ABSTRACT
As a gastrointestinal malignancy, colorectal cancer (CRC) is a main cause of cancer-related deaths worldwide. Mex-3 RNA-binding family member A (MEX3A) is upregulated in multiple types of tumors and plays a critical role in tumor proliferation and metastasis. However, the function of MEX3A in CRC angiogenesis has not been fully understood. Hence, the aim of this study was to explore the role of MEX3A in CRC angiogenesis and investigate its underlying mechanisms. MEX3A expression in CRC was first investigated by bioinformatics means and then measured by qRT-PCR and Western blot. CCK-8 assay was employed to test cell viability. Angiogenesis assay was used to assess angiogenesis. The protein levels of VEGF, FGF and SDF-1 were evaluated using Western blot. The expression levels of MYC, HK2 and PGK1 were investigated by qRT-PCR. Extracellular acidification rate (ECAR) and oxygen consumption rate (OCR) were determined by Seahorse XP 96. The levels of pyruvate, lactate, citric acid and malate were measured by corresponding kits. Bioinformatics analysis demonstrated high MEX3A expression in CRC tissues and MEX3A enrichment in glycolysis and angiogenesis pathways. Cell assays showed high MEX3A expression in CRC cells and its promoting effects in CRC cell proliferation and glycolysis as well as angiogenesis. Rescue experiment confirmed that glycolysis inhibitor 2-DG could offset the promoting effects of MEX3A on the proliferation, angiogenesis and glycolysis of CRC cells. In conclusion, MEX3A could facilitate CRC angiogenesis by activating the glycolytic pathway, suggesting that MEX3A may be a novel therapeutic target for CRC.
1. Introduction
Colorectal cancer (CRC) is the third most common malignant tumor, with the mortality rate second only to lung cancer. Despite achievements in clinical practice and screening techniques, the diagnostic efficiency of CRC in most countries, including developed countries, remains lower than expected [Citation1]. Surgery, chemotherapy and radiotherapy were common methods for the treatment of colorectal adenocarcinoma in the past. However, due to difficulties in early diagnosis of CRC, most patients have been in the advanced stages of the disease when diagnosed with CRC, missing the best time for surgery and suffering more side effects of chemotherapy and radiotherapy [Citation2]. Thus, it is of great practical significance to develop new CRC treatment methods for improving the early diagnosis and survival rate of CRC patients. Tumor angiogenesis has been proved to play a critical role in tumorigenesis and tumor development, becoming a hot research topic in the field of cancer. The antiangiogenic drug bevacizumab has been approved for the first- or second-line treatment of metastatic CRC [Citation3]. As the first biological agent approved for treating metastatic CRC, bevacizumab has demonstrated impressive performance [Citation4]. Therefore, it is meaningful to explore the molecular mechanism of CRC angiogenesis in CRC treatment.
Mex3 RNA-binding family member A (MEX3A) was originally identified as a translational regulator in Caenorhabditis elegans that binds RNA [Citation5,Citation6]. MEX3A has been previously reported to act as oncogene in a variety of cancers, such as breast cancer [Citation6], CRC [Citation7] and osteosarcoma [Citation8]. For example, Liang et al. [Citation9] found that MEX3A promotes lung adenocarcinoma metastasis by down-regulating LAMA2 and then activating PI3K/AKT pathway. In the CRC study, Li et al. [Citation10] found that MEX3A benefits CRC tumorigenesis through RAP1/MAPK signaling. Zhou et al. [Citation7] revealed that MEX3A knockdown inhibits CRC tumorigenesis by up-regulating CDK2 expression. In conclusion, MEX3A is critical to CRC progression, but no studies have reported the role of MEX3A in CRC angiogenesis. Hence, this study explored the function of MEX3A in CRC angiogenesis and its mechanism.
In the present study, we investigated the expression of MEX3A and its biological functions in CRC. We also investigated the mechanism by which MEX3A affects angiogenesis in CRC. According to our results, MEX3A was significantly overexpressed in CRC and could promote CRC angiogenesis through activating the glycolytic pathway. In conclusion, our current findings provided a new perspective for enriching the pathogenesis of CRC and provided theoretical support for using MEX3A as a new therapeutic target for CRC.
1.1. Materials and methods
1.1.1. Bioinformatics analysis
The count data (51 normal samples and 647 cancer samples) of CRC (COAD and READ) were downloaded from The Cancer Genome Atlas (TCGA). Differential analysis was carried out on the TCGA mRNA data in the normal and tumor groups (|FC|>2, FDR<0.05) to obtain differentially expressed mRNAs (DEmRNAs) by using the ‘edgeR’ package. The mRNA expression was analyzed based on the clinical data, and the mRNA to be studied was identified by consulting literature. Gene set enrichment analysis (GSEA) was employed for gene pathway enrichment.
1.2. Cell culture
Normal human colon epithelial cells NCM-460, human umbilical vein endothelial cells (HUVECs), and human CRC cell lines (SW480, HCT116, HT-29) were provided by BeNa Culture Collection (China). Cells were kept in RPMI-1640 (Thermo Fisher Scientific, USA) in a constant temperature incubator with 5% CO2 at 37°C.
1.3. Cell transfection
si-MEX3A/oe-MEX3A and corresponding controls si-NC/oe- NC were purchased from Ribobio (China), while 2-DG and PBS were purchased from Sigma (USA). The plasmids described above were transfected into CRC cells by using the Lipofectamine 2000 kit (Thermo Fisher Scientific, USA) in accordance with the kit operating instructions. 24 h later, transfected cells were harvested for the next experiment.
1.4. Qrt-PCR
Total RNA isolation was performed using TRIzol reagent (Invitrogen, USA) and concentration determination of RNA was completed by a NanoDrop 2000 system (Thermo Fisher Scientific, USA). Total RNA was reversely transcribed using PrimeScriptTM RT Master Mix (Takara, Japan) as descripted in the kit instructions. qRT-PCR was run on an ABI QuantStudio 5 real-time PCR system (Thermo Fisher Scientific, USA) by applying the iQ SYBR Green Supermix kit (BIO-RAD, USA) to evaluate the expression of the genes listed in . β-actin was taken as a standardized endogenous control. The 2−ΔΔCt value was adopted to compare the relative expression of MEX3A between control group and experimental group. Primer sequences are shown in .
Table 1. Primer sequences for Qrt-PCR.
1.5. Western blot
Total proteins were extracted from cells using lysis buffer, separated by SDS-PAGE, blotted onto PVDF membrane, and blocked with 5% skim milk powder for 1 h. The membrane was probed with primary antibodies at 4°C overnight and secondary antibody for 2 h. Protein expression was measured using enhanced chemiluminescence (ECL) substrate kit and gel imaging system. Antibodies involved were: primary antibodies rabbit anti-MEX3A (Abcam, UK), VEGF (Biorbyt, UK), FGF (Abcam, UK) and SDF-1 (Abcam, UK), β-actin (Abcam, UK); secondary antibody goat anti-rabbit IgG (Abcam, UK).
1.6. Cell counting kit-8 (CCK-8) assay
Cell viability was tested using CCK-8 kit (MedChemExpress, USA). Transfected cells were collected and seeded into 96-well plates. After 0, 24, 48, 72 and 96 h of culture, 10 μL of CCK-8 solution was added, and then the cells were incubated for 2 h in an incubator. The absorbance was read at 450 nm by microplate reader. Three same biological experiments were repeated for each group.
1.7. Angiogenesis assay
Angiogenesis assay was utilized to test the angiogenic ability of HUVECs. HUVECs were inoculated into the wells of the 96-well plate (Corning, USA), which was coated with 50 μL of matrigel (Corning, USA). HUVECs were treated with the cell culture medium previously treating CRC cells. The cells were incubated for 24 h at 37°C, and capillary structure formation was observed and photographed under a light microscope.
1.8. Determination of extracellular acidification rate (ECAR) and oxygen consumption rate (OCR)
ECAR and OCR were determined using a Seahorse XP96 extracellular flux analyzer (Seahorse Bioscience, USA) according to the methods of Ling Li et al. [Citation11]. ECAR and OCR were obtained by the Seahorse XP glycolytic Stress Test Kit and the Seahorse XP Cellular Mitochondrial Stress Test Kit (Agilent Technologies, USA), respectively. The glycolytic level, glycolytic capacity, basal OCR and maximum OCR were calculated according to the test results.
1.9. Determination of glycolytic pathway activity in CRC cell lines
Glycolytic activity was measured using pyruvate assay kit (Solarbio, China), lactate assay kit (Solarbio, China), citric acid assay kit (Solarbio, China) and malate assay kit (Sigma, USA). The kits were used to measure pyruvate, lactate, citric acid and malate in HCT116 cells as per instructions. qRT-PCR was applied to measure the expression of key kinases (including MYC, HK2 and PGK1) involved in glycolysis regulation [Citation12].
1.10. Data analysis
All statistical analyses were completed with GraphPad Prism 6 Software (GraphPad Software, USA). All experiments were performed at least three times, and the results were expressed as mean ± standard deviation. Differences between groups were compared by t-test or one-way analysis of variance. P < 0.05 indicated significant difference and denoted by the figure of *.
2. Results
2.1. MEX3A expression is up-regulated in CRC
MEX3A has been previously proved to promote the progression of breast cancer and other cancers [Citation13,Citation14]. Bioinformatics analysis revealed that MEX3A expression was evidently higher in CRC tissues than in normal tissues (). Then, the correlation between MEX3A expression and clinicopathological information was analyzed by bioinformatics databases. The data analyses showed that MEX3A expression was higher in the group of Stage III+Stage IV than in the group of Stage I+Stage II () and showed a significant difference between M0 group and M1 group, with an increasing trend (). In combination with the literature, we took MEX3A as the research object and determined the mRNA expression level of MEX3A in normal colon cells NCM-460 and human CRC cell lines HCT116, SW480 and HT29 by qRT-PCR. As the results demonstrated, the mRNA expression level of MEX3A was up-regulated in CRC cells (). Among the CRC cell lines, HCT116 cell line with relatively high MEX3A expression was selected for further study. These results indicated that MEX3A was markedly overexpressed in CRC.
Figure 1. MEX3A expression is up-regulated in CRC.
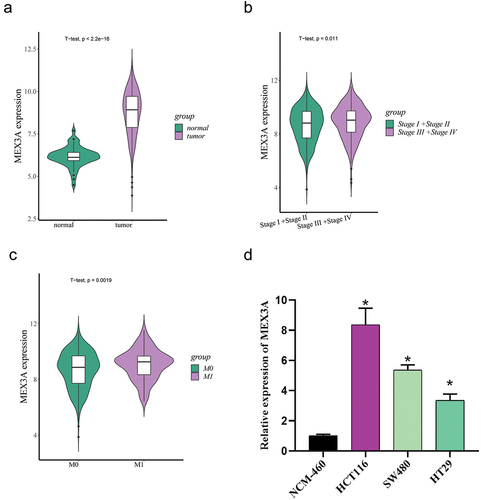
2.2. MEX3A promotes angiogenesis in CRC
We further investigated the mechanism of MEX3A affecting the development of CRC. We first identified MEX3A enrichment in the VEGF pathway using GSEA (), based on which we speculated that MEX3A might facilitate tumor angiogenesis in CRC. Hence, we constructed HCT116 cells with MEX3A knockdown (si-MEX3A). According to qRT-PCR and Western blot results, the mRNA and protein levels of MEX3A in HCT116 cells were remarkably reduced after MEX3A knockdown (). CCK-8 results showed the inhibitory effect of MEX3A knockdown on cell viability of HCT116, as compared with the control (). Afterwards, HUVECs were treated with HCT116 cell medium of the control group (si-NC) and si-MEX3A group, separately. In terms of angiogenesis assay, it demonstrated that knockdown of MEX3A reduced reticular structure length (), indicating that MEX3A knockdown could suppress the ability of angiogenesis. Western blot results demonstrated that the protein levels of angiogenic factors VEGF, FGF and SDF-1 were substantially reduced in the supernatant of HUVEC cells treated with HCT116 cell medium after MEX3A knockdown (). These results indicated that unusual high expression of MEX3A could significantly promote CRC angiogenesis.
Figure 2. MEX3A promotes angiogenesis in CRC.
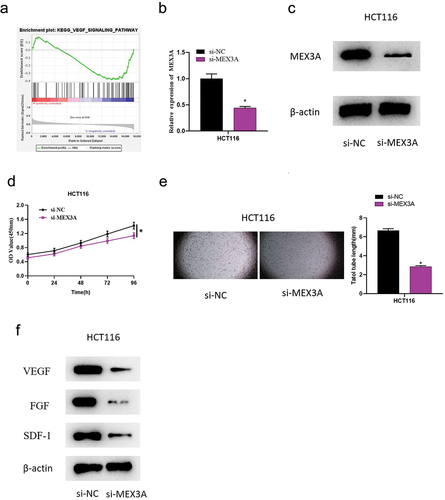
2.3. MEX3A promotes glycolysis in CRC cells
We next studied the potential mechanism of MEX3A promoting CRC angiogenesis. We performed GSEA, which revealed that MEX3A was involved in the glycolysis pathway (), suggesting that MEX3A could affect glycolysis in CRC cells. Therefore, qRT-PCR was employed to measure the expression levels of specific genes related to glycolytic metabolic pathway in cells, such as MYC, HK2, and PGK1, and the results showed that the mRNA expression levels of MYC, HK2 and PGK1 in HCT116 cells with MEX3A knockdown were significantly reduced compared with those with si-NC treatment (). ECAR and OCR of HCT116 cells treated with si-NC or si-MEX3A were analyzed by Seahorse XP 96. The results exhibited that ECAR was sharply reduced in MEX3A-knockdown HCT116 cells compared to the control, indicating the inhibitory effect of low MEX3A expression on glycolysis in CRC cells (). However, OCR was increased in HCT116 cells with MEX3A knockdown treatment, indicating that suppressed expression of MEX3A could significantly promote mitochondrial oxidative respiration (). The levels of pyruvate, lactate, citric acid and malate in si-NC or si-MEX3A treated HCT116 cells were determined by corresponding kits. As the results revealed, MEX3A knockdown suppressed the production of pyruvate, lactate, citric acid and malate in HCT116 cells compared with the control, indicating that MEX3A knockdown could lead to glycolytic process blockade in CRC cells (). In one word, abnormally high MEX3A level could evidently promote glycolysis in CRC cells.
Figure 3. MEX3A promotes glycolysis in CRC cells.

2.4. MEX3A promotes CRC angiogenesis via glycolytic pathway activation
With the aim of exploring the mechanism of MEX3A affecting CRC angiogenesis through glycolysis, we next constructed the following groups based on HCT116 cells: oe-NC+PBS, oe-MEX3A+PBS, and oe-MEX3A+2-DG (which down-regulates glycolysis using aerobic glycolysis inhibitor 2‑DG). As the qRT-PCR and Western blot analyses exhibited, the mRNA and protein expression levels of MEX3A were substantially enhanced in MEX3A overexpressing cells compared with controls, and 2-DG treatment had no significant effect on the expression of MEX3A (). CCK-8 assay indicated the promoting role of MEX3A in cell viability of HCT116, and further treatment with 2-DG could reverse the above-mentioned promoting effect (). Next, in order to test the effect of MEX3A overexpression on the angiogenic ability of CRC cells, HUVECs were treated with the cell mediums constructed above. The results of angiogenesis assay showed that MEX3A overexpression could substantially increase the length of blood vessels compared with control cells. Further treatment with 2-DG reversed the angiogenesis promoting effect of MEX3A overexpression on HCT116 cells (). The expression of angiogenic factors VEGF, FGF and SDF-1 in the HUVECs was measured by Western blot, which showed that MEX3A overexpression could facilitate the protein expression of VEGF, FGF and SDF-1, as compared to the control cells. Further addition of 2-DG reversed the promoting effect of MEX3A overexpression on angiogenic factor protein expression (). qRT-PCR was done to analyze the effect of MEX3A overexpression on the expression levels of specific genes related to glycolytic metabolic pathway, MYC, HK2 and PGK1. According to the results, MEX3A overexpression promoted the expression of MYC, HK2 and PGK1, and further addition of 2-DG reversed the promoting effect of MEX3A overexpression on specific genes related to glycolytic metabolic pathway (). Seahorse XP 96 was utilized to analyze ECAR and OCR of cells in the treatment groups. The results demonstrated that ECAR was evidently increased in HCT116 cells overexpressing MEX3A compared with the control group, indicating that MEX3A overexpression could significantly promote glycolysis of CRC cells. Further addition of 2-DG offset the promoting effect of MEX3A overexpression on ECAR (). While OCR was reduced in HCT116 cells with MEX3A overexpression, indicating that MEX3A overexpression could evidently inhibit mitochondrial oxidative respiration, and addition of 2-DG could restore the effect of MEX3A overexpression on OCR (). The levels of pyruvate, lactate, citric acid and malate in cells of the treatment group were evaluated by detection kits. The kits revealed that MEX3A overexpression facilitated the production of pyruvate, lactate, citric acid and malate in HCT116 cells, indicating that MEX3A overexpression could promote the glycolysis process of CRC. Addition of 2-DG offset the promoting effect of MEX3A overexpression on glycolytic progression in CRC cells (). Taken together, MEX3A promoted CRC angiogenesis by activating the glycolytic pathway.
Figure 4. MEX3A promotes CRC angiogenesis via glycolytic pathway activation.

3. Discussion
Blood vessels can provide oxygen and nutrients for the rapid growth and reproduction of tumor cells [Citation15], so angiogenesis is crucial to the genesis, development and metastasis of tumors [Citation16]. Studies have found that the genesis and progression of CRC are closely related to angiogenesis, which is critical to CRC proliferation and metastasis [Citation17]. Furthermore, increased VEGF levels and VEGFR activity were linked with poor prognosis of CRC [Citation18]. In the present study, MEX3A was substantially overexpressed in CRC tissues relative to normal tissues, and its high expression could promote CRC angiogenesis. According to previous studies, MEX3A is a potential oncogenic gene involved in carcinogenesis and progression of various types of tumors, including lung adenocarcinoma [Citation9], breast cancer [Citation13], and glioma [Citation19]. Wang et al. [Citation8] found that MEX3A knockdown inhibits osteosarcoma cell proliferation, apoptosis, and migration. Wang et al. [Citation20] found the inhibitory effect of low MEX3A expression on the progression of pancreatic ductal adenocarcinoma. Although numerous studies have investigated the functions of MEX3A in cancer, its function in CRC angiogenesis has not been reported. In our study, the involvement of MEX3A in the regulation of CRC angiogenesis was demonstrated for the first time. This result enriched our further understanding of CRC pathogenesis and suggested that targeting MEX3A may be a novel therapeutic strategy for CRC.
In addition, our results exhibited that MEX3A could promote CRC angiogenesis through activation of the glycolytic pathway. In recent years, altered energy metabolism has been considered a key mechanism to explain the typical rapid proliferation, metastasis and chemical resistance of tumor cells [Citation21]. Aerobic glycolysis is a widespread metabolic phenotype in cancer cells. There has been a lot of experimental evidence that metabolic conversion to aerobic glycolysis involves oncogenic events, including transcriptional regulation and signal transduction mechanisms [Citation22]. For example, miR-488 inhibits tumor oxaliplatin and 5-Fu resistance by targeting PFKFB3 and therefore inhibiting CRC glycolysis [Citation23]. MiR-142-3p inhibits aerobic glycolysis of CRC cells through PKM2, and then inhibits tumor cell invasion and migration [Citation24]. In addition, glycolysis has been reported to play a vital role in tumor angiogenesis. For example, Deng et al. [Citation25] found that DKK2 stimulates endothelial angiogenesis by accelerating aerobic glycolysis in CRC cells to produce lactate. Yang et al. [Citation26] found that lymphotoxin-ɑ promotes tumor formation in head and neck squamous cell carcinoma by regulating glycolysis in a PFKFB3-dependent way. Wade et al. [Citation27] revealed that low expression of miR-125a can promote angiogenesis by promoting glycolysis. In the present study, bioinformatics analysis exhibited that MEX3A was enriched in the glycolytic signaling pathway of CRC. Further studies revealed that MEX3A could promote CRC angiogenesis by activating the glycolytic pathway, which was consistent with previous findings.
Taken together, the present study revealed for the first time that MEX3A promoted CRC cell proliferation and angiogenesis, and that MEX3A promoted CRC angiogenesis by activating the glycolytic pathway. The rescue experiment further supports our findings. More importantly, our investigation revealed that high expression level of MEX3A was significantly correlated with the progression of CRC patients. Hence, MEX3A can be considered a promising biomarker for CRC diagnosis, and it be useful in future drug development as a new target for CRC treatment. However, there are still some limitations in our study. Nevertheless, is a lack of clinical studies on MEX3A, and the possibility of its application in clinical practice has not been confirmed. This is also an important direction of our future research. We will collect clinical samples for systematic analysis and verification of this topic. In a word, our study results provide new insights into the search for targets for antiangiogenic drugs.
Authors’ contributions
Y L contributed to conceptualization and data curation. MH G contributed to methodology and formal analysis. TN B and SK Z contributed to writing. All authors have reviewed and approved the final manuscript.
Disclosure statement
No potential conflict of interest was reported by the authors.
Data availability statement
The data used to support the findings of this study are available from the corresponding author upon request.
Additional information
Funding
References
- Bray F, Ferlay J, Soerjomataram I, et al. Global cancer statistics 2018: gLOBOCAN estimates of incidence and mortality worldwide for 36 cancers in 185 countries. CA Cancer J Clin. 2018;68(6):394–9.
- Aguiar Junior S, Oliveira MM, Silva D, et al. Survival of patients with colorectal cancer in a cancer center. Arq Gastroenterol. 2020;57(2):172–177.
- Biller LH, Schrag D. Diagnosis and treatment of metastatic colorectal cancer: a review. JAMA. 2021;325(7):669–685.
- Hurwitz H, Fehrenbacher L, Novotny W, et al. Bevacizumab plus irinotecan, fluorouracil, and leucovorin for metastatic colorectal cancer. N Engl J Med. 2004;350(23):2335–2342.
- Panzeri V, Manni I, Capone A, et al. The RNA-binding protein MEX3A is a prognostic factor and regulator of resistance to gemcitabine in pancreatic ductal adenocarcinoma. Mol Oncol. 2021;15(2):579–595.
- Naef V, De Sarlo M, Testa G, et al. The stemness gene Mex3A is a key regulator of neuroblast proliferation during neurogenesis. Front Cell Dev Biol. 2020;8:549533.
- Zhou X, Li S, Ma T, et al. MEX3A knockdown inhibits the tumorigenesis of colorectal cancer via modulating CDK2 expression. Exp Ther Med. 2021;22(5):1343.
- Wang B, Hong Z, Zhao C, et al. The effects of MEX3A knockdown on proliferation, apoptosis and migration of osteosarcoma cells. Cancer Cell Int. 2021;21(1):197.
- Liang J, Li H, Han J, et al. Mex3a interacts with LAMA2 to promote lung adenocarcinoma metastasis via PI3K/AKT pathway. Cell Death Dis. 2020;11(8):614.
- Li H, Liang J, Wang J, et al. Mex3a promotes oncogenesis through the RAP1/MAPK signaling pathway in colorectal cancer and is inhibited by hsa-miR-6887-3p. Cancer Commun (Lond). 2021;41(6):472–491.
- Li L, Liang Y, Kang L, et al. Transcriptional regulation of the Warburg effect in cancer by SIX1. Cancer Cell. 2018;33(3):368–385 e7.
- Shi T, Ma Y, Cao L, et al. B7-H3 promotes aerobic glycolysis and chemoresistance in colorectal cancer cells by regulating HK2. Cell Death Dis. 2019;10(4):308.
- Shi X, Sun Y, Zhang Y, et al. MEX3A promotes development and progression of breast cancer through regulation of PIK3CA. Exp Cell Res. 2021;404(1):112580.
- Wang Y, Liang Q, Lei K, et al. Targeting MEX3A attenuates metastasis of breast cancer via beta-catenin signaling pathway inhibition. Cancer Lett. 2021;521:50–63.
- Carmeliet P, Jain RK. Molecular mechanisms and clinical applications of angiogenesis. Nature. 2011;473(7347):298–307.
- Hanahan D, Weinberg RA. The hallmarks of cancer. Cell. 2000;100(1):57–70.
- Mihalache A, Rogoveanu I. Angiogenesis factors involved in the pathogenesis of colorectal cancer. Curr Health Sci J. 2014;40(1):5–11.
- Lopez A, Harada K, Vasilakopoulou M, et al. Targeting angiogenesis in colorectal carcinoma. Drugs. 2019;79(1):63–74.
- Yang C, Zhan H, Zhao Y, et al. MEX3A contributes to development and progression of glioma through regulating cell proliferation and cell migration and targeting CCL2. Cell Death Dis. 2021;12(1):14.
- Wang X, Shan YQ, Tan QQ, et al. MEX3A knockdown inhibits the development of pancreatic ductal adenocarcinoma. Cancer Cell Int. 2020;20:63.
- Ganapathy-Kanniappan S, Geschwind JF. Tumor glycolysis as a target for cancer therapy: progress and prospects. Mol Cancer. 2013;12:152.
- Doherty JR, Cleveland JL. Targeting lactate metabolism for cancer therapeutics. J Clin Invest. 2013;123(9):3685–3692.
- Deng X, Li D, Ke X, et al. Mir-488 alleviates chemoresistance and glycolysis of colorectal cancer by targeting PFKFB3. J Clin Lab Anal. 2021;35(1):e23578.
- Ren J, Li W, Pan G, et al. MiR-142-3p modulates cell invasion and migration via PKM2-mediated aerobic glycolysis in colorectal cancer. Anal Cell Pathol (Amst). 2021;2021:9927720.
- Deng F, Zhou R, Lin C, et al. Tumor-secreted dickkopf2 accelerates aerobic glycolysis and promotes angiogenesis in colorectal cancer. Theranostics. 2019;9(4):1001–1014.
- Yang JG, Wang WM, Xia HF, et al. Lymphotoxin-alpha promotes tumor angiogenesis in HNSCC by modulating glycolysis in a PFKFB3-dependent manner. Int J Cancer. 2019;145(5):1358–1370.
- Wade SM, Ohnesorge N, McLoughlin H, et al. Dysregulated miR-125a promotes angiogenesis through enhanced glycolysis. EBioMedicine. 2019;47:402–413.