ABSTRACT
Pulmonary arterial hypertension (PAH) is characterized by vascular remodeling associated with extracellular matrix (ECM) deposition, vascular cell hyperproliferation, and neointima formation in the small pulmonary artery. Endothelial dysfunction is considered a key feature in the initiation of vascular remodeling. Although vasodilators have been used for the treatment of PAH, it remains a life-threatening disease. Therefore, it is necessary to identify novel therapeutic targets for PAH treatment. Periostin (POSTN) is a secretory ECM protein involved in physiological and pathological processes, such as tissue remodeling, cell adhesion, migration, and proliferation. Although POSTN has been proposed as a potential target for PAH treatment, its role in endothelial cells has not been fully elucidated. Here, we demonstrated that POSTN upregulation correlates with PAH by analyzing a public microarray conducted on the lung tissues of patients with PAH and biological experimental results from in vivo and in vitro models. Moreover, POSTN overexpression leads to ECM deposition and endothelial abnormalities such as migration. We found that PAH-associated endothelial dysfunction is mediated at least in part by the interaction between POSTN and integrin-linked protein kinase (ILK), followed by activation of nuclear factor-κB signaling. Silencing POSTN or ILK decreases PAH-related stimuli-induced ECM accumulation and attenuates endothelial abnormalities. In conclusion, our study suggests that POSTN serves as a critical regulator of PAH by regulating vascular remodeling, and targeting its role as a potential therapeutic strategy for PAH.
Introduction
Pulmonary hypertension (PH) is classified into five groups according to its hemodynamic characteristics, clinical presentation, pathophysiological mechanisms, and therapeutic management (Simonneau et al. Citation2019). Pulmonary arterial hypertension (PAH) belongs to group 1 and is defined as an increase in the mean pulmonary arterial pressure (PAP) by > 25 mmHg at rest (Simonneau et al. Citation2019; Yeo et al. Citation2022). PAH is a rare disease with a low survival rate and is characterized by vascular remodeling accompanied by pulmonary arterial vasoconstriction due to neointima formation and hyperproliferation of pulmonary vascular cells, such as smooth muscle cells (SMCs) and endothelial cells (ECs) (Lee et al. Citation2014; Simonneau et al. Citation2019; Yun et al. Citation2020; Chen et al. Citation2022). Vascular remodeling elevates vascular resistance and PAP, eventually leading to right ventricular heart failure (Lee et al. Citation2014; Ranchoux et al. Citation2018; Simonneau et al. Citation2019; Yun et al. Citation2020). Endothelial dysfunction has been proposed as the main player in the pathology of PAH because various studies have demonstrated that EC proliferation, angiogenesis, and endothelial-to-mesenchymal transition (EndMT) are closely associated with vascular remodeling (Ranchoux et al. Citation2018; Yun et al. Citation2020; Russomanno et al. Citation2021; Yun et al. Citation2023). In addition, extracellular matrix (ECM) degradation and reorganization contribute to vascular remodeling in the pathological region of PAH (Thenappan et al. Citation2018; Yun et al. Citation2020; Ambade et al. Citation2021). Given that only vasodilators focused on symptomatic relief have been developed and used for the treatment of PAH (Lee et al. Citation2014; Yun et al. Citation2020), it is important to identify and utilize therapeutic targets related to the pathogenesis of PAH.
Periostin (POSTN) is a secretory ECM protein and acts as a ligand for cell membrane integrins and regulates ECM organization by serving as a scaffold for ECM proteins such as fibronectin (FN), tenascin C (TNC), collagen, and laminin and accessory proteins such as bone morphogenetic protein 1 (BMP-1) and cellular communication network factor 3 (CCN3) (Snider et al. Citation2008; Kudo and Kii Citation2018; Seki et al. Citation2019; Kim BR et al. Citation2022). POSTN is involved in tissue remodeling, migration, invasion, and metastasis in various cancers (Dorafshan et al. Citation2022). Previous studies have demonstrated that POSTN expression is correlated with PAH (Abdul-Salam et al. Citation2010; Kumar et al. Citation2015; Wang XD et al. Citation2016; Seki et al. Citation2019; Nie X et al. Citation2020; Wang J et al. Citation2022; Yoshida et al. Citation2022). POSTN expression was increased in the lung tissue or serum of patients with PAH (Abdul-Salam et al. Citation2010; Kumar et al. Citation2015; Wang J et al. Citation2022; Yoshida et al. Citation2022). Pulmonary vascular remodeling was attenuated in POSTN -/- SU5416 combined with hypoxia (SuHx) mouse model (Yoshida et al. Citation2022). POSTN expressing cell-specific transforming growth factor beta (TGF-β) signaling inhibition animal model (Pn-Cre/Tgfbr1fl/fl mice) prevents PH (Seki et al. Citation2019). Although a few studies have suggested importance of POSTN in PAH, investigations on functional roles of EC-derived POSTN in ECM remodeling has not been fully explored.
Integrin-linked protein kinase (ILK), a serine/threonine kinase, plays an important role in various physiological processes such as signal transduction, cell survival, cell adhesion, and ECM assembly by anchoring to integrins (Hannigan et al. Citation1996; Wu and Dedhar Citation2001; Shen et al. Citation2020). Previous studies have shown that POSTN promotes ILK expression in leukemia (Ma et al. Citation2019), renal cancer (Jia YY et al. Citation2021), macrophages (Wei et al. Citation2023), and endometrial epithelial cells (Zheng et al. Citation2016). Reportedly, elevated ILK expression is seen in the lung tissue of patients with PAH and in animal models of PAH, and ILK inhibitors attenuates PAH in animal models (Kudryashova et al. Citation2016; Shen et al. Citation2020). However, the relationship between POSTN and ILK in pulmonary arterial endothelial cells (PAECs) and their roles in PAH have not been studied.
In this study, we determined the role of POSTN in vascular remodeling. We observed the upregulation of POSTN in the lung tissue of PAH animal models and various PAH-related stimuli in PAECs. POSTN overexpression upregulates ECM proteins and induces endothelial abnormalities. Treatment with PAH-related stimuli showed similar effects that were ameliorated by POSTN knockdown. In this study, we found that POSTN interacted with ILK, which is involved in the nuclear factor-κB (NF-κB) signaling pathway. These findings suggest that POSTN may be a key regulator of vascular remodeling by inducing endothelial dysfunction, a process essential for PAH, and could be a potential therapeutic target for PAH.
Materials and methods
Animals
All animal experiments were approved by the Sookmyung Women's University Institutional Animal Care and Use Committee. Male Sprague–Dawley rats were used in this study. For the SuHx rat model, rats were subcutaneously injected with Sugen5416 (20 mg/Kg body weight) (Sigma Aldrich, Burlington, MA, USA), subsequently exposed to hypoxia (10% oxygen) for three weeks and kept in room air for another two weeks. For the monocrotaline (MCT) rat model, the rats received a single subcutaneous injection of monocrotaline (60 mg/Kg body weight) (Sigma-Aldrich) and were kept in room air for three weeks. The lung tissue was removed and snap-frozen for further experiments.
Microarray datasets
Microarray datasets were obtained from the NCBI GEO dataset (GSE53408) (Zhao et al. Citation2014).
Cell culture and transfection
PAECs (Lonza, Basel, Switzerland) were cultured at 37 °C in a 5% CO2 incubator in endothelial cell growth medium-2 (Lonza), 1% penicillin–streptomycin (Welgene, Daegu, Republic of Korea), and MycoZap (Lonza). For the experimental treatments, PAECs were grown to 70–90% confluency. Lenti-HEK293X cells were cultured in Dulbecco’s modified Eagle’s medium (HyClone, USA) supplemented with 10% fetal bovine serum (Gibco, USA) and 1% penicillin–streptomycin (Welgene). Small interfering RNA (siRNA) (Stealth siRNA; Invitrogen, Grand Island, NY, USA) was transfected using Lipofectamine RNAi MAX (Invitrogen) or DharmaFECT4 according to the manufacturer’s instructions. Plasmid DNAs were transfected into cells using Lipofectamine 2000 (Invitrogen).
Reagents
PAECs were either pre-treated with 10 ng/mL transforming growth factor beta 2 (TGF-β2) (Peprotech, USA, #100-35B) and 1 ng/mL interleukin 1 beta (IL-1β) (R&D Systems, Minneapolis, MN, USA, 201-LB-005) or interleukin 13 (IL-13) (R&D, 213-ILB, 10 ng/mL) for designated time points. TGF-β2 was dissolved in distilled water containing 0.1% bovine serum albumin (BSA). IL-1β and IL-13 were dissolved in Phosphate-Buffered Saline (PBS) containing 0.1% BSA.
Real-time polymerase chain reaction
Total RNA was isolated using the miRNeasy RNA isolation kit (Qiagen, Hilden, Germany). For mRNA analysis, purified RNA was reverse transcribed using the qPCRBIO cDNA Synthesis Kit (PCR BIOSYSTEMS, London, UK). qRT-PCR was performed using qPCRBIO SyGreen Blue Mix Lo-ROX (PCR BIOSYSTEMS), according to the manufacturer’s instructions. Ribosomal 18S RNA was used as an internal control.
Co-immunoprecipitation and western blotting
The cells were lysed with RIPA buffer (Biosesang, Yong-in, Republic of Korea) containing a protease and phosphatase inhibitor cocktail (Roche Diagnostics, Risch-Rotkreuz, Switzerland). Centrifugation was performed at 13,000 rpm and 4 °C for 15 min. Protein concentrations were determined using the Pierce BCA Protein Assay kit (Thermo Fisher Scientific, Waltham, MA, USA). For secreted protein analysis, the medium was collected and centrifugated at 1800rpm at 4 °C for 5 min and then supernatant was harvest. Equal amounts of total protein were separated using sodium dodecyl sulfate-polyacrylamide gel electrophoresis and transferred onto polyvinyl difluoride membranes (Millipore, Burlington, MA, USA). Immunoblotting was performed using primary antibodies specific for FN (1:3000, Santa Cruz Biotechnology, Dallas, TX, USA), POSTN (1:4000, Abcam, Cambridge, UK), Matrix Metallopeptidase (MMP) 2 (1:2000, Cell Signaling Technology, Danvers, MA, USA), and GAPDH (1:5000, Cell Signaling Technology). Immunodetection was performed using horseradish peroxidase-conjugated mouse (1:4000; Thermo Fisher Scientific) and rabbit (1:4000; Cell Signaling Technology) secondary antibodies. An enhanced chemiluminescence detection method (Thermo Fisher Scientific) was used.
Immunofluorescence
Rat lung tissues were fixed with 10% neutral buffered formalin (NBF) for 24 h, then embedded in paraffin. The paraffin blocks were sectioned at 5 μm and deparaffinized using Histoclear (Chayon, Seoul, Republic of Korea, HS-200). After rehydration, antigen retrieval was performed with Borg Decloaker RTU solution (Biocare Medical, Pacheco, CA, USA, BRR1000AG1) for 15 min in a high-pressure cooker and then blocked in blocking buffer (1% BSA, 5% goat serum, 1xPBS) for 1 h at room temperature. The sections were incubated with anti-POSTN (1:200, Abcam, ab14041), Von Willebrand Factor (vWF)-FITC (1:200, Abcam, ab8822) overnight at 4 °C. The following day, the sections were incubated with secondary antibody Alexa Fluor secondary rabbit 568 for 1 h at room temperature and counterstained with 4’,6-Diamidino-2-Phenylindole (DAPI) (Thermo Fisher Scientific) to visualize the nuclei. Imaging was performed using a Zeiss confocal microscope (ZEISS LSM-700, Carl Zeiss, Oberkochen, Germany).
Immunocytochemistry
PAECs were fixed with NBF for 5 min and washed with PBS. For permeabilization, cells were incubated with 0.1% Triton X-100 in PBS for 5 min. After blocking with 1% BSA in PBS for 1 h, the primary antibodies were applied overnight at 4 °C. FN (1:200; BD Biosciences, Franklin Lakes, NJ, USA), MMP2 (1:200; Cell Signaling Technology), and POSTN (1:300; Abcam) antibodies were used. The secondary antibodies used were goat anti-rabbit Alexa Fluor 488 and 568. Images were obtained using a Leica DMI8 microscope and a Zeiss confocal microscope.
Lentivirus production
Lentiviral open reading frame (ORF) clones of the human POSTN vector were purchased from OriGene technologies (Rockville, MD, USA). Lentiviral particles were produced by cotransfection with pMDLg/pRRE, pRSV-Rev, or pMD2.G. After 72 h of transfection, the supernatant was collected and concentrated using an Amicon centrifugal filter (Millipore; MWCO 10,000). Titrations were performed using a qPCR Lentivirus Titration kit (Abcam, LV900).
Migration assay
PAECs were plated in 12 well plate at a density of 2 × 105 cells/well. After infection or transfection with or without drug treatment, the cells were scratched using a P200 pipette tip. The cells were allowed to migrate, and the gap distance was captured using a camera-equipped microscope at the designated time points. Gap closure was calculated using ImageJ software.
Permeability assay
PAECs transfected with siRNA were seeded into a 0.2% gelatin pre-coated SPLInsert Hanging 24 well (SPL, Pyeongtaek, Republic of Korea) at a density of 1 × 105 cells per well. 500 μL medium was added into the lower chamber. The cells were treated with the drugs daily for 3 days. The permeability assay was performed using fluorescein isothiocyanate (FITC)-dextran (Sigma-Aldrich, FD40S). Serum free medium containing 1 mg/mL FITC-dextran was added to insert and 500 μL serum free media into lower chamber. After 10–30 min, the medium in the lower chamber was harvested and the fluorescence intensity was measured using a microplate reader (BioTek Instruments, Winooski, VT, USA) at 428/525 nm.
Statistical analysis
All experiments were performed at least three times, and analyses were performed using GraphPad Prism software (version 8.0). When two groups were compared, statistical differences were assessed using an unpaired two-tailed Student's t-test. Statistical significance was determined using a one-way analysis of variance, followed by Turkey’s multiple comparison test. Statistical significance was set at P value < 0.05.
Results
POSTN is upregulated in the lung tissue of patients with PAH and in PH animal models
First, we analyzed public GEO profiles (GSE53408) to identify the involvement of POSTN in PAH. Transcriptomic analysis showed that the lung tissues of patients with PAH had higher POSTN expression than the normal tissues ((a)) (Zhao et al. Citation2014). Next, we analyzed POSTN expression in the lung tissues of two well-known animal models of PAH, the SuHx and MCT injection models (Stenmark et al. Citation2009), and found significantly increased protein and mRNA expression ((b, c)). Since ECs play a key role in vascular homeostasis, and endothelial dysfunction contributes to the pathogenesis of PAH (Kim et al. Citation2013; Ranchoux et al. Citation2018; Yun et al. Citation2020), we co-stained POSTN with an EC marker (vWF) to investigate which vascular cells were the main origin of POSTN. We found that POSTN expression was significantly increased in the vascular ECs of the lung tissues of SuHx and MCT models ((d)). These results indicate that endothelial POSTN plays an important role in PAH pathogenesis.
Figure 1. Upregulation of POSTN in the lung tissue of patients with PAH and PAH animal models.: a, POSTN mRNA level in the lung tissue of patients with PAH from public GEO dataset (GSE53408). b, Protein and c, mRNA expression of POSTN in the lung tissue of SuHx and MCT rat models. d, Representative images of POSTN/vWF co-localization in the lung tissue of SuHx and MCT rat models. Lung sections were stained for POSTN (red), vWF (green) and DAPI (blue). Scale bar = 50 µm. * P < 0.05, ** P < 0.01, *** P < 0.001 determined by the unpaired two-tailed Student’s t-test. Error bars, S.E.M.
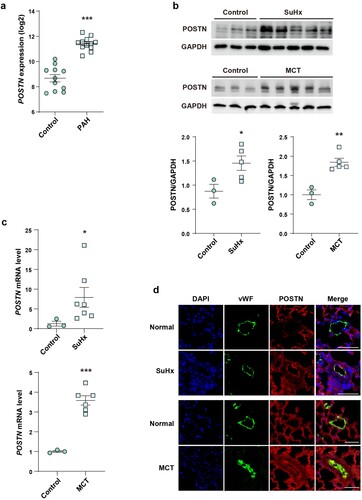
PAH-related stimuli increased POSTN expression in PAECs
We investigated whether POSTN expression was affected by various PAH-related stimuli in PAECs. Many studies have reported that various cytokines including IL-1β, IL-6, and IL-13 and growth factors such as TGF-β, fibroblast growth factor, and vascular endothelial growth factor are correlated with PAH (Soon et al. Citation2010; Hu et al. Citation2020; Guignabert and Humbert Citation2021; Monteiro et al. Citation2021; Rafikov et al. Citation2022). A previous study reported that co-treatment with TGF-β2 and IL-1β induces EndMT, an important pathological process that contributes to vascular remodeling in PAH (Maleszewska et al. Citation2013; Monteiro et al. Citation2021). PAECs stimulated with TGF-β2 (10 ng/mL) and IL-1β (1 ng/mL) exhibited elevated POSTN expression ((a, b)). In addition, IL-13 (10 ng/mL) upregulated POSTN expression ((c, d)). Mutated or reduced expression of the Bone Morphogenetic Protein Receptor Type 2 (BMPR2) is closely linked to PAH (Hopper et al. Citation2016). BMPR2 knockdown indeed upregulated POSTN expression (Supplementary figure 1(a)). The pro-inflammatory cytokine IL-6 slightly upregulated POSTN expression (Supplementary figure 1(b)). Hypoxia is a key process that leads to pulmonary vascular proliferation and remodeling in PAH by regulating vasoactive factors and inflammatory cytokines (Pugliese et al. Citation2015). Exposure to hypoxia increased the POSTN levels in PAECs (Supplementary figure 1(c)). Collectively, we confirmed that POSTN upregulation was significantly associated with endothelial abnormalities in PAH-related conditions.
Figure 2. POSTN expression is increased in lysates and conditioned medium in PAECs stimulated with PAH-related stimuli.: a, Protein expression and b, Immunostaining images of FN and MMP2 in PAECs stimulated with of TGF-β2 (10 ng/mL) and IL-1β (1 ng/mL) for 5 days. Scale bar = 20 µm. c, Protein expression and d, Immunostaining images of FN in PAECs stimulated with IL-13 (10 ng/mL) for 4 days. Scale bar = 20 µm. ** P < 0.01, *** P < 0.001 determined by the unpaired two-tailed Student’s t-test. Error bars represent S.E.M.
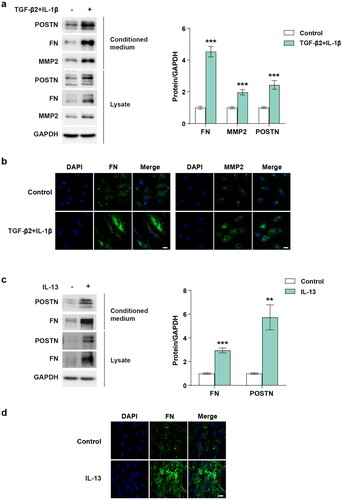
POSTN overexpression upregulated ECM proteins and induced endothelial abnormalities PAECs
Given that POSTN is upregulated by PAH-related stimuli in PAECs, we overexpressed POSTN in PAECs using lentiviruses or recombinant proteins to further investigate the role of POSTN. ECM deposition is the main characteristic of vascular remodeling that increases in the lung tissue of patients with PAH (Ambade et al. Citation2021; Liu et al. Citation2022). During ECM remodeling, proteases, including MMP, play a central role in ECM degradation (Ambade et al. Citation2021). Therefore, we speculated that POSTN regulated ECM deposition. We analyzed the correlation between POSTN and ECM proteins using lentivirus-transduced PAECs. POSTN overexpression upregulated FN expression in both the lysate and conditioned medium ((a)). In addition, we found that the secretion of MMP2 was increased by POSTN (Watanabe et al. Citation2012). Immunocytochemistry confirmed the elevated FN levels after POSTN overexpression ((b)). Collectively, ECM protein expression positively correlated with POSTN expression. To investigate the effects of POSTN on cell function, we performed migration assay and phalloidin staining of PAECs. Wound closure was significantly increased in PAECs overexpressing lentiviral POSTN compared with that in the control group ((c)). Actin cytoskeleton reorganization is related to EC permeability because actin plays an important role in determining endothelial barrier integrity (Prasain and Stevens Citation2009; Ling et al. Citation2022). To determine the changes in the actin cytoskeleton, we seeded PAECs on rhPOSTN-pre-coated glass. Phalloidin staining was performed 1 h after seeding, and stress fiber formation was increased in the rhPOSTN-coated group compared with that in the control group ((d)). These data demonstrated that POSTN induced altered phenotypes and functional abnormalities in PAECs associated with PAH pathogenesis.
Figure 3. POSTN induces ECM deposition and endothelial abnormalities in PAECs.: a, Protein expression of FN and MMP2 in response to infection with the lentiviral POSTN vector for 4 days. b, Immunostaining images of FN after POSTN overexpression. Scale bar = 50 µm. c, Migration assay after overexpression of the POSTN lentivirus for 3 days. Scale bar = 200 µm. d, Representative images of F-actin after POSTN overexpression in PAECs. Cells were plated onto glass coverslips coated with or without rhPOSTN (10 µg/mL) and then stained with rhodamine-phalloidin. Scale bar = 50 µm. * P < 0.05, ** P < 0.01, *** P < 0.001 determined by the unpaired two-tailed Student’s t-test. Error bars represent S.E.M.
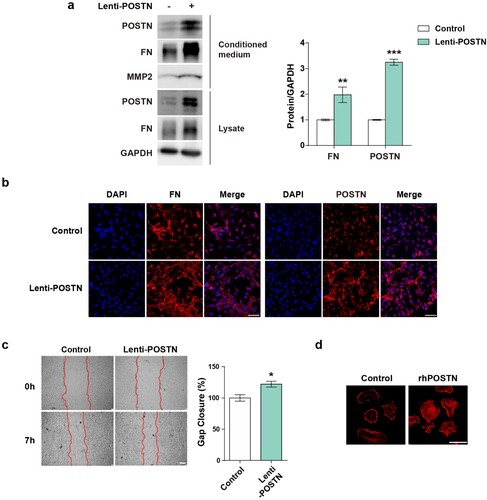
POSTN knockdown ameliorates ECM deposition and endothelial abnormalities
We further tested whether POSTN knockdown affected ECM deposition. We found the TGF-β2 and IL-1β induced MMP2 was abrogated by POSTN knockdown ((a)). To elucidate the effect of POSTN on endothelial function, we examined the ability of ECs to migrate. PAECs stimulated with TGF-β2 and IL-1β showed increased migration, which was alleviated by silencing POSTN ((b)). To further examine the endothelial permeability, we performed a vascular permeability assay using dextran. Co-treatment of TGF-β2 and IL-1β markedly increased permeability, which was reduced by POSTN knockdown ((c)). Similarly, POSTN knockdown reduced FN expression elevated by IL-13 and alleviated the migratory ability of PAECs (Supplementary Figure 2(a, b)). We investigated the effect of POSTN on hypoxia-exposed PAECs and found that FN deposition was reduced by POSTN knockdown (Supplementary Figure 3(a, b)). Finally, we examined the NF-κB activity in this context, which is a key transcription factor generally activated by PAH-related stimuli and found that p-p65 was elevated by co-treatment of TGF-β2 and IL-1β, which were restored by POSTN knockdown ((d)). These results indicate that targeting POSTN may be a potential therapeutic strategy for treating PAH by modulating endothelial function.
Figure 4. POSTN knockdown ameliorates ECM deposition and endothelial function.: a, Protein expression of MMP2 after TGF-β2 (10 ng/mL) and IL-1β (1 ng/mL) co-treatment for 5 days with or without POSTN knockdown (2 nM). b, Migration assay after TGF-β2 (10 ng/mL) and IL-1β (1 ng/mL) co-treatment with or without POSTN knockdown (10 nM). Scale bar = 200 µm. c, Permeability assay after TGF-β2 (10 ng/mL) and IL-1β (1 ng/mL) co-treatment with or without POSTN knockdown (5 nM). d, Protein expression of phosphorylated p65 by co-treatment of TGF-β2 (10 ng/mL) and IL-1β (1 ng/mL) for 24 h with or without POSTN siRNA (5 nM) transfection. P < 0.05, ** P < 0.01, *** P < 0.001, as determined by unpaired two-tailed Student’s t-test or one-way ANOVA with Bonferroni’s multiple comparison test. Error bars represent S.E.M.
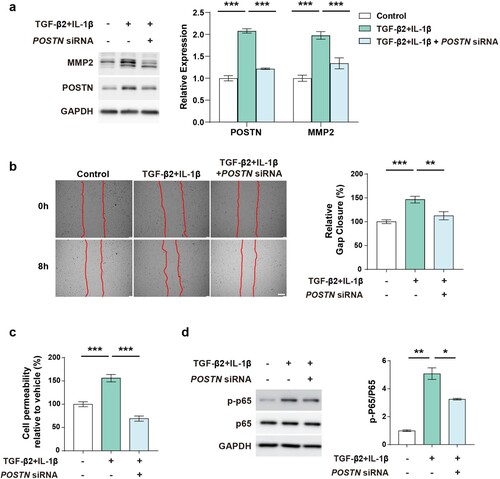
ILK binds to POSTN and regulates endothelial functions
Next, we investigated the mechanism by which POSTN regulates ECM remodeling in PAH. Recent studies have reported that POSTN binds to integrins and increases ILK, which activates various downstream pathways such as NF-κB and Akt/mTOR signaling in cancer cells (Ma et al. Citation2019; Jia YY et al. Citation2021; Wei et al. Citation2023). However, the molecular mechanisms underlying the expression of POSTN and ILK in ECs remain unclear. We hypothesized that ILK plays a key role in the regulation of endothelial function by binding to POSTN. As expected, the interaction between POSTN and ILK was confirmed by coimmunoprecipitation ((a)). As ILK expression was increased by co-treatment of TGF-β2 and IL-1β, we next investigated whether inhibition of ILK shows similar effects with POSTN knockdown. ILK knockdown decreased FN expression, which was upregulated by TGF-β2 and IL-1β, indicating reduced ECM accumulation ((b)). To further assess whether ILK knockdown could ameliorate endothelial function, we performed migration and permeability assays. ILK silencing reduced the cell migration induced by PAH-related stimuli ((c)). In addition, ILK knockdown significantly restored cell permeability impaired by co-treatment of TGF-β2 and IL-1β ((d)). These data suggest that targeting ILK could be an alternative strategy for reducing ECM deposition and attenuating endothelial function.
Figure 5. ILK interacts with POSTN and ILK inhibition reduces ECM deposition and attenuates endothelial function.: a, Interaction between ILK and POSTN in PAECs as determined by co-immunoprecipitation. b, Protein expression of ILK and FN after co-treatment of TGF-β2 (10 ng/ml) and IL-1β (1 ng/ml) for 3 days in conjugation with ILK siRNA (24 nM) transfection. c, Migration assay after co-treatment with TGF-β2 (10 ng/ml) and IL-1β (1 ng/ml) (upper) or IL-13 (10 ng/ml) (lower) with or without ILK knockdown (5 nM). Scale bar = 200 µm. d, Permeability assay after TGF-β2 (10 ng/ml) and IL-1β (1 ng/ml) co-treatment with or without ILK knockdown (5 nM). * P < 0.05, ** P < 0.01, *** P < 0.001 determined by unpaired two-tailed Student’s t test or one-way ANOVA with Bonferroni’s multiple comparison test. Error bars represent S.E.M.
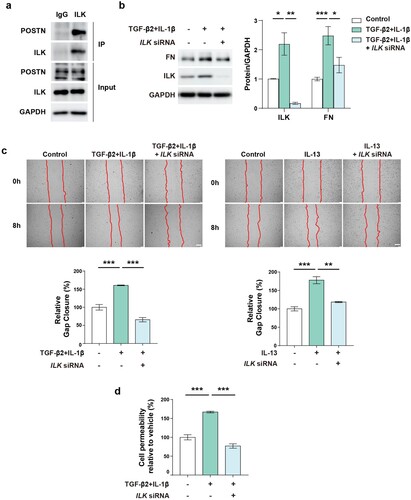
Discussion
PAH is a rare but fatal disease with a low survival rate due to right ventricular hypertrophy and right heart failure (Humbert et al. Citation2019; Yun et al. Citation2020). Currently, PAH treatments target three pathways: stimulating the nitric oxide–cyclic guanosine monophosphate biological pathway, increasing the effects of prostacyclin on receptors, and antagonizing the endothelin pathway (Ruopp and Cockrill Citation2022). These treatments induce vasodilation and lower pulmonary vascular resistance and blood pressure (Yun et al. Citation2020; Ruopp and Cockrill Citation2022). However, there is no effective cure for this condition. The main features of PAH are ECM deposition and vascular remodeling, accompanied by neointima formation and abnormal hyperproliferation of vascular cells such as ECs and SMCs (Cho et al. Citation2018; Thenappan et al. Citation2018; Humbert et al. Citation2019). Interestingly, it has been proposed that endothelial injury leads to endothelial abnormalities and EndMT, initiating ECM remodeling in the early stages of PAH pathogenesis (Thenappan et al. Citation2018), suggesting that ECM deposition plays a critical role in the progression of PAH and targeting its process can be a promising therapeutic strategy.
POSTN has been shown to be associated with fibrotic diseases such as asthma (Jia G et al. Citation2012), skin sclerosis (Yamaguchi et al. Citation2013), renal disease (Raman et al. Citation2017), cardiac fibrosis (Oka et al. Citation2007), pulmonary fibrosis (Naik et al. Citation2012), and PH (Abdul-Salam et al. Citation2010; Kumar et al. Citation2015; Nie X et al. Citation2020; Wang J et al. Citation2022; Yoshida et al. Citation2022). Previous studies reported that POSTN is differentially expressed in the lung tissues of rats with hypoxia-induced PH and in patients with PAH (Abdul-Salam et al. Citation2010; Wang J et al. Citation2022). In addition, it has been demonstrated that serum POSTN was elevated in the serum of patients with PH (Yoshida et al. Citation2022). Despite the importance of ECs on pathogenesis of PAH, studies on the role of POSTN in PAECs are barely researched. Nie et al. performed cytokine antibody array analysis and revealed that POSTN is the most increased protein in the pulmonary arteries of patients with PH (Nie X et al. Citation2020). Here, we showed that POSTN levels were significantly increased, especially in the endothelium, among vascular cells, as well as in the lung tissue of SuHx- and MCT-induced PH rat lungs.
Many studies have shown that imbalance between TGF-β and BMP signaling is a major player in PAH (Rol et al. Citation2018). Cytokine-mediated inflammation is an important contributor to the pathogenesis of PAH (Groth et al. Citation2014).. According to the previous studies, various cytokines including IL-1β, IL-2, IL-4, IL-6, and IL-13 are elevated in the blood of patients with PAH (Soon et al. Citation2010; Rafikov et al. Citation2022). TGF-β family and cytokines are well known to contribute to endothelial dysfunction and EndMT (Cho et al. Citation2018; Yun et al. Citation2020; Unenkhuu et al. Citation2021). TGF-β2 increases POSTN secretion in human pulmonary microvascular ECs (PMVECs) (Yoshida et al. Citation2022). IL-1β induces cytoskeletal reorganization and alteration of EC morphology in human dermal microvascular ECs (Romero et al. Citation1997). Several studies have reported that co-treatment of TGF-β2 and IL-1β synergistically induces EndMT in various ECs including PAECs, human umbilical vein ECs, and esophageal microvascular ECs (Maleszewska et al. Citation2013; Nie L et al. Citation2014; Monteiro et al. Citation2021). Expression of IL-13 and its target POSTN was increased in the lung tissues of patients with Schistosoma-PAH and Schistosoma-exposed mice (Kumar et al. Citation2015). Reportedly, IL-13 treatment induces EndMT by downregulating miR-424/503 (Takagi et al. Citation2018). We demonstrated that POSTN expression is upregulated in PAECs stimulated with IL-13 alone or co-treatment with TGF-β2 and IL-1β. We also investigated POSTN expression under various PAH-related conditions, such as BMPR2 silencing, IL-6, and 1% O2 hypoxia. Since BMPR2 mutations and reduced expression are closely related to PAH, BMPR2 dysfunction is considered an initiating factor for PAH (Evans et al. Citation2016; Hiepen et al. Citation2019). Furthermore, impairment of BMPR2 induces endothelial dysfunction and EndMT, leading to PH (Teichert-Kuliszewska et al. Citation2006; Ranchoux et al. Citation2015; Hopper et al. Citation2016). In our study, POSTN was significantly increased in response to BMPR2 knockdown, unlike a previous study that reported no expression changes in POSTN by silencing BMPR2 (Nie X et al. Citation2020). Chronic hypoxia is thought to be a major stimulus for PH development and vascular remodeling of the pulmonary arteries, and directly affects ECs, affecting permeability, coagulation, inflammation, and other processes (Stenmark et al. Citation2006). IL-6 is considered a key cytokine in PAH and is mainly regulated through JAK/STAT signaling (Steiner et al. Citation2009; Soon et al. Citation2010; Groth et al. Citation2014). In this study, we demonstrated that exposure to both 1% O2 and IL-6 increased POSTN expression. Although previous studies on the role of POSTN focused on human pulmonary artery smooth muscle cells (PASMCs) in PH context so far (Li et al. Citation2004; Li et al. Citation2007; Wang XD et al. Citation2016), recent studies have attempted to identify its role in both PASMCs and pulmonary ECs, including PAECs and PMVECs (Nie X et al. Citation2020; Yoshida et al. Citation2022). Our group is the first to discover that consistent upregulation of POSTN occurs in PAECs stimulated by several pathogenic factors involved in PAH. Collectively, our findings indicate that POSTN plays an important role in PAH development.
POSTN is known to interact with the ECM and accessory proteins such as collagen, TNC, laminin, CCN3, BMP-1, and FN (Kudo and Kii Citation2018). Reportedly, POSTN overexpression promotes secretion and accumulation of FN in the endoplasmic reticulum in fibroblasts (Kii et al. Citation2016; Nikoloudaki et al. Citation2020). MMP2 and MMP9 are involved in POSTN-mediated TGF-β activation that leads to collagen expression in epithelial cells (Sidhu et al. Citation2010). Moreover, POSTN promotes MMP2 expression in human periodontal ligament cells under hypoxic conditions (Watanabe et al. Citation2012). Although POSTN is closely related to ECM remodeling, its effect on ECs has not been thoroughly investigated. Here, we showed, for the first time, that POSTN increased MMP and FN synthesis in PAECs. ECM deposition was also confirmed in the lung tissues of the SuHx model. Although our results indicate that POSTN expression in PAECs contributes to ECM deposition during PAH pathogenesis, studies on EC-specific POSTN overexpression or deletion are required to demonstrate the EC-derived POSTN in animal models.
PAH is characterized by vascular remodeling, including EC proliferation, migration, and EndMT; thus, endothelial dysfunction is considered a trigger for PAH (Xue et al. Citation2020; Yun et al. Citation2020; Kurakula et al. Citation2021). Proproliferative and antiapoptotic ECs have been found in the distal pulmonary arteries of patients with idiopathic PAH (Tu et al. Citation2011). EndMT-induced ECs show increased migration ability (Ranchoux et al. Citation2015). Our data showing that POSTN increases the migration of PAECs is in line with the results of a previous study (Nie X et al. Citation2020). We further demonstrated that POSTN was involved in the permeability of PAECs. As POSTN knockdown reduced ECM deposition and ameliorated migration ability, EC-derived POSTN caused endothelial abnormalities and induced tissue remodeling.
Although several studies have reported that POSTN increases the mRNA and protein levels of ILK in cancer (Zheng et al. Citation2016; Ma et al. Citation2019; Jia YY et al. Citation2021) and autosomal dominant polycystic kidney disease (ADPKD) cells (Raman et al. Citation2018), the relationship between POSTN and ILK in ECs has not been explored. Here, we show that ILK expression was increased by co-treatment of TGF-β2 and IL-1β, moreover, ILK interacts with POSTN. Given that ILK silencing ameliorates endothelial function in PAECs stimulated by PAH-related stimuli, the POSTN-ILK complex may play an important role in POSTN-mediated abnormal endothelial function.
ILK acts as a scaffold for ECM proteins, receptors, integrins, and actin cytoskeleton (Wu and Dedhar Citation2001). The interaction between ILK and the actin cytoskeleton regulates the physical strength of the connections and signal transduction, leading to morphological changes (Wu and Dedhar Citation2001). Cytoskeletal changes in ECs cause abnormal endothelial functions such as increased permeability (Kayyali et al. Citation2002). Reportedly, POSTN induces stress fiber formation in ADPKD cells and PAECs (Raman et al. Citation2018; Nie X et al. Citation2020). Hiepen et al. reported that BMPR2-deficient ECs show increased integrin β1-ILK complexes at the cell junction, which associate with increased F-actin and accumulation of ECM protein, Fibrillin 1 with FN remodeling (Hiepen et al. Citation2019). These studies may provide clues to our data that PAECs seeded on rhPOSTN-coated glasses show increased F-actin expression and increased permeability after exposure to PAH-related stimuli.
ILK activates NF-κB signaling by phosphorylating p65 at serine 536 (Wani et al. Citation2011; Ma et al. Citation2019; Wei et al. Citation2023). Recent studies showed that POSTN promotes the expression of p-p65 and ILK (Ma et al. Citation2019; Wei et al. Citation2023). Activated NF-κB signaling was abrogated by integrin or ILK inhibitors (Ma et al. Citation2019; Wei et al. Citation2023). In addition, POSTN knockdown reduced p-p65 levels in chondrocytes (Duan et al. Citation2021). Here, we showed that POSTN knockdown decreased NF-κB activation in PAECs stimulated with PAH-related stimuli. These data indicate that NF-κB signaling was involved in POSTN-mediated ECM deposition and abnormal endothelial function. However, further research to elucidate inhibitory effect of ILK in POSTN-mediated NF-κB signaling in PAECs are needed.
In summary, we demonstrated that POSTN expression was significantly increased in the lung tissues of PAH animal models and in PAH-related stimuli-treated PAECs. POSTN promotes ECM deposition and endothelial abnormalities through its association with ILK. Given that POSTN or ILK inhibition strategies improve endothelial function and ECM accumulation, we provide the insight that the POSTN-ILK association is a key regulator of vascular remodeling, an essential process in PAH, and can be a potential therapeutic target for PAH ().
Author contributions
Jongmin Kim and Aram Lee designed and supervised the study. Dawn Lee, Heeyoung Lee, Ha-neul Jo, and Aram Lee performed the experiments and analyzed the data. The manuscript was written by Dawn Lee, Heeyoung Lee, Aram Lee, and Jongmin Kim. All the authors contributed to the discussion. All the authors have read and approved the final version of the manuscript.
Ethics approval
All animal experiments were performed in accordance with the policies of the Institutional Animal Care and Use Committee of Sookmyung Women’s University (SMWU-IACUC-1809-021-06).
Disclosure statement
No potential conflict of interest was reported by the author(s).
Additional information
Funding
References
- Abdul-Salam VB, Wharton J, Cupitt J, Berryman M, Edwards RJ, Wilkins MR. 2010. Proteomic analysis of lung tissues from patients with pulmonary arterial hypertension. Circulation. 122(20):2058–2067. doi:10.1161/CIRCULATIONAHA.110.972745.
- Ambade AS, Hassoun PM, Damico RL. 2021. Basement membrane extracellular matrix proteins in pulmonary vascular and right ventricular remodeling in pulmonary hypertension. Am J Respir Cell Mol Biol. 65(3):245–258. doi:10.1165/rcmb.2021-0091TR.
- Chen T, Sun MR, Zhou Q, Guzman AM, Ramchandran R, Chen J, Fraidenburg DR, Ganesh B, Maienschein-Cline M, Obrietan K, et al. 2022. MicroRNA-212-5p, an anti-proliferative miRNA, attenuates hypoxia and sugen/hypoxia-induced pulmonary hypertension in rodents. Mol Ther Nucleic Acids. 29:204–216. doi:10.1016/j.omtn.2022.06.008.
- Cho JG, Lee A, Chang W, Lee MS, Kim J. 2018. Endothelial to mesenchymal transition represents a Key link in the interaction between inflammation and endothelial dysfunction. Front Immunol. 9:294. doi:10.3389/fimmu.2018.00294.
- Dorafshan S, Razmi M, Safaei S, Gentilin E, Madjd Z, Ghods R. 2022. Periostin: biology and function in cancer. Cancer Cell Int. 22(1):315. doi:10.1186/s12935-022-02714-8.
- Duan X, Cai L, Pham CTN, Abu-Amer Y, Pan H, Brophy RH, Wickline SA, Rai MF. 2021. Amelioration of posttraumatic osteoarthritis in mice using intraarticular silencing of periostin via nanoparticle-based small interfering RNA. Arthritis Rheu. 73(12):2249–2260. doi:10.1002/art.41794.
- Evans JD, Girerd B, Montani D, Wang XJ, Galie N, Austin ED, Elliott G, Asano K, Grunig E, Yan Y, et al. 2016. BMPR2 mutations and survival in pulmonary arterial hypertension: an individual participant data meta-analysis. Lancet Resp Med. 4(2):129–137. doi:10.1016/S2213-2600(15)00544-5.
- Groth A, Vrugt B, Brock M, Speich R, Ulrich S, Huber LC. 2014. Inflammatory cytokines in pulmonary hypertension. Respir Res. 15(1):47. doi:10.1186/1465-9921-15-47.
- Guignabert C, Humbert M. 2021. Targeting transforming growth factor-β receptors in pulmonary hypertension. Eur Respir J. 57(2)):2002341. doi:10.1183/13993003.02341-2020.
- Hannigan GE, Leung-Hagesteijn C, Fitz-Gibbon L, Coppolino MG, Radeva G, Filmus J, Bell JC, Dedhar S. 1996. Regulation of cell adhesion and anchorage-dependent growth by a new β1-integrin-linked protein kinase. Nature. 379(6560):91–96. doi:10.1038/379091a0.
- Hiepen C, Jatzlau J, Hildebrandt S, Kampfrath B, Goktas M, Murgai A, Cuellar Camacho JL, Haag R, Ruppert C, Sengle G, et al. 2019. BMPR2 acts as a gatekeeper to protect endothelial cells from increased TGFβ responses and altered cell mechanics. PLoS Biol. 17(12):e3000557. doi:10.1371/journal.pbio.3000557.
- Hopper RK, Moonen JR, Diebold I, Cao A, Rhodes CJ, Tojais NF, Hennigs JK, Gu M, Wang L, Rabinovitch M. 2016. In Pulmonary Arterial Hypertension, Reduced BMPR2 Promotes Endothelial-to-Mesenchymal Transition via HMGA1 and Its Target Slug. Circulation. 133(18):1783–1794.
- Hu Y, Chi L, Kuebler WM, Goldenberg NM. 2020. Perivascular inflammation in pulmonary arterial hypertension. Cells. 9(11). 2338.
- Humbert M, Guignabert C, Bonnet S, Dorfmuller P, Klinger JR, Nicolls MR, Olschewski AJ, Pullamsetti SS, Schermuly RT, Stenmark KR, et al. 2019. Pathology and pathobiology of pulmonary hypertension: state of the art and research perspectives. Eur Respir J. 53(1):1801887. doi:10.1183/13993003.01887-2018.
- Jia G, Erickson RW, Choy DF, Mosesova S, Wu LC, Solberg OD, Shikotra A, Carter R, Audusseau S, Hamid Q, et al. 2012. Periostin is a systemic biomarker of eosinophilic airway inflammation in asthmatic patients. J Allergy Clin Immunol. 130(3):647–654 e610.
- Jia YY, Yu Y, Li HJ. 2021. POSTN promotes proliferation and epithelial-mesenchymal transition in renal cell carcinoma through ILK/AKT/mTOR pathway. J Cancer. 12(14):4183–4195. doi:10.7150/jca.51253.
- Kayyali US, Pennella CM, Trujillo C, Villa O, Gaestel M, Hassoun PM. 2002. Cytoskeletal changes in hypoxic pulmonary endothelial cells are dependent on MAPK-activated protein kinase MK2. J Biol Chem. 277(45):42596–42602. doi:10.1074/jbc.M205863200.
- Kii I, Nishiyama T, Kudo A. 2016. Periostin promotes secretion of fibronectin from the endoplasmic reticulum. Biochem Biophys Res Commun. 470(4):888–893. doi:10.1016/j.bbrc.2016.01.139.
- Kim BR, Yoon JW, Choi H, Kim D, Kang S, Kim JH. 2022. Application of periostin peptide-decorated self-assembled protein cage nanoparticles for therapeutic angiogenesis. BMB Rep. 55(4):175–180. doi:10.5483/BMBRep.2022.55.4.137.
- Kim J, Kang Y, Kojima Y, Lighthouse JK, Hu X, Aldred MA, McLean DL, Park H, Comhair SA, Greif DM, et al. 2013. An endothelial apelin-FGF link mediated by miR-424 and miR-503 is disrupted in pulmonary arterial hypertension. Nat Med. 19(1):74–82. doi:10.1038/nm.3040.
- Kudo A, Kii I. 2018. Periostin function in communication with extracellular matrices. J Cell Commun Signal. 12(1):301–308. doi:10.1007/s12079-017-0422-6.
- Kudryashova TV, Goncharov DA, Pena A, Kelly N, Vanderpool R, Baust J, Kobir A, Shufesky W, Mora AL, Morelli AE, et al. 2016. HIPPO-Integrin-linked kinase cross-talk controls self-sustaining proliferation and survival in pulmonary hypertension. Am J Respir Crit Care Med. 194(7):866–877. doi:10.1164/rccm.201510-2003OC.
- Kumar R, Mickael C, Chabon J, Gebreab L, Rutebemberwa A, Garcia AR, Koyanagi DE, Sanders L, Gandjeva A, Kearns MT, et al. 2015. The causal role of IL-4 and IL-13 in schistosoma mansoni pulmonary hypertension. Am J Respir Crit Care Med. 192(8):998–1008. doi:10.1164/rccm.201410-1820OC.
- Kurakula K, Smolders V, Tura-Ceide O, Jukema JW, Quax PHA, Goumans MJ. 2021. Endothelial dysfunction in pulmonary hypertension: cause or consequence? Biomedicines. 9(1):57. doi:10.3390/biomedicines9010057.
- Lee A, McLean D, Choi J, Kang H, Chang W, Kim J. 2014. Therapeutic implications of microRNAs in pulmonary arterial hypertension. BMB Rep. 47(6):311–317. doi:10.5483/BMBRep.2014.47.6.085.
- Li P, Oparil S, Feng W, Chen YF. 2004. Hypoxia-responsive growth factors upregulate periostin and osteopontin expression via distinct signaling pathways in rat pulmonary arterial smooth muscle cells. J Appl Physiol (1985). 97(4):1550–1558; discussion 1549. doi:10.1152/japplphysiol.01311.2003.
- Li P, Oparil S, Novak L, Cao X, Shi W, Lucas J, Chen YF. 2007. ANP signaling inhibits TGF-β-induced Smad2 and Smad3 nuclear translocation and extracellular matrix expression in rat pulmonary arterial smooth muscle cells. J Appl Physiol (1985). 102(1):390–398. doi:10.1152/japplphysiol.00468.2006.
- Ling X, Peng S, Xu Y, Chu F. 2022. Beneficial effect of simvastatin on human umbilical vein endothelial cells gap junctions induced by TNF-α. Animal Cells Syst (Seoul). 26(1):10–18. doi:10.1080/19768354.2021.2023037.
- Liu S-F, Nambiar Veetil N, Li Q, Kucherenko MM, Knosalla C, Kuebler WM. 2022. Pulmonary hypertension: linking inflammation and pulmonary arterial stiffening. Front Immunol. 13:959209. doi:10.3389/fimmu.2022.959209.
- Ma Z, Zhao X, Deng M, Huang Z, Wang J, Wu Y, Cui D, Liu Y, Liu R, Ouyang G. 2019. Bone marrow mesenchymal stromal cell-derived periostin promotes B-ALL progression by modulating CCL2 in leukemia cells. Cell Rep. 26(6):1533–1543 e1534. doi:10.1016/j.celrep.2019.01.034.
- Maleszewska M, Moonen JR, Huijkman N, van de Sluis B, Krenning G, Harmsen MC. 2013. IL-1β and TGFβ2 synergistically induce endothelial to mesenchymal transition in an NFκB-dependent manner. Immunobiology. 218(4):443–454. doi:10.1016/j.imbio.2012.05.026.
- Monteiro JP, Rodor J, Caudrillier A, Scanlon JP, Spiroski AM, Dudnakova T, Pfluger-Muller B, Shmakova A, von Kriegsheim A, Deng L, et al. 2021. Mir503hg loss promotes endothelial-to-mesenchymal transition in vascular disease. Circ Res. 128(8):1173–1190. doi:10.1161/CIRCRESAHA.120.318124.
- Naik PK, Bozyk PD, Bentley JK, Popova AP, Birch CM, Wilke CA, Fry CD, White ES, Sisson TH, Tayob N, et al. 2012. Periostin promotes fibrosis and predicts progression in patients with idiopathic pulmonary fibrosis. Am J Physiol-Lung C. 303(12):L1046–L1056. doi:10.1152/ajplung.00139.2012.
- Nie L, Lyros O, Medda R, Jovanovic N, Schmidt JL, Otterson MF, Johnson CP, Behmaram B, Shaker R, Rafiee P. 2014. Endothelial-mesenchymal transition in normal human esophageal endothelial cells cocultured with esophageal adenocarcinoma cells: role of IL-1β and TGF-β2. Am J Physiol-Cell Physiol. 307(9):C859–C877. doi:10.1152/ajpcell.00081.2014.
- Nie X, Shen C, Tan J, Wu Z, Wang W, Chen Y, Dai Y, Yang X, Ye S, Chen J, et al. 2020. Periostin: A Potential Therapeutic Target For Pulmonary Hypertension?. Circ Res. 127(9):1138–1152. doi:10.1161/CIRCRESAHA.120.316943.
- Nikoloudaki G, Snider P, Simmons O, Conway SJ, Hamilton DW. 2020. Periostin and matrix stiffness combine to regulate myofibroblast differentiation and fibronectin synthesis during palatal healing. Matrix Biol. 94:31–56. doi:10.1016/j.matbio.2020.07.002.
- Oka T, Xu J, Kaiser RA, Melendez J, Hambleton M, Sargent MA, Lorts A, Brunskill EW, Dorn GW, Conway SJ, et al. 2007. Genetic manipulation of periostin expression reveals a role in cardiac hypertrophy and ventricular remodeling. Circ Res. 101(3):313–321. doi:10.1161/CIRCRESAHA.107.149047.
- Prasain N, Stevens T. 2009. The actin cytoskeleton in endothelial cell phenotypes. Microvasc Res. 77(1):53–63. doi:10.1016/j.mvr.2008.09.012.
- Pugliese SC, Poth JM, Fini MA, Olschewski A, El Kasmi KC, Stenmark KR. 2015. The role of inflammation in hypoxic pulmonary hypertension: from cellular mechanisms to clinical phenotypes. Am J Physiol-Lung C. 308(3):L229–L252. doi:10.1152/ajplung.00238.2014.
- Rafikov R, Rischard F, Vasilyev M, Varghese MV, Yuan JX, Desai AA, Garcia JGN, Rafikova O. 2022. Cytokine profiling in pulmonary arterial hypertension: the role of redox homeostasis and sex. Transl Res. 247:1–18. doi:10.1016/j.trsl.2022.03.013.
- Raman A, Parnell SC, Zhang Y, Reif GA, Dai Y, Khanna A, Daniel E, White C, Vivian JL, Wallace DP. 2018. Periostin overexpression in collecting ducts accelerates renal cyst growth and fibrosis in polycystic kidney disease. Am J Physiol-Renal. 315(6):F1695–F1707. doi:10.1152/ajprenal.00246.2018.
- Raman A, Reif GA, Dai Y, Khanna A, Li X, Astleford L, Parnell SC, Calvet JP, Wallace DP. 2017. Integrin-Linked kinase signaling promotes cyst growth and fibrosis in polycystic kidney disease. J Am Soc Nephrol. 28(9):2708–2719. doi:10.1681/ASN.2016111235.
- Ranchoux B, Antigny F, Rucker-Martin C, Hautefort A, Pechoux C, Bogaard HJ, Dorfmuller P, Remy S, Lecerf F, Plante S, et al. 2015. Endothelial-to-mesenchymal transition in pulmonary hypertension. Circulation. 131(11):1006–1018. doi:10.1161/CIRCULATIONAHA.114.008750.
- Ranchoux B, Harvey LD, Ayon RJ, Babicheva A, Bonnet S, Chan SY, Yuan JX, Perez VJ. 2018. Endothelial dysfunction in pulmonary arterial hypertension: an evolving landscape (2017 Grover Conference Series). Pulm Circ. 8(1):1–17. doi:10.1177/2045893217752912.
- Rol N, Kurakula KB, Happe C, Bogaard HJ, Goumans MJ. 2018. TGF-beta and BMPR2 signaling in PAH: two black sheep in one family. Int J Mol Sci. 19(9):2585.
- Romero LI, Zhang DN, Herron GS, Karasek MA. 1997. Interleukin-1 induces major phenotypic changes in human skin microvascular endothelial cells. J Cell Physiol. 173(1):84–92. doi:10.1002/(SICI)1097-4652(199710)173:1<84::AID-JCP10>3.0.CO;2-N.
- Ruopp NF, Cockrill BA. 2022. Diagnosis and treatment of pulmonary arterial hypertension. JAMA. 327(14):1379–1391. doi:10.1001/jama.2022.4402.
- Russomanno G, Jo KB, Abdul-Salam VB, Morgan C, Endruschat J, Schaeper U, Osman AH, Alzaydi MM, Wilkins MR, Wojciak-Stothard B. 2021. miR-150-PTPMT1-cardiolipin signaling in pulmonary arterial hypertension. Mol Ther - Nucleic Acids. 23:142–153. doi:10.1016/j.omtn.2020.10.042.
- Seki M, Furukawa N, Koitabashi N, Obokata M, Conway SJ, Arakawa H, Kurabayashi M. 2019. Periostin-expressing cell-specific transforming growth factor-β inhibition in pulmonary artery prevents pulmonary arterial hypertension. PLoS One. 14(8):e0220795. doi:10.1371/journal.pone.0220795.
- Shen Y, Goncharov DA, Avolio T, Ray A, Okorie E, DeLisser H, Mora AL, Vanderpool R, Kudryashova TV, Goncharova EA. 2020. Differential effects of integrin-linked kinase inhibitor Cpd22 on severe pulmonary hypertension in male and female rats. Pulm Circ. 10(1):1–12. doi:10.1177/2045894019898593.
- Sidhu SS, Yuan S, Innes AL, Kerr S, Woodruff PG, Hou L, Muller SJ, Fahy JV. 2010. Roles of epithelial cell-derived periostin in TGF-β activation, collagen production, and collagen gel elasticity in asthma. Proc Natl Acad Sci USA. 107(32):14170–14175. doi:10.1073/pnas.1009426107.
- Simonneau G, Montani D, Celermajer DS, Denton CP, Gatzoulis MA, Krowka M, Williams PG, Souza R. 2019. Haemodynamic definitions and updated clinical classification of pulmonary hypertension. Eur Respir J. 53(1):1801913. doi:10.1183/13993003.01913-2018.
- Snider P, Hinton RB, Moreno-Rodriguez RA, Wang J, Rogers R, Lindsley A, Li F, Ingram DA, Menick D, Field L, et al. 2008. Periostin is required for maturation and extracellular matrix stabilization of noncardiomyocyte lineages of the heart. Circ Res. 102(7):752–760. doi:10.1161/CIRCRESAHA.107.159517.
- Soon E, Holmes AM, Treacy CM, Doughty NJ, Southgate L, Machado RD, Trembath RC, Jennings S, Barker L, Nicklin P, et al. 2010. Elevated levels of inflammatory cytokines predict survival in idiopathic and familial pulmonary arterial hypertension. Circulation. 122(9):920–927. doi:10.1161/CIRCULATIONAHA.109.933762.
- Steiner MK, Syrkina OL, Kolliputi N, Mark EJ, Hales CA, Waxman AB. 2009. Interleukin-6 overexpression induces pulmonary hypertension. Circ Res. 104(2):236–244, 236p following 244. doi:10.1161/CIRCRESAHA.108.182014.
- Stenmark KR, Fagan KA, Frid MG. 2006. Hypoxia-Induced pulmonary vascular remodeling. Circ Res. 99(7):675–691. doi:10.1161/01.RES.0000243584.45145.3f.
- Stenmark KR, Meyrick B, Galie N, Mooi WJ, McMurtry IF. 2009. Animal models of pulmonary arterial hypertension: the hope for etiological discovery and pharmacological cure. Am J Physiol-Lung C. 297(6):L1013–L1032. doi:10.1152/ajplung.00217.2009.
- Takagi K, Yamakuchi M, Matsuyama T, Kondo K, Uchida A, Misono S, Hashiguchi T, Inoue H. 2018. IL-13 enhances mesenchymal transition of pulmonary artery endothelial cells via down-regulation of miR-424/503 in vitro. Cell Signal. 42:270–280. doi:10.1016/j.cellsig.2017.10.019.
- Teichert-Kuliszewska K, Kutryk MJ, Kuliszewski MA, Karoubi G, Courtman DW, Zucco L, Granton J, Stewart DJ. 2006. Bone morphogenetic protein receptor-2 signaling promotes pulmonary arterial endothelial cell survival: implications for loss-of-function mutations in the pathogenesis of pulmonary hypertension. Circ Res. 98(2):209–217. doi:10.1161/01.RES.0000200180.01710.e6.
- Thenappan T, Chan SY, Weir EK. 2018. Role of extracellular matrix in the pathogenesis of pulmonary arterial hypertension. Am J Physiol-Heart C. 315(5):H1322–H1331. doi:10.1152/ajpheart.00136.2018.
- Tu L, Dewachter L, Gore B, Fadel E, Dartevelle P, Simonneau G, Humbert M, Eddahibi S, Guignabert C. 2011. Autocrine fibroblast growth factor-2 signaling contributes to altered endothelial phenotype in pulmonary hypertension. Am J Respir Cell Mol Biol. 45(2):311–322. doi:10.1165/rcmb.2010-0317OC.
- Unenkhuu B, Kim DB, Kim HS. 2021. MKP-3 suppresses LPS-induced inflammatory responses in HUVECs via inhibition of p38 MAPK/NF-κB pathway. Animal Cells Syst (Seoul). 25(4):235–244. doi:10.1080/19768354.2021.1954551.
- Wang J, Niu Y, Luo L, Lu Z, Chen Q, Zhang S, Guo Q, Li L, Gou D. 2022. Decoding ceRNA regulatory network in the pulmonary artery of hypoxia-induced pulmonary hypertension (HPH) rat model. Cell Biosci. 12(1):27. doi:10.1186/s13578-022-00762-1.
- Wang XD, Li F, Ma DB, Deng X, Zhang H, Gao J, Hao L, Liu DD, Wang J. 2016. Periostin mediates cigarette smoke extract-induced proliferation and migration in pulmonary arterial smooth muscle cells. Biomed Pharmacother. 83:514–520. doi:10.1016/j.biopha.2016.07.007.
- Wani AA, Jafarnejad SM, Zhou J, Li G. 2011. Integrin-linked kinase regulates melanoma angiogenesis by activating NF-κB/interleukin-6 signaling pathway. Oncogene. 30(24):2778–2788. doi:10.1038/onc.2010.644.
- Watanabe T, Yasue A, Fujihara S, Tanaka E. 2012. PERIOSTIN regulates MMP-2 expression via the αvβ3 integrin/ERK pathway in human periodontal ligament cells. Arch Oral Biol. 57(1):52–59. doi:10.1016/j.archoralbio.2011.07.010.
- Wei T, Wang K, Liu S, Fang Y, Hong Z, Liu Y, Zhang H, Yang C, Ouyang G, Wu T. 2023. Periostin deficiency reduces PD-1+ tumor-associated macrophage infiltration and enhances anti-PD-1 efficacy in colorectal cancer. Cell Rep. 42(2):112090. doi:10.1016/j.celrep.2023.112090.
- Wu C, Dedhar S. 2001. Integrin-linked kinase (ILK) and its interactors: a new paradigm for the coupling of extracellular matrix to actin cytoskeleton and signaling complexes. J Cell Biol. 155(4):505–510. doi:10.1083/jcb.200108077.
- Xue C, Senchanthisai S, Sowden M, Pang J, White RJ, Berk BC. 2020. Endothelial-to-Mesenchymal transition and inflammation play Key roles in cyclophilin A-induced pulmonary arterial hypertension. Hypertension. 76(4):1113–1123. doi:10.1161/HYPERTENSIONAHA.119.14013.
- Yamaguchi Y, Ono J, Masuoka M, Ohta S, Izuhara K, Ikezawa Z, Aihara M, Takahashi K. 2013. Serum periostin levels are correlated with progressive skin sclerosis in patients with systemic sclerosis. Br J Dermatol. 168(4):717–725. doi:10.1111/bjd.12117.
- Yeo Y, Jeong H, Kim M, Choi Y, Kim KL, Suh W. 2022. Crosstalk between BMP signaling and KCNK3 in phenotypic switching of pulmonary vascular smooth muscle cells. BMB Rep. 55(11):565–570. doi:10.5483/BMBRep.2022.55.11.098.
- Yoshida T, Nagaoka T, Nagata Y, Suzuki Y, Tsutsumi T, Kuriyama S, Watanabe J, Togo S, Takahashi F, Matsushita M, et al. 2022. Periostin-related progression of different types of experimental pulmonary hypertension: a role for M2 macrophage and FGF-2 signalling. Respirology. 27(7):529–538. doi:10.1111/resp.14249.
- Yun E, Kook Y, Yoo KH, Kim KI, Lee MS, Kim J, Lee A. 2020. Endothelial to mesenchymal transition in pulmonary vascular diseases. Biomedicines. 8(12).
- Yun E, Kwon BS, Kim J, Lee A. 2023. Ginsenoside Rg3 attenuates pulmonary fibrosis by inhibiting endothelial to mesenchymal transition. Animal Cells Syst (Seoul). 27(1):159–170. doi:10.1080/19768354.2023.2244549.
- Zhao Y, Peng J, Lu C, Hsin M, Mura M, Wu L, Chu L, Zamel R, Machuca T, Waddell T, et al. 2014. Metabolomic heterogeneity of pulmonary arterial hypertension. PLoS One. 9(2):e88727. doi:10.1371/journal.pone.0088727.
- Zheng QM, Lu JJ, Zhao J, Wei X, Wang L, Liu PS. 2016. Periostin facilitates the epithelial-mesenchymal transition of endometrial epithelial cells through ILK-Akt signaling pathway. Biomed Res Int. 2016:9842619.