ABSTRACT
Parkinson's disease (PD), the second common neurodegenerative disease, is characterized by the loss of the dopaminergic neurons in the substantia nigra (SN). Because of L-dopa's side effects, new therapeutic methods were considered such as stem cells for PD treatment. The major challenge facing stem cell therapy following transplantations is their peripheral sequestration and decreased survival. Thus, the ability of erythropoietin (EPO) and low-level laser therapy (LLLT) to enhance mesenchymal stem cell (MSC) proliferation and therapeutic efficiency for alleviating rotenone-induced PD was investigated. Therefore, we compared the influence of stem cell preconditioning with erythropoietin versus LLLT on MSCs’ homing ability and the chances of survival. Forty-eight male Swiss mice were included in the in vivo protocol and ten in the in vitro work. The mice in the in vivo study were divided randomly into six groups (8 in each); group I; control group and group II; induced PD (untreated) group, group III; L-dopa, group IV; MSCs, group V; EPO-activated MSCs and group VI; laser-activated MSCs. Treatment with MSCs (either preconditioned or not) improved these neurological features by restoring the normal balance between glutamate and GABA neurotransmitters, and dopamine as well as increased tyrosine hydroxylase levels. Stem cell therapy decreased oxidative stress, miRNA-155 levels, enhanced neuronal architecture in SN, and decreased the number of apoptotic cells. LLLT enhanced MSC expression of integrin β1 which was reflected in the homing of PKH26-labelled MSCs into corpus striatum and SN. Thus, the optimum results were achieved with laser-activated MSCs.
1. Introduction
Parkinson’s disease (PD) is a widespread neurodegenerative disorder characterized by movement disorders (Dorsey and Bloem Citation2018). Accumulating evidence suggests that oxidative stress is a key driving factor for the complex degenerating cascade of PD (Dias et al. Citation2013). Meanwhile, the progressive loss of dopaminergic neurons in substantia nigra (SNc) must be treated or stopped (Dorsey and Bloem Citation2018).
Recently, the therapeutic potential of mesenchymal stem cells (MSCs) became highly promising in regenerative medicine and is widely viewed as a line for treating neurodegenerative disorders, particularly PD (Chen et al. Citation2020). The exact mechanism of stem cell-mediated therapy for PD wasn't yet fully understood. Some literature associated it with enhanced neurogenesis and revascularization, while others related it to the downregulation of apoptosis and anti-inflammatory effects (Baglio et al. Citation2012; Sun et al. Citation2017).
Stem cells can migrate and engraft to the site of damage (Lo Furno et al. Citation2018). Unfortunately, the major problems with stem cell transplantation are the rapid and massive loss after transplantation (Pagani et al. Citation2003). Thus, the challenge is to maintain their viability and survival (Wu et al. Citation2018).
In this context, pre-conditioning may be a therapeutic way to improve stem cell survival (Liu et al. Citation2005). Low-level laser therapy (LLLT), one form of photo-biomodulation, describes the utilization of laser or light-emitting diodes to encourage growth and improve both cell and tissue regeneration (Fekrazad et al. Citation2016). Applying LLLT for mouse MSCs was successful in stimulating their proliferation without causing nuclear alterations (Staffoli et al. Citation2017). Moreover, the application of LLLT to stem cells can increase their viability which may augment the success of regenerative treatment (Gutiérrez et al. Citation2021).
The ability of erythropoietin (EPO) to enhance MSC proliferation was previously reported, additionally the long-term MSCs culturing with EPO increases their resistance to various microenvironmental factors (Lykov et al. Citation2020). Furthermore, Zhou and colleagues (Citation2018) demonstrated that pre-treatment of MSCs with EPO before the infusion significantly increased their homing ability.
Thus, the current study investigated the therapeutic potential of stem cells and the capability to enhance it for combating a mouse model of Parkinson's disease.
To the best of our knowledge, the current study is the first to evaluate and compare the effectiveness of using LLLT versus EPO activation on the expression of integrin protein that mediates stem cell homing.
Furthermore, we sought to examine the effect of the BM-MSCs, either activated (LLLT/EPO) or not in attenuation of neuro-inflammation and apoptosis that can develop secondary to rotenone injection.
2. Materials and methods
2.1. Experimental animals
A total of fifty-eight adult male Swiss mice, weighing between 30–35 grams were included. Ten of them were used for an In vitro study, and the remaining were included in the in vivo study. The experimental procedures and animal care have been endorsed by the Institutional Animal Care and Use Committee-Cairo University (CU-IACUC); IACUC Protocol No.: CU/III/F/4/20.
2.2. The in vivo (animal) study
Forty-eight mice were randomly divided into six groups (n = 8 in each), then a baseline neurological examination was performed for all the mice. Subsequently, the mice in group I (the normal control group) received a vehicle. Whereas the mice in groups II, III, IV, V, and VI received rotenone that was injected subcutaneously at a dose of 1.5 mg/kg sparing a day between each dose dissolved in 0.1 ml of DMSO (dimethyl sulphoxide) over 2 weeks (Mitra et al. Citation2015).
After induction of PD, all the mice were subjected to neurological tests for the verification of the symptoms of the disease. Then, the mice in group II (induced PD): the untreated group; received no further medication. However, the mice in groups III, IV, V, and VI started to receive its medication as follows:
Group III (L-dopa): represents PD mice treated with an intraperitoneal injection of L-DOPA at a dose of 6 mg/kg/day for 4 weeks (Westin et al. Citation2001). Group IV (MSCs): represents PD mice treated with 5 × 105 BM-MSCs (Chi et al. Citation2019). Group V (EPO-activated MSCs): PD mice treated with 5 × 105 BM-MSCs preconditioned with EPO (at a dose of 50 IU/ml) for seven days (Liu et al. Citation2013). Group VI (Laser-activated MSCs): Parkinson's disease mice treated with 5 × 105 laser-activated BM-MSCs. BM-MSCs were injected once into the mice's tail veins. (Timeline for study; )
Then all mice were subjected to the third time of the neurological examination which was just before sacrifice.
2.3. Drugs and chemicals
Rotenone (RT), DMSO (dimethyl sulphoxide), L-DOPA methyl ester, and erythropoietin (EPO) were all purchased from Sigma-Aldrich Chemical Co. (St. Louis, Mo, USA)
2.4. Neurological examination
2.4.1. Ladder climbing test
The ladder was 88 cm in length x 6 cm in width; to which 43 round wooden rungs of 2 mm diameter were glued at equal intervals of 2 cm (Apostolova et al. Citation2006), and was secured in a slanted position at an angle of 45°. The ladder climbing test provided effectively assessed the complex motor behavior. The ladder was fixed. The mice climbing was recorded by video-camera and the ventral aspect of the animals was observed. All the mice have been evaluated and received a score ranging from 0 to 12 (Benkler et al. Citation2016).
2.4.2. Pole test
The pole test is used to assess the agility of the animals. In this test, a vertical wooden pole (50 cm height and 1 cm diameter) with a rough surface was placed in the home cage and each mouse had a trial to descend. The time required to turn (t-turn) and descend (t-descend) was measured (sec) (Fleming et al. Citation2004).
2.4.3. Wire -Hanging test
The wire-hanging test is known as the horizontal bar test that can assess muscle power (Crawley Citation1999). Briefly, the mice were suspended from the front limbs by a plastic rod (25 cm long and 0.2 cm diameter) placed 25 cm on the top of the bench. The time each mouse could stay hanging itself is referred to as latency with a 180-second cut-off time (Shao et al. Citation2019).
2.4.4. Forced swimming test
The mice were individually placed in an open cylindrical container filled with 19 cm water at 25 ± 1 °C. The mice were allowed to swim for 6 min, and the last 4 min were recorded, and then each mouse was judged for immobility (Ghasemi et al. Citation2009). Additionally, the climb time was recorded (Rial et al. Citation2014).
2.5. The in-vitro study
2.5.1. Bone marrow samples preparation
10 mice were sacrificed, and their femur bones were washed with saline several times. Then, the bone marrow cavity was opened under a sterile condition in the hood the cavities were irrigated 2–3 times with a syringe filled with 200–500 μl of phosphate buffer saline (PBS) and flushed into a 15 ml falcon (ShamsEldeen et al. Citation2019).
2.5.2. Isolation, culturing, and expansion of bone marrow-mesenchymal stem cells (BM-MSCs)
The marrow samples were centrifuged to get the mononuclear cell (MNC) layer. Then, the obtained MNCs were delicately mixed with 40 mL of PBS. The complete media utilized for culturing stem cells was composed of DMEM. Afterward, all the flasks were placed in the incubator at a constant temperature of 37 °C, 5% CO2, and maintained humidity. The cells were subcultured twice and maintained in the incubator until they reached 90% confluence (Bittira et al. Citation2003).
MSCs were identified at the 3rd passage through the morphology and Florescent Analysis Cell Sorting technique (FACS) for CD 90 +, and CD 105 +, and a negative of CD 34 +, and CD45 + was done ((a)–2(d)).
2.5.3. BM-MSCs preconditioning
The obtained BM-MSCs were divided into 3 groups: Group (a) Control group of non-conditioned (BM-MSCs), group (b) of EPO-activated BM-MSCs; cells were preconditioned with EPO (at a dose of 50 IU/ml) for seven days (Liu et al. Citation2013), and group (c) laser-activated BM-MSCs; cells were preconditioned with low-level laser.
To optimize the effect of low-level laser therapy, MSCs were exposed to 5 J/cm2, a power density of 5.5 mW/cm2, and a wavelength of 635 nm for 20 min (LED Technology Adilight2®) (Sayed et al. Citation2019).
All types of stem cells were injected at the same dose.
2.5.4. Cell proliferation assay and labelling of BM-MSCs using PKH-26s
The proliferation of non-conditioned and conditioned stem cells was determined using the colorimetric assay of 3-(4,5-dimethylthiazol-2-yl)−2.5-diphenyltetrazolium bromide (MTT) cell proliferation kit (Trevigen Inc., Gaithersburg, MD). All the procedures were performed according to the previously described manufacturer's instructions.
Then BM-MSCs were labeled with PKH26 red fluorescent cell linker kit PKH26 (Sigma-Aldrich, St. Louis, MO, USA) before being injected into the mice (ShamsEldeen et al. Citation2019).
2.5.5. Migration Assay for Detection of Stem Cell homing in mice Substantia Nigra by the Fluorescent Microscope
The substantia nigra's unstained sections were examined under a fluorescent microscope to detect PKH26-labeled cells in the tissue section of all MSCs-treated groups. The sections were examined with a Leica fluorescent microscope (Leica Microsystems CMS GmbH, Wetzlar, Germany).
2.6. Biochemical Measurements in Substantia Nigra Tissue Homogenate
2.6.1. Measurement of Glutamate and Gamma-Aminobutyric Acid (GABA)
The levels of glutamate and GABA were estimated using the designed mouse glutamate (GLU) ELISA kit for the quantitative determination of the mouse GLU (Cat. #MBS2601720, Mybiosource), and mouse gamma-aminobutyric acid (GABA) ELISA Kit for the quantitative determination of GABA (Cat. # MBS260709, Mybiosource).
2.6.2. Measurement of Brain-derived neurotrophic factor (BDNF), tumour Necrosis Factor alpha (TNF-α), reactive oxygen species (ROS), and glutathione (GSH) levels
The levels of BDNF, TNF-α, and ROS were estimated using the BDNF-mouse ELISA Kit (Cat. # MBS724249, Mybiosource), TNF-α mouse ELISA Kit (Cat. #BMS607-3, Invirtogen), and mouse ROS ELISA Kit (Cat. #; MBS728678, MyBioSource). The quantitative detection of the GSH concentration was also carried out using the Colorimetric Assay Kit (Cat. #K261-100; BioVision).
2.7. Biochemical Estimation of Dopamine levels, and Tyrosine Hydroxylase (TH) levels
After obtaining STR samples, tissues were homogenized, and the obtained homogenate was centrifuged. Then the supernatant was collected for assay of dopamine levels using a mouse Dopamine (DA) ELISA Kit (Cat. # MBS269234, Mybiosource).
The levels of TH were estimated in striatum (STR), and substantia nigra tissue homogenate using a mouse Tyrosine Hydroxylase (TH) ELISA Kit (Cat. # MBS2019774, Mybiosource).
2.8. Estimation of miR-155 in Substantia Nigra Tissue Homogenate, and integrin β1, and bcl-XL Expression Levels in MSCs
The total RNA including the miRNA was extracted from the tissue homogenate (SN) and cultured MSCs. Then the RNAs were detected after the purification with spectrophotometry (JENWAY, USA) at 260 nm. The generated RNA was reverse transcribed into cDNA by the high-capacity cDNA reverse transcription kit (#K4374966, Thermo Fisher Scientific, USA).
After performing amplification of Real-time qPCR, the analysis, and the assay with the primer was optimized. Using Sequence Detection Software Version No. 1.7, the obtained real-time assays were calculated using the comparative Ct method as stated by the manufacturer's recommendations (Applied Biosystems, USA).
The analysis of the miRNA was done using the microRNA primers. The data were normalized to the endogen control (SnU6RNA for miR-155, and b-actin for integrin and Bcl-xl) ().
Table 1. Primers Sequence of miR-155, integrin β1, and bcl-xl.
2.9. Immunohistochemical and Histo-Morphometric Study
After routine paraffin processing, the histological sections undergone heat-mediated antigen retrieval and incubation with rabbit polyclonal anti-Bcl2 (ab59348, 1:100, Abcam, USA) and the secondary antibody ImmPRESS™ HRP Anti-Rabbit IgG Polymer Detection Kit derived from horse serum (MP-7401, Vector, CA, USA) as previously described (El Asar et al. Citation2019).
The histomorphometric study included evaluation of the count of apoptotic neurons, the count of Bcl2-immunostained cells and grading of astrogliosis, vacuolation and angiopathy in the substantia nigra in six non-overlapping randomly selected microscopic fields of H&E-stained sections under magnification 400 inside a standard measuring frame of 85,550 mm2 using Leica Qwin-500 LTD-software image analysis computer system (Cambridge, England).
The apoptotic neurons count in the different study groups’ substantia nigra was performed in six non-overlapping randomly selected microscopic fields of H&E-stained sections under magnification 400 inside a standard measuring frame of 85,550 mm2 using Leica Qwin-500 LTD-software image analysis computer system (Cambridge, England).
2.10. Statistical Methods
The data were coded and entered using the statistical package for the Social Sciences (SPSS) version No.: 28 (IBM Corp., Armonk, NY, USA). The data were presented as mean and standard deviation. The comparisons between groups were done using variance (ANOVA) analysis with multiple comparisons post hoc tests in normally distributed quantitative variables while the non-parametric Kruskal–Wallis test and Mann–Whitney test (Chan Citation2003). P-values less than 0.05 were considered statistically significant. The correlations between the quantitative variables were performed using the Spearman correlation coefficient. P-values less than 0.05 were statistically significant.
3. Results
3.1. A-Neurological assessment
• Baseline Neurological Assessment:
The baseline assessment of neurological functions revealed that all mice had a ladder score of 0, and the time needed to turn and descend the vertical pole showed no statistical difference between groups. At baseline, the mice remained hanging for the whole test duration. The mean values of climbing time and immobility time showed no significant difference between groups.
• Verification of development of PD following rotenone injection:
Ladder climbing score and time to turn and descend (sec) at the pole test were significantly increased in all RT-injected groups compared to the control group.
Latency time (sec) at the wire hanging test and climbing time (sec) was significantly decreased in all RT-injected groups compared to the control group. whereas the immobility time significantly decreased in all RT-injected groups compared to the control group (Supplementary file 2 (Figures 1, 2, 3, and 4)).
• Verifying the efficiency of different lines of treatment at the end of the study:
The induced PD (untreated) group still has motor and non-motor symptoms of PD with no regression of disease symptoms. However, there was a significant decrease in ladder score in all treated groups compared to the induced-PD group. Laser-activated MSCs treated group showed a significant decrease in ladder score compared to the other treated groups.
Additionally, the mean values of descending time, and t-time in the laser-activated MSCs group were significantly lower than the L-dopa group. There was no significant difference regarding descending time between MSCs, EPO-activated MSCs, laser-activated MSCs, and the control group.
Wire hanging test latency time was significantly increased in all treated groups compared to the induced PD (untreated) group. The latency time was significantly increased in EPO-activated MSCs and laser-activated MSCs groups compared to MSCs group. With the superiority of Laser-activated MSCs over EPO-activated MSCs group.
The climbing time of the swimming test was significantly improved in all treated groups compared to the induced PD (untreated) group. Moreover, the laser-activated MSCs group showed a significant increase in climbing time compared to other MSCs groups. Laser-activated MSCs group immobility time was significantly lower than that of L-dopa and EPO-activated MSCs groups (Supplementary file (Figures 5–8)).
3.2. B-Biochemical results
• Glutamate, Gamma-aminobutyric acid (GABA), Glutamate/GABA ratio, Dopamine, and tyrosine hydroxylase (TH):
Glutamate level, and glutamate/GABA ratio were significantly increased, whereas GABA level was significantly decreased in the induced PD (untreated) group compared to that of the control group. The Laser-activated MSCs were able to induce a significant decrease in glutamate compared to both L-dopa and MSCs groups. Both EPO and laser-activated MSCs groups showed a significant increase in GABA and a significant decrease in glutamate/GABA ratio compared to L-dopa and MSCs groups ((a) and 3(b)).
Both dopamine and TH showed a significant decrease in the induced PD group compared to the control. Dopamine levels were significantly increased in all treated groups compared to the induced PD group. MSCs-treated groups showed a significant increase in both dopamine and TH compared to L-dopa, and the highest effectiveness was observed in the laser-activated MSCs group ().
Figure 3. (a, b): (a) Glutamate and gamma-aminobutyric acid (GABA) in substantia nigra tissue homogenate of all studied groups. (b) glutamate/GABA ratio. Values are presented as mean ± SD. *: statistically significant compared to the corresponding value in the control group (P < 0.05), #: statistically significant compared to the corresponding value in the untreated PD group (P < 0.05), $: statistically significant compared to the corresponding value in the L-dopa group (P < 0.05), @: statistically significant compared to the corresponding value in the MSCs group (P < 0.05), &: statistically significant compared to the corresponding value in EPO-activated MSCs group (P < 0.05) (n = 8).
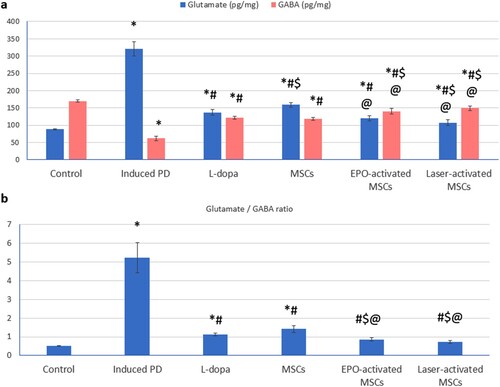
Figure 4. Dopamine, and tyrosine hydroxylase in STR of all studied groups, Values are presented as mean ± SD. *: statistically significant compared to the corresponding value in the control group (P < 0.05), #: statistically significant compared to the corresponding value in the untreated PD group (P < 0.05), $: statistically significant compared to the corresponding value in the L-dopa group (P < 0.05), @: statistically significant compared to the corresponding value in the MSCs group (P < 0.05), &: statistically significant compared to the corresponding value in EPO-activated MSCs group (P < 0.05) (n = 8).
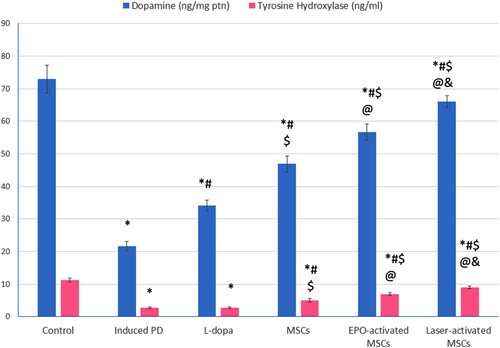
• Estimating TNF-α, BDNF, GSH, ROS, and miRNA-155 levels:
The induced PD group showed a significant increase in TNF-α, and ROS, and a significant decrease in BDNF, and GSH compared to the control group. Treatment with L-dopa, MSCs (either conditioned or not) significantly decreased TNF-α, and ROS, and significantly increased BDNF, and glutathione levels compared to the induced-PD (untreated) group. Treatment with EPO-activated MSCs and laser-activated MSCs significantly decreased TNF-α, and ROS and significantly increased BDNF and glutathione as well compared to L-dopa and MSCs ().
miRNA-155 was significantly increased in SN of the induced-PD (untreated) group (7.61 ± 0.8) compared to the control group (1.02 ± 0.01). Treatment with L-dopa (2.4 ± 0.46), MSCs (3.3 ± 0.35), EPO-activated MSCs (1.78 ± 0.38), and laser-activated MSCs (1.8 ± 0.35) showed a significant decrease (P < 0.05) in miRNA-155 level compared to both induced-PD (untreated) group and MSCs group ((a)).
Figure 5. (a, b): (a) Brain-derived neurotrophic factor (BDNF), tumour necrosis factor-alpha (TNF-α), (b) glutathione (GSH) level, and reactive oxygen species (ROS) in substantia nigra tissue homogenate of all studied groups Values are presented as mean ± SD. *: statistically significant compared to the corresponding value in the control group (P < 0.05), #: statistically significant compared to the corresponding value in the untreated PD group (P < 0.05), $: statistically significant compared to the corresponding value in the L-dopa group (P < 0.05), @: statistically significant compared to the corresponding value in the MSCs group (P < 0.05), &: statistically significant compared to the corresponding value in EPO-activated MSCs group (P < 0.05) (n = 8).
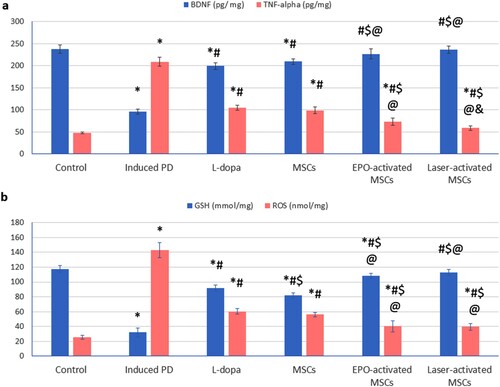
Figure 6. (a, b & c): (a) statistical analysis of miR-155, (b) count of apoptotic cells in substantia nigra, and (c) correlation study that showed a positive correlation between miR-155 and number of apoptotic cells. Data are represented as the mean of experiments ± S.D. All values are statistically significant at p < 0.05.
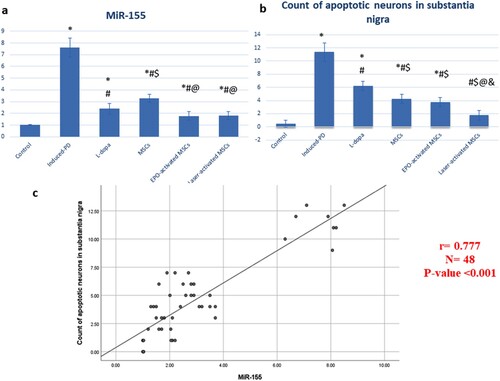
3.3. C-In vitro results
Both EPO and LLLT were able to increase MTT% significantly (113.33 ± 4.23, and 135.17 ± 8.54 respectively) compared to non-conditioned cells (99.5 ± 1.87). Additionally, the expression levels of integrin β1 were significantly increased in EPO and laser-activated BM-MSCs (3.11 ± 0.48, and 4.46 ± 0.37 respectively) compared to non-conditioned cells (1.01 ± 0.01). In this study, we found enhanced expression of the Bcl-xl gene secondary to EPO and LLLT preconditioning (4.56 ± 0.29, and 6.64 ± 0.71 respectively) compared to non-conditioned cells (1.02 ± 0.02). The increased MTT% and expression of integrin β1 anti-apoptotic Bcl-xl were all significantly increased in Laser-activated BM-MSCs compared to EPO-activated BM-MSCs.
• EPO-activated MSCs and laser-activated MSCs improves substantia nigra apoptosis nuclear pyknosis, astrogliosis, and neuropil vacuolation:
Treatment with L-dopa (6.25 ± 0.71), MSCs (4.25 ± 0.71), EPO-activated MSCs (3.75 ± 0.71), and laser-activated MSCs (1.75 ± 0.71) showed a significant decrease in the count of apoptotic neurons compared to induced-PD (untreated) group.
Treatment with EPO-activated MSCs and laser-activated MSCs showed a significant decrease in the count of apoptotic neurons compared to the MSCs group. Treatment with laser-activated MSCs showed a significant decrease in the count of apoptotic neurons compared to the EPO-activated MSCs group ((b)).
Evaluation of H&E-stained sections of the substantia nigra region of the Parkinson group displayed numerous dark pyknotic neurons in the vicinity of infiltrated glial cells, interstitial edema, and areas of marked neuronal loss in highly vacuolated neuropil. The L-dopa group displayed few Lewy body inclusions along with marked glial cell infiltration surrounding the degenerated dopaminergic neurons, other dopaminergic neurons appeared normal with vesicular nuclei and lightly stained cytoplasm in healthy neuropil, The MSCs group showed improvement in the neuronal architecture but with residual peri-neuronal halos and Lewy’s inclusions. The EPO-activated MSCs group showed several intact neurons with only a few degenerated ones with karyolitic nuclei. The Laser-activated MSCs group exhibited numerous neurons with preserved structure in an intact neuropil akin to the control group ((a)–7(f)).
Figure 7. (a-f): Photomicrograph (H&E) of the substantia nigra region of (a) control mice displaying normal dopaminergic neuronal cells (N) with vesicular nuclei and lightly stained cytoplasm in a healthy neuropil, (b) Induced parkinsonism group showing numerous dark pyknotic neurons in the vicinity of infiltrated glial cells (circle), interstitial edema (E). Areas of marked neuronal loss in highly vacuolated neuropil are also observed (v), (c) L-dopa group displaying few Lewy body inclusions (L) and marked glial cell infiltration surrounding the degenerated dopaminergic neurons (circle), (d) MSCs group showing improvement in the neuronal architecture but with residual peri-neuronal halos (H) and Lewy’s inclusions (L), (e) EPO-activated MSCs group showing several intact neurons with only a few degenerated ones with karyolitic nuclei (curved arrows), (f) Laser-activated MSCs group exhibiting numerous neurons with preserved structure in intact neuropil akin to the control group. (g) Scoring of astrogliosis, neuronal vascuolation and microgliosis in the different study groups at (P < 0.05) using ANOVA, Bonferroni post hoc testing. Values are presented as mean ± SD. *: significant versus control, #: significant versus PD, $: significant versus L-dopa, @: significant versus MSCs (P < 0.05), &: significant versus EPO-activated MSCs (n = 8).
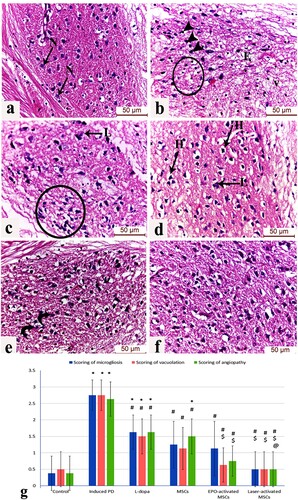
Evaluation of the striatum (caudate/putamen) of the Parkinsonism group displayed shrunken apoptotic neurons in a picture of astrogliosis and degenerated Wilson fibres. The blood vessels showed mild congestion and widening of the perivascular (Virchow-Robin) spaces separating the vessel from the neuropil. The L-dopa group exhibited few degenerated neurons surrounded by glial cells. The MSCs group showed improvement in the striatal neuronal and neuropil architecture. The EPO-activated MSCs group displayed preservation of the striatal structure with minimal nuclear pyknosis. The Laser-activated MSCs group showed the preserved structure of the striatum with healthy neurons, blood capillaries, and pencil fibres of Wilson unique to the striatum in addition to glial cells with the classical fried-egg appearance ((a)–8(f)).
Figure 8. (a-f): Effect of laser and erythropoietin mesenchymal stem cell enhancement on L dopa-induced striatal histological changes. H&E-stained sections of the striatum (caudate/putamen) of (a) control group showing normal structure of striatum displayed as healthy neurons (N), blood capillaries (B), and pencil fibres of Wilson (W) unique to the striatum in addition to glial cells (G) with the classical fried-egg appearance. (b) Induced Parkinsonism group showing shrunken apoptotic neurons in a picture of astrogliosis and degenerated Wilson fibres (W). Blood vessels show mild congestion and widening of the perivascular (Virchow-Robin) spaces separating the vessel from the neuropil (spiral arrow) are also noticed. (c) L-dopa group showing degenerated neurons surrounded by glial cells (circle) (d) MSCs group showing moderate improvement of the striatal neuronal and neuropil architecture (f) EPO-activated MSCs group displaying preservation of the striatal structure with minimal nuclear pyknosis. (e) Laser-activated MSCs group showing the preserved structure of the neurons and glial cells. (H&E x400, scale bar 50 μm) (n = 8).
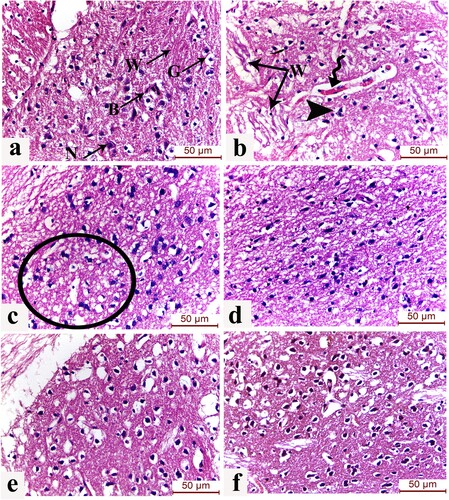
• Immunohistochemical results
BCL-2 immunostained sections in substantia nigra region of control group revealed widespread immunostaining of dopaminergic neuronal cells. The Parkinson group exhibited negative BCL-2 immunostained pyknotic neurons with significant decrease of the mean count of BCL-2 immunostained cells. The L-dopa group demonstrated numerous negative BCL-2 immunostained pyknotic neuron among few BCL-2 positive immunostained dopaminergic neuronal cells. The MSCs group displayed significant increase of the mean count of BCL-2 immunostained cells. The EPO-activated MSCs and Laser-activated MSCs groups showed numerous positive immunostained dopaminergic neuronal cells with significant increase of mean count of BCL-2 immunostained cells ((a)–9(g)).
Figure 9. (a-g): Photomicrograph of BCL-2 immunostained sections in substantia nigra region of (a) control mice exhibiting widespread BCL-2 immunostaining of dopaminergic neuronal cells (arrows) with minimal negative BCL-2 immunostained neuronal cells (bifid arrow). (b) Induced parkinsonism group displaying many negative BCL-2 immunostained pyknotic neurons (bifid arrows). (c) L-dopa group revealing negative BCL-2 immunostained pyknotic neuron (bifid arrow) among some BCL-2 positive immunostained dopaminergic neuronal cells (arrows). (d) MSCs group displaying some negative BCL-2 immunostained pyknotic neuron (bifid arrows) among BCL-2 positive immunostained dopaminergic neuronal cells (arrows). (e) EPO-activated MSCs group exhibiting BCL-2 positive immunostained dopaminergic neuronal cells (arrows) with few negative BCL-2 immunostained neuronal cells (bifid arrows). (f) Laser-activated MSCs group showing many BCL-2 positive immunostained dopaminergic neuronal cells (arrows) with few negative BCL-2 immunostained neuronal cells (bifid arrows). (Scale Bar 50 µm) (g) Count of Bcl2-immunostained cells in the different study groups at (P < 0.05) using ANOVA, Bonferroni post hoc testing. Values are presented as mean ± SD. *: significant versus control, #: significant versus PD, $: significant versus L-dopa, @: significant versus MSCs (P < 0.05), &: significant versus EPO-activated MSCs (n = 8).
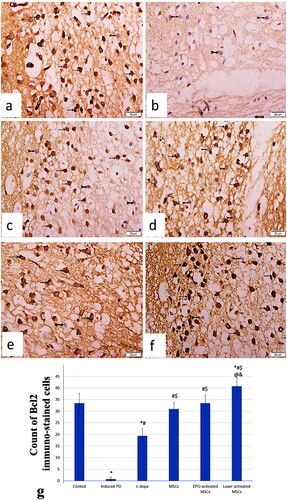
• Erythropoietin and Laser activation enhance homing of the MSCs into the substantia nigra:
The count of PKH26-labeled immunofluorescent cells was significantly increased in both the EPO-activated MSCs (87.75 ± 5.6) and Laser-activated MSCs (111.13 ± 8.11) compared to the MSCs group (74.75 ± 7.61). The count of PKH26-labeled MSCs was significantly increased in Laser-activated MSCs compared to EPO-activated MSCs ().
3.4. D-Correlations
As shown in (c), a positive correlation between miR-155 and the number of apoptotic cells in substantia nigra was observed (r = 0.777, and P-value <0.001).
Figure 10. (a & b): (a) PKH26-labeled immunofluorescent images showing enhanced homing of the MSCs into the corpus striatum and substantia nigra with Laser and erythropoietin activation. (b): Statistical analysis of PKH26-labeled MSCs in MSCs, EPO-activated MSCs, and Laser-activated MSCs. Values are presented as mean ± SD. @ statistically significant compared to the corresponding value in the MSCs group (P < 0.05). &: statistically significant compared to the corresponding value in EPO-activated MSCs group (P < 0.05) (n = 8).
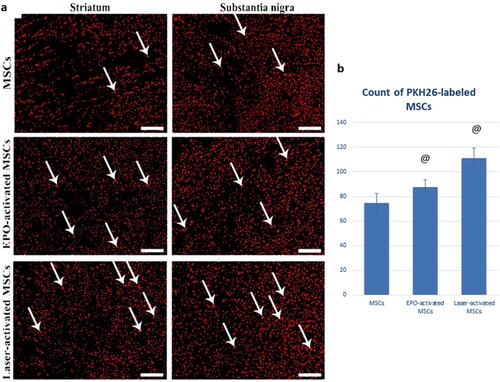
3. Discussion
Rotenone (RT), the lipophilic naturally occurring insecticide, can easily cross the blood–brain barrier (BBB) (Johnson and Bobrovskaya Citation2015). Once it enters the cell, RT accumulates in different organelles, including the mitochondria, where it binds specifically to complex I, and increases ROS production, leading eventually to oxidative damage and cell death (Xiong et al. Citation2012). Rotenone-treated rodents showed behavioral changes consistent with PD (Sherer et al. Citation2003). At tissue levels, its effects are accompanied by a highly selective nigrostriatal dopaminergic neuronal degeneration and the presence of cytoplasmic inclusions containing α-synuclein, resembling Lewy bodies found in humans with PD (Cannon et al. Citation2009). The effect of rotenone administration was confirmed in the present study, and the obtained neurological results documented the successful induction of PD.
L-dopa is the gold standard therapy for PD. However, it causes many side effects, and the most important adverse effect is the development of motor complications such as fluctuations, dyskinesia, dystonia, and wearing-off (Jankovic and Aguilar Citation2008).
BM-MSC transplantation is a promising approach for treating neurodegenerative disorders because of its homing capability to the injured area, and its potential for tissue repair (Capitelli et al. Citation2014). At the injury site, the stem cells release many trophic factors that can accelerate tissue repair and control local inflammation (Glavaski-Joksimovic and Bohn Citation2013).
Our results reinforce the beneficial effects of BM-MSCs. The data of our in vitro study clarified the in vivo therapeutic potential of both EPO and laser-activated stem cells. In agreement with Abdelwahab et al. (Citation2021), stem cell homing into the corpus striatum and substantia nigra was detected by the number of PKH26-labeled cells. At tissue levels, there was a dramatic increase in the count of the homed cells detected in the unstained brain sections of the EPO-activated, and laser-activated MSCs groups compared to the MSCs group.
The stem cell adhesion to the tissue matrix is mainly mediated by integrins that can improve cell survival and therapeutic potentials (Cai et al. Citation2016; Li et al. Citation2017). In this regard, the current results showed a significant increase in expression levels of anti-apoptotic Bcl-xl and integrin β1 in EPO and LLLT preconditioned cells compared to the non-conditioned cells, thus it may explain the better homing of both EPO and laser-activated MSCs compared to MSCs group. The pretreatment of MSCs with EPO resulted in a significant increase in stem cell viability and migration (Wang et al. Citation2020).
Photobiomodulation therapy, particularly LLLT, has multiple advantages such as promoting cell proliferation. The application of LLLT of 635 nm with an energy density of 5 J/cm2 increases the proliferation rate of bone marrow-derived mesenchymal stem cells (Gutiérrez et al. Citation2021). This also was reported by Soleimani et al. (Citation2012), who analysed the effect of LLLT applied on human BM-stem cells and observed a significant increase in the proliferation rate of irradiated cells compared to the non-irradiated cells.
In our in vivo study, high levels of glutamate that may result in the excitotoxic death of neurons were observed in rotenone-treated mice. The decrease in GABA level can be explained by the low dopamine level in the striatum because of the dopaminergic degeneration (Sharma et al. Citation2020).
Currently, all the MSCs treated groups showed decreased glutamate and increased GABA levels. In a study conducted by Seo and colleagues (Citation2011), utilizing the human umbilical cord blood-derived mesenchymal stem caused a rise in GABA levels.
In our study, the neurotrophic ability of MSCs was observed, which complies with Somoza and colleagues (Citation2010) who concluded that MSCs might be strong candidates for treating Parkinson’s disease. BDNF exerts its neuroprotective activity and promotes synaptic plasticity (Castrén and Antila Citation2017), dendritic growth, pine maturation and stabilization, development of synapses, apoptosis, and neurons’ survival (Palasz et al. Citation2020).
The treatment with either EPO-activated or laser-activated MSCs resulted in a significant decrease in miRNA-155 in comparison with both the induced-PD and MSCs groups. miRNA-155 is the epigenetic regulator of inflammatory processes. The overexpression of miRNA-155 associates the decreased BDNF, and increased caspase-3 activity (Duan et al. Citation2018). The current study pointed to the important role of miRNA-155 in cell survival, where the miR-155 levels positively correlated with the apoptotic cells in SN number. In line with previous studies, silencing the miR-155 promoted cell proliferation inhibited apoptosis, and alleviated inflammation (Chen et al. Citation2019).
We recommend further investigations to explore other dysregulated miRNAs that may be involved in the pathogenesis and the progression of PD disease and uncover their mechanisms and targets. Therefore, future studies can develop a potential drug that may be a candidate for treating PD.
In conclusion, the treatment with MSCs (either activated or not) resulted in the improvement of the cognitive functions that were confirmed by the neurological examination. Both EPO and LLLT of BM-MSCs were able to improve the expression of the integrin β1 and Bcl-xl, with the superiority of LLLT over EPO, thus it can explain the increased number of homed cells in SN. The apoptotic cells in SN number decreased with all the treatment lines, however, the best results were obtained with the laser-activated MSCs.
Disclosure statement
No potential conflict of interest was reported by the author(s).
Data availability statement
All data are available from the corresponding author.
References
- Abdelwahab S, Elsebay SAG, Fouli Gaber M, Abdel-Hafez SMN. 2021. Comparative study between bone marrow mesenchymal stem cell and their conditioned medium in the treatment of rat model of parkinsonism. J Cell Physiol. 236(1):440–457. doi:10.1002/jcp.29872.
- Apostolova I, Irintchev A, Schachner M. 2006. Tenascin-R restricts posttraumatic remodeling of motoneuron innervation and functional recovery after spinal cord injury in adult mice. J Neurosci. 26(30):7849–7859. doi:10.1523/JNEUROSCI.1526-06.2006.
- Baglio SR, Pegtel DM, Baldini N. 2012. Mesenchymal stem cell secreted vesicles provide novel opportunities in (stem) cell-free therapy. Front Physiol. 3:359. doi:10.3389/fphys.2012.00359.
- Benkler C, Barhum Y, Ben-Zur T, Offen D. 2016. Multifactorial gene therapy enhancing the glutamate uptake system and reducing oxidative stress delays symptom onset and prolongs survival in the SOD1-G93A ALS mouse model. J Mol Neurosci. 58(1):46–358. doi:10.1007/s12031-015-0695-2.
- Bittira B, Shum-Tim D, Al-Khaldi A, Chiu RC. 2003. Mobilization and homing of bone marrow stromal cells in myocardial infarction. Eur J Cardiothorac Surg. 24(3):393–398. doi:10.1016/S1010-7940(03)00325-7.
- Cai WF, Liu GS, Wang L, Paul C, Wen ZL, Wang Y. 2016. Repair injured heart by regulating cardiac regenerative signals. Stem Cells Int. 2016:6193419.
- Cannon JR, Tapias V, Na HM, Honick AS, Drolet RE, Greenamyre JT. 2009. A highly reproducible rotenone model of Parkinson's disease. Neurobiol Dis. 34(2):279–290. doi:10.1016/j.nbd.2009.01.016.
- Capitelli CS, Lopes CS, Alves AC, Barbiero J, Oliveira LF, da Silva VJD, Vital MABF. 2014. Opposite effects of bone marrow-derived cells transplantation in MPTP-rat model of Parkinson's disease: a comparison study of mononuclear and mesenchymal stem cells. Int J Med Sci. 11(10):1049. doi:10.7150/ijms.8182.
- Castrén E, Antila H. 2017. Neuronal plasticity and neurotrophic factors in drug responses. Mol Psychiatry. 22(8):1085–1095. doi:10.1038/mp.2017.61.
- Chan YH. 2003. Biostatistics102: quantitative data – parametric & Non-parametric tests. Singapore Med J. 44(8):391–396.
- Chen J, Li C, Liu W, Yan B, Hu X, Yang F. 2019. MiRNA-155 silencing reduces sciatic nerve injury in diabetic peripheral neuropathy. J Mol Endocrinol. 63(3):227–238. doi:10.1530/JME-19-0067.
- Chen Y, Shen J, Ke K, Gu X. 2020. Clinical potential and current progress of mesenchymal stem cells for Parkinson’s disease: a systematic review. Neurol Sci. 41(5):1051–1061.
- Chi H, Guan Y, Li F, Chen Z. 2019. The effect of human umbilical cord mesenchymal stromal cells in protection of dopaminergic neurons from apoptosis by reducing oxidative stress in the early stage of a 6-OHDA-induced Parkinson’s disease model. Cell Transplant. 28(1_suppl):87S–99S. doi:10.1177/0963689719891134.
- Crawley J. 1999. Behavioral phenotyping of transgenic and knockout mice: experimental design and evaluation of general health, sensory functions, motor abilities, and specific behavioral tests1Published on the world wide Web on 2 December 1998.1. Brain Res. 835(1):18–26. doi:10.1016/S0006-8993(98)01258-X.
- Dias V, Junn E, Mouradian MM. 2013. The role of oxidative stress in Parkinson's disease. J Parkinsons Dis. 3(4):461–491. doi:10.3233/JPD-130230.
- Dorsey ER, Bloem BR. 2018. The Parkinson pandemic—a call to action. JAMA Neurol. 75(1):9–10. doi:10.1001/jamaneurol.2017.3299.
- Duan W, Chen Y, Wang XR. 2018. MicroRNA-155 contributes to the occurrence of epilepsy through the PI3 K/Akt/mTOR signaling pathway. Int J Mol Med. 42(3):1577–1584.
- El Asar HM, Mohammed EA, Aboulhoda BE, Emam HY, Imam AAA. 2019. Selenium protection against mercury neurotoxicity: modulation of apoptosis and autophagy in the anterior pituitary. Life Sci. 231:116578. doi:10.1016/j.lfs.2019.116578.
- Fekrazad R, Eslaminejad MB, Shayan AM, Kalhori KA, Abbas FM, Taghiyar L, Pedram MS, Ghuchani MS. 2016. Effects of photobiomodulation and mesenchymal stem cells on articular cartilage defects in a rabbit model. Photomed Laser Surg. 34(11):543–116549. doi:10.1089/pho.2015.4028.
- Fleming SM, Salcedo J, Fernagut PO, Rockenstein E, Masliah E, Levine MS, Chesselet MF. 2004. Early and progressive sensorimotor anomalies in mice overexpressing wild-type human α-synuclein. J Neurosci. 24(42):9434–9440. doi:10.1523/JNEUROSCI.3080-04.2004.
- Ghasemi M, Sadeghipour H, Poorheidari G, Dehpour AR. 2009. A role for nitrergic system in the antidepressant-like effects of chronic lithium treatment in the mouse forced swimming test. Behav Brain Res. 200(1):76–82. doi:10.1016/j.bbr.2008.12.032.
- Glavaski-Joksimovic A, Bohn MC. 2013. Mesenchymal stem cells and neuroregeneration in Parkinson's disease. Exp Neurol. 247:25–38. doi:10.1016/j.expneurol.2013.03.016.
- Gutiérrez D, Rouabhia M, Ortiz J, Gaviria D, Alfonso C, Muñoz A, Inostroza C. 2021. Low-Level laser irradiation promotes proliferation and differentiation on apical papilla stem cells. J Lasers Med Sci. doi:10.34172/jlms.2021.75.
- Jankovic J, Aguilar LG. 2008. Current approaches to the treatment of Parkinson’s disease. Neuropsychiatr Dis Treat. 4(4):743–757. doi:10.2147/NDT.S2006.
- Johnson ME, Bobrovskaya L. 2015. An update on the rotenone models of Parkinson's disease: their ability to reproduce the features of clinical disease and model gene–environment interactions. Neurotoxicology. 46:101–116. doi:10.1016/j.neuro.2014.12.002.
- Li L, Guan Q, Dai S, Wei W, Zhang Y. 2017. Integrin β1 increases stem cell survival and cardiac function after myocardial infarction. Front Pharmacol. 8:135.
- Liu J, Narasimhan P, Yu F, Chan PH. 2005. Neuroprotection by hypoxic preconditioning involves oxidative stress-mediated expression of hypoxia-inducible factor and erythropoietin. Stroke. 36(6):1264–1269. doi:10.1161/01.STR.0000166180.91042.02.
- Liu NM, Tian J, Wang WW, Han GF, Cheng J, Huang J, Zhang JY. 2013. Effect of erythropoietin on mesenchymal stem cell differentiation and secretion in vitro in an acute kidney injury microenvironment. Genet Mol Res. 12(4):6477–6487. doi:10.4238/2013.February.28.14.
- Lo Furno D, Mannino G, Giuffrida R. 2018. Functional role of mesenchymal stem cells in the treatment of chronic neurodegenerative diseases. J Cell Physiol. 233(5):3982–3999. doi:10.1002/jcp.26192.
- Lykov AP, Surovtseva MA, Kim II, et al. 2020. Effect of erythropoietin on morphofunctional properties of mesenchymal stem cells. Bull Exp Biol Med. 170:164–170. doi:10.1007/s10517-020-05024-z.
- Mitra S, Chakrabarti N, Dutta SS, Ray S, Bhattacharya P, Sinha P, Bhattacharyya A. 2015. Gender-specific brain regional variation of neurons, endogenous estrogen, neuroinflammation and glial cells during rotenone-induced mouse model of Parkinson's disease. Neuroscience. 292:46–70. doi:10.1016/j.neuroscience.2014.12.052.
- Pagani FD, DerSimonian H, Zawadzka A, Wetzel K. 2003. Autologous skeletal myoblasts transplanted to ischemia-damaged myocardium in humans. J Am Coll Cardiol. 41(5):879–888. doi:10.1016/S0735-1097(03)00081-0.
- Palasz E, Wysocka A, Gasiorowska A, Chalimoniuk M, Niewiadomski W, Niewiadomska G. 2020. BDNF as a promising therapeutic agent in Parkinson’s disease. Int J Mol Sci. 21(3):1170. doi:10.3390/ijms21031170.
- Rial D, Castro AA, Machado N, Garção P, Gonçalves FQ, Silva HB, Tomé AR, Köfalvi A, et al. 2014. Behavioral phenotyping of parkin-deficient mice: looking for early preclinical features of Parkinson's disease. PloS one. 9(12):e114216. doi:10.1371/journal.pone.0114216.
- Sayed SY, Salem SI, Abdallah AN, Khalil GM, Mohammed FF. 2019. Clinicopathological studies on the use of laser-activated adipose-derived stromal vascular fraction in treatment of streptozotocin-induced diabetes in rats. Comp Clin Path. 28(5):1515–1526. doi:10.1007/s00580-019-03008-8.
- Seo Y, Yang SR, Jee MK, Joo EK, Roh KH, Seo MS, Han TH, Lee SY, et al. 2011. Human umbilical cord blood-derived mesenchymal stem cells protect against neuronal cell death and ameliorate motor deficits in niemann pick type C1 mice. Cell Transplant. 20(7):1033–1047. doi:10.3727/096368910X545086.
- ShamsEldeen AM, Ashour H, Shoukry HS, Fadel M, Kamar SS, Aabdelbaset M, Rashed LA, Ammar HI. 2019. Combined treatment with systemic resveratrol and resveratrol preconditioned mesenchymal stem cells, maximizes antifibrotic action in diabetic cardiomyopathy. J Cell Physiol. 234(7):10942–10963. doi:10.1002/jcp.27947.
- Shao QH, Chen Y, Li FF, Wang S, Zhang XL, Yuan YH, Chen NH. 2019. TLR4 deficiency has a protective effect in the MPTP/probenecid mouse model of Parkinson’s disease. Acta Pharmacol Sin. 40(12):1503–1512. doi:10.1038/s41401-019-0280-2.
- Sharma S, Raj K, Singh S. 2020. Neuroprotective effect of quercetin in combination with piperine against rotenone- and iron supplement–induced Parkinson’s disease in experimental rats. Neurotox Res. 37(1):198–209. doi:10.1007/s12640-019-00120-z.
- Sherer TB, Betarbet R, Testa CM, Seo BB, Richardson JR, Kim JH, Miller GW, Yagi T, et al. 2003. Mechanism of toxicity in rotenone models of Parkinson's disease. J Neurosci. 23(34):10756–10764. doi:10.1523/JNEUROSCI.23-34-10756.2003.
- Soleimani M, Abbasnia E, Fathi M, Sahraei H, Fathi Y, Kaka G. 2012. The effects of low-level laser irradiation on differentiation and proliferation of human bone marrow mesenchymal stem cells into neurons and osteoblasts—an in vitro study. Lasers Med Sci. 27(2):423–430. doi:10.1007/s10103-011-0930-1.
- Somoza R, Juri C, Baes M, Wyneken U, Rubio FJ. 2010. Intranigral transplantation of epigenetically induced BDNF-secreting human mesenchymal stem cells: implications for cell-based therapies in Parkinson's disease. Biol Blood Marrow Transplant. 16(11):1530–1540. doi:10.1016/j.bbmt.2010.06.006.
- Staffoli S, Romeo U, Amorim RNS, Migliau G, Palaia G, Resende L, Polimeni A. 2017. The effects of low level laser irradiation on proliferation of human dental pulp: a narrative review. Clin Ter. 168(5):e320–e326.
- Sun L, Li D, Song K, Wei J, Yao S, Li Z, Su X, Ju X, Chao L, Deny X, et al. 2017. Ror2 signaling regulates Golgi structure and transport through IFT20 for tumor invasiveness. Sci Rep. 7(1):1–13. doi:10.1038/s41598-016-0028-x.
- Wang X, Wang H, Lu J, Feng Z, Liu Z, Song H, Wang H, Zhou Y, Xu J. 2020. Erythropoietin-modified mesenchymal stem cells enhance anti-fibrosis efficacy in mouse liver fibrosis model. Tissue Engineering and Regenerative Medicine. 17(5):683–693. doi:10.1007/s13770-020-00276-2.
- Westin JE, Andersson M, Lundblad M, Cenci MA. 2001. Persistent changes in striatal gene expression induced by long-terml-DOPA treatment in a rat model of Parkinson's disease. Eur J Neurosci. 14(7):1171–1176. doi:10.1046/j.0953-816x.2001.01743.x.
- Wu S, FitzGerald KT, Giordano J. 2018. On the viability and potential value of stem cells for repair and treatment of central neurotrauma: overview and speculations. Front Neurol. 9:602. doi:10.3389/fneur.2018.00602.
- Xiong N, Long X, Xiong J, Jia M, Chen C, Huang J, Ghoorah D, Kong X, Lin Z, Wang T. 2012. Mitochondrial complex I inhibitor rotenone-induced toxicity and its potential mechanisms in Parkinson’s disease models. Crit Rev Toxicol. 42(7):613–632. doi:10.3109/10408444.2012.680431.
- Zhou S, Liu YG, Zhang Y, Hu JM, Liu D, Chen H, Li M, Guo Y, Fan LP, et al. 2018. Bone mesenchymal stem cells pretreated with erythropoietin enhance the effect to ameliorate cyclosporine A-induced nephrotoxicity in rats. J Cell Biochem. 119(10):8220–8232. doi:10.1002/jcb.26833.