated during the current study are available from the corresponding author on reasonable request.
Additional information
Funding
References
- Cambronero-Urena A, Choi S, Choi S, Kim KK, Kim EM. 2021. Polyhexamethylene guanidine phosphate, chloromethylisothiazolinone, and particulate matter are dispensable for stress granule formation in human airway epithelial cells. Anim Cells Syst. 25:146–151.
- Consonni D, Carugno M, De Matteis S, Nordio F, Randi G, Bazzano M, Caporaso NE, Tucker MA, Bertazai PA, Pesatori AC, et al. 2018. Outdoor particulate matter (PM10) exposure and lung cancer risk in the EAGLE study. PLoS One. 13:e0203539.
- Dorfel MJ, Lyon GJ. 2015. The biological functions of Naa10 – From amino-terminal acetylation to human disease. Gene. 567:103–131.
- Falcon-Rodriguez CI, Osornio-Vargas AR, Sada-Ovalle I, Sequra-Medina P. 2016. Aeroparticles, composition, and lung disease. Front Immunol. 7:1–9.
- Fuller R, Landrigan PJ, Balakrishnan K, Bathan G, Bose-O’Reilly S, Brauer M, Caravanos J, Chiles T, Cohen A, Corra L, et al. 2022. Pollution and health: a progress update. Lancet Planetary Health. 6:e535–e547.
- Hamra GB, Guha N, Cohen A, Laden F, Raaschou-Nielsen O, Samet JM, Vineis P, Forastiere F, Saldiva P, Yorifuji T, et al. 2014. Outdoor particulate matter exposure and lung cancer: a systematic review and meta-analysis. Environ Health Perspect. 122:906–911.
- Hebelbach K, Kim GJ, Flemming S, Haupl T, Bonin M, Dornhof R, Gunther S, Merfort I, Humur M. 2017. Disease relevant modifications of the methylome and transcriptome by particulate matter (PM2.5) from biomass combustion. Epigenetics. 12:779–792.
- Hoang PH, Landi MT. 2022. DNA methylation in lung cancer: mechanisms and association with histological subtypes, molecular alterations, and major epidemiological factors. Cancers. 14:961–983.
- Holtzman M, Byers D, Alexander-Brett J, Wang X. 2014. The role of airway epithelial cells and innate immune cells in chronic respiratory disease. Nat Rev Immunol. 14:686–698.
- Huang SK, Tripathi P, Koneva LA, Cavalcante RG, Craig N, Scruggs AM, Sartor MA, Deng F, Chen Y. 2021. Effect of concentration and duration of particulate matter exposure on the transcriptome and DNA methylome of bronchial epithelial cells. Environ Epigenet. 7:1–18.
- Kim DS, Lee WK, Park JY. 2018. Hypermethylation of normal mucosa of esophagus-specific 1 is associated with an unfavorable prognosis in patients with non-small cell lung cancer. Oncol Lett. 16:2409–2415.
- Kim SM, Ha E, Kim J, Cho C, Shin SJ, Seo JH. 2020. NAA10 as a new prognostic marker for cancer progression. Int J Mol Sci. 21:8010–8024.
- Lamichhane DK, Kim HC, Choi CM, Shin MH, Shim YM, Leem JH, Ryu JS, Nam HS, Park SM. 2017. Lung cancer risk and residential exposure to air pollution: a Korean population-based case-control study. Yonsei Med J. 58:1111–1118.
- Leclercq B, Platel A, Antherieu S, Alleman LY, Hardy EM, Perdrix E, Grova N, Riffault V, Appenzeller BM, Happillon M, et al. 2017. Genetic and epigenetic alterations in normal and sensitive COPD-diseased human bronchial epithelial cells repeatedly exposed to air pollution-derived PM2.5. Environ Pollut. 210:163–177.
- Lee CF, Ou DS, Lee SB, Chang LH, Lin RK, Li YS, Upadhyay AK, Cheng X, Wang YC, Hsu HS, et al. 2010. Hnaa10p contributes to tumorigenesis by facilitating DNMT1-mediated tumor suppressor gene silencing. J Clin Invest. 120:2920–2930.
- Lee JY, Lee WK, Kim DS. 2022. Particulate matter-induced hypomethylation of Alu and LINE1 in normal human bronchial epithelial cells and epidermal keratinocytes. Genes Environ. 44:8–15.
- Li R, Zhou R, Zhang J. 2018. Function of PM2.5 in the pathogenesis of lung cancer and chronic airway inflammatory disease. Oncol Lett. 15:7506–7514.
- Raaschou-Nielsen O, Bak H, Sorensen M, Jensen SS, Ketzel M, Hvidberg M, Schnohr P, Tjonneland A, Overvad K, Loft S. 2010. Air pollution from traffic and risk for lung cancer in three Danish cohorts. Cancer Epidermiol Biomarkers Prev. 19:1284–1291.
- Rider CF, Carlsten C. 2019. Air pollution and DNA methylation: effects of exposure in humans. Clin Epigenetics. 11:131–145.
- Ruckerl R, Schneider A, Breitner S, Peters A. 2011. Health effects of particulate air pollution: A review of epidemiological evidence. Inhal Toxicol. 23:555–592.
- Ryu YS, Kang KA, Piao MJ, Ahn MJ, Yi JM, Bossls G, Hyun YM, Park CO, Hyun JW. 2019. Particulate matter-induced senescence of skin keratinocytes involves oxidative stress-dependent epigenetic modifications. Exp Mol Med. 51:1–14.
- Samet J, Krewski D. 2007. Health effects associated with exposure to ambient air pollution. J Toxicol Environ Health A. 70:227–242.
- Schraufnagel DE, Balmes JR, Cowl CT, De Matteis S, Jung SH, Mortimer K, Perez-Padilla R, Rice MB, Riojas-Rodriguez H, Sood A, et al. 2019. Air pollution and noncommunicable diseases: A review by the forum of international respiratory societies’ environmental committee, Part 2: air pollution and organ systems. Chest. 155:417–426.
- Scott CA, Duryea JD, MacKay H, Baker MS, Laritsky E, Gunasekara CJ, Coarfa C, Waterland RA. 2020. Identification of cell type-specific methylation signals in bulk whole genome bisulfite sequencing data. Genome Biol. 21:156–178.
- Shi Y, Zhao T, Yang X, Sun B, Li Y, Duan J, Sun Z. 2019. PM2.5-induced alteration of DNA methylation and RNA transcription are associated with inflammatory response and lung injury. Sci Total Environ. 650:908–921.
- Siegel RL, Miller KD, Fuchs HE, Jemal A. 2021. Cancer statistics, 2021. CA Cancer J Clin. 71:7–33.
- Sun B, Shi Y, Yang X, Zhao T, Duan J, Sun Z. 2018. DNA methylation: A critical epigenetic mechanism underlying the detrimental effects of airborne particulate matter. Ecotoxicol Environ Saf. 161:173–183.
- Turner MC, Andersen ZJ, Baccarelli A, Diver WR, Gapstur SM, Arden Pope C, Prada D, Samet J, Thurston G, Cohen A. 2020. Outdoor air pollution and cancer: An overview of the current evidence and public health recommendations. CA Cancer J Clin. 70:460–479.
- Valavanidis A, Fiotakis K, Vlachogianni T. 2008. Airborne particulate matter and human health: toxicological assessment and importance of size and composition of particles for oxidative damage and carcinogenic mechanisms. J Environ Sci Health C Environ Carcinog Ecotoxicol Rev. 26:339–362.
- Vo TTL, Jeong CH, Lee S, Kim KW, Ha E, Seo JH. 2018. Versatility of ARD1/NAA10-mediated protein lysine acetylation. Exp Mol Med. 50:1–13.
- Wang B, Li R, Cai Y, Li B, Qin S, Zheng K, Zeng M, Xiao F, Zhang Z, Xu X. 2020. Alteration of DNA methylation induced by in human bronchial epithelial cells. Toxicol Res. 9:552–560.
- Yang CY, Yang JCH, Yang PC. 2020. Precision management of advanced non–small cell lung cancer. Annu Rev Med. 71:117–136.
- Yang L, Zhang H, Zhang X, Xing W, Wang Y, Bai P, Zhang L, Hayakawa K, Toriba A, Tang N. 2021. Exposure to atmospheric particulate matter-bound polycyclic aromatic hydrocarbons and their health effects: a review. Int J Environ Res Public Health. 18:2177–2201.