ABSTRACT
Streptococcus tigurinus is a new member of the Mitis group and is associated with infective endocarditis. Low and high virulent variants have been described. A search was made in the national reference collection of endocarditis isolates for S. tigurinus–like strains by sequencing housekeeping genes (16S rRNA-gene, gdh, groEL, sodA). The strains were further profiled by polymerase chain reaction (PCR) targeting a choice of virulence genes (rib-like, cshA-like, gtfR, int, pitA, hylA). To study the prevalence and abundance of S. tigurinus in the saliva and on the mucosal membranes of 35 healthy adults, PCRs detecting two variants of the 16S operon and virulence genes were applied. Among the endocarditis isolates, eight strains (all gtfR-negative and former S. oralis) holding the specific S. tigurinus 16S motif were found, but the pattern of genes related to high virulence found in the S. tigurinus type strain could not be detected in any of these strains. A close phylogenetic proximity between S. tigurinus and S. oralis was observed, with intersectional hybrid strains formed. This was supported by concatenated housekeeping sequences, in silico DNA–DNA hybridization, pathogenomic profiling, and multidimensional scaling. In the oral samples, S. tigurinus could be detected frequently, especially in the most common operon variant, but none of the type strain–related virulence factors were found. Low virulent S. tigurinus–like strains can be found frequently and in high prevalence (66%) and abundance (12.5%) in the oral cavity of healthy adults. In strain collections, they are among the formerly known gtfR-negative S. oralis. Highly virulent strains seem to be uncommon. Though closely related, S. oralis and S. tigurinus can be separated by the presence or absence of gtfR and dextran production. Hybrids of both species can be found. The variable arsenal of virulence genes found in this study emphasizes the genetic plasticity of Mitis group streptococci.
Introduction
The precise description and classification of prokaryotic microorganisms has never been an easy task [Citation1]. One reason for the difficulties might be that the zoological definition of a species as ‘groups of interbreeding or potentially interbreeding natural populations that are reproductively isolated from other such groups’ cannot be applied to prokaryotes [Citation2]. Nevertheless, a kind of (man-made) rule of thumb has been established, stating that strains of Bacteria or Archaea possessing 16S rRNA-gene sequences with >97% identity belong to the same species, but this needs to be checked by DNA–DNA hybridization. This concept has been updated, stating that the cutoff of 97% was too low and can be raised to 98.7% [Citation3]. However, a proof by DNA–DNA hybridization, where a rate >70% is accepted to be indicative for the same species, is still useful. One problem here is that – especially in some taxa such as oral fusobacteria [Citation4] or viridans streptococci [Citation5] – the exact border between species is not well defined (or definable), and this interferes with the sometimes compulsive intention of scientists to describe a species precisely.
Within the genus Streptococcus, the classification of members of the Mitis group is especially challenging, as it shows extensive sequence polymorphisms resulting in intra-species DNA–DNA hybridization rates <70% [Citation6,Citation7]. Streptococcus tigurinus is a recently discovered oral pathogen, which is able to cause infective endocarditis, meningitis, and spondylodiscitis [Citation8–Citation10]. After the first isolates (AZ_3a [type strain, synonym DSM 24864], AZ_1-AZ_15) were described in Zürich, Switzerland, in 2012 [Citation10], additional S. tigurinus isolates were found again in Switzerland (strain 1366 with small colony variants nos. 2425 and 2426), in Japan (two isolates of infective endocarditis [Citation11]), in India (single isolate from the oral cavity of a periodontitis patient [Citation12]), again in Switzerland (several isolates from the oral cavity [Citation13], again in Japan (culture-negative endocarditis case [Citation8] and one from bacteremia [Citation14]), in Spain (endocarditis case [Citation15]), in France (two endocarditis cases [Citation16]), and in Washington, USA, where 14 isolates were recovered from numerous body sides, expanding the spectrum of associated diseases [Citation17]. Up to November 2016, seven S. tigurinus genome sequences (strains AZ_3a, 1366, 2425, 2426, DGIIBVI and JPIBVI, UC5873) have been made accessible in GenBank [Citation18–Citation20].
Among the Mitis group reference strains, Streptococcus oralis ATCC 35037T and ATCC 49296, ATCC 6249 (formerly Streptococcus mitis), and Streptococcus dentisani DSM 27088T are the nearest relatives to S. tigurinus, while S. mitis strains, including ATCC 49456T, as well as Streptococcus infantis ATCC 700779T and Streptococcus peroris ATCC 700780T, are more distantly related [Citation21]. S. mitis ATCC 15914 was reclassified as S. tigurinus recently. Confusingly, an ongoing NCBI genome sequencing BioProject (see https://gold.jgi.doe.gov/project?id=33720) on this strain is named ‘S. sanguinis ATCC 15914’.
The strain collection of the National Reference Center for Streptococci in Aachen (Germany) contained 18 previously identified S. oralis, four S. infantis, and one ‘S. tigurinus’ isolates (pre-identified by sodA-sequencing) from proven cases of infective endocarditis. The aims of this study were first to screen these strains for the newly described S. tigurinus by comparing four different housekeeping genes and, second, to profile a choice of their virulence genes. Furthermore, by applying S. tigurinus– and virulence factor–specific polymerase chain reactions (PCRs), the prevalence, abundance, and virulence of this species in saliva and on buccal mucosal membranes of 35 healthy adults were determined.
Materials and methods
Identification of endocarditis-associated strains and pathogenomic profiling
Strains and phylogenetic analysis
DNA was extracted from S. oralis SN 16495, SN 17127, SN 28194, SN 31376, SN 37569, SN 37737, SN 39325, SN 40525, SN 45448, SN 48861, SN 50746, SN 51446, SN 54788, SN 58364, SN 58746, SN 59433, SN 60579, SN 63707, S. infantis SN 54787, SN 57625, SN 17128, SN 19640, ‘S. tigurinus’ SN 62386 (all proven and epidemiologically unrelated endocarditis isolates collected by the National Reference Center for Streptococci, Aachen), and from four S. tigurinus reference strains, including type strain AZ_3a, as well as small colony variants (2425, 2426) of parental strain 1366 [Citation19] kindly provided by A. Zbinden (Zürich). The 16S rRNA-gene (Escherichia coli position 27–557) and three other housekeeping genes known for their species-specific resolution [Citation22,Citation23], gdh (coding for glucose-6-phosphate 1-dehydrogenase, gene position 745–1404), groEL (coding for a heat shock protein, molecular chaperone GroEL, gene position 555–1312), and sodA (coding for a superoxide dismutase, gene position 36–471) were amplified and sequenced. The ambiguity-free and most informative core-fragment of each gene (a length of 442, 541, 678, and 398 bp, respectively, summing up to 2,059 bp in total) was analyzed. In the case of the 16S rRNA-gene of Mitis group streptococci, complete sequencing does not lead to a concentration but to a dilution of information, and thus sequencing of the V1–V2 region (covering all annealing sites of specific oligonucleotides described so far [Citation13]) was preferred as most efficient. Sequences were compared in silico with each other and with reference strain genomes of related species available in GenBank. In addition, a concatemeric tree (‘housekeeping tree’ based on all 2,059 bp, maximum likelihood) of the genes mentioned above was built to estimate phylogenetic relatedness with reliable bootstrap values.
In silico DNA–DNA hybridization
To investigate further the phylogenetic relationship among S. tigurinus, S. oralis, S. infantis, S. peroris, and S. mitis, in silico DNA–DNA hybridization was performed using the genome-to-genome distance calculator (GGDC2) provided on the DSMZ website [Citation24]. This tool is able to calculate both the genomic distance and the probability that two strains, compared on genome level, belong to the same species defined by a DNA–DNA hybridization rate of >70%.
Multidimensional scaling of distance data
To visualize the actual phylogenetic distance between species based on in silico DNA–DNA hybridization (GGDC2) and concatemer data (16S/gdh/groEL/sodA, maximum composite likelihood), multidimensional scaling (MDS) was performed using the software ‘orange data mining’ (version 3.2, and explained by Demsar et al. [Citation25]). In brief, a principal component analysis (Torgerson) was used to initialize the plot and – in (maximal) 106 iterations – the two-dimensional distance was iteratively optimized.
In silico pathogenomic profiling
To investigate virulence gene profiles further across multiple strains of the Mitis group, including S. tigurinus, S. oralis, S. infantis, and S. peroris, the Pathogenomic Profiling Tool (PathoProT) of StreptoBase was used (http://streptococcus.um.edu.my and explained by Zheng et al. [Citation20]).
In vitro pathogenomic profiling
The presence of six virulence-associated genes was investigated using self-designed PCR assays (). These genes were rib-like (Resistance to protease, protective Immunity, originally described in invasive serotype III group B-streptococci (Streptococcus agalactiae), coding for an M6- or M28-like repetitive protein [Citation26,Citation27]), cshA-like (coding for a fibronectin-binding protein originally described in Streptococcus gordonii [Citation28,Citation29]), pitA (coding for a metal ion-depending adhesin [MIDAS] with a Willebrand factor type A, pfam13519 or VWA_2 domain also found in second type pili [PI-2] of Streptococcus pneumoniae [Citation30,Citation31]), hylA (coding for an enzyme involved in degradation of hyaluronate described in S. pneumoniae [Citation31]), and int (encoding an integrase of a transposable element found in the genome sequence of the S. tigurinus type strain). Furthermore, the gtfR gene (coding for a glycosyltransferase type GtfR) was analyzed, as it plays an important role in the adhesion of S. oralis but – interestingly enough and unusually for viridans streptococci – is described to be present in only 51% of S. oralis strains [Citation22]. All PCR conditions are summarized in . The actual expression of the gtfR gene, resulting in dextran production, was tested on sucrose-enriched (5%) Columbia Blood Agar or Mitis Salivarius Agar.
Table 1. Primers and polymerase chain reaction conditions used in this study.
In situ detection of S. tigurinus variants
To determine the prevalence, abundance, and virulence gene profile of S. tigurinus in the oral cavity as its natural habitat known so far, saliva and buccal swab samples of 35 healthy young adults (see study population for details) were screened for the presence of (a) all bacteria, (b) all Mitis group streptococci, (c) S. tigurinus in two 16S operon variants (with AGA- and CTT-motif; see below and Supplementary Figure S1), and (d) a selection of six S. tigurinus–associated virulence genes.
Study population and sampling
As probands, dental students of the University Hospital (Aachen) were recruited. Subjects were included if they had no or minimal signs of gingival inflammation and demineralization. In total, 35 students (23–32 years of age, Mage = 25.3 years; 11 male) fulfilled these criteria. All participants were free of systemic diseases, and none of them had taken any antibiotics within the last 3 months. Participating subjects were informed about the microbial and molecular biological analysis and signed an informed consent that had been approved in accordance with the guidelines of the Ethics Committee of the University Hospital (Aachen, Germany). A volume of 1.5 mL of freshly stimulated saliva, as well as swab samples of both buccal mucosae, were collected from all participants. Bacterial cells were recovered by vortexing with glass beads (diameter 1–2 mm) and centrifugation. All suspensions were then stored frozen at –72°C until further analysis.
DNA extraction and quantitative PCR
After thawing, cell suspensions were washed and recovered. To dissolve the salivary mucus, an incubation step with Bioténe® (PBF Oral Rinse, SKB, containing biofilm matrix digesting enzymes such as mutanase and dextranase) for 30 min at 37°C and additional washing ensued. After addition of 20 µL of a lysozyme/mutanolysin solution (0.3 mg of lysozyme + 10 IU of mutanolysin) to the pellet, a 30 min incubation step at 37°C preceded DNA extraction and purification using the QIAamp DNA Mini kit (‘tissue protocol’; Qiagen, Hilden, Germany) according to the manufacturer’s instructions. One microliter of purified DNA (from standards and from samples) was used as the template for real-time quantitative PCR (qPCR) on the Light Cycler 2.0 system (Roche, Mannheim, Germany). The total number of bacterial genome equivalents per microliter of DNA extract (referred to as ‘bacterial cell counts’) was measured according to Nadkarni et al. [Citation37] using S. tigurinus AZ_3a DNA as standard. Accordingly, total cell counts of Mitis group streptococci, S. tigurinus holding the AGA-motif in the 16S rRNA gene, and S. tigurinus holding the CTT-motif in the 16S rRNA gene were measured using S. tigurinus AZ_3a (AGA-version) and S. tigurinus 1366 (CTT-version) as standards and applying the primers and protocols listed in . The identity of amplicons was verified by spot-check sequencing using the Applied Biosystems 310 DNA sequencer (Applied Biosystems, Foster City, CA). All calculations were carried out using Microsoft® Excel 2010.
Results
Identification of endocarditis-associated strains and pathogenomic profiling
Among the endocarditis isolates tested, eight S. tigurinus–like strains (SN 28194, SN 37569, SN 37737, SN 40525, SN 45448, SN 48861, SN 50746, and SN 62386) could be easily identified by a specific 16S motif starting at E. coli position 176–184. In comparison to all published S. tigurinus–like 16S sequences so far, this motif demonstrated some variations, forming the consensus signature AAT(G/T)GATTATCGCATGAT(A/G). Whereas the G/T alteration was frequently found, and may even vary within a strain from operon to operon, the A/G alteration was only found in strain 7117668 (KT780462, French isolate [Citation16]). The 16S rRNA gene-based tree with 73 Mitis group strains (a few represented by two operon variants) and S. oligofermentans strain AS 1.3089T as an outgroup (see constructed by MEGA6 [Citation38]) demonstrated a distinct S. tigurinus–like cluster, including the eight endocarditis isolates of the collection (labelled ●), 23 S. tigurinus strains of other origin, but also four genome-sequenced gtfR-negative ‘S. oralis’ strains (SK255, SK304, SK313, SK1074; corresponding accession nos. NZ_AFNM00000000.1, NZ_ALJN01000025.1, AFUU00000000.1, NZ_AICT00000000.1). Because of the close phylogenetic relationship with the S. tigurinus type strain – according to 16S, housekeeping genes and whole genome (see below) data –these strains were further named ‘S. tigurinus–like (formerly S. oralis)’.
Figure 1. Phylogenetic tree based on the 16S rRNA-gene of 73 Mitis group streptococci. Program MEGA6, maximum likelihood algorithm subsequently to ClustalW-alignment, standard preferences, 100 bootstraps [Citation38]; ●, SN strains from the collection; CTT-motif (strains framed hold a variant of the 16S rRNA gene characterized by a CTT-motif). Streptococcus oligofermentans strain AS 1.3089T was used as the outgroup.
![Figure 1. Phylogenetic tree based on the 16S rRNA-gene of 73 Mitis group streptococci. Program MEGA6, maximum likelihood algorithm subsequently to ClustalW-alignment, standard preferences, 100 bootstraps [Citation38]; ●, SN strains from the collection; CTT-motif (strains framed hold a variant of the 16S rRNA gene characterized by a CTT-motif). Streptococcus oligofermentans strain AS 1.3089T was used as the outgroup.](/cms/asset/79f1f7b0-e321-4eaf-bb77-14a790e6742f/zjom_a_1307079_f0001_b.gif)
Interestingly, a few S. tigurinus or –like isolates (1366, 2425, 2426, SN 37569 from the collection; strain 7117668 from a French collection) hold two 16S rRNA gene operon variants, one very common AGAGGAGCTTGCTCTTCTT (which is abbreviated to ‘AGA-motif’) and one with the sequence CTTGGTGCTTGCDCCGAGC (which is abbreviated to ‘CTT-motif’) both starting at E. coli position 77 (or 69 counting from the 5ʹ end of primer F1). Of even more interest, the same CTT-motif was found in a few strains of closely related species at the same position, including the S. oralis type strain ATCC 35037T, the S. parasanguinis type strain ATCC 15912T (in all 16S rRNA-operons), the S. infantis strains SK970 and SK1076 (in all 16S rRNA-operons, whereas S. infantis ATCC 700779T holds a 16S rRNA operon variant with AGA-motif), and strains SN 16495 and SN 63707 of the collection. An overview of these results is given in where strains with a 16S CTT-motif are framed. Other clusters were formed by S. infantis–S. peroris, S. oralis (gtfR-positive strains only), S. mitis, and a few strains together with ATCC 6249, the latter of which were further named ‘ATCC 6249-like’.
To some extent, the Mitis group phylogeny based on the 16S gene was found to be reflected in gdh-, groEL-, and sodA-derived trees (Supplementary Figs. S2–S4). However, whereas S. infantis (together with S. peroris, a species that is based on a single strain – a singleton) and S. mitis form relatively distinct clusters (confirmed by DNA–DNA hybridization; see below and ), S. tigurinus–like strains are positioned in close proximity to or even intermingling with S. oralis, challenging the species definition. However, a concatemeric tree constructed by combining 16S, gdh, groEL, and sodA sequence data was able to separate S. tigurinus from S. oralis further, leaving S. mitis and S. infantis–S. peroris as distinct clusters (). From this concatemeric tree, two other phylogentical characteristics became obvious: (a) linker strains (hybrids) of S. oralis and S. tigurinus do exist, such as SN 63707; (b) S. tigurinus 1366 (and its progenies 2425 and 2426) is relatively distantly related to type strain AZ_3a; (c) ATCC 6249 together with five of the endocarditis isolates (SN 39325, SN 48861, SN 54787, SN 54788, and SN 58364) form another distinct cluster.
Table 2. Probability matrix comparing strains within the Streptococcus tigurinus–Streptococcus oralis supercluster with each other and with closely related species (Streptococcus infantis, Streptococcus peroris, Streptococcus mitis). Numbers show the probability that two strains compared at the genome level in silico are of the same species [Citation24]. Boxes indicate probabilities of gtfR-negative or gtfR-positive S. tigurinus–S. oralis strains and the outgrouping of ATCC 6249, respectively.
Figure 2. Phylogenetic tree based on the 16S/gdh/groEL/sodA-concatenated sequences of 50 Mitis group streptococci. Program MEGA6, maximum likelihood algorithm subsequently to ClustalW-alignment, standard preferences, 100 bootstraps [Citation38]; ●, SN strains from the collection. S. oligofermentans strain AS 1.3089T was used as the outgroup.
![Figure 2. Phylogenetic tree based on the 16S/gdh/groEL/sodA-concatenated sequences of 50 Mitis group streptococci. Program MEGA6, maximum likelihood algorithm subsequently to ClustalW-alignment, standard preferences, 100 bootstraps [Citation38]; ●, SN strains from the collection. S. oligofermentans strain AS 1.3089T was used as the outgroup.](/cms/asset/c9abb7ca-d43c-4528-ba8b-4a599f805570/zjom_a_1307079_f0002_oc.jpg)
To investigate further the phylogenetic relationship among S. tigurinus, S. oralis, S. infantis, S. peroris, and S. mitis, in silico DNA–DNA hybridization was performed, calculating a matrix that indicated the probability that both strains belong to the same species [Citation24]. The results are presented in . For background information, two identical strains usually result in a probability of 98%, and strains of a well-defined species, such as S. pyogenes, in a probability between 94 and 98%. In contrast, the S. oralis strains showed a reduced probability between 38 and 51% of belonging to the same species. S. tigurinus, including –like strains, also showed a reduced probability between 22 and 72%, with the small-colony variant strain 1366 even producing values as low as 21%. Comparing S. tigurinus(–like) with S. oralis strains resulted in a probability between 14 and 24%. Taken together, the results demonstrate the heterogeneity within the S. tigurinus–S. oralis supercluster at the genome level, again challenging the species definition.
The MDS visualization of genomic distances of M-clade streptococcal species revealed clustering ()). While S. infantis (together with S. peroris) and S. mitis formed distant clusters, S. oralis and S. tigurinus were found to be closely related. S. tigurinus genomes and those genomes classified as S. tigurinus–like formed one cluster in direct vicinity to the cluster build by gtfR-positive S. oralis strains. Reference strain ATCC 6249 – recently reclassified as S. oralis – can be found at the edge of the S. oralis cluster. In comparison to the genomic distance, the MDS plot of the concatemer data of four in series connected partial housekeeping genes showed that most species distinctions were retained, albeit with some rearrangement of the spatial distributions ()). S. infantis (together with S. peroris) formed a cluster at a greater distance to S. mitis, S. oralis, and S. tigurinus, with the latter two converging. Strain S. tigurinus 1366 and its derivatives 2425 and 2426 exhibited a great distance, not only to the S. tigurinus type strain AZ_3a, but also to all other clusters of the Mitis group. S. oralis ATCC 6249 (together with five ATCC6249-like isolates of the collection) is again found at the edge of the S. oralis–S. tigurinus supercluster, but this time it is more adjacent to S. tigurinus. In both MDS plots, gtfR-positive S. oralis and gtfR-negative S. tigurinus(–like) strains formed separate clusters but still in close contact (like the two halves of a coffee bean). An intermingling between both species can be observed and linking (or hybrid) strains identified (compare with above and ).
Table 3. Presence of the 16S Tigurinus motif, the 16S CTT-motif, and six virulence-associated genes among endocarditis strains in the collection (SN) and S. tigurinus reference strains.
Figure 3. Two-dimensional representation of a multidimensional scaling (MDS, Torgerson scaling). (a) Plot is based on genomic data of selected Mitis group strains. Genomic distances were calculated by in silico DNA–DNA hybridization (GGDC2 algorithm [Citation18]). (b) Plot is based on concatemer data (16S/gdh/groEL/sodA) of selected Mitis group strains, including the SN strains from the collection. Distances were calculated with the maximum composite likelihood algorithm. S. oligofermentans strain AS 1.3089T was used as the outgroup in both plots.
![Figure 3. Two-dimensional representation of a multidimensional scaling (MDS, Torgerson scaling). (a) Plot is based on genomic data of selected Mitis group strains. Genomic distances were calculated by in silico DNA–DNA hybridization (GGDC2 algorithm [Citation18]). (b) Plot is based on concatemer data (16S/gdh/groEL/sodA) of selected Mitis group strains, including the SN strains from the collection. Distances were calculated with the maximum composite likelihood algorithm. S. oligofermentans strain AS 1.3089T was used as the outgroup in both plots.](/cms/asset/4ea68010-7772-424a-8349-ce5fcc327482/zjom_a_1307079_f0003_oc.jpg)
More insights were obtained by applying the recently published Pathogenomic Profiling Tool (PathoProT of StreptoBase [Citation20]) resulting in an informative heat map matrix (). This tool screens for the presence of virulence genes in a selection of published streptococcal genomes. In principal, the presence and absence of the virulence gene is labeled differentially, but this is threshold dependent (both of sequence identity and completeness) as the BLAST algorithm is used. The results underline the uniqueness of the S. tigurinus type strain AZ_3a. This strain possesses a combination of virulence factor gene homologues of S. pneumoniae absent in other S. tigurinus(–like) strains or other streptococci belonging to the Mitis group (or M-clade [Citation20]). These virulence factors include: cbpG, coding for a pneumococcal cholin binding serine protease with adhesive properties; hylA and hysA homologues, coding for hyaluronidases; the pneumolysin-gene ply, coding for a pore-forming toxin; and the autolysin-gene lytA, coding for a peptidoglycan hydrolase. On the other hand, all typical (sensu stricto) S. oralis strains differ from S. tigurinus(–like) not only in the presence of gtfR (here identified as a gtfD–gtfG homologue), but also in zmpC, encoding a zinc-metalloproteinase involved in neutrophil extravasation, inflammation, and tissue remodeling, and in possessing iga encoding an immunoglobulin A1 protease, both typically found in S. pneumoniae. Additionally, ATCC 49296-like S. oralis strains lack – in comparison to all other Mitis group strains except and interestingly enough S. tigurinus AZ_3a – a whole range of genes responsible for rhamnose synthesis, such as rmlA-C and rfbA-D, as well as homologues responsible for the formation of streptococcal capsules, such as wchA or cpsE. ATCC 6249, differing from S. tigurinus and S. oralis sensu stricto both in in silico DNA–DNA hybridization () and concatemeric tree position, possesses the genes for capsule formation but lacks rhamnose synthesis genes, a combination also found in the S. infantis type strain.
Figure 4. An informative heat map of streptococcal virulence genes generated by the PathoProT tool (StreptoBase). Presence of a virulence gene is labeled in gray and absence in white. Note that ‘absence’ is quite threshold dependent. The standard preferences used here are thresholds for sequence identity and completeness of both >50%. Genes framed in bold are further discussed in the text.
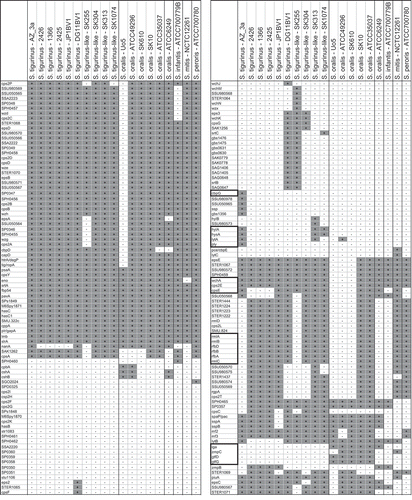
By analyzing the pattern of virulence associated genes rib-like, int, cshA-like, pitA-like, and hylA-like in the strains, the following was observed (): The rib-like and the integrase encoding gene were only present in the S. tigurinus type strain AZ_3a and in the S. tigurinus candidate strain SN 62386 of the collection. The cshA-like gene was present in all four S. tigurinus reference strains, as well as in four S. oralis and in two S. oralis-S. tigurinus intersectional strains, but not in any of the S. tigurinus–like isolates. The pitA-like and hylA-like genes were only present in the S. tigurinus type strain AZ_3a and in SN 50746 of the collection.
Finally, the gtfR-gene distribution among S. oralis/tigurinus/infantis was investigated (). To confirm that gftR-positive strains actually do produce dextran by glycosyltransferase activity, a Mitis Salivarius Agar or Columbia Blood Agar medium was enriched with 5% sucrose. As representatively shown in Supplementary Figure S5, all of the gtfR-negative strains showed flat, dry, and not very entrenching colonies, whereas gtfR-positive strains showed much higher, rounded, water-absorbing and entrenching colonies due to strong dextran production. The following principles were found: (a) S. tigurinus(–like) strains did not possess the gtfR-gene and did not produce dextran, except SN 37569 (see ), interestingly the only S. tigurinus-like strain with a second 16S rRNA-operon variant holding the CTT-motif; (b) all S. oralis sensu stricto strains (both ATCC 35037-like and ATCC 49296-like) hold the gtfR gene producing dextran; (c) S. infantis (together with the S. peroris strain) did not have a gtfR homologue, as it is missing in ATCC 6249-like strains. The latter strain was first deposed as ‘S. viridans’, later reclassified as ‘S. mitis’, and recently reclassified as ‘S. oralis’, but this may not be correct either. Instead, ATCC 6249-like strains seem to form a distinct subclade, which should be referred to as S. oralis genomo-subspecies 1 until further description. Finally, (d) even after intensively trying to categorize the strains by several methods, some strains (SN 16495, SN 50746, and SN 63707) showed a hybrid profile.
In situ detection of S. tigurinus variants
Testing oral samples of healthy young adults for the abundance of S. tigurinus (two different operon variants [AGA and CTT] were tested separately) in relation to all bacteria and all Mitis group streptococci revealed the following results: S. tigurinus was found in 23/35 (66%) saliva samples, with a proportion between 0.01 and 12.5% of all bacteria and between 0.05 and >90% (the latter observed in three cases) of Mitis group streptococci. The CTT-variant was only found in two samples and with low abundance. In the buccal swab samples, S. tigurinus was found and confirmed by amplicon sequencing only once, suggesting that this species (associated with invasiveness) is not particularly enriched on mucosal membranes. However, the buccal samples were, probably because of the self-sampling procedure chosen, not very cell rich, reducing the overall sensitivity of this investigation.
The PCR-based analysis of S. tigurinus–associated virulence genes in the same oral samples revealed that the rib-, pitA-, and hylA-like genes, presumably representing highly virulent S. tigurinus strains, were absent. However, the virulence genes encoding the fibronectin-binding domain CshA and the integrase of a transposable element were found in 69 and 54%, respectively, of saliva samples, but their presence was independent of the S. tigurinus abundance, indicating that these factors are not species specific. Furthermore, gtfR – specific for S. oralis sensu stricto as outlined above – was found in all saliva samples.
Discussion
Based on a Tigurinus-16S signature sequence, S. tigurinus–like strains were frequently found in the collection of endocarditis isolates and in databases worldwide. It became obvious from the 16S rRNA gene–based phylogenetic tree that the correct placement of strains within the Mitis group is at least partially hampered because of different 16S operon variations and numbers. According to a BLAST search and the rrn Data Base [Citation39], the number of 16S operons within the Mitis group strains seems to vary from one (S. oralis ATCC 35037, S. pseudopneumoniae strains) to five (as in SK255). However, BLAST results may be incomplete if fewer than four operons were detected or even wrong if more than four operons were detected (possibly because of duplication during assembly process), as almost all of the genomes are not closed. In fact, all closed Streptococcus genomes have four rRNA operons. However, there are reports of different variants of the operons generated by recombination even between species [Citation6].
In particular, among different operons, at least two variations of the V1 region of S. tigurinus and a few other Mitis group species were observed (starting with an AGA- or CTT-motif, respectively; Supplementary Figure S1). Interestingly, both variations lead to the same stem-loop structure with similar dG (–12.9 kJ·mol−1 in AGA vs. –16.2 kJ·mol−1 in the CTT version applying Mfold [Citation40]). A simple BLAST search revealed that this V1 stem structure is hypervariable, for instance several motifs can be found among E. coli strains. However, as the resolution of the 16S rRNA gene is rather low for Mitis group species and as the same V1 variant occurs species independently, this may lead to phylogenetical deception.
With a few exceptions discussed below, S. tigurinus–like strains were only found among ‘S. oralis’ strains, which do not hold the glycosyltransferase-gene gtfR. Therefore, it is assumed that all (or at least most) of the gtfR-negative S. oralis might be S. tigurinus. This assumption is further supported by the fact that S. oralis is the only non-hemolytic Streptococcus species possessing gtf-positive and -negative strains [Citation22]. In the study cited (from 2005) – testing 148 strains (24 taxa) of non-hemolytic streptococci – it is demonstrated that gtf sequences were either present in all strains of a given taxon or completely absent. As the only exception, about half (51%) of S. oralis possessed a gtf gene (gtfR variant). It is postulated that the other half of these S. oralis strains might have been (at least partially) S. tigurinus. Exceptions of this assumption (gtfR negative = S. tigurinus, gtfR positive = S. oralis), however, do exist in a few strains interestingly co-arising with the CTT-motif (e.g. strain SN 37569; ). By further categorizing the strains, applying concatemer (16S/gdh/groEL/sodA) tree analysis and DNA–DNA hybridization, linker strains with a S. oralis–S. tigurinus hybrid character became recognizable (SN 16495, SN 50746, and SN 63707; ), with two of them holding the CTT-motif. Such hybrids have been described before within the Mitis group, for example formed between S. oralis and S. mitis (strain SK597 [Citation6]). Thus, a clear separation between S. oralis and S. tigurinus, preferred by whatever reason such as ‘different risks for endocarditis’, will never be sharp. Another group of strains with a comparable distance to S. oralis (and S. mitis) is for instance formed by ATCC 6249 and five endocarditis isolates of the collection according to the tree of concatenated sequences (), the probability matrix (), as well as the MDS plot (). This could be evidence for another ‘(sub-)species’. If so, it explains the difficulties and the inconsistency in nomenclature of this strain in the literature and databases. On the other hand, among S. tigurinus(–like) strains, strain 1366 and its small-colony forming derivatives 2425 and 2426 exhibited some distance not only to the S. tigurinus type strain AZ_3a, but also to all other clusters of the Mitis group. This was found in several other studies before including a recent study from France, where shetA encoding for exfoliative toxin was used as a phylogenetic marker [Citation16]. However, recent analysis of 195 Mitis group core genome sequences showed that strain 1366 and its progenies, even with some distance to AZ_3a, clearly belong to the S. tigurinus cluster [Citation7]. This study, a re-evaluation of the taxonomy of the Mitis group of the genus Streptococcus based on core genomes and MLSA, was contemporaneously and independently (from the present study) conducted, and came to the following analogue conclusions. First is the need for reclassification of S. tigurinus as S. oralis subsp. tigurinus comb. nov., with the addition that most strains do not produce extracellular polysaccharide (thus are gtfR and GTFR negative). In addition, they found that most of these strains also do not produce IgA1 protease. Second, strains related to ATCC 6249 form another distinct cluster within the S. oralis clade (S. oralis genomo-subspecies 1). Third, a proportion of publicly available Mitis group genomes in GenBank are incorrectly identified, which is worrying, and a critical curation is needed. Strains SK255, SK394, SK313, and SK1074 are members of the S. tigurinus but not S. oralis cluster. It should also be mentioned that according to the same study, S. oligofermentans strain AS 1.3089T, used as outgroup in the present study, was reclassified as a later synonym of Streptococcus cristatus.
The impossibility (or frustration) of a sharp species definition becomes even more evident when virulence genes are included into the stratification. Genes identified to be associated with high virulence in S. tigurinus AZ_3a [Citation31] are not found in every S. tigurinus strain. To be exact and according to the in silico and in vitro analysis, rib, pitA, and hylA homologues are rare, as are lytA and ply (in silico analysis only, applying thresholds of sequence identity and completeness > 50%) among S. tigurinus strains. Finding a strain matching the S. tigurinus type strain AZ_3a virulence is unlikely. However, as S. pneumoniae is the pathogenic variant of S. mitis, AZ_3a-like strains may be a more virulent form within the S. tigurinus–S. oralis supercluster, and parallels in evolution should exist. Interestingly, only AZ_3a contains a collection of close homologues of S. pneumoniae virulence genes, in particular those coding for pneumolysin Ply together with autolysin LytA, cholin-binding protein CbpG, and hyaluronidases HylA/HysA (the latter three, however, are also found in a few other strains). This could be an explanation for its exceptional invasiveness. From a recent study [Citation41], it is known that pneumococcal lytA and ply genes are located on a pathogenic island with a diversity of types evolved in eight steps. Their corresponding products might function together forming a (patho)physiological protein network. In addition, previous experiments have shown that the combination of ply and lytA is supporting optimal biofilm formation in vitro. Homologues of ply and lytA were described in S. pseudopneumoniae, S. mitis, S. oralis, S. infantis, and S. dentisani [Citation41], the latter with less pathogenic [Citation7] and possibly more probiotic potential, as it was isolated from caries-free subjects [Citation42] and might have a caries-protective activity (López-lópez et al. [Citation43]). This shows that in principal, ply-lytA-like genes are frequent, but (patho)physiologically relevant variants might be rare. The increased ply-lytA sequence identity and completeness (lytA: 99% coverage, 78% positive matches; ply: 99% coverage, 71% positive matches) found in S. tigurinus AZ_3a might be indicative for its (patho)physiological relevance. Possibly, AZ_3a evolved from a horizontal gene transfer event between a S. tigurinus–like strain and S. pneumoniae.
S. tigurinus strains were graduated in low- and high-virulence strains before according to in vitro [Citation19,Citation31] as well as in vivo [Citation9] data. There is ample evidence that the virulence of Mitis group strains in general is very variable. For instance, in a neutropenic mouse model, the lethal infective dose (LD50) of bacterial cells of different S. mitis strains varied between 1.9 × 104 CFU and 1.6 × 106 CFU [Citation44]. Likewise, the infective dose (IF90) for the S. tigurinus AZ_3a (and AZ_14) tested in rats was 104 CFU, but that of S. tigurinus strain AZ_8 was higher, emphasizing intraspecies virulence variability. Very recently, strain AZ_8 was confirmed as low virulent by pathogenomic profiling [Citation31].
The prevalence and abundance of S. tigurinus was further investigated in the oral specimens of 35 volunteers. The prevalence found was 66%. The prevalence had been determined before but with inconsistent results. Zbinden et al. [Citation10], screening saliva specimens of 31 volunteers by selective growth, MALDI-TOF MS, and subsequent 16S-sequencing, did not identify S. tigurinus among 608 isolates. In contrast, the same group found the species in roughly 50% of both periodontitis cases and controls by applying TaqMan PCR in a later study [Citation13]. From oral samples, culture-based methods are limited in accurate selection (because of the absence of a typical colony morphology and the dominance of S. mitis), but also identification of S. tigurinus (including MALDI-TOF MS as tested by Isaksson et al. [Citation45]). Thus, an underestimation of S. tigurinus in the oral cavity is likely by choosing a culture-based approach. Concerning S. tigurinus abundance, it was found that it can be very high in a few individuals, reaching >90% of Mitis group streptococci. The present study is also the first to screen oral samples directly for S. tigurinus genes presumably associated with high virulence. From the results – even if not testing all relevant genes – it can be excluded that strains with a type-strain-like virulence pattern are frequent.
In conclusion and visualized in the MDS plots of genomic as well as concatemer data (), ample evidence was found that some species within the Mitis group are more confluent than distinct, especially comparing S. oralis and S. tigurinus clusters forming ‘two halves of one coffee bean’. Moreover, hybrid strains can be expected that do not fit into man-made pigeonholes. However, though closely related, S. oralis and S. tigurinus can be genetically and phenotypically separated by the presence or absence of gtfR and dextran, as well as IgA protease production. Taken together, the present results challenge the current species concept within the Mitis group, and S. tigurinus is no exception here. Another consequence is that the species name given to a strain has almost no predictive value for its virulence gene arsenal and especially not for the actual expression of these genes. It is recommended that those Mitis group strains causing a fatal clinical outcome should be sent to a reference laboratory for pathogenomic profiling. Furthermore, the present data underline that Mitis group streptococci harbor an exceptional ‘talent’ for recombination, diversification, and – ultimately – evolution.
Supplementary Material
Download MS Word (1.1 MB)Disclosure statement
No potential conflict of interest was reported by the authors.
Supplementary Material
The supplemental material for this article can be accessed here.
Additional information
Notes on contributors
Georg Conrads
Professor Georg Conrads is a micro- and molecular biologist and specialist in oral (dental) and anaerobic microbiology. His areas of interest include periodontal, endodontic, periapical, periimplantitis-associated, and caries-associated dysbiosis and corresponding odontogenic infections. He also developed diagnostic tests for mixed anaerobic infections and new treatment strategies (laser application, new antimicrobials, and antimicrobial nanogels). He is an affiliated professor at the Department of Operative Dentistry, Periodontology and Preventive Dentistry and (since 2002) head of the Division of Oral Microbiology and Immunology, RWTH University Hospital, Aachen, Germany.
Svenja Barth
Svenja Barth is M.Sc. in biology and finished her studies in 2015 with a thesis about Streptococcus tigurinus and its phylogeny at the Division of Oral Microbiology and Immunology, RWTH University Hospital, Aachen, Germany.
Maureen Möckel
Maureen Möckel is M.Sc. in biology and finished her studies in 2017 with a thesis about Streptococcus tigurinus and its virulence at the Division of Oral Microbiology and Immunology, RWTH University Hospital, Aachen, Germany.
Lucas Lenz
Lucas Lenz is master student in biology and currently writing his thesis about Streptococcus tigurinus isolation and phenotypic characterization at the Division of Oral Microbiology and Immunology, RWTH University Hospital, Aachen, Germany.
Mark van der Linden
Dr. Mark P. G. van der Linden (PhD, Privatdozent) studied biochemistry at the University of Groningen, The Netherlands, and received a PhD in 1993. After Postdoc positions at the Max-Planck Institute of Molecular Genetics, Berlin, Germany and German Cancer Research Centre (DGFZ), Heidelberg, he moved to industry and became Director Bioinformatics at Europroteome AG, Henningsdorf, Germany. In 2004 he received a scientific position at the Department of Medical Microbiology and three years later he became Head of the National Reference Centre for Streptococci, both located at the RWTH University Hospital, Aachen, Germany. In 2016 he finished his professorial theses (“Habilitation”) on pneumococcal vaccination and epidemiology.
Karsten Henne
Dr. rer. nat. Karsten Henne studied biochemistry at the Ruhr University Bochum (Germany) and received a PhD at the Helmholtz Centre for Infection Research (HZI), Brunswick (Germany), in drinking water microbiology. His areas of interest include microbial communities of the oral cavity and their metabolic activities, with a focus on periodontal and caries diseases. His special interests are oral streptococci and campylobacter. He is a postdoc at the Division of Oral Microbiology and Immunology, in the Department of Operative Dentistry, Periodontology & Preventive Dentistry at the RWTH Aachen University Hospital, Germany.
References
- Oren A, Garrity GM. Then and now: a systematic review of the systematics of prokaryotes in the last 80 years. Antonie Van Leeuwenhoek. 2014;106:1–14.
- Stackebrand E, Goebel BM. Taxonomic note: a place for DNA-DNA reassociation and 16S rRNA sequence analysis in the present species definition in bacteriology. Int J Syst Evol Microbiol. 1994;44:846–849.
- Stackebrand E, Ebers J. Taxonomic parameters revisited: tarnished gold standards. Microbiol Today. 2006;33:152–155.
- Conrads G, Claros MC, Citron DM, et al. 16S-23S rDNA internal transcribed spacer sequences for analysis of the phylogenetic relationships among species of the genus Fusobacterium. Int J Syst Evol Microbiol. 2002;52:493–499.
- Bishop CJ, Aanensen DM, Jordan GE, et al. Assigning strains to bacterial species via the internet. BMC Biol. 2009;7:3.
- Kilian M, Poulsen K, Blomqvist T, et al. Evolution of Streptococcus pneumoniae and its close commensal relatives. PLoS One. 2008;3:e2683.
- Jensen A, Scholz CF, Kilian M. Re-evaluation of the taxonomy of the Mitis group of the genus Streptococcus based on whole genome phylogenetic analyses, and proposed reclassification of Streptococcus dentisani as Streptococcus oralis subsp. dentisani comb. nov., Streptococcus tigurinus as Streptococcus oralis subsp. tigurinus comb. nov., and Streptococcus oligofermentans as a later synonym of Streptococcus cristatus. Int J Syst Evol Microbiol. 2016;66:4803–4820.doi:10.1099/ijsem.0.001433.
- Kanamori H, Kakuta R, Yano H, et al. A case of culture-negative endocarditis due to Streptococcus tigurinus. J Infect Chemother. 2015;21:138–140.
- Veloso TR, Zbinden A, Andreoni F, et al. Streptococcus tigurinus is highly virulent in a rat model of experimental endocarditis. Int J Med Microbiol. 2013;303:498–504.
- Zbinden A, Mueller NJ, Tarr PE, et al. Streptococcus tigurinus sp. nov., isolated from blood of patients with endocarditis, meningitis and spondylodiscitis. Int J Syst Evol Microbiol. 2012;62:2941–2945.
- Miyazato A, Ohkusu K, Tachi Y, et al. Two cases of infective endocarditis caused by Streptococcus tigurinus. Kansenshogaku Zasshi. 2014;88:304–306.
- Dhotre SV, Mehetre GT, Dharne MS, et al. Isolation of Streptococcus tigurinus - a novel member of Streptococcus mitis group from a case of periodontitis. FEMS Microbiol Lett. 2014;357:131–135.
- Zbinden A, Aras F, Zbinden R, et al. Frequent detection of Streptococcus tigurinus in the human oral microbial flora by a specific 16S rRNA gene real-time TaqMan PCR. BMC Microbiol. 2014;14:231.
- Hirai J, Sakanashi D, Hagihara M, et al. Bacteremia due to Streptococcus tigurinus: a case report and literature review. J Infect Chemother. 2016 Nov;22(11):762–766. doi:10.1016/j.jiac.2016.04.011.
- Michelena A, Bonavila C, Zubeltzu B, et al. Endocarditis due to Streptococcus tigurinus: presentation of a case and a review of the literature. Enferm Infecc Microbiol Clin. 2015;33:575–576.
- Peuchant O, Wirth G, Tixier R, et al. Infective endocarditis caused by Streptococcus tigurinus-like organisms. New Microbes New Infect. 2016;13:51–53.
- Bourassa L, Clarridge JE 3rd. Clinical significance and characterization of Streptococcus tigurinus isolates in an adult population. J Clin Microbiol. 2015;53:3574–3579.
- Gizard Y, Zbinden A, Schrenzel J, et al. Whole-Genome sequences of Streptococcus tigurinus Type Strain AZ_3a and S. tigurinus 1366, a strain causing prosthetic joint infection. Genome Announc. 2013;1(2):e00210–12.
- Zbinden A, Quiblier C, Hernandez D, et al. Characterization of Streptococcus tigurinus small-colony variants causing prosthetic joint infection by comparative whole-genome analyses. J Clin Microbiol. 2014;52:467–474.
- Zheng W, Tan TK, Paterson IC, et al. StreptoBase: an oral Streptococcus mitis group genomic resource and analysis platform. PLoS One. 2016;11:e0151908.
- Zbinden A, Bostanci N, Belibasakis GN. The novel species Streptococcus tigurinus and its association with oral infection. Virulence. 2015;6:177–182.
- Hoshino T, Fujiwara T, Kilian M. Use of phylogenetic and phenotypic analyses to identify nonhemolytic streptococci isolated from bacteremic patients. J Clin Microbiol. 2005;43:6073–6085.
- Teng LJ, Hsueh PR, Tsai JC, et al. groESL sequence determination, phylogenetic analysis, and species differentiation for viridans group streptococci. J Clin Microbiol. 2002;40:3172–3178.
- Meier-Kolthoff JP, Klenk HP, Goker M. Taxonomic use of DNA G+C content and DNA-DNA hybridization in the genomic age. Int J Syst Evol Microbiol. 2014;64:352–356.
- Demsar J, Curk T, Erjavec A, et al. Orange: data mining toolbox in python. J Machine Learn Res. 2013;14:2349–2353.
- Stalhammar-Carlemalm M, Stenberg L, Lindahl G. Protein rib: a novel group B streptococcal cell surface protein that confers protective immunity and is expressed by most strains causing invasive infections. J Exp Med. 1993;177:1593–1603.
- Wastfelt M, Stalhammar-Carlemalm M, Delisse AM, et al. The Rib and alpha proteins define a family of group B streptococcal surface proteins that confer protective immunity. Adv Exp Med Biol. 1997;418:619–622.
- Jenkinson HF, McNab R, Holmes AR, et al. Function and immunogenicity of cell-wall-anchored polypeptide CshA in oral streptococci. Adv Exp Med Biol. 1997;418:703–705.
- McNab R, Jenkinson HF, Loach DM, et al. Cell-surface-associated polypeptides CshA and CshB of high molecular mass are colonization determinants in the oral bacterium Streptococcus gordonii. Mol Microbiol. 1994;14:743–754.
- Bagnoli F, Moschioni M, Donati C, et al. A second pilus type in Streptococcus pneumoniae is prevalent in emerging serotypes and mediates adhesion to host cells. J Bacteriol. 2008;190:5480–5492.
- Diene SM, Francois P, Zbinden A, et al. Comparative genomics analysis of Streptococcus tigurinus strains identifies genetic elements specifically and uniquely present in highly virulent strains. PLoS One. 2016;11:e0160554.
- Liu WT, Marsh TL, Cheng H, et al. Characterization of microbial diversity by determining terminal restriction fragment length polymorphisms of genes encoding 16S rRNA. Appl Environ Microbiol. 1997;63:4516–4522.
- Molbak L, Klitgaard K, Jensen TK, et al. Identification of a novel, invasive, not-yet-cultivated Treponema sp. in the large intestine of pigs by PCR amplification of the 16S rRNA gene. J Clin Microbiol. 2006;44:4537–4540.
- Chalmers NI, Palmer RJ Jr., Cisar JO, et al. Characterization of a Streptococcus sp.-Veillonella sp. community micromanipulated from dental plaque. J Bacteriol. 2008;190:8145–8154.
- Glazunova OO, Raoult D, Roux V. Partial sequence comparison of the rpoB, sodA, groEL and gyrB genes within the genus Streptococcus. Int J Syst Evol Microbiol. 2009;59:2317–2322.
- Peyyala R, Kirakodu SS, Ebersole JL, et al. Novel model for multispecies biofilms that uses rigid gas-permeable lenses. Appl Environ Microbiol. 2011;77:3413–3421.
- Nadkarni MA, Martin FE, Jacques NA, et al. Determination of bacterial load by real-time PCR using a broad-range (universal) probe and primers set. Microbiology. 2002;148:257–266.
- Tamura K, Stecher G, Peterson D, et al. MEGA6: molecular evolutionary genetics analysis version 6.0. Mol Biol Evol. 2013;30:2725–2729.
- Stoddard SF, Smith BJ, Hein R, et al. rrnDB: improved tools for interpreting rRNA gene abundance in bacteria and archaea and a new foundation for future development. Nucleic Acids Res. 2015;43:D593–D598.
- Zuker M. Mfold web server for nucleic acid folding and hybridization prediction. Nucleic Acids Res. 2003;31:3406–3415.
- Morales M, Martin-Galiano AJ, Domenech M, et al. Insights into the evolutionary relationships of LytA autolysin and Ply pneumolysin-like genes in Streptococcus pneumoniae and related streptococci. Genome Biol Evol. 2015;7:2747–2761.
- Camelo-Castillo A, Benitez-Paez A, Belda-Ferre P, et al. Streptococcus dentisani sp. nov., a novel member of the mitis group. Int J Syst Evol Microbiol. 2014;64:60–65.
- López-lópez A, Camelo-castillo AJ, Ferrer MD, et al. Health-associatedniche inhabitants as oral probiotics: the case of Streptococcus dentisani. Front Microbiol. 2017;8:379. doi: 10.3389/fmicb.2017.00379.
- Shelburne SA, Sahasrabhojane P, Saldana M, et al. Streptococcus mitis strains causing severe clinical disease in cancer patients. Emerg Infect Dis. 2014;20:762–771.
- Isaksson J, Rasmussen M, Nilson B, et al. Comparison of species identification of endocarditis associated viridans streptococci using rnpB genotyping and 2 MALDI-TOF systems. Diagn Microbiol Infect Dis. 2015;81:240–245.