ABSTRACT
Introduction: Nitrite has been found to protect liver graft from cold preservation injury. However, the cell signaling pathway involved in this protection remains unclear. Here, we attempt to clarify if the NOS pathway by using the NOS inhibitor, L-NAME (L-NG-Nitroarginine methyl ester).
Animals and methods: Rat livers were conserved for 24 h at 4°C in (IGL-1) solution enriched or not with nitrite at 50 nM. In a third group, rats were pretreated with 50 mg/kg of L-NAME before their liver procurement and preservation in IGL-1 supplemented with nitrite (50 nM) and L-NAME (1 mM). After 24 h of cold storage, rat livers were ex-vivo perfused at 37°C during 2 h. Control livers were perfused without cold storage.
Results: Nitrite effectively protected the rat liver grafts from the onset of cold I/R injury. L-NAME treatment did not abolish the beneficial effects of nitrite. Liver damage, protein oxidation and lipid peroxidation remained at low levels in both nitrite-treated groups when compared to IGL-1 group. Antioxidant enzyme activities and functional parameters were unchanged after NOS inhibition.
Conclusion: Despite NOS inhibition by L-NAME, nitrite can still provide hepatic protection during cold I/R preservation. This suggests that nitrite acts through a NOS-independent pathway.
Introduction
It is well established that an ischemia/reperfusion (I/R) injury, inherent to cold preservation, is the main cause of primary dysfunction and primary non-function of liver allograft [Citation1]. In fact, the steps of organ transplantation, from graft harvest to graft revascularization, contribute to enhance organ susceptibility to an I/R injury and further increase tissue vulnerability towards injuries. One of the aforementioned steps is organ preservation in which the goal is to maintain the functional, biochemical and morphological integrity of the graft. Various hypothermic preservation solutions have been formulated to maintain cell viability during ischemia and to allow their early functional resumption on reoxygenation. Although institut Georges Lopez (IGL-1) liquid extends graft viability, severe organ injury is quite common with prolonged cold storage, and graft dysfunction after transplantation still occurs at significant rates. So, improvement of the composition of preservation solution is fundamental to optimize the outcome of liver transplantation (LT).
Several studies have demonstrated that nitric oxide (NO) effectively protected grafts from the onset of an I/R injury [Citation2–Citation4]. NO is able to induce vasodilatation and so to improve graft revascularization [Citation4]. It could reduce platelet aggregation and leukocytes adhesion which limits inflammatory damage during I/R insult [Citation5,Citation6]. It regulates interleukins and others inflammatory mediators’ release [Citation7]. More recently, it has been reported that NO could modulate autophagy and apoptosis [Citation8]. In addition, we have demonstrated that the activation of the endothelial nitric oxide synthase (eNOS) and the production of NO by this enzyme increased liver graft preservation and improve liver function after reperfusion [Citation9–Citation11]. Previous reports have also shown that tissue damage during the early phase of reperfusion appears to be associated with decreased NO availability related to eNOS down-regulation [Citation12]. Taken these facts into account, several teams have sought to optimize the composition of the storage solutions by their supplementation with exogenous source of NO [Citation7,Citation13]. In this sense, the use of nitrite therapy as exogenous source of NO proved to be an useful tool to protect organ function and integrity from any I/R damage, such as those encountered in organ transplantation [Citation14].
In our recent report, we confirmed that enrichment of IGL-1 preservation solution with nitrite improved liver graft preservation [Citation15]. However, the pathway by which nitrite mediates its hepatocellular protection remains controversial. Some authors suggested that nitrite activity is dependent on NO production but independent from eNOS activity [Citation14,Citation16,Citation17], whereas others showed that NO production is dependent from eNOS activation [Citation3,Citation9]. Duranski et al. showed that nitrite induced a cardioprotective effect in eNOS-deficient mice submitted to warm I/R demonstrating thus that NO protection is independent from eNOS [Citation14].
The main purpose of the present study was to examine whether the NOS pathway is involved in the protective effect of nitrite against a cold I/R injury in isolated liver by using the NOS inhibitor, L-NAME (L-NG-Nitroarginine methyl ester).
Animals and methods
Animals
Male Sprague-Dawley rats weighting between 250 and 300 g were used. Animals were housed under periodic cycle of 12 h of light/dark and they had free access to water and rat food. This experimental protocol was performed according to European Union regulations for animal experiments (Directive 86/609 CEE) and after agreement of local ethical committee.
Liver extraction and ex vivo perfusion
Rats were anesthetized with an intraperitoneal injection of urethane (5%). Livers were harvested as described previously [Citation11]. Briefly, after cannulation of the common bile duct, livers were flushed, by cold preservative solution, through a catheter introduced into the aorta. After cooling, a second catheter was inserted into the portal vein to complete organ rinsing and the whole liver was excised and trimmed of surrounding tissues. Then, extracted livers were conserved in 50 mL of preservative solution in a hermetically closed container, for 24 h at 4°C.
After cold preservation, livers were maintained during 20 min at ambient temperature in order to mitigate the rewarming phase in liver graft transplantation. Next, livers were rinsed with 10 mL of Ringer’s lactate solution and immediately connected to ex-vivo perfusion circuit. Time point 0 min corresponds to the collection of rinse solution effluent which coincides with the connection of the portal catheter to the circuit. Livers were then perfused at 37°C via the portal vein in a closed and controlled pressure circuit. The flow was progressively increased over the first 15-min in order to stabilize the portal pressure at 12 mm Hg (Pression Monitor BP-1; Pression Instruments, Sarasota, FL). The flow was controlled by a peristaltic pump (Minipuls 3; Gilson, France).
After 120 min of normothermic perfusion, vena cava effluent and tissue specimens were collected for biochemical determinations.
Experimental groups
Rats were randomly divided into 4 experimental groups (n = 6 for each):
Control: After procurement, livers were ex-vivo perfused for 120 min as described above without prior cold storage.
IGL-1: Livers were preserved in IGL-1 storage solution for 24 h and then perfused ex-vivo at 37°C during 120 min.
IGL-1 + Nitrite: Same as IGL-1 group but IGL-1 preservation solution was supplemented with nitrite at 50 nM.
IGL-1 + Nitrite + NAME: 30 min before liver procurement, L-NAME (50 mg/kg) was injected through the vena cave [Citation4]. Then, livers were flushed and preserved in cold IGL-1 solution supplemented with 50 nM of nitrite and 1 mM of L-NAME [Citation4]. After cold preservation, livers were ex-vivo perfused at 37°C during 120 min.
Liver damage evaluation
In order to evaluate liver injury, we measured the aspartate aminotransferase (AST) and alanine aminotransferase (ALT) activities in the effluent of rinse solution (time corresponding to 0-min reperfusion) and in the effluent of perfusion solution (time corresponding to 120-min reperfusion). Transaminase activities were determined spectrophotometrically with commercial kit according to provider’s indications (Biomerieux, France).
Gamma-glutamyl transferase (GGT) and alkaline phosphatase (PAL) are associated with hepatocellular damage [Citation18,Citation19]. They were measured in liver effluents after 120-min reperfusion, by an UV spectrophotometer at 410 and 405 nm, respectively (Siemens, France).
Bile flow
Liver function was assessed by measuring bile production during 120-min reperfusion. Bile was collected through the cannulated common bile duct, and output was reported as μL/min/g of liver [Citation11,Citation20].
Vascular resistance
Vascular resistance is calculated according to the following formula: Vascular resistance = Portal pressure/portal flow, and expressed as mL/min/g of liver. Portal pressure was fixed at 12 mm Hg during the 120-min perfusion and portal flow was controlled by a peristaltic pump.
Malondialdehyde assay
Malondialdehyde (MDA) is an indicator of membrane’s lipid peroxidation induced by oxidative stress which generates oxygen species. MDA is measured in hepatic tissue by means of thiobarbituric acid test [Citation11,Citation20].
Sulfhydryl and carbonyl proteins assays
Sulfhydryl compounds (PSH) are scavengers of reactive oxygen species [Citation21,Citation22] whereas protein carbonyls are produced consecutively to lipid peroxidation but essentially to glyco and protein-oxydation after oxidative stress [Citation23]. PSH were assayed by Ellman’s test using DTNB (5,5’-Dithionitrobenzoic acid) and measured spectrophotometrically at 412 nm [Citation24,Citation25]. Carbonyl proteins were measured spectrophotometrically at 350 and 375 nm after derivatization in presence of 2,4-dinitrophenylhydrazine (DNPH) [Citation23,Citation26].
Antioxidant enzymes activities in the hepatic tissue
The total superoxide dismutase (Cu-Zn SOD and Mn SOD) activity was assessed by following the inhibition of pyrogallol oxidation according to the method of Marklund and Marklund [Citation27]. It was measured at 420 nm and expressed as U/mg protein (one unit of SOD was defined as the enzyme amount causing 50% inhibition of pyrogallol oxidation).
The catalase (CAT) activity was assessed by the method of Claiborne [Citation28], which consists of following the decomposition rate of H2O2 spectrophotometrically at 240 nm [Citation28]. It was expressed as µmol of decomposed H2O2/min/mg of protein.
We also appraised the activity of glutathione peroxidase (GPx) and the rate of glutathione (GSH) following Flohe and Gunzler reaction by measuring the reduction of the absorbance of GSH at 412 nm in presence of H2O2 [Citation29,Citation30]. GSH was expressed as µg/mg of protein and GPx activity was expressed as µmol GSH/min/mg of protein.
Statistical analysis
Results were expressed as mean values ± standard errors (SEM). Differences between groups were examined for statistical significance using Kruskal – Wallis non parametric test followed by Dunn’s multiple comparison test (Graph Pad Prism software, version 6 for Windows). Data was considered statistically significant as p-value <0.05.
Results
We conducted these experiments to explore whether NOS inhibition could influence the anti ischemic effect of nitrite. As shown in and , significant reduction of ALT and AST activities were observed in IGL-1 + Nitrite group both before and after normothermic ex vivo reperfusion as compared to IGL-1 group (p < 0.05, respectively). Regarding ALT, we observed 27.8 ± 2.5 vs 89.7 ± 2.7 U/L (p < 0.05) before reperfusion ( A). For AST, we found 44 ± 8 vs 221 ± 28 U/L (p < 0.05) at 0 min ()). After 120 min of reperfusion, we found 90.6 ± 5.5 vs 388.1 ± 51.6 U/L (p < 0.05) for ALT ()) and 157.6 ± 31.1 vs 323.8 ± 47.8 U/L (p < 0.05) for AST ()). Concerning GGT activity, we found 8.30 ± 0.11 vs 7.07 ± 0.14 U/L for IGL-1 and IGL-1 + Nitrite groups, respectively (p < 0.05, C). For PAL activity, we detected 14.5 ± 0.5 vs 11.5 ± 0.5 for IGL-1 and IGL-1 + Nitrite groups, respectively (p < 0.05, ()). About IGL-1 + Nitrite + L-NAME group, we noted for ALT: 30 ± 28 U/L and 109 ± 31 U/L at respectively 0 min and 120 min; for AST: 51 ± 33 U/L and 116 ± 23 U/L at respectively 0 min and 120 min; for GGTand PAL at 120 min: 7.35 ± 0.01 U/L and 12.01 ± 0.01 U/L, respectively. No statistical differences were found between IGL-1 + Nitrite and IGL-1 + Nitrite + L-Name groups for ALT and AST activities at both 0 min and 120 min of reperfusion.
Figure 1. Alanine aminotransferase (ALT) and aspartate aminotransferase (AST) activities in liver effluent obtained after 24 h of cold preservation (a, b). Livers’ rat (n = 6) were flushed and preserved in IGL-1 solution (4°C for 24 h) supplemented with 50 nM of nitrite or with 50 nM of nitrite + 1 mM of L-NAME (L-NG-Nitroarginine methyl ester). Sham: livers were flushed and perfused ex vivo without cold storage. Data are expressed as means ± SE (n = 6 for each group). a: p < 0.05 vs Sham; b: p < 0.05 vs IGL-1.
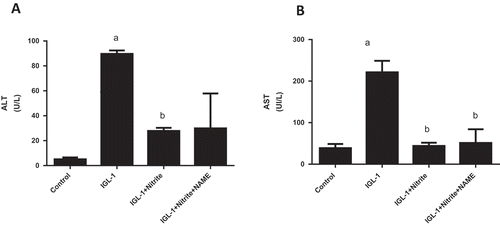
Figure 2. Alanine aminotransferase (ALT) and aspartate aminotransferase (AST) activities in liver effluent obtained after 24 h of cold preservation and 120 min of normothermic reperfusion (a, b), Gamma-glutamyl transferase (G-GT) (c), and alkaline phosphatase (PAL) (d) after of normothermic reperfusion. Livers’ rat (n = 6) were flushed and preserved in IGL-1 solution (4°C for 24 h) supplemented with 50 nM of nitrite or with 50 nM of nitrite + 1 mM of L-NAME (L-NG-Nitroarginine methyl ester). Sham: livers were flushed and perfused ex vivo without cold storage. Data are expressed as means ± SE (n = 6 for each group). a: p < 0.05 vs Sham; b: p < 0.05 vs IGL-1.
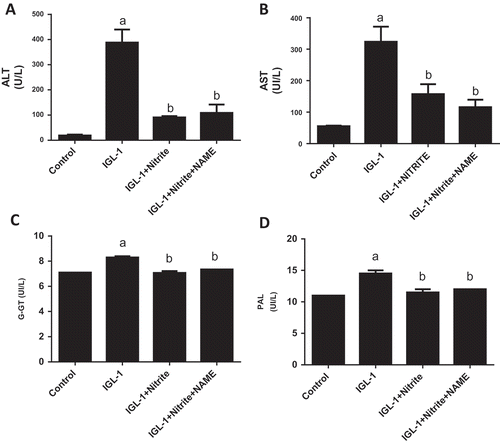
Nitrite was also found to protect hepatic function. This is evidenced by the improvement of bile flow (6.41 ± 0.07 vs 36.01 ± 7.22 µL/min/g for IGL-1 and IGL-1 + Nitrite groups respectively, p < 0.05) ()), as well as the attenuation of portal resistance (3.54 ± 0.18 vs 0.85 ± 0.11 mm Hg.min/mL/g for IGL-1 and IGL-1 + Nitrite groups respectively, p < 0.05) ()). The use of L-NAME didn’t induce significant changes in any of these parameters as compared to IGL-1 + nitrite group. Actually, we noted 46.04 ± 2.43 µL/min/g for bile flow and 1.08 ± 0.10 mm Hg.min/mL/g for portal resistance.
Figure 3. Bile output (a) and portal resistance (b), after 120 min of normothermic reperfusion. Livers’ rat (n = 6) were flushed and preserved in IGL-1 solution (4°C for 24 h) supplemented with 50 nM of nitrite or with 50 nM of nitrite + 1 mM of L-NAME (L-NG-Nitroarginine methyl ester). Sham: livers were flushed and perfused ex vivo without cold storage. Data are expressed as means ± SE (n = 6 for each group). a: p < 0.05 vs Sham; b: p < 0.05 vs IGL-1.
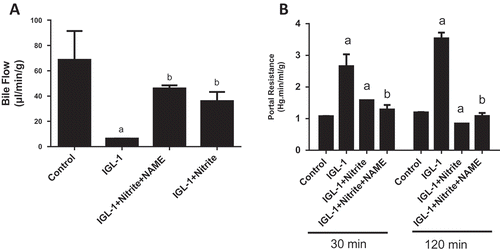
The next step of the study explored the relationship between oxidative stress, nitrite and NOS. We found that, comparing with IGL-1 group, nitrite supplementation markedly reduced lipid and protein oxidation as attested by lower levels of MDA (3.28 ± 0.32 vs 1.35 ± 0.28 nmol/mg protein, respectively, p < 0.05) ()) and of carbonyl proteins (0.36 ± 0.06 vs 0.17 ± 0.01 nmol/mg protein, respectively, p < 0.05) ()) on one hand, and a higher level of PSH (3.64 ± 0.42 vs 7.19 ± 0.17 μg/mg protein, respectively, p < 0.05) ()), on the other hand. In line with this, livers stored in IGL-1 + nitrite solution exhibited a marked rise of the activity of antioxidant enzymes catalase (221.05 ± 3.34 μmol H2O2/min/mg protein) ()), GPx (24.16 ± 1.10 μmol GSH/min/mg protein) ()), SOD (4.73 ± 0.42 U/mg protein) ()) and of GSH level as well (0.65 ± 0.07 μg/mg protein) ()) when referred to those conserved in IGL-1 (177 ± 3.4 μmol H2O2/min/mg protein, 5.44 ± 0.36 μmol GSH/min/mg protein, 3.53 ± 0.22 U/mg protein, 0,37 ± 0.07 μg/mg protein, respectively). Upon L-NAME association to nitrite, no statistical changes were found in the hepatic oxidative status as compared to nitrite alone. We noted 1.09 ± 0.05 nmol/mg protein for MDA, 0.06 ± 0.01 nmol/mg protein for carbonyl proteins, 8.24 ± 1.17 μg/mg protein for PSH, 236.09 ± 7.38 μmol H2O2/min/mg protein for CAT, 30.90 ± 3.67 μmol GSH/min/mg protein for GPx, 4.81 ± 0.47 U/mg protein for SOD and 0,57 ± 0.01 μg/mg protein for GSH.
Figure 4. Lipid peroxidation expressed as malondialdehyde (MDA) activity (a), carbonyl proteins (Prot Carb) (b), and sulfhydryl proteins (PSH) levels (c) in liver tissues after normothermic reperfusion. Livers’ rat (n = 6) were flushed and preserved in IGL-1 solution (4°C for 24 h) supplemented with 50 nM of nitrite or with 50 nM of nitrite + 1 mM of L-NAME (L-NG-Nitroarginine methyl ester). Sham: livers were flushed and perfused ex vivo without cold storage. Data are expressed as means ± SE (n = 6 for each group). a: p < 0.05 vs Sham; b: p < 0.05 vs IGL-1.
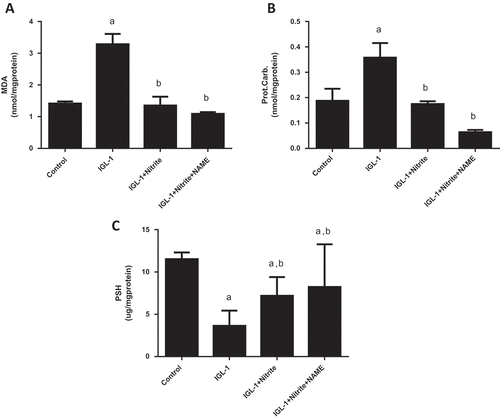
Figure 5. Evaluation of antioxidant enzymes catalase (CAT) (a), glutathione peroxidase (GPX) (b), superoxide dismutase (SOD) (c), reduced gluthatione (GSH) (d) activities. Livers’ rat (n = 6) were flushed and preserved in IGL-1 solution (4°C for 24 h) supplemented with 50 nM of nitrite or with 50 nM of nitrite + 1 mM of L-NAME (L-NG-Nitroarginine methyl ester). Sham: livers were flushed and perfused ex vivo without cold storage. Data are expressed as means ± SE (n = 6 for each group). a: p < 0.05 vs Sham; b: p < 0.05 vs IGL-1.
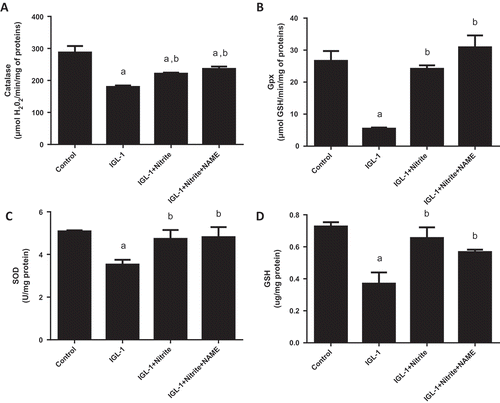
Discussion
An Ischemia-reperfusion injury associated to liver surgery remains until now an unresolved problem. Damages are caused by warm ischemia during graft procurement and implementation, cold ischemia during preservation and reperfusion [Citation4]. All of these steps are unavoidable during LT. Many groups are focusing of strategies reducing I/R injury in order to limit primary graft dysfunction or non-function [Citation31]. Otherwise, it is widely valued that I/R associated with LT induces endothelial insult characterized by marked trouble in NO homeostasis [Citation9,Citation16,Citation32]. The decrease in NO bioavailability appears early after reperfusion, and seems to be the consequence of the disruption of NO synthesis and the inactivation of NO by the overproduction of superoxide anion, or both. It is largely appreciated that eNOS activation induces liver protection [Citation33].
In the present study, we aimed to determine if the NOS pathway is involved in the protective effect of nitrite against cold I/R injury in isolated liver by using the NOS inhibitor, L-NAME. We found that nitrite supplementation to IGL-1 solution at 50 nM improves all biochemical, oxidative stress and functional parameters of the liver graft, and that L-NAME addition to nitrite enriched IGL-1 solution didn’t abolish the protective effect of nitrite whereas Name addition to IGL-1 solution without nitrite didn’t shown any significant results from IGL-1 group (results are not shown). These results suggest that nitrite could act through an independent NOS pathway. Our results are in accordance with those of Wei Li et al. demonstrating that the use of nitrite induced protection against cold I/R injury in liver graft [Citation34]. Also, they support Duranski et al. hypothesis [Citation14] stating that nitrite induces its protecting issues on liver and heart through a pathway independent from eNOS. This team has reported that nitrite remains cytoprotective even in eNOS-deficient mouse and the effect is dependent on NO production. Nevertheless, other authors found that nitrite did not protect against renal I/R injury [Citation35].
The strongest data supporting the thesis that nitrite could serve as an important source of endocrine NO appear from studies of I/R although the precise mechanism of how it induces tissue protection is still misleading. Endogenous NO is synthesized from L-arginine in an oxidation reaction, catalyzed by the NOS enzymes [Citation3]. This complex reaction requires the presence of co-substrates O2 and the reduced form of nicotinamide adenine dinucleotide phosphate, as well as many cofactors including flavin adenine dinucleotide, flavin mononucleotide and tetrahydrobiopterin . In vivo, nitrite is considered as a catabolism product of NO. Indeed, NO is inactivated primarily by superoxide anion, but other pathways could be implicated. The first one is related to the autoxidation of NO to nitrite and then to nitrate. Other pathways may be mediated by metal-catalyzed oxidation reactions. The copper-containing protein ceruloplasmin (P-Cu2+) has been found to quickly oxidize NO to nitrite in physiological conditions. Besides to the P-Cu2+-mediated reaction, ferrous deoxygenated hemoglobin rapidly converts NO to nitrate [Citation36,Citation37]. However during ischemic condition, some investigations have emphasized the ability for nitrite to serve as a biological precursor of NO in the vascular compartment. This allowed some studies to demonstrate that nitrite can protect or reverse tissue damage during ischemia, especially in settings characterized by loss of NO bioavailability such those encountered during cold preservation of organs. Nitrite is reduced to NO during hypoxia and acidosis by the enzymatic action of xanthine oxidoreductase (XOR) and by heme proteins such as deoxyhemoglobin, myoglobin, and tissue heme [Citation38–Citation40]. The L-NAME has been shown, in vitro and in vivo, to be potent inhibitor of NOS and the production of NO [Citation41]. Therefore, our results suggest that nitrite action would be mediated through these ischemia-activated pathways and not through NOS activation.
Taken together, our results show that nitrite may be an efficient additive to cold preservation solution to fill up the losses of NO and to correct NO disorders associated with organ storage. The mechanism of action of nitrite seems to be independent from NOS pathway.
Acknowledgments
The Tunisian Ministry of Higher Education and Scientific Research.
Disclosure statement
No potential conflict of interest was reported by the authors.
Additional information
Funding
References
- Deschenes M. Early allograft dysfunction: causes, recognition, and management. Liver Transplant. 2013;19(Suppl 2):1–8.
- Li W, Meng Z, Liu Y, et al. The hepatoprotective effect of sodium nitrite on cold ischemia-reperfusion injury. J Transplant. 2012;2012:635179.
- Ben Ben Abdennebi H, Zaouali MA, Alfany-Fernandez I, et al. How to protect liver graft with nitric oxide. World J Gastroenterol. 2011;17(24):2879–2889.
- Diao TJ, Chen X, Deng LH, et al. Protective effect of nitric oxide on hepatopulmonary syndrome from ischemia-reperfusion injury. World J Gastroenterol. 2012;18(25):3310–3316.
- Goto R, Issa F, Heidt S, et al. Ischemia-reperfusion injury accelerates human antibody-mediated transplant vasculopathy. Transplant J. 2013;96(2):139–145.
- Jadert C, Petersson J, Massena S, et al. Decreased leukocyte recruitment by inorganic nitrate and nitrite in microvascular inflammation and NSAID-induced intestinal injury. Free Radic Biol Med. 2012;52(3):683–692.
- Yang SL, Lou YJ. Sodium nitroprusside decreased leukotriene C4 generation by inhibiting leukotriene C4 synthase expression and activity in hepatic ischemia-reperfusion injured rats. Biochem Pharmacol. 2007;73(5):724–735.
- Loughran PA, Bagci EZ, Zamora R, et al. The role of nitric oxide in apoptosis and autophagy: biochemical and computational studies. In: Press A, editor. Nitric oxide: biology and pathobiology. 2nd ed. Elsevier; 2010. p. 513–537.
- Tabka D, Bejaoui M, Javellaud J, et al. Effects of Institut Georges Lopez-1 and Celsior preservation solutions on liver graft injury. World J Gastroenterol. 2015;21(14):4159–4168.
- Zaouali MA, Ben Ben Abdennebi H, Padrissa-Altés S, et al. How Institut Georges Lopez preservation solution protects nonsteatotic and steatotic livers against ischemia-reperfusion injury. Transplant Proc. 2011;43(1):77–79.
- Zaouali MA, Ben Ben Mosbah I, Padrissa-Altés S, et al. Relevance of epidermal growth factor to improve steatotic liver preservation in IGL-1 solution. Transplant Proc. 2010;42(8):3070–3075.
- Theruvath TP, Zhong Z, Currin RT, et al. Endothelial nitric oxide synthase protects transplanted mouse livers against storage/reperfusion injury: role of vasodilatory and innate immunity pathways. Transplant Proc. 2006;38(10):3351–3357.
- Quintana AB, Rodriguez JV, Scandizzi AL, et al. The benefit of adding sodium nitroprusside (NPNa) or S-nitrosoglutathion (GSNO) to the University of Wisconsin solution (UW) to prevent morphological alterations during cold preservation/reperfusion of rat livers. Ann Hepatol. 2003;2(2):84–91.
- Duranski MR, Greer JJM, Dejam A, et al. Cytoprotective effects of nitrite during in vivo ischemia-reperfusion of the heart and liver. J Clin Investig. 2005;115(5):1232–1240.
- Cherif-Sayadi A, Hadj-Ayed Hadj-Ayed Tka K, Bejaoui M, et al. Effects of nitrite addition to IGL-1 solution on rat liver preservation. Ann Transplant. 2016;21:602–610.
- Rassaf T, Ferdinandy P, Schulz R. Nitrite in organ protection. Br J Pharmacol. 2014;171(1):1–11.
- Wimalawansa SJ. Nitric oxide: new evidence for novel therapeutic indications. Expert Opin Pharmacother. 2008;9(11):1935–1954.
- Sepodes B, Maio R, Pinto R, et al. Recombinant human erythropoietin protects the liver from hepatic ischemia-reperfusion injury in the rat. Transpl Int. 2006;19(11):919–926.
- Li H, Sun -J-J, Chen G-Y, et al. Carnosic acid nanoparticles suppress liver ischemia/reperfusion injury by inhibition of ROS, caspases and NF-κB signaling pathway in mice. Biomed Pharmacother. 2016;82:237–246.
- Ben Ben Mosbah I, Rosello-Catafau J, Franco-Gou R, et al. Preservation of steatotic livers in IGL-1 solution. Liver Transplant. 2006;12(8):1215–1223.
- Inci I, Erne B, Arni S, et al. Prevention of primary graft dysfunction in lung transplantation by N-acetylcysteine after prolonged cold ischemia. J Heart Lung Transplant. 2010;29(11):1293–1301.
- Wang C, Chen K, Xia Y, et al. N-acetylcysteine attenuates ischemia-reperfusion-induced apoptosis and autophagy in mouse liver via regulation of the ROS/JNK/Bcl-2 pathway. PLoS One. 2014;9(9):e108855.
- Weber D, Davies MJ, Grune T. Determination of protein carbonyls in plasma, cell extracts, tissue homogenates, isolated proteins: focus on sample preparation and derivatization conditions. Redox Biol. 2015;5:367–380.
- Dingova D, Leroy J, Check A, et al. Optimal detection of cholinesterase activity in biological samples: modifications to the standard Ellman’s assay. Anal Biochem. 2014;462:67–75.
- Ishiguro K, Ando T, Watanabe O, et al. Specific reaction of α,β-unsaturated carbonyl compounds such as 6-shogaol with sulfhydryl groups in tubulin leading to microtubule damage. FEBS Lett. 2008;582(23–24):3531–3536.
- Levine RL, Williams JA, Stadtman ER, et al. Carbonyl assays for determination of oxidatively modified proteins. Methods Enzymol. 1994;233:346–357.
- Marklund S, Marklund G. Involvement of the superoxide anion radical in the autoxidation of pyrogallol and a convenient assay for superoxide dismutase. Eur J Biochem/FEBS. 1974;47(3):469–474.
- Chander V, Chopra K. Protective effect of nitric oxide pathway in resveratrol renal ischemia-reperfusion injury in rats. Arch Med Res. 2006;37(1):19–26.
- Flohe L, Gunzler WA. Assays of glutathione peroxidase. Methods Enzymol. 1984;105:114–121.
- Barros MA, Vasconcelos PR, Souza CM, et al. L-alanyl-glutamine attenuates oxidative stress in liver transplantation patients. Transplant Proc. 2015;47(8):2478–2482.
- He D, Guo Z, Pu J-L, et al. Resveratrol preconditioning protects hepatocytes against hepatic ischemia reperfusion injury via toll-like receptor 4/nuclear factor-κB signaling pathway in vitro and in vivo. Int Immunopharmacol. 2016;35:201–209.
- Shiva Shiva S. Nitrite: a physiological store of nitric oxide and modulator of mitochondrial function. Redox Biol. 2013;1(1):40–44.
- Ben Ben Mosbah I, Massip-Salcedo M, Fernandez-Monteiro I, et al. Addition of adenosine monophosphate-activated protein kinase activators to University of Wisconsin solution: a way of protecting rat steatotic livers. Liver Transplant. 2007;13(3):410–425.
- Guan LY, Fu PY, Li PD, et al. Mechanisms of hepatic ischemia-reperfusion injury and protective effects of nitric oxide. World J Gastrointest Surg. 2014;6(7):122–128.
- Basireddy M, Isbell TS, Teng X, et al. Effects of sodium nitrite on ischemia-reperfusion injury in the rat kidney. Am J Physiol Renal Physiol. 2006;290(4):F779–86.
- Lundberg JO, Weitzberg E, Gladwin MT. The nitrate-nitrite-nitric oxide pathway in physiology and therapeutics. Nat Rev Drug Discov. 2008;7(2):156–167.
- Lundberg JO, Weitzberg E. NO-synthase independent NO generation in mammals. Biochem Biophys Res Commun. 2010;396(1):39–45.
- Huang KT, Keszler A, Patel N, et al. The reaction between nitrite and deoxyhemoglobin. Reassessment of reaction kinetics and stoichiometry. J Biol Chem. 2005;280(35):31126–31131.
- Luchsinger BP, Rich EN, Gow AJ, et al. Routes to S-nitroso-hemoglobin formation with heme redox and preferential reactivity in the beta subunits. Proc Natl Acad Sci U S A. 2003;100(2):461–466.
- Tiravanti E, Samouilov A, Zweier JL. Nitrosyl-heme complexes are formed in the ischemic heart: evidence of nitrite-derived nitric oxide formation, storage, and signaling in post-ischemic tissues. J Biol Chem. 2004;279(12):11065–11073.
- Pfeiffer S, Leopold E, Schmidt K, et al. Inhibition of nitric oxide synthesis by NG-nitro-L-arginine methyl ester (L-NAME): requirement for bioactivation to the free acid, NG-nitro-L-arginine. Br J Pharmacol. 1996;118(6):1433–1440.