ABSTRACT
Introduction: Campylobacter concisus is an oral bacterium that is associated with inflammatory bowel disease (IBD) and Barrett’s esophagus (BE). Programmed cell death ligand-1 (PD-L1) is an immune checkpoint protein that is used by tumor cells for immune evasion and has increased expression in patients with IBD and BE. We examined whether C. concisus upregulates PD-L1 expression in intestinal and esophageal epithelial cells.
Methods: Human intestinal epithelial HT-29 cells and esophageal epithelial FLO-1 cells with and without interferon (IFN)-γ sensitization were incubated with C. concisus strains. The level of PD-L1 mRNA was quantified using quantitative real-time PCR. Cytokines were measured using Enzyme-Linked Immunosorbent Assay (ELISA). Apoptosis of HT-29 and FLO-1 cells were measured using caspase 3/7 assay.
Results: We found that intestinal epithelial cells with IFN-γ sensitization incubated with C. concisus significantly upregulated PD-L1 expression and significantly increased the production of interleukin (IL)-8. Whereas, PD-L1 expression was significantly inhibited in IFN-γ sensitized FLO-1 cells incubated with C. concisus strains. Furthermore, FLO-1 cells with and without IFN-γ sensitization incubated with C. concisus strains both had significantly higher levels of cell death.
Conclusion: C. concisushas the potential to cause damage to both intestinal and esophageal epithelial cells, however, with different pathogenic effects.
Introduction
Campylobacter concisus is a Gram-negative, spiral-shaped bacterium [Citation1]. C. concisus normally colonizes the human oral cavity and behaves as a commensal oral bacterium [Citation2]. However, the presence of C. concisus in other parts of the gastrointestinal tract is associated with gastrointestinal diseases [Citation3]. A higher prevalence of C. concisus in the intestinal tract is associated with inflammatory bowel disease (IBD). IBD is a group of chronic inflammatory diseases of the gastrointestinal tract and a risk factor for colon cancer [Citation4–6]. Crohn’s disease (CD) and ulcerative colitis (UC) are the two major clinical forms of IBD [Citation7]. C. concisus has two genomospecies (GS) [Citation8,Citation9]. Studies found that C. concisus strains associated with IBD are GS2 strains [Citation9–11].
C. concisus is also associated with esophageal diseases [Citation12–14]. Barrett’s esophagus (BE) is a condition occurring at the lower esophagus, in which the normal squamous epithelium is replaced by intestinal-type columnar epithelium (intestinal metaplasia) [Citation15,Citation16]. BE is a complication of gastroesophageal reflux disease (GERD) and a risk factor for esophageal adenocarcinoma [Citation17–19].
Programmed cell death ligand-1 (PD-L1) is a molecule that is expressed by hematopoietic cells and nonhematopoietic cells [Citation20–23]. PD-L1 interacts with its receptor programmed cell death protein-1 (PD-1) expressed on activated T cells, leading to inhibition of T cells [Citation24,Citation25]. The physiological role of the PD-L1 and PD-1 pathway is to prevent excessive immune responses. Some tumor cells express PD-L1 on their surface to evade the attacks from cytotoxic T cells [Citation25–27]. Increased expression of PD-L1 was also detected in intestinal tissues of patients with IBD and esophageal tissues of patients with BE [Citation28–30]
Several cytokines upregulate the expression of PD-L1 such as interferon (IFN)-γ, tumor necrosis factor (TNF)-α, interleukin (IL)-1α and IL-17 [Citation25,Citation31–38]. In addition to cytokines, microbial factors were recently found to upregulate the epithelial expression of PD-L1 [Citation39]. For example, Helicobacter pylori, a bacterium that causes chronic gastric inflammation, increases the gastric epithelial expression of PD-L1, and a commensal Escherichia coli strain K12 increases intestinal epithelial PD-L1 expression in IFN-γ sensitized intestinal epithelial cells [Citation40–42].
We hypothesize that C. concisus, an oral bacterium that is associated with both IBD and BE, has the potential to upregulate the expression of PD-L1 in both intestinal and esophageal epithelial cells and examined this hypothesis using HT-29 and FLO-1 cells as intestinal and esophageal cell culture models, respectively. Interestingly, we found that C. concisus showed different pathogenic effects in intestinal and esophageal epithelial cells.
Materials and methods
Isolation of C. concisus strains from a patient with BE
Currently, C. concisus strains have not been isolated from patients with BE. We, therefore, isolated C. concisus strains from the saliva samples of a patient with BE in this study. Briefly, C. concisus isolation was conducted as previously described [Citation43]. Given that previous studies have found that some individuals are colonized with multiple different C. concisus strains, multiple isolates were collected in this study [Citation9,Citation44]. The bacterial proteins were subjected to sodium dodecyl sulphate-polyacrylamide gel electrophoresis (SDS-PAGE) for the initial determination of the strains as previously described [Citation44]. Isolates with identical SDS-PAGE pattern were defined as the same strain. The putative strains were subjected to genome sequencing for confirmation and identification of genomospecies as described in our previous study [Citation45]. The raw reads were assembled using the St. Petersburg genome assembler to obtain the draft genomes (SPAdes, Ver. 3.15.2) [Citation46]. Genomes of these strains were annotated using the combination of National Center for Biotechnology Information (NCBI) Prokaryotic Genome Annotation Pipeline, Prokka (Ver. 1.11) and Rapid Annotations using Subsystems Technology server (RAST, Ver. 2.0) [Citation47,Citation48]. Genomes assemblies were submitted to NCBI genome database under the Bioproject ID PRJNA735562, the genome accession numbers for C. concisus strains BEO1 and BEO2 were JAHKNB000000000 and JAHKNC000000000 respectively.
C. concisus strains used in this study
A total of four C. concisus strains were used in this study. P2CDO4 and P15UCO-S2 were isolated from patients with IBD in our previous studies [Citation49–51]. P2CDO4 has pICON plasmid and csep1 gene, which were found to be associated CD, and P15UCO-S2 has pSma1 plasmid which was shown to be associated with severe UC [Citation9,Citation11,Citation45]. Strains BEO1 and BEO2 were isolated in this study as described in the previous section.
Maintenance of HT-29 and FLO-1 cells
Human intestinal epithelial cell line HT-29 (ATCC no. HTB-38) and esophageal adenocarcinoma epithelial cell line FLO-1 (ECACC 11012001) were used in this study. HT-29 cells were cultured in McCoy’s 5A medium (Invitrogen, CA, USA) supplemented with 10% fetal bovine serum (Global Life Sciences Solutions, Parramatta, Australia), 100 U/ml penicillin, and 100 U/ml streptomycin (Thermo Fisher Scientific, CA, USA). FLO-1 cells were cultured in Dulbecco’s Modified Eagle Medium (Invitrogen) supplemented with 10% fetal bovine serum (Global Life Sciences Solutions), 2 mM Glutamine (Thermo Fisher Scientific) and 100 U/ml penicillin, and 100 U/ml streptomycin (Thermo Fisher Scientific). The cells were maintained at 37°C in a humidified incubator containing 5% CO2.
Measurement of PD-L1 expression
Quantitative real-time PCR
IFN-γ is known to upregulate PD-L1 expression in tumor tissues, IBD and BE [Citation25,Citation28–34,Citation37,Citation52]. Therefore, IFN-γ was used as the positive control and to sensitize both HT-29 and FLO-1 cells in this study. The concentration of IFN-γ used in this study and incubation time were established in our previous study [Citation42].
HT-29 and FLO-1 cells were cultured in 6-well cell culture plates at a concentration of 2 × 106/well in a complete medium alone or containing 50 ng/ml recombinant human IFN-γ (Roche, Mannheim, Germany) for 12 hours. HT-29 and FLO-1 cells with and without IFN-γ sensitization were incubated with C. concisus strains at a multiplicity of infection (MOI) of 10 and 100 for 4 hours. In order to investigate whether C. concisus strains isolated from a patient with BE (strains BEO1 and BEO2) upregulate PD-L1 mRNA expression in FLO-1 cells following a longer incubation period, FLO-1 cells with and without IFN-γ sensitization were also incubated with strains BEO1 and BEO2 for 24 hours at MOI 100. Cells were washed three times with Dulbecco’s phosphate-buffered saline (DPBS) before collecting for the assessment of PD-L1 expression at the mRNA level. Cells without IFN-γ sensitization and bacterial treatment were used as the negative control.
The levels of PD-L1 mRNA expression in HT-29 cells and FLO-1 cells were quantified using qRT-PCR. RNA was isolated from cells using TRIzol reagent (Invitrogen) following the manufacturer’s instructions. Total RNA (2 μg) was reverse transcribed to cDNA using the Tetro cDNA Synthesis kit (Bioline, NSW, Australia) according to the manufacturer’s instructions. The PD-L1 cDNA was then quantified using SYBR green (Bioline). Primers and the conditions were adapted from our previous study [Citation42]. The levels of PD-L1 mRNA expression were normalized to the levels of glyceraldehyde 3-phosphate dehydrogenase (GAPDH) and expressed as the fold change relative to untreated cells using the comparative threshold cycle CT (2-ΔΔCT) method [Citation53]. Reactions were performed in triplicate and the experiment was repeated three times.
Enzyme-linked immunosorbent assay
The cell culture supernatants of HT-29 and FLO-1 cells treated in the sections above were collected and subjected for measurement of five pro-inflammatory cytokines, including IL-1β, IL-18, IL-6, IL-8, and TNF-α using commercially available ELISA kits (Invitrogen), following the manufacturer’s instructions.
Measurement of cell death
Caspase 3/7 activities in HT-29 and FLO-1 cells incubated with C. concisus strains at MOI 10 and 100 were measured to investigate whether C. concisus strains induces cell death after 4 hours. In addition, caspase 3/7 activities in FLO-1 cells with and without IFN-γ sensitization incubated with C. concisus strains BEO1 and BEO2 at MOI 100 after 24 hours were also measured. Cells (1 × 105/well) were first seeded on black-walled 96-well plates with transparent bottoms for 1 day. Cells were incubated with C. concisus strains at MOI 10 and 100 for 4 hours. For 24 hours, FLO-1 cells with and without IFN-γ sensitization were incubated with BEO1 and BEO2 at MOI 100. Cells without bacterial treatment were used as the negative control and staurosporine-treated (1 μM; Sigma-Aldrich) cells were used as the positive control [Citation54,Citation55]. Caspase 3/7 activities were then measured using the CellEvent Caspase-3/7 Green ReadyProbes reagent (Invitrogen) according to the manufacturer’s instructions. The levels of active caspase 3/7 activity were expressed as fold change relative to the negative control.
Measurement of C. concisus adherence to FLO-1 cells
C. concisus was previously found to adhere to HT-29 cells [Citation56]. The adherence of C. concisus to FLO-1 cells was examined in this study using a previously described gentamicin assay [Citation56]. FLO-1 cells were cultured with and without IFN-γ sensitization as described above, then were incubated with C. concisus strains BEO2 or P2CDO4 at MOI 100. Following 24 hours incubation, FLO-1 cells were washed three times with DPBS and incubated with 200 µg/ml gentamicin (Thermo Fisher Scientific) for 1 hour. Cells were then lysed with 1% Triton X-100 and placed onto HBA plates to grow for 24 hours. The CFU accounts of the same C. concisus strains in FLO-1 cells with and without IFN-γ sensitization were compared.
Statistical analysis
One-way analysis of variance (ANOVA) with Dunnett’s test was performed to compare the levels of PD-L1 mRNA, IL-8 concentrations, and caspase 3/7 activities between different samples. P values less than 0.05 were considered as statistically significant.
Results
Isolation and genome information of C. concisus strains BEO1 and BEO2 from saliva samples of a patient with BE
Protein profile analysis of 20 C. concisus isolates revealed two different patterns, showing that this patient was colonized by two different C. concisus strains. Two strains (BEO1 and BEO2), one from each of the protein profiles, were used for genome sequencing and experiments in this study (Supplementary Figure 1). The genome sizes of strains BEO1 and BEO2 were 1.86 and 1.77 Mb, respectively. BEO1 had 93 contigs and BEO2 had 95 contigs with a fold coverage of 107.26× and 195.66×, respectively. N50 for BEO1 was 37,628 bp and for BEO2 was 29,126 bp. Both strains belonged to GS2.
C. concisus upregulated PD-L1 expression in IFN-γ sensitized HT-29 cells but not in FLO-1 cells
C. concisus alone did not affect PD-L1 expression in both HT-29 cells and FLO-1 cells following 4 hours of incubation. The mRNA levels of PD-L1 in both HT-29 cells and FLO-1 cells incubated with four C. concisus strains at MOI 10 and MOI 100 did not show significant differences in comparison to cells without any treatment (P > 0.05) ().
Figure 1. The effects of C. concisus strains on PD-L1 mRNA expression in HT-29 cells and FLO-1 cells after 4 hours. HT-29 cells (A) or FLO-1 cells (B) with and without IFN-γ sensitization were incubated with C. concisus strains (P2CDO4, P15UCO-S2, BEO1 or BEO2) at MOI 10 or 100 for 4 hours. PD-L1 mRNA expressions were measured by qRT-PCR. IFN-γ sensitized HT29 cells were used as the positive control and untreated cells were used as the negative control. The straight-line bar indicates significance test compared with IFN-γ sensitized cells. One-way analysis of variance (ANOVA) with Dunnett’s test was performed. Graphs are representative of averages of triplicate experiments ± standard error (* = P < 0.05; ** = P < 0.01; **** = P < 0.0001 indicates statistical significance). MOI: multiplicity of infection
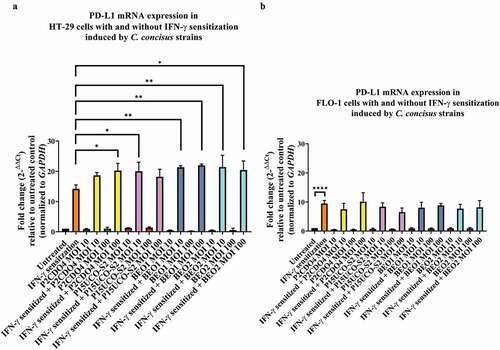
In HT-29 cells sensitized with IFN-γ, C. concisus strains P15UCO-S2, BEO1 and BEO2 at MOI 10 induced a significantly higher expression of PD-L1 mRNA compared to IFN-γ sensitized HT-29 cells without any bacterial treatment (P < 0.05, P < 0.01, and P < 0.01 respectively). The fold changes of PD-L1 mRNA levels in cells incubated with these strains were 20.01 ± 2.12, 21.33 ± 0.34 and 21.39 ± 2.78, respectively (). At MOI 100, strains P2CDO4, BEO1 and BEO2 induced a significantly higher expression of PD-L1 in IFN-γ sensitized HT-29 cells compared to IFN-γ sensitized HT-29 cells without any bacterial treatment (P < 0.05, P < 0.01, and P < 0.05 respectively). The fold changes of PD-L1 mRNA levels in HT-29 cells incubated with these strains were 20.25 ± 1.68, 21.98 ± 0.30 and 20.38 ± 2.16, respectively ().
In FLO-1 cells, IFN-γ sensitization significantly increased PD-L1 mRNA expression (P < 0.0001) (). However, C. concisus strains did not significantly affect the PD-L1 mRNA expression in IFN-γ sensitized FLO-1 cells. The levels of PD-L1 mRNA in IFN-γ sensitized FLO-1 cells incubated with four C. concisus strains at MOI 10 and MOI 100 were not significantly different from IFN-γ sensitized FLO-1 cells without bacterial treatment (P > 0.05).
C. concisus strains did not induce apoptosis in both HT-29 cells and FLO-1 cells following 4 hours of incubation. The caspase 3/7 activities in HT-29 cells and FLO-1 cells did not show a significant change after 4 hours of incubation with the four C. concisus strains (Supplementary Figure 2).
C. concisus induced IL-8 production in HT-29 cells but not in FLO-1 cells
The IL-8 concentrations in the supernatants of HT-29 cells incubated with C. concisus strains P2CDO4 and P15UCO-S2 at MOI 10 and P2CDO4 at MOI 100 for 4 hours were 183.78 ± 6.45, 169.71 ± 6.19 and 183.84 ± 7.66 pg/ml, respectively. These concentrations were higher than the IL-8 concentration of the untreated HT-29 cells (151.12 ± 5.77 pg/ml), however, it did not reach a significant difference (P > 0.05) (). HT-29 cells incubated with BEO1 and BEO2 at MOI 10 induced a significantly higher production of IL-8 as compared to untreated HT-29 cells. The concentrations of HT-29 cells incubated with BEO1 and BEO2 at MOI 10 were 211.25 ± 14.30 and 253.30 ± 6.04 pg/ml respectively (P < 0.05 and P < 0.0001 respectively). At MOI 100, strains P15UCO-S2, BEO1 and BEO2 induced a significantly higher production of IL-8 as compared to untreated HT-29 cells, with the concentrations being 231.11 ± 37.20, 343.24 ± 9.85, and 320.58 ± 13.06 pg/ml, respectively (P < 0.01, P < 0.0001 and P < 0.0001 respectively). We also measured IL-1β, IL-18, IL-6, and TNF-α but the levels of these cytokines were below the detection level (data not shown).
Figure 2. IL-8 production by HT-29 cells with and without IFN-γ sensitization induced by C. concisus strains after 4 hours.Concentrations of IL-8 in the cell culture supernatants of HT-29 cells without (A) and with IFN-γ (B) sensitization were measured by ELISA after 4 hours of incubation with C. concisus strains (P2CDO4, P15UCO-S2, BEO1 or BEO2). The dash-line bar indicates significance test compared with untreated HT-29 cells and the straight-line bar indicates significance test compared with IFN-γ sensitized HT-29 cells. One-way analysis of variance (ANOVA) with Dunnett’s test was performed. Graphs are representative of averages of triplicate experiments ± standard error (* = P < 0.05; ** = P < 0.01; **** = P < 0.0001 indicates statistical significance). MOI: multiplicity of infection
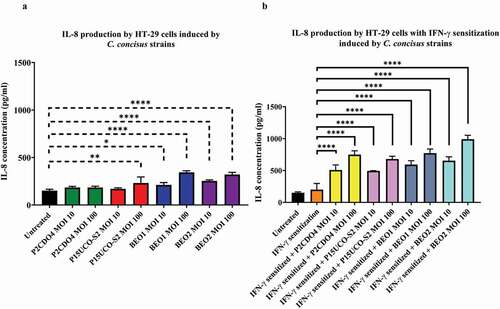
IFN-γ sensitization has greatly enhanced the response of HT-29 cells to C. concisus in the production of IL-8. In IFN-γ sensitized HT-29 cells, the IL-8 concentrations in the supernatants of HT-29 cells incubated with C. concisus strains P2CDO4, P15UCO-S2, BEO1 and BEO2 at MOI 10 were 507.38 ± 46.47, 492.61 ± 3.88, 591.32 ± 36.05 and 653.39 ± 34.92 pg/ml respectively, which were significantly different from that in the supernatant of IFN-γ sensitized HT-29 cells without bacterial treatment (P < 0.0001) (). The levels of IL-8 induced by the four C. concisus strains at MOI 100 were even higher, being 748.26 ± 34.90, 679.25 ± 25.46, 770.98 ± 37.99 and 989.43 ± 36.35 pg/ml respectively (P < 0.0001) (). The levels of IL-1β, IL-18, IL-6, and TNF-α in IFN-γ sensitization with and without bacterial treatment were below deletion levels (data not shown). C. concisus strains did not induce the production of the cytokines measured in this study in FLO-1 cells, with or without IFN-γ sensitization.
C. concisus strains BEO1 and BEO2 induced cell death in FLO-1 cells after 24 hours of incubation
Given that C. concisus strains did not show significant effects on PD-L1 expression, cytokine production and apoptosis in FLO-1 cells after 4 hours of incubation, we conducted further experiments to investigate these effects after 24 hours of incubation of C. concisus with FLO-1 cells. For these experiments, strains BEO1 and BEO2 at MOI 100 were used to incubate FLO-1 cells.
C. concisus strains BEO1 and BEO2 alone did not significantly affect PD-L1 expression in FLO-1 cells after 24 hours of incubation. The levels of PD-L1 mRNA in FLO-1 cells without IFN-γ sensitization incubated with C. concisus strains BEO1 and BEO2 for 24 hours were not significantly different from that in FLO-1 cells without bacterial treatment (P > 0.05). (). In IFN-γ sensitized FLO-1 cells, both C. concisus strains significantly inhibited the level of PD-L1 induced by IFN-γ. The mRNA level of PD-L1 in IFN-γ sensitized FLO-1 cells incubated with BEO1 was 1.52 ± 0.08, which was significantly lower than the IFN-γ sensitized FLO-1 cells without bacterial treatment (9.61 ± 0.65) (P < 0.0001). The mRNA level of PD-L1 in IFN-γ sensitized FLO-1 cells incubated with BEO2 was 1.82 ± 0.05, which was also significantly lower than the IFN-γ sensitized FLO-1 cells without bacterial treatment (P < 0.0001) ().
Figure 3. PD-L1 mRNA expression and caspase 3/7 activities in FLO-1 cells with and without IFN-γ sensitization induced by C. concisus BEO1 and BEO2 at MOI 100 after 24 hours. (A) The mRNA levels of PD-L1 in FLO-1 cells were measured using qRT-PCR. (B) The apoptotic effect of BEO1 and BEO2 on FLO-1 cells were determined by measuring caspase 3/7 levels using CellEvent™ caspase 3/7 green detection reagent. Staurosporine (STS) treated FLO-1 cells were used as the positive control. One-way analysis of variance (ANOVA) with Dunnett’s test was performed. Graphs are representative of averages of triplicate experiments ± standard error (* = P < 0.05; ** = P < 0.01; *** = P < 0.001 **** = P < 0.0001 indicates statistical significance). MOI: multiplicity of infection
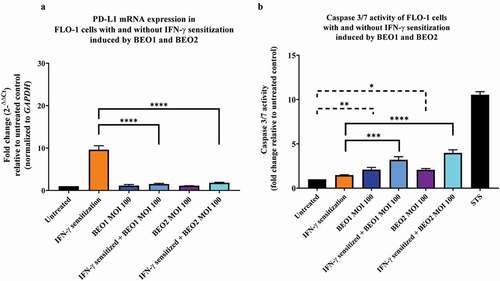
Interestingly, while C. concisus strains BEO1 and BEO2 inhibited PD-L1 expression in IFN-γ sensitized FLO-1 cells, they induced apoptosis in these cells. The levels of caspase 3/7 activity in IFN-γ sensitized FLO-1 cells incubated with BEO1 and BEO2 were 3.20 ± 0.20 and 3.98 ± 0.25 respectively which were significantly higher than that of the IFN-γ sensitized FLO-1 cells (P < 0.001 and P < 0.0001 respectively) (). Furthermore, FLO-1 cells incubated with BEO1 (2.05 ± 0.14) and BEO2 (2.07 ± 0.10) had significantly higher levels of caspase 3/7 activity than that of the untreated cells (P < 0.01 and P < 0.05 respectively). There was no detectable cytokine production in the supernatants of FLO-1 cells incubated with strains BEO1 and BEO2 after 24 hours.
IFN-γ sensitization increased C. concisus adhesion to FLO-1 cells
Both C. concisus strains BEO2 and P2CDO4 significantly increased adhesion to IFN-γ sensitized FLO-1 cells as compared to FLO-1 cells without IFN-γ sensitization (P < 0.05 and P < 0.01 respectively). In both strains, the adhesion increased more than two folds in IFN-γ-sensitized FLO-1 cells (Supplementary Figure 3).
Discussion
In this study, we examined the effects of C. concisus strains on PD-L1 mRNA expression, pro-inflammatory cytokine production and caspase 3/7 activities in intestinal and esophageal epithelial cells using HT-29 and FLO-1 as the cell culture models.
In a local environment without the presence of IFN-γ, the predominant role of C. concisus on intestinal epithelial cells was to induce acute inflammation. Production of IL-8 by HT-29 cells was observed after a short period (4 hours) of incubation with C. concisus but PD-L1 mRNA expression was not significantly changed (). Sufficient numbers of bacteria are required to induce the production of IL-8 in HT-29 cells, as C. concisus strains induced a significantly higher IL-8 production at MOI 100 (). IL-8 is a key inflammatory cytokine involved in the recruitment of neutrophils to the infection site [Citation57]. These results show that C. concisus has the potential to induce acute inflammation in the intestinal tract without the need of establishing long-term enteric colonization as long as sufficient C. concisus bacterial cells are translocated to the intestinal tract from the oral cavity. C. concisus is a spiral to curved shape microorganism with a flagellum and this structure may have provided the bacteria capability to cross the intestinal mucus layer and contact epithelial cells [Citation1,Citation56].
C. concisus strains induced a higher IL-8 production and significantly upregulated the expression of PD-L1 mRNA in IFN-γ sensitized intestinal epithelial cells. The threshold of bacterial numbers required for C. concisus to induce epithelial production of IL-8 in IFN-γ sensitized HT-29 cells was greatly reduced as compared to HT-29 cells without IFN-γ treatment. All C. concisus strains induced a significantly higher production of IL-8 at MOI 10 and the levels of IL-8 induced by C. concisus strains in IFN-γ sensitized HT-29 cells were greatly higher than that in HT-29 cells with IFN-γ sensitization (). A previous study has shown that IFN-γ upregulates intracellular Toll-like receptor-4-MD-2 complex, which may have played a part in contributing to the upregulated response of IL-8 production induced by C. concisus in IFN-γ sensitized HT-29 cells [Citation58]. Interestingly, C. concisus strains also significantly upregulated the expression of PD-L1 mRNA in IFN-γ sensitized HT-29 cells. PD-L1 is an immune checkpoint protein and the binding of PD-L1 to its receptor on T cells delivers an inhibitory signal, which is a mechanism by which the immune system limits immune-mediated tissue damages [Citation24,Citation25,Citation35]. In chronic inflammatory conditions such as IBD, PD-L1 expression including epithelial PD-L1 expression is upregulated [Citation28]. Prolonged upregulation of PD-L1 expression provides opportunities for pre-cancer and cancer cells to evade the attacks from the immune system, which may contribute to an increased risk of developing cancer associated with chronic inflammation. Our findings in this study suggest that in patients with chronic enteric inflammation such as IBD, IBD-associated oral bacterium C. concisus may further increase the enteric inflammation via enhancing the innate inflammatory immune response and also have the potential to inhibit the functions of effector T cells via upregulating epithelial PD-L1. In this study, we have examined PD-L1 mRNA expression. Future studies examining the effects of C. concisus on PD-L1 protein expression in IFN-γ sensitized HT-29 cells and the induced PD-L1 protein on the functions of effector T cells such as proliferation, cytokine productions, and cytotoxicity could further explore the possible role of C. concisus in immune evasion.
The effects of C. concisus on esophageal cells were different from that on intestinal epithelial cells. C. concisus did not induce production of IL-8 in FLO-1 cells following 4 hours of incubation at both MOI 10 and MOI 100. The bacterium also did not significantly affect PD-L1 mRNA expression in FLO-1 cells with and without IFN-γ sensitization after 4 hours of incubation (). C. concisus did not significantly change the caspase 3/7 activities in both FLO-1 cells and HT-29 cells after 4 hours of incubation. Therefore, apoptosis was not the reason why C. concisus did not significantly affect PD-L1 mRNA expression in FLO-1 cells after 4 hours (Supplementary Figure 2). Following incubation of C. concisus with FLO-1 cells with and without IFN-γ sensitization for 24 hours, there was still no IL-8 production by FLO-1 cells. We also measured IL-1β, IL-18, IL-6, and TNF-α and these were all below the detection level (data not shown). It appears that FLO-1 cells do not secret these common pro-inflammatory cytokines in response to C. concisus incubation. Interestingly, C. concisus significantly inhibited PD-L1 mRNA expression in IFN-γ sensitized FLO-1 cells (). Such a reduction of PD-L1 was due to cell death induced by C. concisus (). Previous studies detected increased C. concisus in esophageal tissues and increased cytokines [Citation12–14]. Our finding that C. concisus induced apoptosis in FLO-1 with and without IFN-γ sensitization suggests that C. concisus may contribute to esophageal disease by damaging the epithelial barrier. Interestingly, such damage requires C. concisus to contact esophageal cells for sufficient time as apoptosis occurred in FLO-1 cells incubated with C. concisus for 24 hours but not in FLO-1 cells incubated with C. concisus for 4 hours. IFN-γ sensitization of FLO-1 cells resulted in a two-fold increase of C. concisus adhesion but no invasion was observed (Supplementary Figure 3), further supporting that adhesion of C. concisus to FLO-1 cells is required for causing epithelial damage.
Cell lines including HT-29 and FLO-1 have been used as in vitro models in this study. While cell line models provide the ease of cultivation and the consistency of results produced, they are tumor-derived cells and lack of the normal architecture of intestinal or esophageal epithelium. Future studies using human intestinal and esophageal organoids may provide further information more resembling the human physiological conditions.
In summary, in this study, we found that C. concisus caused different pathogenic effects in intestinal and esophageal epithelial cells. C. concisus induced IL-8 production in intestinal epithelial HT-29 cells without IFN-γ sensitization. In IFN-γ sensitized HT-29 cells, induction of IL-8 by C. concisus was enhanced and PD-L1 mRNA expression was upregulated. C. concisus induced apoptosis in esophageal epithelial FLO-1 cells and IFN-γ sensitization enhanced C. concisus induced cell death in FLO-1 cells. These data suggest a role that C. concisus has the potential to cause damage to both intestinal and esophageal epithelial cells, however, with different pathogenic effects.
Author contributions
S.A.L. played a major role in performing the experiments and writing the manuscript. S.A.L. and F.L. performed genome analysis. D.Y.Y. contributed to the setup of initial experimental conditions. A.C.Y.T. performed genome sequencing. F.L., S.M.R., A. C. Y. T., C. S. L., and L.L. provided important feedback and helped in editing the manuscript. S.M.R. collected the saliva sample for isolation of BEO1 and BEO2 strains. L.Z. conceived the project and played an important role in editing the manuscript. All authors have approved the final version of the manuscript.
Ethical statement
Procedures for saliva collection from the patient were approved by the Ethics Committees of the University of New South Wales and the South East Sydney Local Health District, Australia (HREC/17/ POWH/173). A completed consent form regarding saliva collection was obtained from the patient.
Supplemental Material
Download Zip (720.1 KB)Disclosure statement
No potential conflict of interest was reported by the author(s).
Supplementary material
Supplemental data for this article can be accessed here.
Additional information
Funding
References
- Lastovica AJ, On S, Zhang L. The family Campylobacteraceae. Springer-, Berlin, Heidelberg. 2014.
- Zhang L. Campylobacter concisus and inflammatory bowel disease. World J Gastroenterol. 2014;20(5):1259.
- Liu F, Ma R, Wang Y, et al. The clinical importance of Campylobacter concisus and other human hosted Campylobacter species. Front Cell Infect Microbiol. 2018;8:243.
- Bernstein CN, Blanchard JF, Kliewer E, et al. Cancer risk in patients with inflammatory bowel disease: a population‐based study. Cancer. 2001;91(4):854–10.
- Jensen AB, Larsen M, Gislum M, et al. Survival after colorectal cancer in patients with ulcerative colitis: a nationwide population-based Danish study. Am J Gastroenterol. 2006;101(6):1283–1287.
- Taylor CC, Millien VO, Hou JK, et al. Association Between Inflammatory Bowel Disease and Colorectal Cancer Stage of Disease and Survival. J Surg Res. 2020;247:77–85.
- Kaplan GG. The global burden of IBD: from 2015 to 2025. Nat Rev Gastroenterol Hepatol. 2015;12(12):720–727.
- On SLW, Zhang J, Cornelius AJ, et al. Markers for discriminating Campylobacter concisus genomospecies using MALDI-TOF analysis. Curr Res Microb Sci. 2021;2:100019.
- Liu F, Ma R, Tay CYA, et al. Genomic analysis of oral Campylobacter concisus strains identified a potential bacterial molecular marker associated with active Crohn’s disease. Emerg Microbes Infect. 2018;7:1–14.
- Wang Y, Liu F, Zhang X, et al. Campylobacter concisus genomospecies 2 is better adapted to the human gastrointestinal tract as compared with Campylobacter concisus genomospecies 1. Front Physiol. 2017;8:543.
- Liu F, Chen S, Luu LDW, et al. Analysis of complete Campylobacter concisus genomes identifies genomospecies features, secretion systems and novel plasmids and their association with severe ulcerative colitis. Microb Genom. 2020;6(11).
- Macfarlane S, Furrie E, Macfarlane GT, et al. Microbial colonization of the upper gastrointestinal tract in patients with Barrett’s esophagus. Clin Infect Dis. 2007;45(1):29–38.
- Blackett KL, Siddhi SS, Cleary S, et al. Oesophageal bacterial biofilm changes in gastro-oesophageal reflux disease, Barrett’s and oesophageal carcinoma: association or causality? Aliment Pharmacol Ther. 2013;37(11):1084–1092.
- Mozaffari Namin B, Soltan Dallal MM, Ebrahimi Daryani N. The Effect of Campylobacter concisus on Expression of IL-18, TNF-α and p53 in Barrett’s Cell Lines. Jundishapur J Microbiol. 2015;8(12):e26393–e93.
- Barrett NR. The lower esophagus lined by columnar epithelium. Surgery. 1957;41:881–894.
- Shaheen NJ, Richter JE. Barrett’s oesophagus. Lancet. 2009;373(9666):850–861.
- Runge TM, Abrams JA, Shaheen NJ. Epidemiology of Barrett’s Esophagus and Esophageal Adenocarcinoma. Gastrointest Endosc Clin N Am. 2015;44(2):203–231.
- Cook MB, Wild CP, Forman D. A Systematic Review and Meta-Analysis of the Sex Ratio for Barrett’s Esophagus, Erosive Reflux Disease, and Nonerosive Reflux Disease. Am J Epidemiol. 2005;162(11):1050–1061.
- Hvid-Jensen F, Pedersen L, Drewes AM, et al. Incidence of adenocarcinoma among patients with Barrett’s esophagus. N Engl J Med. 2011;365(15):1375–1383.
- Mueller SN, Vanguri VK, Ha S-J, et al. PD-L1 has distinct functions in hematopoietic and nonhematopoietic cells in regulating T cell responses during chronic infection in mice. J Clin Investig. 2010;120(7):2508–2515.
- Guan J, Lim KS, Mekhail T, et al. Programmed Death Ligand-1 (PD-L1) Expression in the Programmed Death Receptor-1 (PD-1)/PD-L1 Blockade: a Key Player Against Various Cancers. Arch Pathol Lab Med. 2017;141(6):851–861.
- Dong H, Chen L. B7-H1 pathway and its role in the evasion of tumor immunity. J Mol Med (Berl). 2003;81:281–287.
- Keir ME, Butte MJ, Freeman GJ, et al. PD-1 and its ligands in tolerance and immunity. Annu Rev Immunol. 2008;26(1):677–704.
- Butte MJ, Keir ME, Phamduy TB, et al. Programmed death-1 ligand 1 interacts specifically with the B7-1 costimulatory molecule to inhibit T cell responses. Immunity. 2007;27(1):111–122.
- Dong H, Strome SE, Salomao DR, et al. Tumor-associated B7-H1 promotes T-cell apoptosis: a potential mechanism of immune evasion. Nat Med. 2002;8(8):793–800.
- Mühlbauer M, Fleck M, Schütz C, et al. PD-L1 is induced in hepatocytes by viral infection and by interferon-alpha and -gamma and mediates T cell apoptosis. J Hepatol. 2006;45(4):520–528.
- Brown JA, Dorfman DM, Ma FR, et al. Blockade of programmed death-1 ligands on dendritic cells enhances T cell activation and cytokine production. J Immunol. 2003;170(3):1257–1266.
- Rajabian Z, Kalani F, Taghiloo S, et al. Over-Expression of Immunosuppressive Molecules, PD-L1 and PD-L2, in Ulcerative Colitis Patients. Iran J Immunol. 2019;16:62–70.
- Van Der Kraak L, Goel G, Ramanan K, et al. 5-Fluorouracil upregulates cell surface B7-H1 (PD-L1) expression in gastrointestinal cancers. J Immunother Cancer. 2016;4(1):65.
- Derks S, Nason KS, Liao X, et al. Epithelial PD-L2 Expression Marks Barrett’s Esophagus and Esophageal Adenocarcinoma. Cancer Immunol Res. 2015;3(10):1123–1129.
- Ishida M, Iwai Y, Tanaka Y, et al. Differential expression of PD-L1 and PD-L2, ligands for an inhibitory receptor PD-1, in the cells of lymphohematopoietic tissues. Immunol Lett. 2002;84(1):57–62.
- Curiel TJ, Wei S, Dong H, et al. Blockade of B7-H1 improves myeloid dendritic cell–mediated antitumor immunity. Nat Med. 2003;9(5):562–567.
- Chen S, Crabill GA, Pritchard TS, et al. Mechanisms regulating PD-L1 expression on tumor and immune cells. J Immunother Cancer. 2019;7(1):305.
- Yamazaki T, Akiba H, Iwai H, et al. Expression of programmed death 1 ligands by murine T cells and APC. J Immunol. 2002;169(10):5538–5545.
- Chen L. Co-inhibitory molecules of the B7–CD28 family in the control of T-cell immunity. Nat Rev Immunol. 2004;4(5):336–347.
- Ou J-N, Wiedeman AE, Stevens AM. TNF-α and TGF-β counter-regulate PD-L1 expression on monocytes in systemic lupus erythematosus. Sci Rep. 2012;2(1):295.
- Kondo A, Yamashita T, Tamura H, et al. Interferon-γ and tumor necrosis factor-α induce an immunoinhibitory molecule, B7-H1, via nuclear factor-κB activation in blasts in myelodysplastic syndromes. Blood. 2010;116(7):1124–1131.
- Wang X, Yang L, Huang F, et al. Inflammatory cytokines IL-17 and TNF-α up-regulate PD-L1 expression in human prostate and colon cancer cells. Immunol Lett. 2017;184:7–14.
- Chulkina M, Beswick EJ, Pinchuk IV. Role of PD-L1 in Gut Mucosa Tolerance and Chronic Inflammation. Int J Mol Sci. 2020;21(23):9165.
- Wu -Y-Y, Lin C-W, Cheng K-S, et al. Increased programmed death-ligand-1 expression in human gastric epithelial cells in Helicobacter pylori infection. Clin Exp Immunol. 2010;161(3):551–559.
- Holokai L, Chakrabarti J, Broda T, et al. Increased Programmed Death-Ligand 1 is an Early Epithelial Cell Response to Helicobacter pylori Infection. PLoS Pathog. 2019;15(1):e1007468.
- Lee SA, Wang Y, Liu F, et al. Escherichia coli K12 Upregulates Programmed Cell Death Ligand 1 (PD-L1) Expression in Gamma Interferon-Sensitized Intestinal Epithelial Cells via the NF-κB Pathway. Infect Immun. 2020;89(1):e00618-20.
- Zhang L, Budiman V, Day AS, Zhang L, Budiman V, Day AS, Mitchell H, Lemberg DA, Riordan SM, et al. Isolation and Detection of Campylobacter concisus from Saliva of Healthy Individuals and Patients with Inflammatory Bowel Disease. J Clin Microbiol. 2010;48(8):2965–2967.
- Ismail Y, Mahendran V, Octavia S, Day AS, Riordan SM, Grimm MC, et al. Investigation of the enteric pathogenic potential of oral Campylobacter concisus strains isolated from patients with inflammatory bowel disease. PloS One. 2012;7:e38217.
- Chung HKL, Tay A, Octavia S, et al. Genome analysis of Campylobacter concisus strains from patients with inflammatory bowel disease and gastroenteritis provides new insights into pathogenicity. Sci Rep. 2016;6(1):1–14.
- Bankevich A, Nurk S, Antipov D, et al. SPAdes: a new genome assembly algorithm and its applications to single-cell sequencing. J Comput Biol. 2012;19(5):455–477.
- Tatusova T, DiCuccio M, Badretdin A, et al. NCBI prokaryotic genome annotation pipeline. Nucleic Acids Res. 2016;44(14):6614–6624.
- Aziz RK, Bartels D, Best AA, et al. The RAST Server: rapid annotations using subsystems technology. BMC Genomics. 2008;9(1):75.
- Zhang L, Man SM, Day AS, et al. Detection and Isolation of Campylobacter Species Other than C. jejuni from Children with Crohn’s Disease. J Clin Microbiol. 2009;47(2):453–455.
- Mahendran V, Riordan SM, Grimm MC, et al. Prevalence of Campylobacter Species in Adult Crohn’s Disease and the Preferential Colonization Sites of Campylobacter Species in the Human Intestine. PloS One. 2011;6(9):e25417.
- Mahendran V, Octavia S, Demirbas OF, et al. Delineation of genetic relatedness and population structure of oral and enteric Campylobacter concisus strains by analysis of housekeeping genes. Microbiology. 2015;161(8):1600–1612.
- Konishi J, Yamazaki K, Azuma M, et al. B7-H1 Expression on Non-Small Cell Lung Cancer Cells and Its Relationship with Tumor-Infiltrating Lymphocytes and Their PD-1 Expression. Clin Cancer Res. 2004;10(15):5094–5100.
- Livak KJ, Schmittgen TD. Analysis of Relative Gene Expression Data Using Real-Time Quantitative PCR and the 2−ΔΔCT Method. Methods. 2001;25(4):402–408.
- Belmokhtar CA, Hillion J, Ségal-Bendirdjian E. Staurosporine induces apoptosis through both caspase-dependent and caspase-independent mechanisms. Oncogene. 2001;20(26):3354–3362.
- Qiao L, Koutsos M, Tsai LL, et al. Staurosporine inhibits the proliferation, alters the cell cycle distribution and induces apoptosis in HT-29 human colon adenocarcinoma cells. Cancer Lett. 1996;107(1):83–89.
- Man SM, Kaakoush NO, Leach ST, et al. Host Attachment, Invasion, and Stimulation of Proinflammatory Cytokines by Campylobacter concisus and Other Non– campylobacter jejuni Campylobacter Species. J Infect Dis. 2010;202(12):1855–1865.
- Remick DG. Interleukin-8. Crit Care Med. 2005;33(12):S466-S467.
- Suzuki M, Hisamatsu T, Podolsky DK. Gamma interferon augments the intracellular pathway for lipopolysaccharide (LPS) recognition in human intestinal epithelial cells through coordinated up-regulation of LPS uptake and expression of the intracellular Toll-like receptor 4-MD-2 complex. Infect Immun. 2003;71(6):3503–3511.